- Bauhaus-Institute for Infrastructure Solutions (b.is), Bauhaus-Universität Weimar, Weimar, Germany
Antimicrobial resistances (AMR) are ranked among the top ten threats to public health and societal development worldwide. Toilet wastewater contained in domestic wastewater is a significant source of AMR entering the aquatic environment. The current commonly implemented combined sewer systems at times cause overflows during rain events, resulting in the discharge of untreated wastewater into the aquatic environment, thus promoting AMR. In this short research article, we describe an approach to transform combined sewer systems into source separation-modified combined sewer systems that separately treat toilet wastewater. We employ simulations for demonstrating that source separation-modified combined sewer systems reduce the emission of AMR-causing substances by up to 11.5 logarithm levels. Thus, source separation-modified combined sewer systems are amongst the most effective means of combating AMR.
1. Introduction
Antimicrobial resistance (AMR) is ranked by the World Health Organization (WHO) as one of the top 10 global problems of our time (1). The consequences of spreading AMR are increased mortality and heightened rates of morbidity (2). The economic impact alone is considerable: for example, Taylor et al. (3) assume an annual reduction in gross national product of 0.14% after 40 years owing to AMR, assuming—optimistically but not likely—that AMR remains constant.
A number of measures are proposed for reducing AMRs (4). In addition to a reduction of antibiotics in agriculture, wastewater treatment plants (WWTPs) in particular are mentioned as a means of treating wastewater and thus as a focus of current research on reducing AMR emissions (5–10). Approximately half of antibiotics are used in human medicine (4) and finally end up in wastewater via human feces. Accordingly, domestic wastewater should be considered an important entry pathway of AMR into the environment. Hence, it is purposeful considering possible wastewater treatment measures for reducing the emission of AMRs.
In wastewater treatment, sanitation systems that use central WWTPs (“end-of-pipe”) are predominant. Wastewater with varying degrees of contamination, for example from showers and kitchens (“graywater”) and from toilets (“blackwater”), is combined, transported by sewer, and subjected to joint treatment in central WWTPs. Source separation has been proposed for some time to optimize these measures (11). The principle of source separation has proven itself in waste management with the advantages of carrying out targeted source-specific treatment and ultimately recovering resources, such as glass, paper or compost (12, 13). In wastewater treatment, source separation refers to the separate collection, discharge, and treatment of graywater and blackwater, recovering energy, nutrients, biomass and process water.
Antibiotics used in human medicine and their degradation products are found in toilet wastewater, blackwater, particularly via human feces. If the blackwater enters the aquatic environment, the contained antibiotics (AB), their degradation products, antibiotic-resistant bacteria (ARB), and antibiotic-resistant genes (ARG) promote the spread and emergence of AMR. Simplistically, therefore, AMR are characterized by the levels of AB, ARB, and ARG. Blackwater contains nearly 100% of the loads of AB, ARB, and ARG of domestic wastewater (14). The loads of blackwater and harmful constituents, such as AB, ARB, and ARG, released into the aquatic environment are significantly influenced by the type of sanitation system.
1.1. Combined system (CS)
Combined systems transport all wastewater together with stormwater in a single sewer system to the WWTP, where wastewater treatment is carried out. CSs are the most prevalent among conventional sanitation systems, it is estimated that 70% of sewers in Europe belong to CSs (15). A typical characteristic of CSs are Combined Sewer Overflows (CSOs): when the sewer system is overloaded by heavy rain, part of the wastewater is released untreated—including the load of AMR—into water bodies (16–19).
1.2. Separate system (SES)
Separate systems discharge wastewater and stormwater in separate sewer systems. SESs are to be considered an improvement over CSs based on current technology. As a result, high volumes of stormwater in separate systems generally do not cause sewer overflows. Depending on the misconnection rate, small emissions of AMR could occur to the aquatic environment from untreated stormwater with wastewater components.
1.3. Entry pathways
CSOs are a major entry pathway of AMR into the aquatic environment. The second entry pathway is the wastewater treatment plant, which only reduces AMR but does not remove it completely. Efforts are currently being made to increase the efficiency of AMR removal at the WWTP through a so-called fourth treatment stage.
1.4. Source separation-modified combined system (SMCS)
Using the principles of source separation, a so-called source-modified combined system is proposed to be developed from the existing CS. In a SMCS (Figure 1), the toilet wastewater is collected separately as blackwater, conveyed to the modified WWTP and processed in an upflow anaerobic sludge blanket (UASB) digestion reactor. Compared to other digestion processes, the UASB reactor produces two streams—digestate and liquid phase—, which can be processed separately. The digestate is dewatered and incinerated, the liquid phase is subjected to phosphorus precipitation, nitrogen recovery by ammonia stripping or deammonification, and thermal disinfection, which ensures the elimination of AMR to the greatest possible extent. The liquid phase treated in this way is thereafter returned to the WWTP, which is now dedicated to the treatment of graywater, extraneous water and unavoidable rainwater. The effluent treated is passed once again through an Advanced Oxidation Process (AOP) stage and subjected to ultrafiltration, which further reduces AMR.
Consequently, a few measures are necessary to transfer existing CSs into SMCSs: The blackwater has to be collected separately, which may be achieved, for example, by retrofittable inliners in the wastewater pipes of the building (20) or by sensor-controlled wastewater switches that are to be installed in the two-chamber inspection shaft outside of the building and that divert the resulting wastewater into receiving tanks for blackwater and graywater, depending on the actual wastewater type (21). Furthermore, the blackwater is to be transported to the UASB reactor to be built on the WWTP. A vacuum system is foreseen for this transport. Also, to be built at the WWTP is the treatment section upstream and downstream of the UASB (Figure 1), such as the treatment facilities for Advanced Oxidation Processes (AOP). The AOP adopted in this study is ozonation. The capacity required for the treatment of the liquid phase of the UASB, e.g., thermal disinfection, is reduced by a factor of 3 compared to a wastewater system without source separation.
1.5. Sanitation system comparison
For comparing the AMR emissions of the proposed source separation-modified mixed system (SMCS) with the AMR emissions of CSs and SESs, we assume that CS and SESs are each supplemented with a fourth treatment stage to adjust them up to the prognosed future state of the technology with the goal of AMR reduction. The assumed fourth treatment stage consists of an ultrafiltration. A CS not equipped with a fourth treatment stage is selected for representing the current state of technology as a baseline (Table 1).
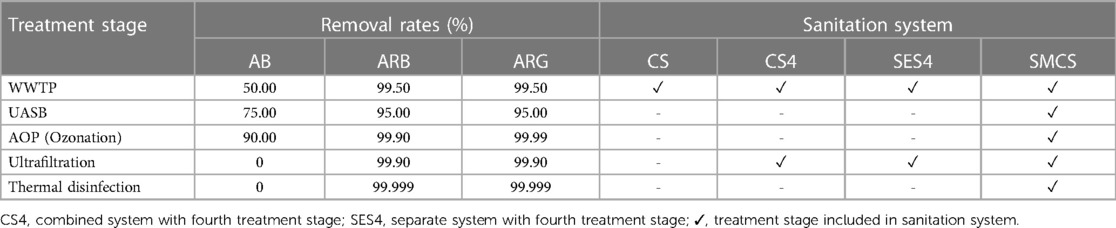
Table 1. Removal rates per treatment stage and mapping of treatment stages to sanitation systems under study.
2. Methods
For comparing the AMR emissions of the selected four sanitation systems into the aquatic environment, a simulation using the software SAmpSONS (22), which is specialized in assessment of source separation-based sanitation systems, was used. The model area of an established German standard for urban sanitation was used as the area for which the four selected sanitation systems were modeled in each case (23). The model area was developed aiming at representativeness for sanitation systems in existing buildings and consists of various differently equipped sub-areas. Therefore, it is also considered suitable for comparing the four sanitation systems. Further variables defined for the simulation models are the selection of representative proxy substances for AB, ARB and ARG and the removal rates in the treatment processes applied. Sulfamethoxazole is used as a proxy for AB because it is widely applied and data availability for levels and removal rates is also high. As a proxy for ARB, extended-spectrum betalactamase E. coli (ESBL-E. coli) is used, which a number of antibiotics are ineffective against. Again, the data availability is acceptable. For ARG, blaTEM is used because it is one of the most frequently genes found in wastewater (24). The parameters of the simulation are detailed in (25). Also included in (25) is a derivation of the assumed levels, loads, and removal rates with respect to the proxy substances. In contrast to the parameters described in (25), CS and SES were each equipped with a fourth treatment stage referencing to the most likely approach of wastewater treatment being established in future. The removal rates applied in the simulation are listed in Table 1. Removal rates for ultrafiltration are defined based on (7, 26, 27). The simulations may be reproduced using the SAmpSONS software (22) and the configuration files provided in the Supplementary Material.
3. Results
The loads of the three proxy substances emitted to the aquatic environment by each of the four sanitation systems, as determined by simulation, are presented in Table 2 (rows #2—#5). Row #1 denotes the loads contained in wastewater.
Table 3 provides the comparison of the removal rates of the systems achieved in the simulation. The comparison of SMCS and CS4 also shows an improvement of 1.7 logarithm levels regarding AB. Significant improvements of 10.5 and 11.5 logarithm levels are also observed for ARB and ARG due to the implementation of SMCS. Comparing the SMCS with the SES4 also demonstrates that a 1.7 logarithm level increase in removal rates may be achieved for AB. Significant improvements of 7 logarithm levels for ARB and 8 logarithm levels for ARG are still achievable, respectively.
Overall, it appears from the evaluation of Table 3 that the treatment components selected achieve significantly better removal rates for ARB and ARG. This can be attributed in part to thermal disinfection, which is not assumed to have any removal rate for AB. Likewise, filtration has no effect on the AB residues present in the wastewater. Obviously, to target all three components of AMR, a combination of multiple treatment methods is required. Combining the multiple treatment components, enabled by source separation of wastewater sub-streams, allows for significant removal rates improvements, even when compared to SES4.
4. Discussion
Up to now, CSs are the most prevalent sanitation systems. There are several challenges to be overcome when converting CSs into SMCSs:
• Young Key Technologies: Some of the key technologies that enable low-effort retrofits of existing buildings have not been available too long. Previously, separation or vacuum toilets required a change in user interface that is fraught with acceptance issues.
• Lock-in Costs: The predominant system is the CS. Most prevalent rehabilitation strategies are damage-driven. Conversion to SMCS requires systematic planning and change of the rehabilitation strategy for entire urban quarters. Furthermore, wastewater boards need to acquire both technical and organizational expertise.
• Uncertain Data: The simulation is based in part only on estimations for levels, loads and removal rates, because scientific data is lacking. For example, to our knowledge, there is little reliable data on the removal of AB by disinfection. By estimating the values conservatively, we are nevertheless confident that the simulation reflects a realistic status.
In summary, the current state-of-the-art-technology permits the transformation of the currently existing combined systems to source separation-modified combined systems, which are to be regarded to be one of the most—if not the most—effective measures to reduce AMR emission into the aquatic environment. With a transformation, in addition to resource savings and resource recovery, AMR emissions are significantly more reduced compared to conventional sanitation systems implementing “end-of-pipe” approaches that are currently predominantly under discussion. However, the transformation is not trivial and requires policy changes of the wastewater boards. Yet, there is a lack of incentives for implementing such sanitation systems, such as legal framework conditions or economic control mechanisms. However, the unique value proposition of source separation described here—given the urgency to reduce AMR—can be seen as a strong argument in favor of such sanitation systems, which provide not only short-term sanitation—as in the early days of sanitation systems—but also long-term sanitation by reducing AMR (28).
Data availability statement
The original contributions presented in the study are included in the article/Supplementary Material, further inquiries can be directed to the corresponding author.
Author contributions
Conceptualization: JL and MB. Methodology: JL and MB. Software: MB and HS. Validation: JL and MB. Formal analysis: JL. Investigation: JL and MB. Writing—original draft preparation: JL, HS and MB. Writing—review and editing: JL, HS and MB. Supervision: JL. Project administration: JL. Funding acquisition: JL. All authors contributed to the article and approved the submitted version.
Conflict of interest
The authors declare that the research was conducted in the absence of any commercial or financial relationships that could be construed as a potential conflict of interest.
Publisher's note
All claims expressed in this article are solely those of the authors and do not necessarily represent those of their affiliated organizations, or those of the publisher, the editors and the reviewers. Any product that may be evaluated in this article, or claim that may be made by its manufacturer, is not guaranteed or endorsed by the publisher.
Supplementary material
The Supplementary Material for this article can be found online at: https://www.frontiersin.org/articles/10.3389/fenvh.2023.1151898/full#supplementary-material.
References
1. World Health Organization. Antimicrobial resistance. World Health Organization (2020). Available at: https://www.who.int/news-room/fact-sheets/detail/antimicrobial-resistance (Accessed May 23, 2021).
2. Berendonk TU, Manaia CM, Merlin C, Fatta-Kassinos D, Cytryn E, Walsh F, et al. Tackling antibiotic resistance: the environmental framework. Nat Rev Microbiol. (2015) 13(5):310–7. doi: 10.1038/nrmicro3439
3. Taylor J, Hafner M, Yerushalmi E, Smith R, Bellasio J, Vardavas R, et al. Estimating the economic costs of antimicrobial resistance: model and results (2014).
4. Adler N, Balzer F, Blondzik K, Brauer F, Chorus I, Ebert I, et al. Antibiotics and antibiotic resistances in the environment: background, challenges and options for action. Umweltbundesamt (German Environment Agency/UBA) (2018).
5. Quach-Cu J, Herrera-Lynch B, Marciniak C, Adams S, Simmerman A, Reinke RA. The effect of primary, secondary, and tertiary wastewater treatment processes on antibiotic resistance gene (ARG) concentrations in solid and dissolved wastewater fractions. Water. (2018) 10(1):13–8. doi: 10.3390/w10010037
6. Jäger T, Hembach N, Elpers C, Wieland A, Alexander J, Hiller C, et al. Reduction of antibiotic resistant bacteria during conventional and advanced wastewater treatment, and the disseminated loads released to the environment. Front Microbiol. (2018) 9:1–16. doi: 10.3389/fmicb.2018.02599
7. Hiller CX, Hübner U, Fajnorova S, Schwartz T, Drewes JE. Antibiotic microbial resistance (AMR) removal efficiencies by conventional and advanced wastewater treatment processes: a review. Sci Total Environ. (2019) 685:596–608. doi: 10.1016/j.scitotenv.2019.05.315
8. Hembach N, Alexander J, Wieland A, Hiller CX, Schwartz T. Untersuchung der Eliminationsleistung verschiedener weiterführender Abwasserreinigungsverfahren zur Keimreduktion. Vom Wasser - Das J. (2018) 18(2):65–68.
9. Pallares-Vega R, Blaak H, van der Plaats R, de Roda Husman AM, Hernandez Leal L, van Loosdrecht MCM, et al. Determinants of presence and removal of antibiotic resistance genes during WWTP treatment: a cross-sectional study. Water Res. (2019) 161:319–28. doi: 10.1016/j.watres.2019.05.100
10. Quintela-Baluja M, Abouelnaga M, Romalde J, Su JQ, Yu Y, Gomez-Lopez M, et al. Spatial ecology of a wastewater network defines the antibiotic resistance genes in downstream receiving waters. Water Res. (2019) 162:347–57. doi: 10.1016/j.watres.2019.06.075
11. Larsen TA, Udert KM, Lienert J. Source separation and decentralization for wastewater management. In: Larsen TA, Udert KM, Lienert J, editors. Source separation and decentralization for wastewater management. London, United Kingdom: IWA Publishing (2013).
12. Gundupalli SP, Hait S, Thakur A. A review on automated sorting of source-separated municipal solid waste for recycling. Waste Manag. (2017) 60:56–74. doi: 10.1016/j.wasman.2016.09.015
13. Sukholthaman P, Sharp A. A system dynamics model to evaluate effects of source separation of municipal solid waste management: a case of Bangkok, Thailand. Waste Manag. (2016) 52:50–61. doi: 10.1016/j.wasman.2016.03.026
14. Weiterbildendes Studium Wasser und Umwelt. New alternative sanitation systems - NASS - terminology, material flows, treatment of partial flows, utilisation. 1st ed Weimar, Germany: VDG BAUHAUS-UNIVERSITÄTSVERLAG (2016).
15. Botturi A, Ozbayram EG, Tondera K, Gilbert NI, Rouault P, Caradot N, et al. Combined sewer overflows: a critical review on best practice and innovative solutions to mitigate impacts on environment and human health. Crit Rev Environ Sci Technol. (2021) 51(15):1585–618. doi: 10.1080/10643389.2020.1757957
16. Eramo A, Reyes HD, Fahrenfeld NL. Partitioning of antibiotic resistance genes and fecal indicators varies intra and inter-storm during combined sewer overflows. Front Microbiol. (2017) 8:1–13. doi: 10.3389/fmicb.2017.02024
17. Mutzner L, Bohren C, Mangold S, Bloem S, Ort C. Spatial differences among micropollutants in sewer overflows: a multisite analysis using passive samplers. Environ Sci Technol. (2020) 54(11):6584–93. doi: 10.1021/acs.est.9b05148
18. Munro K, Martins CPB, Loewenthal M, Comber S, Cowan DA, Pereira L, et al. Evaluation of combined sewer overflow impacts on short-term pharmaceutical and illicit drug occurrence in a heavily urbanised tidal river catchment (London, UK). Sci Total Environ. (2019) 657:1099–111. doi: 10.1016/j.scitotenv.2018.12.108
19. Petrie B. A review of combined sewer overflows as a source of wastewater-derived emerging contaminants in the environment and their management. Environ Sci Pollut Res. (2021) 28(25):32095–110. doi: 10.1007/s11356-021-14103-1
20. Veser S. Intelligent infrastructure systems for urban wastewater management. Research project EvaSENS. Bluefacts Int J Water-Manag. (2012) 2012:110–4.
21. Londong J, Hörnlein S. Development of a wastewater switch supplemented by separated wastewater collection as a preliminary stage to efficient water reuse and energy production (2021). Available at: https://www.uni-weimar.de/en/civil-engineering/chairs/chair-of-urban-water-management-and-sanitation/research/completed-projects/awas/ (Accessed Mar 15, 2022).
22. Schütze M, Wriege-Bechtold A, Söbke H, Wißmann I, Schulz M, Veser S, et al. Simulation and visualization of material flows in sanitation systems for streamlined sustainability assessment. Water Sci Technol. (2019) 79(10):1966–76. doi: 10.2166/wst.2019.199
23. DWA-Arbeitsgruppe ES-2.1, Systembezogene Anforderungen und Grundsätze. Arbeits– und Merkblattreihe DWA-A/M 102 (BWK-A/M 3) Zusatzdatei: Anwendungsbeispiele. Vol. 102. Hennef, Germany, Germany (2020).
24. Alexander J, Hembach N, Schwartz T. Evaluation of antibiotic resistance dissemination by wastewater treatment plant effluents with different catchment areas in Germany. Sci Rep. (2020) 10:8952. doi: 10.1038/s41598-020-65635-4
25. Londong J, Barth M, Söbke H. Modeling and simulation of source separation in sanitation systems for reducing emissions of antimicrobial resistances. Water. (2021) 13(23):3342. doi: 10.3390/w13233342
26. Wellbrock K, Knobloch JK-W, Heim M, Grottker M. Spurenstoffe und Multiresistente Bakterien in den Entwässerungssystemen Schleswig-Holsteins. Kiel/Lübeck/Hamburg (2019). Available at: https://www.th-luebeck.de/fileadmin/media/01_Hochschule/05_Fachbereiche/Bauwesen/Bilder/Projekte/PrioSH/PrioSH-Abschlussbericht_gesamt.pdf
27. Ferrer O, Casas S, Galvañ C, Lucena F, Bosch A, Galofré B, et al. Direct ultrafiltration performance and membrane integrity monitoring by microbiological analysis. Water Res. (2015) 83:121–31. doi: 10.1016/j.watres.2015.06.039
Keywords: microbial resistances, antimicrobial resistance genes, source separation, resource oriented sanitation, UASB, SAmpSONS
Citation: Londong J, Barth M and Söbke H (2023) Reducing antimicrobial resistances by source separation of domestic wastewater. Front. Environ. Health 2:1151898. doi: 10.3389/fenvh.2023.1151898
Received: 26 January 2023; Accepted: 22 February 2023;
Published: 21 March 2023.
Edited by:
Pam Factor-Litvak, Columbia University, United StatesReviewed by:
Jennifer Taylor, Tennessee Technological University, United States© 2023 Londong, Barth and Söbke. This is an open-access article distributed under the terms of the Creative Commons Attribution License (CC BY). The use, distribution or reproduction in other forums is permitted, provided the original author(s) and the copyright owner(s) are credited and that the original publication in this journal is cited, in accordance with accepted academic practice. No use, distribution or reproduction is permitted which does not comply with these terms.
*Correspondence: Jörg Londong am9lcmcubG9uZG9uZ0B1bmktd2VpbWFyLmRl Marcus Barth bWFyY3VzLmJhcnRoQHVuaS13ZWltYXIuZGU=
Specialty Section: This article was submitted to Housing Conditions and Public Health, a section of the journal Frontiers in Environmental Health