- 1Department of Geography and Geology, University of Turku, Turku, Finland
- 2Finnish Transport and Communications Agency (Traficom), Helsinki, Finland
- 3Finnish Environment Institute, Marine Research Centre, Helsinki, Finland
Shipping is the most pervasive source of anthropogenic underwater continuous noise and local intermittent noise. This study focused on the separation of anthropogenic intermittent noise from dynamic background noise in the Gulf of Finland using an adaptive threshold level (ATL) technique. The intermittent noise was validated with Automatic Identification System (AIS) data and the background noise with selected environmental factors. Separated components were characterized and compared with a sound exposure level (SEL) in three 1/3 octave bands. Intermittent noise can be separated with ATL in the Baltic Sea, and vessel traffic identified as the primary source. Background noise varies spatially and is partially explained by environmental factors. Intermittent noise has strong persisting influence on the acoustic environment near shipping lanes, elevating the SEL in each of the 1/3 octave bands: by 20–30 dB in the 63 Hz band, by 13–22 dB in the 125 Hz band and by 5–8 dB in the 2000 Hz band. We conclude that strong intermittent noise is characteristic to the underwater acoustic environment in the study area with heavy shipping traffic. By combining ATL with data from AIS, intermittent noise peaks in underwater hydrophone recordings can be associated with passages of individual vessels.
Introduction
The issue of anthropogenic underwater noise has received increased attention over the last few decades. The increased human activity in the world’s seas has elevated the underwater noise levels, changing the underwater acoustic environment. This noise has been formally recognized as a pollutant to the marine environment (IISD, 2018).
The underwater acoustic environment is comprised of biotic, abiotic and anthropogenic sound sources (Pijanowski et al., 2011). Natural sounds of biotic or abiotic origin are generated by, for example, vocalizations and foraging activities of animals or surface agitation from breaking waves and rain showers, respectively (Hildebrand, 2009). These sound sources have been a part of the underwater acoustic environment throughout the natural history of earth. The anthropogenic sounds generated by, for example, shipping, underwater construction and seabed exploration, have been introduced after the industrial revolution and have been increasing ever since, making the seas noisier than ever before (Andrew et al., 2002; McDonald et al., 2006; Hildebrand, 2009). From these sound sources, shipping is the most pervasive source of anthropogenic noise.
Generally anthropogenic noise can be divided into two categories: impulsive noise originating from, for example constructions and explosions, and continuous noise originating primarily from shipping. Distant vessel traffic generates continuous low-frequency noise which is a ubiquitous component of the background noise in most of the world’s seas, locally shipping also generates intermittent noise (Van der Graaf et al., 2012; Dekeling et al., 2014). Intermittent noise is defined by the local, relatively rapid, increase and decrease in sound pressure levels (SPL) following the bypass of a single or multiple vessel(s).
Anthropogenic noise is known to have multiple potential impacts on the marine biota, for example on mammals (Richardson et al., 1995; National Research and Council, 2003), fishes (Slabbekoorn et al., 2010; Cox et al., 2018; Di Franco et al., 2020), and invertebrates (Di Franco et al., 2020). Underwater noise can cause distractions and induce masking, where the auditory frequencies of an animal are saturated with noise and its ability to detect important acoustic cues in its environment, such as communication calls of other individuals or the whereabouts of predators and prey is lowered (Erbe et al., 2016). Additionally, noise can cause physiological stress (Rolland et al., 2012; Nichols et al., 2015) and in extreme cases induce direct or indirect physical injuries that can lead to hearing loss, injury or even the death of an animal.
The effective assessment of the potential impacts of underwater noise requires baseline knowledge about what kinds of sounds are naturally present and about the contribution of anthropogenic sounds. The separation of these two components in the acoustic environment can be complicated due to a multitude of overlapping sound sources that may vary over time. The use of fixed thresholds (e.g., percentile-based exceedance levels) might lead to erroneous results by over-emphasizing the extremities of SPL distribution; this may lead to a situation where, for example, background noise would be represented by the SPLs during calm weather, although rough seas, rain showers, deformation of ice cover and other naturally generated loud sounds are very much a part of the natural underwater acoustic environment. To overcome this challenge, Merchant et al. (2012a) introduced the adaptive threshold level (ATL) methodology, which adapts to gradual changes in SPLs and is more able to separate anthropogenic sound sources.
Enhanced methods are needed since international regulations are being established to mitigate the negative impacts of underwater noise (Dolman and Jasny, 2015). In 2008, the European Union adopted the Marine Strategy Framework Directive (MSFD 2008/56/EC), which was revised in the Commission Directive 2017/845/EU, 2017 with underwater noise as one of the recognized pressures of the marine environment. However, the technical subgroup (TSG Noise) working on MSFD has concluded that not much is known about the underwater acoustic environment in the European seas (Van der Graaf et al., 2012). To help close this knowledge gap in the Baltic Sea, EU LIFE + funded the BIAS project (Baltic Sea Information of the Acoustic Soundscape) was established (Sigray et al., 2016). One of the objectives of the project was to provide the first insight into the underwater acoustic environment of the Baltic Sea, emphasizing the continuous low-frequency shipping noise as defined by the Commission Decision 2010/477/EU, 2010 descriptor 11.2 (Betke et al., 2015; Nikolopoulos et al., 2016).
In this study, the ATL methodology is applied for the first time in the Baltic Sea for the data from four hydrophone loggers of the BIAS setting. The shallow and stratified waters of this coastal sea area combined with its strong seasonality and heavy shipping activity create a unique acoustic environment that offers a perfect case for this study. The separation of the natural and anthropogenic components of the underwater acoustic environment in this area would help to define criteria for its Good Environmental Status (GES), thereby contributing to the goals of the MSFD. We base our study on three working hypotheses: (1) anthropogenic intermittent noise can be separated from the underwater hydrophone recordings, (2) Automatic Identification System (AIS) data can be used to identify the sources of intermittent noise, and (3) variations in the background noise level can be explained with environmental factors.
Materials and Methods
Study Area and Data Sources
The Baltic Sea is a shallow (average depth 55 m), semi-enclosed sea basin in Northern Europe (Figure 1). It is one of the largest brackish water bodies in the world, with the mean surface-water salinity varying between 1.8 and 11.3‰, the lowest values occurring in the Gulf of Bothnia and the Gulf of Finland (Leppäranta and Myrberg, 2009, p. 59; Snoeijs-Leijonmalm and Andrén, 2017, p. 30). There is also a strong vertical salinity gradient in this sea area as the saltiest water settles in the deep-water layers. The climate in the study area is strongly seasonal as, in most winters, at least parts of the study area get ice cover. The sea depth varies mainly between ca. 30 and 70 m, and the seabed comprises mud in the open-sea areas, and clay, sand and rocks closer to the seacoast. For the heavy shipping activity in this area, the Gulf of Finland is divided by a shipping lane system that continues until the Russian ports but is also diverted to other ports such as Helsinki, Hamina-Kotka, Tallinn, and Paldiski.
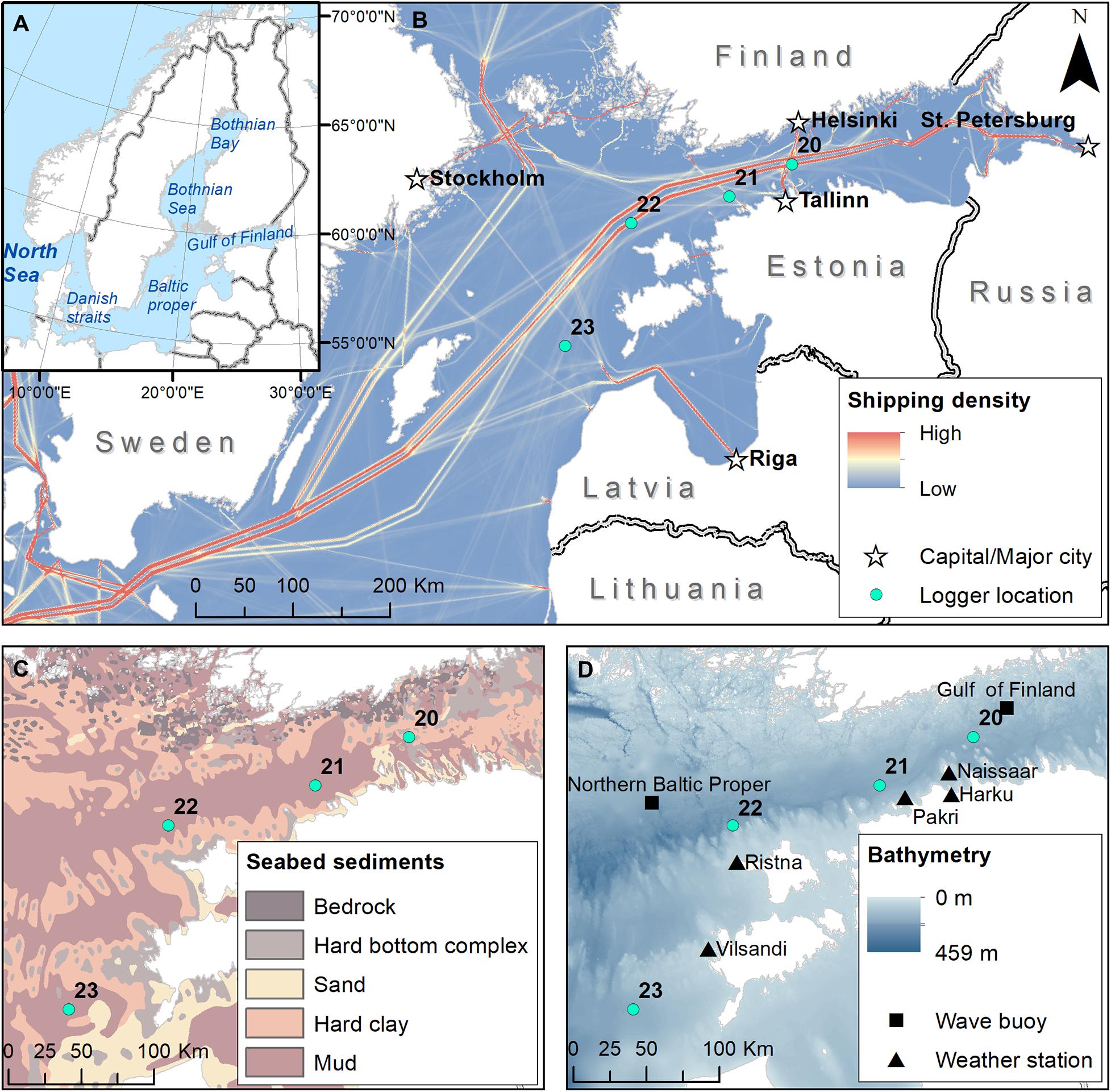
Figure 1. Geographical characteristics of the study area. (A) Location in the Baltic Sea region. (B) Major shipping routes based on AIS data. (C) Seabed substrate. (D) Sea depth and locations of the acoustic data loggers. Individual logger depths are 60, 90, 80, and 80 m for loggers 20, 21, 22, and 23, respectively. In (B–D), cyan dots with numbers from 20 to 23 show the locations of the hydrophone loggers used in this study. Data sources: HELCOM AIS database (2017); BALANCE project, EU BSR Interreg III B (2008); Baltic Sea Bathymetry Database, BSBD (2013); OpenStreetMap. Downloaded from HELCOM map and data service: http://maps.helcom.fi.
The acoustic measurements used in this study were the processed recordings of the four BIAS hydrophone loggers with ID numbers 20, 21, 22, and 23 recorded by Tallinn University of Technology (Table 1 and Figure 1). The hydrophone loggers were stand-alone SM2M-loggers produced by Wildlife Acoustics, that were calibrated by Swedish Defence Research Agency FOI before the deployment. The loggers were anchored to the bottom with a rig design consisting of a concrete block, acoustic releaser, extra float and the logger itself secured by polypropylene rope. The rig design above the anchor was positively buoyant, which ensured that the loggers were suspended 3 m above the bottom throughout the deployment (For more information, see Verfuß et al., 2015). The recordings covered the frequency range of 10–10,000 Hz (Nikolopoulos et al., 2016). The data from each logger were in separate tables depicting the 20-s logarithmic means of the SPL in the three 1/3 octave frequency bands of 63, 125, and 2000 Hz, and the broadband recording of 10–10,000 Hz (see Betke et al., 2015). Of these, the 63 and 125 Hz bands were defined in the Commission Decision 2010/477/EU, 2010 descriptor 11.2 as proxies for low-frequency shipping noise. In addition, the BIAS project included a 2000 Hz band that can be related to shipping activity through sound peaks in higher frequencies (Nikolopoulos et al., 2016).
Our data come from four Estonian hydrophone loggers near the junction of the Baltic Sea Proper and the Gulf of Finland, with their maximum distance of ca. 100 km in between (for locations, see Figure 1). Two loggers (ID numbers 20 and 21) were located in the Gulf of Finland, and two others (ID numbers 22 and 23) were in the NW margin of the Baltic Proper. During the wintertime, the water mass should be as homogenous as possible. During January 2014, the vertical water temperature gradient was minimal (Figure 2), and no sea ice had yet been formed in the open sea (FMI, 2014a,b,c). The selected week (from noon on January 16th until noon on January 23th) had relatively calm wind speeds in the nearby weather stations with mean wind speed of 4.5, 3.2, 1.7, and 3.4 m per second with standard deviation of 2.4, 1.5, 0.7, and 0.9 near the loggers 20, 21, 22, and 23, respectively. The significant wave height mean was 0.7 and 1.3 m with standard deviation of 0.3 and 0.4 in the wave buoys of Gulf of Finland and Baltic proper, respectively. During this week, leisure boating hardly contributed to the underwater acoustics at all.
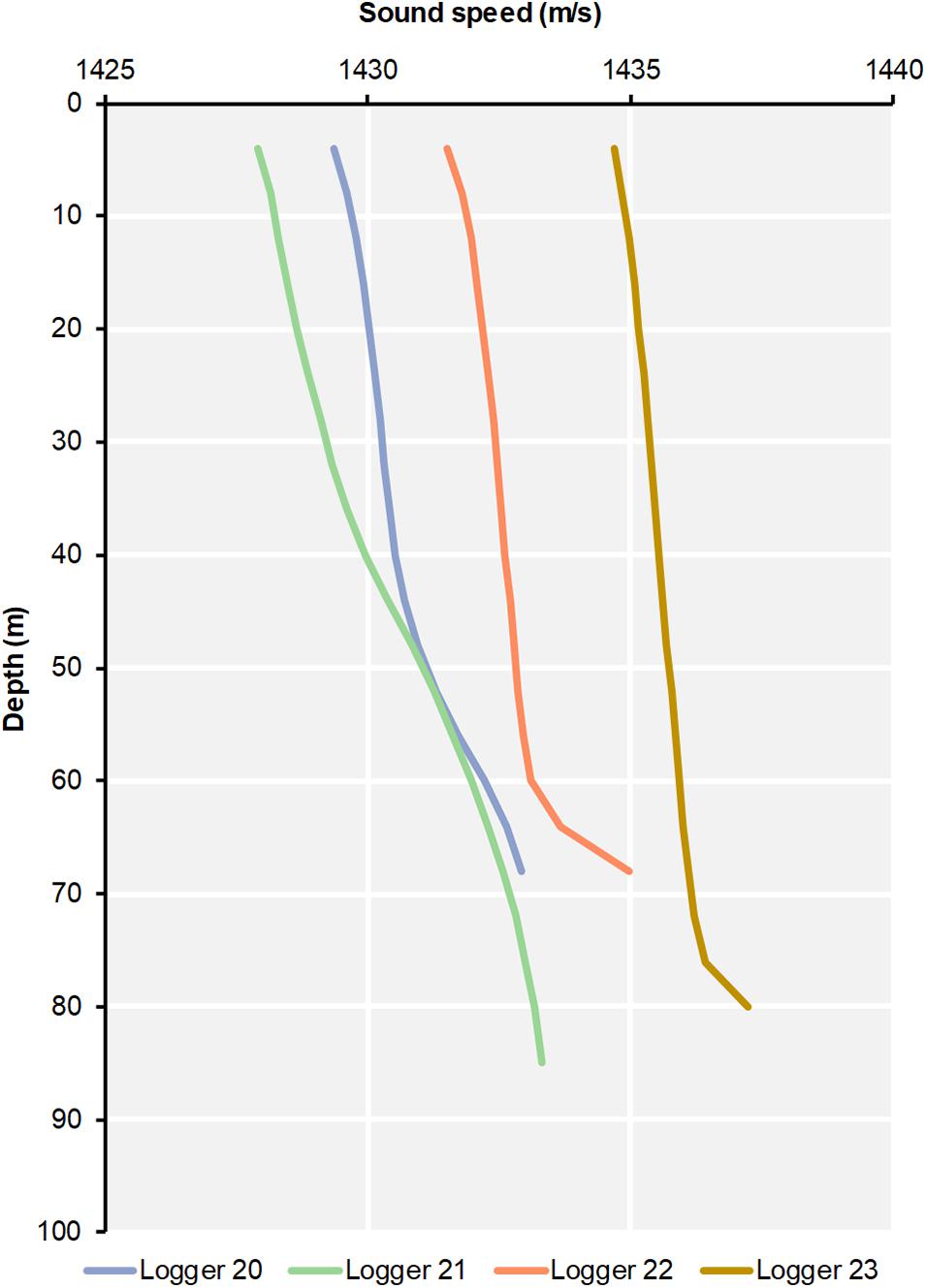
Figure 2. Vertical sound speed profiles recorded in the logger deployment locations in 15.01.2014. Data source: SMHI HIROMB BS01 forecast model, provided by the BIAS-project.
To identify sound sources, data were applied from both the Vessel AIS and the selected environmental parameters (Table 2). AIS data were extracted according to vessel location transmissions within a buffer zone radius of 20 km around each hydrophone logger. Each time, the data record included the time of transmission, the location of the vessel, the identity of the vessel as an MMSI number (Maritime Mobile Service Identity), the type of vessel, the speed of the vessel in knots and information about the size of the vessel. The time interval between two consecutive AIS records of a vessel can vary from under a minute to ten minutes. Meteorological data include hourly precipitation levels and average wind speed records in the Estonian weather stations Ristna, Pakri, and Harku. Only wind speed data were available in Naissaar and Vilsandi. Data on the significant wave height came from two wave buoys from the Finnish Meteorological Institute (FMI), one located in the Gulf of Finland and the other in the northern Baltic Proper.
Acoustic Data Processing
We aggregated the acoustic data into one-minute average values to decrease the sizes of the datasets and to exclude brief impulsive sounds that cannot be related to the gradual increase of SPLs from transient shipping. The averaging of the data was made using the logarithmic mean instead of the arithmetic mean as the SPL data is in decibel scale. According to Merchant et al. (2012b), this is the most relevant averaging method to further assess the cumulative sound exposure level. Data operations were carried out using the open-source software environment R1 with the package Seewave (Sueur et al., 2008).
Intermittent noise was separated from background noise using the ATL method with running minimum values (Merchant et al., 2012a):
where ATL is the adaptive threshold level at the time t, which is the number of decibels C above the running minimum determined by a minimum SPL within a time window W.
The running minimum is interpreted to be representative of the background noise level. The moving time window adapts to changes in the underwater acoustic environment. If SPL(t) is greater than ATL(t), the record is classified as intermittent, and if SPL(t) is smaller than ATL(t), it is classified as background. These computations were made in R-studio using the Tidyverse packages (Wickham, 2017) with the Lubridate and CaTools packages (Wickham and Grolemund, 2011; Tuszynski, 2014). This function was applied only to the 10–10 000 Hz broadband data, which may include both anthropogenic and natural sound sources. The ATL function parameters were determined after Merchant et al. (2012a) with a time window W of 3 h and threshold ceiling C of 6 dB. The time window of 3 h is long enough to not be affected by transient shipping noise but short enough to adapt to changes in natural sound sources. The threshold ceiling was set to 6 dB, which corresponds to the doubling of the acoustic pressure.
Consecutive intermittent records were classified as individual sound events with unique ID numbers, and they were used to compose a table of all observed intermittent sound events. Intermittent sound events with durations under 5 min were removed from the data as they are most likely caused by impulsive sounds from an unidentified source that does not represent transient shipping noise.
Sources of Intermittent and Background Sounds
We processed the AIS data into segments depicting the route of an individual vessel based on the MMSI number. The segments were further separated by identifying all bypasses of an individual vessel regardless of whether the same vessel had also been recorded earlier. If there was a gap of over 30 min between two consecutive AIS records, they were handled as different visits by the same vessel (e.g., return passage after visiting a port). The routes of individual vessels were processed from the AIS data, assuming that the actual route between two consecutive locations is linear.
As underwater sound attenuates with distance: the highest received SPLs caused by a vessel should be recorded when the distance between the vessel and the hydrophone is shortest; this location is known as the closest point of approach (CPA). The closest AIS point is not necessarily the same as the CPA because the CPA is usually located along the trajectory between two of the closest AIS points (Figure 3). To calculate the correct location from the AIS records, we projected the segment between the two closest AIS points and computed the location of the CPA along the line to the location of the logger. The time a vessel was at the CPA was calculated based on the speed of the vessel at the previous AIS point and the distance between the AIS point and the CPA. This estimation relied on the assumption that vessels maintain the same speed and course between two AIS points. The calculations were made with custom functions in R using the rgeos package (Bivand and Rundel, 2017).
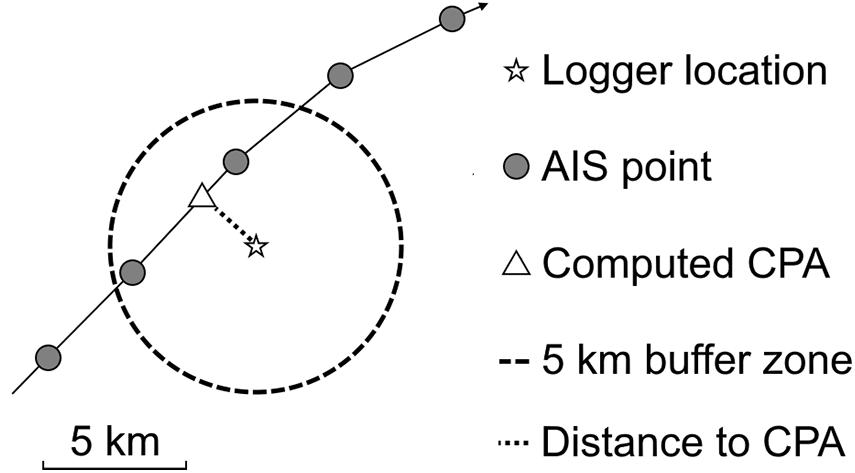
Figure 3. Illustration of determining the location of CPA along the trajectory between two AIS points closest to the hydrophone logger.
Intermittent sound events were compared to the proximities of individual CPAs. As individual ships can be generally recognized within 5 km of the hydrophone in the Gulf of Finland (Sairanen, 2014, unpublished), we applied a distance criterion of 5 km as a buffer from the hydrophone. Additionally, after Merchant et al. (2012a), we applied a time criterion of ±7.5 min from the peak SPL value of the intermittent sound event to identify a given vessel as the source of the intermittent sound. If there was only one CPA that met both criteria, the intermittent sound event was classified to be caused by a single vessel. If there were more than one CPA, the intermittent sound event was classified to be from multiple possible sources. When no CPAs within the spatial and temporal zone were found, the sound source was classified as unknown (false positive), which could originate, for example, from a vessel without an onboard AIS.
The background component of the acoustic environment represents the noise levels without the transient sound sources. The background noise is generated by the noise of distant shipping and biotica and abiotic sources. All the loggers are located relatively close to a shipping lane with high traffic distributed evenly throughout the entire study period. Thus, the distant shipping noise can be assumed to be ubiquitous component of the background noise that cannot be distinguished as a temporally independent variable.
Abiotic sources such as wind, rain and wave height are spatially and temporally varying sound sources that can be distinguished from the 10–10,000 Hz broadband SPL data. In order to examine the contribution of abiotic sound sources to the background component, a multiple regression analysis was applied. We used the background SPL as a dependent variable and average wind speed, precipitation and significant wave height as independent variables. The data about precipitation at logger 23 was missing and was removed from the analysis.
The adjusted R-squared was interpreted as the coefficient of determination, which describes the percentage of how much of the variation in the dependent variable can be explained by independent variables. The statistical significance of the model and individual variables is determined with a p-value below 0.05.
Characterization of Local Acoustic Environments
Three 1/3 octave bands with center frequencies of 63, 125, and 2000 Hz were used as proxies to examine the spectral characteristics of the acoustic environment around each hydrophone. We first separated the intermittent and background SPL values into different subset tables so that there were three for each logger: complete dataset, intermittent component and background component. Following Merchant et al. (2012a), the data were then averaged with the 24-h sound exposure level (SEL). The SEL is used to average and compare sounds with different durations as a time-integrated value (Robinson et al., 2014). The underwater acoustic environment comprises, for most of the time, of generally stable background noise with intermittent SPL peaks of shorter duration. The use of time-integrated SEL enables the comparison of these components that vary in duration. According to Verfuß et al. (2015), the SEL can be expressed as follows:
where reference time T0 is a reference time of 1 s and T is the time period of interest in seconds. We programmed the SEL calculation in R and executed it on all the datasets. SEL is first calculated in one-minute time periods, which is the integration time of all the datasets. The resulting SEL values are then averaged to the 24-h logarithmic mean of 1-min SEL values.
The 24-h SEL values of the intermittent and background components of the acoustic environment can be compared to one another and to the total 24-h SEL of the 1/3 octave bands. The comparison allows the assessment of the contribution of ship-generated intermittent noise to the total acoustic environment related to the background noise (Merchant et al., 2012a).
Results
Distributions of the one-minute average 10–10,000 Hz broadband SPLs from the entire study period show considerable differences between the four loggers (Figure 4). The SPL values were relatively low in the loggers located in the Gulf of Finland (20 and 21) when compared to those located in the northern Baltic Proper (22 and 23). On the other hand, in the loggers located close to the major shipping lanes (20 and 22 but also 21), histograms were skewed toward high SPL values resulting from vessels passing near them. The SPL histogram of logger 23 showed a nearly normal distribution. The adaptive threshold method effectively separated the intermittent and background components of the SPL data, and these showed good correspondence with the closest vessel vicinities in AIS (Figure 5 and Table 3). Accordingly, near the main shipping lines (loggers 20 and 22), high numbers of intermittent events were recorded, accounting for about one-third of the total monitoring time. In contrast, in more distant areas from the main shipping routes (loggers 22 and 23), intermittent sound events accounted for less than 10% of the total monitoring time. AIS data also showed a few cases where adjacent vessel locations, possibly small craft, did not yield a notable intermittent sound peak in the SPL data (Figure 5). Between 78 and 94% of the intermittent sound events could be associated with one or more identified vessel passes in the AIS data (Figure 6). Although multisource intermittent sound events were common in the loggers situated near the main shipping lanes, the data from these loggers also resulted in the best identification results. Logger 20 showed a particularly high number of intermittent sound events with both single- and multi-source vessel passes. The proportions of the types of large peak-generating vessels (cargo, tanker, and passenger) were generally consistent with the total vessel traffic within the 5-km zone from the loggers (Table 3). However, there was a substantial increase in the proportion of peak-generating passenger vessels at logger 21. Fishing vessels and other vessel types rarely generated intermittent noise peaks in all the loggers.
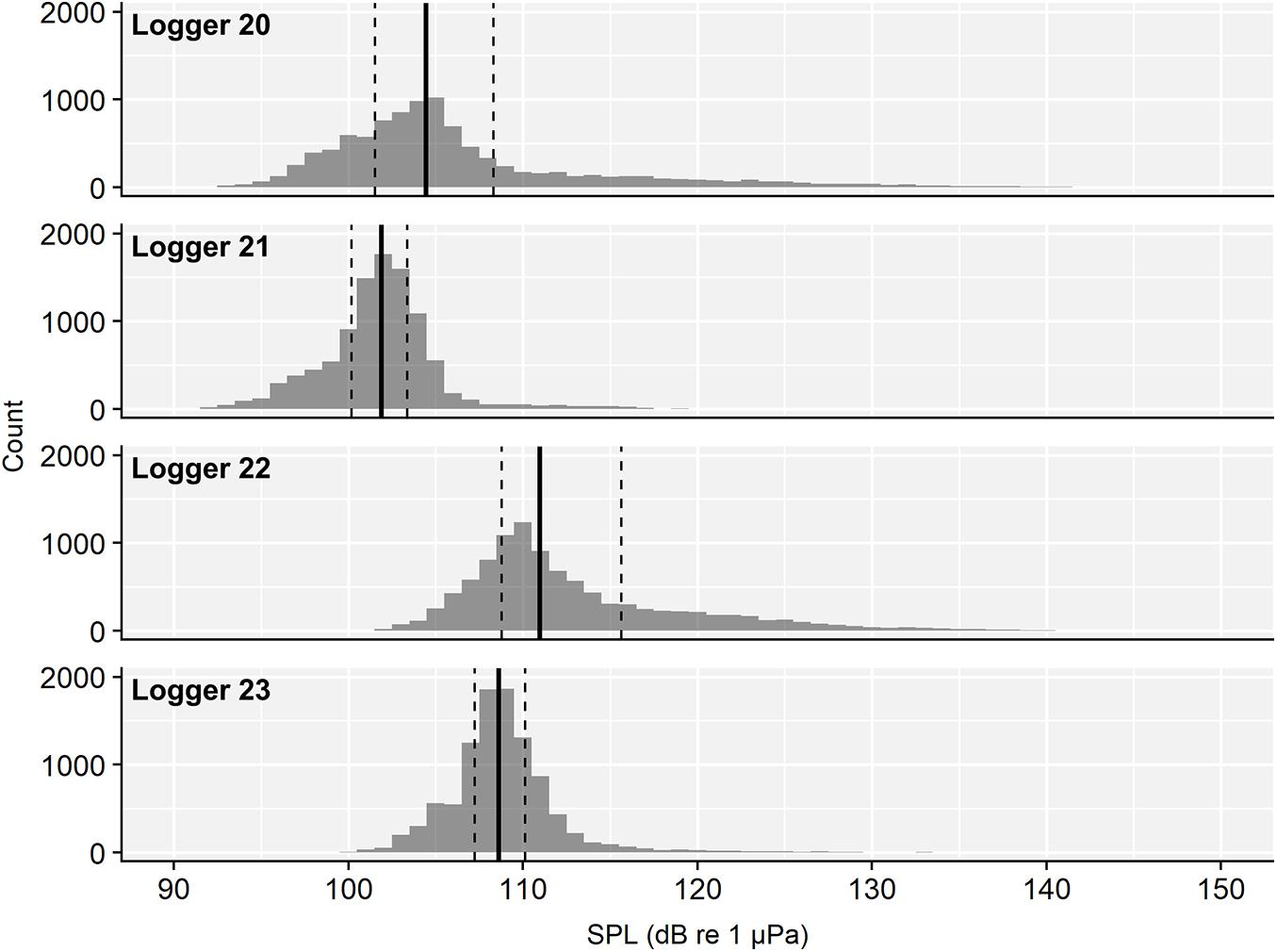
Figure 4. One-minute average 10–10,000 Hz broadband SPL histograms of all four loggers from the entire study period. The solid black line represents the median and the dashed black lines the first and third quartiles.
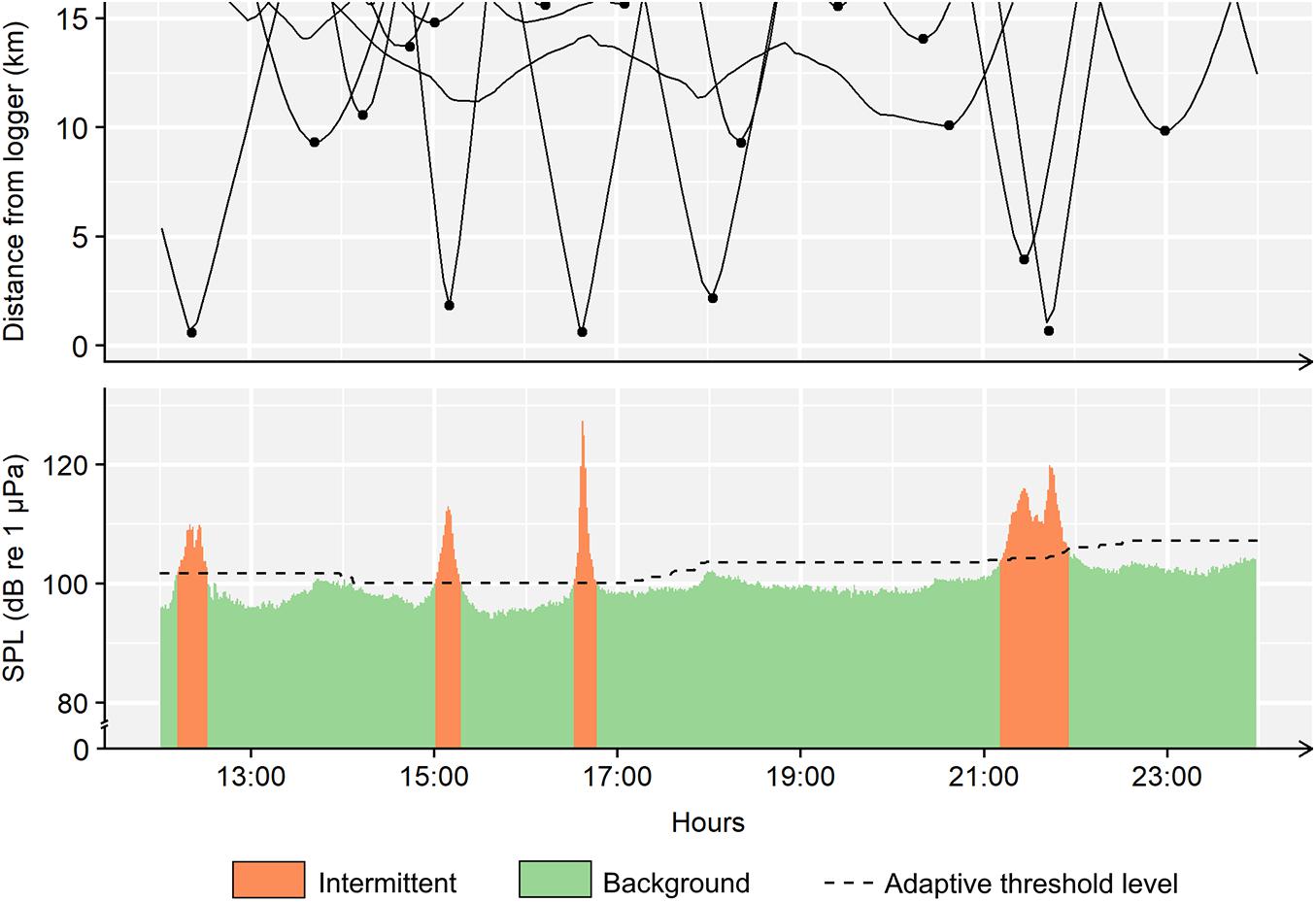
Figure 5. An example of comparing data AIS and 10–10,000 Hz broadband SPL data (hydrophone 21 between 12:00 and 23:59 on January 17th, 2014). The lower diagram shows the identification of the intermittent and background components of the acoustic environment. The adaptive threshold level is illustrated as a dashed black line. The upper diagram shows the proximities of moving vessels with the computed CPA as black dots.
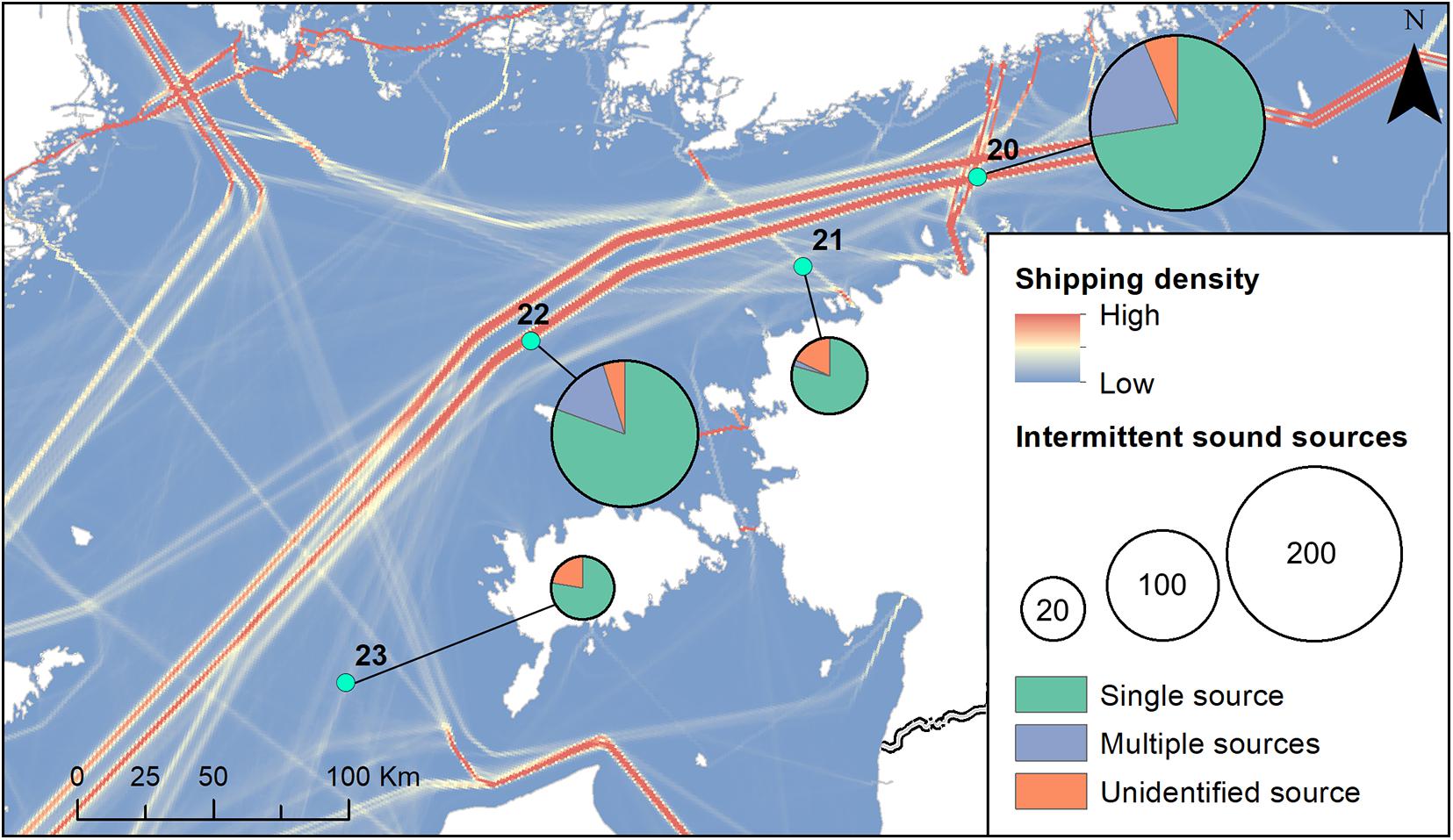
Figure 6. Number of intermittent events and the proportions of their identified origins within the 5-km buffer zone from the hydrophone loggers. Background data sources: HELCOM AIS database (2017); OpenStreetMap. Downloaded from HELCOM map and data service: http://maps.helcom.fi.
The background acoustic component was contributed by the environmental variables of significant wave height (p < 0.001) and wind speed (p < 0.001 in two of the loggers, which have this information), while precipitation did not show statistically significant results (Table 4). In logger 21, a positive statistical significance (p < 0.001) was found only with the wind speed. The collinearity between significant wave height and average wind speed was significant in logger 22, and thus the wind speed was ruled out as an independent variable at logger 22. The residuals of the regression analyses were distributed normally in all the loggers.
The coefficient of determination showed a large variation between the loggers (Table 4). The highest value was found in the logger with the most frequent vessel traffic (logger 20). The next highest coefficient of determination was found in the sea area with the least vessel traffic (logger 23), which otherwise had the longest distance to the nearest weather observation station and wave buoy.
The 24-h SEL of the three measured frequency bands varied considerably between the intermittent and background components (Figure 7). The intermittent component showed a similarity in all the loggers except in logger 21. The background component, on the other hand, showed a similarity in the loggers located in the same sub-basin: loggers 20 and 21 in the Gulf of Finland and loggers 22 and 23 in the northern Baltic Proper. Loggers 20 and 22, which had the heaviest vessel traffic of our logger sites, also showed a particularly strong disparity in the low-frequency SEL (63 Hz band) between the intermittent and background components. Contrarily, the 24-h SEL of the 2000 Hz band showed rather similar values in all our recordings. During the intermittent components of the 24-h SEL recordings in all our loggers, values in the 2000 Hz band were lower than those of the other frequencies. During the background components, loggers 20 and 21 showed the opposite behavior.
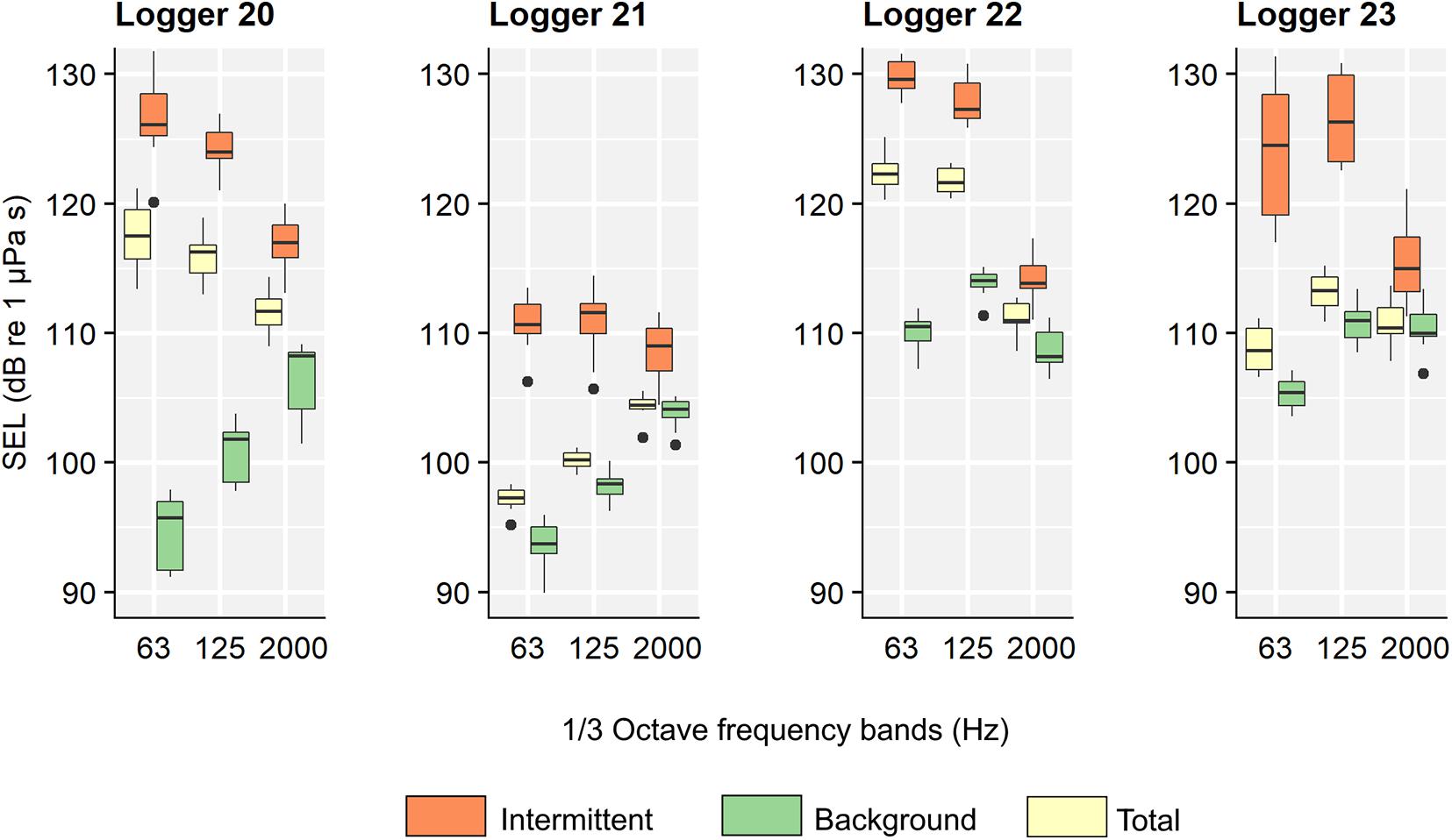
Figure 7. Distributions of the 24-h sound exposure level (SEL) in three 1/3 octave bands with center frequencies of 63, 125, and 2000 Hz. Loggers 20, 21, 22, and 23 are described in the total (yellow), intermittent (orange), and background (green) 24-h SEL distributions. The solid black line represents a median and the colored box a middle 50% of the values. Whiskers represent the range of all the data and black dots the outliers.
Discussion
Anthropogenic noise has strong persisting influence on the wintertime underwater acoustic environment of the northern Baltic Sea near shipping lanes. SPLs in all the loggers studied were above 100 dB re 1 μPa most of the time, but a number of intermittent events with SPL values up to more than 140 dB re 1 μPa were also recorded. Using the ATL methodology as a basis (Merchant et al., 2012a), over a third of the monitored time fell into the intermittent category in the hydrophone loggers that were located close the main shipping lanes. Their sound sources were also unmistakably from vessel traffic. The AIS data based vessel recognition rates of our data ranged from 78 to 95%, mainly consisting of cargo ships.
According to HELCOM (2010), approximately 2,000 individual vessels navigate the Baltic Sea at any given time. According to our results, such heavy vessel traffic may locally dominate the underwater acoustic environment in terms of both SPLs and the proportion of time with intermittent sound events. As the number of ships in the Baltic Sea is expected to double between 2010 and 2030 (WWF, 2010)—largely due to the expansion of oil terminals and subsequently increasing oil transportation (HELCOM, 2018, p. 184)—the underwater noise will likely increase in the future. An increase has also been reported by Andrew et al. (2002) and McDonald et al. (2006) along the California coast, suggesting a 10–12 dB rise between 1960 and 2010. Due to such heavy changes, permanent monitoring of the underwater acoustic environment could be considered.
In Falmouth Bay at the western entrance to the English Channel, 63% of intermittent events were caused by a single vessel or multiple vessels, and the rest remained unidentified (Merchant et al., 2012a). Similar vessel identification rates have been reported in the inner Moray Firth of Scotland (Merchant et al., 2014). Our study showed clearly better vessel recognition rates, which may be attributed to vessel activity, bandwidth, AIS data and the time of year. Merchant et al. (2012a) reported multiple vessels mooring, bunkering or maneuvering, which decreased the source detection rate, but in this study such activity was absent. The broader bandwidth of our data (10 – 10,000 Hz versus 5 – 8000 Hz) may also be more capable of capturing the higher-frequency noise caused by propeller cavitation (Ross, 1976; Arveson and Vendittis, 2000). Although high sound frequencies effectively attenuate over large distances due to dissipative properties of the seawater, in ranges below 3 km from the source, they can still be at high levels (Veirs et al., 2016). Frequencies above 1000 Hz generate a larger contrast between the presence and absence of a vessel, thus enhancing the recognition rate. Another possible factor contributing to vessel recognition is the proportion of vessels without an onboard AIS device. Our data are from the winter, but for example, Merchant et al. (2012a, 2014) studied the summertime acoustics. At that time, smaller vessels and boats without AIS can generate noise peaks that are deemed to remain unidentified.
The background noise in our data could largely be explained by a few abiotic parameters, particularly wind speed and significant wave height, which reasonably behave like surrogates of the sea-surface agitation, which is considered the primary sound source at frequencies above 500 Hz (Hildebrand, 2009). However, we found a lot of variation between the different loggers in our data (Table 4). This suggests that not all possible sound sources were included in the multiple regression model. Similar to Merchant et al. (2012a), it is important to note that the background component of our analysis does not represent the background noise in the absence of human activity. Distant shipping noise contributes especially in lower frequencies and cannot be distinguished from the natural sound sources in the same bands.
Also, the 24-h sound exposure levels (SEL) of the intermittent and background sound components confirmed the importance of transient shipping in the local background noise levels. The median 24-h SEL of transient shipping elevates the SEL to 20–30 dB above the background noise levels in the 63 Hz 1/3 octave band and to 13–22 dB in the 125 Hz band. The SEL rise was lowest in the highest frequency band (2000 Hz), where it varied from 5 dB to 8 dB. The increase of 30 dB denotes the increase of sound pressure by a factor of 31.6. Such vast disparity between the presence and absence of shipping activity can have multiple detrimental effects on biota. Wysocki et al. (2006) conducted an experiment where three freshwater fish species, from which the European perch (Perca fluviatilis) is also common in the Baltic Sea, were exposed to ship noise with average SPL of 153 dB with instantaneous levels ranging 32 dB. The results indicated increased stress hormone (cortisol) secretion in all three fish species studied. Similar reactions of fish to intermittent noise was also reported by Nichols et al. (2015). Based on these results, the intermittent shipping noise described in this study could potentially influence on the Baltic Sea fish species. This, however, requires further research on fish physiology and behavior in the region.
A comparison of the intermittent and background components to the total 1/3 octave 24-h SEL yields a general overview of the contribution of anthropogenic noise to the local acoustic environment. The loggers next to a major shipping lane (20 and 22) have significantly more bypassing traffic than the more distant loggers (21 and 23) The contribution of traffic can be assessed by comparing the distribution of total SEL to the intermittent and background components. The total SEL clearly resembles the intermittent SEL in loggers with high shipping activity (Figure 7). In loggers with sparse bypassing vessel traffic, the total SEL resembles the background component. Similar results were obtained by Merchant et al. (2012a) as intermittent component had clear contribution to the total 24-h SEL. These resemblances enable the conclusion that the acoustic environment in loggers 20 and 22 is controlled by intermittent shipping noise, and in loggers 21 and 23, the acoustic environment is controlled by background noise. This is relatively sharp distinction, given that all the loggers are relatively close to a major international shipping route (Figure 1A). One way to mitigate the detrimental impacts of underwater noise would to establish spatial and/or temporal rerouting of shipping lanes in the vicinity of ecologically sensitive areas such as fish spawning grounds. Noise mapping and management are essential to ecosystem-based marine spatial planning (Merchant et al., 2017; Farcas et al., 2020).
The background component exhibits similarities with the loggers located in the same sub-basin regardless of the shipping intensity. The 24-h SEL is distributed similarly in the Gulf of Finland (20 and 21) and in the northern Baltic Proper (22 and 23). The difference is clear, especially at the low-frequency bands of 63 and 125 Hz, with higher values in the northern Baltic Proper. As these lower frequencies are dominated by distant shipping activity (Hildebrand, 2009), the background sound levels can be elevated by a larger number of vessels navigating through the same sub-basin. The Baltic Proper forms the largest sub-basin of the Baltic Sea, where shipping traffic is routed not only to the Gulf of Finland but also to the Bothnian Sea and major ports in Latvia, Lithuania, Russian Kaliningrad and Poland. There are arguably more vessels in the Baltic Proper at any given time compared to the Gulf of Finland, and thus, the distant shipping noise is possible cause for the elevated SEL in lower frequencies. These regional differences are also in line with the annual estimated probability density functions of the SPL calculated from the same BIAS-data by Mustonen et al. (2019).
The European Union’s Commission Decision 2010/477/EU, 2010 established the monitoring of the continuous underwater noise in its criterion 2. Underwater noise is monitored in the 63 Hz and 125 Hz 1/3 octave bands. Based on the results of this study, these bands are indeed significantly elevated due to nearby vessel traffic. The same sound frequencies are also known to be audible to local marine fauna, such as seals and fish (Enger, 1967; Chapman and Hawkins, 1973; Offutt, 1974; Hawkins and Johnstone, 1978; Sills et al., 2015). For example, the gray seal (Halichoerus grypus) vocalizes in low frequencies, which could indicate that auditory masking by vessel traffic could occur (Asselin et al., 1993). In order to account for the ecological impacts of underwater noise, Commission Decision 2017/848/EU, 2017 requested threshold values that allow the assessment of GES for the marine waters in the EU. The method described in this study could be used in determining the threshold regarding shipping noise by comparing the ratio of the background and intermittent components. The areas with intense shipping traffic could be identified and mitigation considered in order to reach GES. Practical measures could be operational, such as rerouting or speed limits, or they could be technical, like enhanced propeller design or resilient mounting of the machinery. They would nevertheless need improved knowledge about the ecological impacts that frequent vessel traffic can cause in the Baltic Sea environment. The source levels were not assessed in this study, and thus the effect of individual characteristics of a vessel, such as vessel type, speed or size, cannot be assessed from the received noise levels.
Our study demonstrated the efficiency of the combined use of ATL with data from AIS to distinguish intermittent underwater noise and to discriminate passages of individual vessels. By incorporating longer study periods this methodology could illuminate new details in the underwater acoustic realm and finally, be developed toward its automatized real-time monitoring. Such development would, however, require open access to comprehensive data sets with good spectral and temporal coverage. This dilemma could be solved by agreeing about a standard form of continuous hydrophone data, which would be generalized enough to safeguard the national safety interests but detailed enough to be useful in the study of underwater noise. Such integration could, for example, be SPLs of 20 – 60 s intervals covering the 1/3 octave bands from 10 Hz to 10 000 Hz.
Conclusion
Using ATL methodology, intermittent noise in the Baltic Sea can be separated, and with the use of AIS data, vessel traffic can be identified as their primary source. Background noise generated by distant shipping and natural sound sources (wind, sea-state and rain) varies in space and time. Intermittent noise by bypassing vessel traffic elevates the median sound exposure level (SEL) to 20–30 dB above the background noise levels in the 63 Hz 1/3 octave band, to 13–22 dB in the 125 Hz band and to 5–8 dB in the 2000 Hz band. Intermittent noise has strong persisting influence on the total acoustic environment in areas close to intensive shipping routes.
Data Availability Statement
The one-third octave band SPL values and AIS-data analyzed during the current study are available from Finnish Environment Institute’s Marine Research Center on reasonable request.
Author Contributions
All the authors contributed to research planning and methodological design and provided edits on the manuscript. JS was the main actor in all of these work phases, and he performed the entire data analysis. JP stood for the quality assurance of the BIAS-data.
Funding
This study was supported by a small grant provided by Merenkulun säätiö/Sjöfartsstiftelsen (Grant number: 201700060).
Conflict of Interest
The authors declare that the research was conducted in the absence of any commercial or financial relationships that could be construed as a potential conflict of interest.
Acknowledgments
We would like to thank the BIAS-project, Finnish Meteorological Institute and Estonian Weather Service for kindly providing the data for this research. The corresponding author would also like to thank Merenkulun säätiö/Sjöfartsstiftelsen for the grant provided.
Footnotes
References
Andrew, R. K., Howe, B. M., and Mercer, J. A. (2002). Ocean ambient sound: comparing the 1960s with the 1990s for a receiver off the California coast. Acoust. Res. Lett. Online 3, 65–70. doi: 10.1121/1.1461915
Arveson, P. T., and Vendittis, D. J. (2000). Radiated noise characteristics of a modern cargo ship. J. Acoust. Soc. Am. 107, 118–129. doi: 10.1121/1.428344
Asselin, S., Hammill, M. O., and Barrette, C. (1993). Underwater vocalizations of ice breeding grey seals. Can. J. Zool. 71, 2211–2219. doi: 10.1139/z93-310
Betke, K., Folegot, T., Matuschek, R., Pajala, J., Persson, L., Tegowski, J., et al. (2015). BIAS Standards for Signal Processing. Aims, Processes and Recommendations. Amended version. 2015. Available online at: https://biasproject.files.wordpress.com/2016/01/bias_sigproc_standards_v5_final.pdf (accessed November 02, 2020).
Bivand, R., and Rundel, C. (2017). rgeos: Interface to Geometry Engine - Open Source (GEOS). R-package version 0.3-26. Available online at: https://CRAN.R-project.org/package=rgeos (accessed November 02, 2020).
Chapman, C. J., and Hawkins, A. D. (1973). A field study of hearing in the cod, Gadus morhua L. J. Comp. Physiol. 85, 147–167. doi: 10.1007/BF00696473
Commission Decision 2010/477/EU (2010). Commission Decision 2010/477/EU of 1 September 2010 on Criteria and Methodological Standards on Good Environmental Status of Marine Waters. OJ L 232/14. Available online at: http://data.europa.eu/eli/dec/2010/477(2)/oj (accessed November 02, 2020).
Commission Decision 2017/848/EU (2017). Commission Decision 2017/848/EU of 17 May 2017 Laying Down Criteria and Methodological Standards on Good Environmental Status of Marine Waters and Specifications and Standardised Methods for Monitoring and Assessment, and Repealing Decision 2010/477/EU (2017). OJ L 125/43. Available online at: http://data.europa.eu/eli/dec/2017/848/oj (accessed November 02, 2020).
Commission Directive 2017/845/EU (2017). Commission Directive 2017/845/EU of 17 May 2017 Amending Directive 2008/56/EC of the European Parliament and of the Council as Regards the Indicative Lists of Elements to be Taken into Account for the Preparation of Marine Strategies. OJ L 125/27. Available online at: http://data.europa.eu/eli/dir/2017/845/oj (accessed November 02, 2020).
Cox, K., Brennan, L. P., Gerwing, T. G., Dudas, S. E., and Juanes, F. (2018). Sound the alarm: a meta-analysis on the effect of aquatic noise on fish behavior and physiology. Glob. Change Biol. 24, 3105–3116. doi: 10.1111/gcb.14106
Dekeling, R. P. A., Tasker, M. L., Van der Graaf, A. J., Ainslie, M. A., Andersson, M. H., André, M., et al. (2014). Monitoring Guidance for Underwater Noise in European Seas, Part III: Background Information and Annexes, eds Annexes, R. Dekeling, M. Tasker, M. Ferreira, and N. Zampoukas (Luxembourg: Publications Office of the European Union). doi: 10.2788/2808
Di Franco, E., Pierson, P., Di Iorio, L., Calò, A., Cottalorda, J. M., Derijard, B., et al. (2020). Effects of marine noise pollution on Mediterranean fishes and invertebrates: a review. Mar. Pollut. Bull. 159:111450. doi: 10.1016/j.marpolbul.2020.111450
Directive 2008/56/EC (2008). Directive 2008/56/EC of the European Parliament and of the Council of 17 June 2008 Establishing a Framework for Community Action in the Field of Marine Environmental Policy (Marine Strategy Framework Directive) (2008). OJ L 164/19. Available online at: http://data.europa.eu/eli/dir/2008/56/oj (accessed November 02, 2020).
Dolman, S. J., and Jasny, M. (2015). Evolution of marine noise pollution management. Aquat. Mamm. 41, 357–374. doi: 10.1578/AM.41.4.2015.357
Enger, P. S. (1967). Hearing in herring. Comp. Biochem. Physiol. 22, 527–538. doi: 10.1016/0010-406X(67)90615-9
Erbe, C., Reichmuth, C., Cunningham, K., Lucke, K., and Dooling, R. (2016). Communication masking in marine mammals: a review and research strategy. Mar. Pollut. Bull. 103, 15–38. doi: 10.1016/j.marpolbul.2015.12.007
Farcas, A., Powell, C. F., Brookes, K. L., and Merchant, N. D. (2020). Validated shipping noise maps of the Northeast Atlantic. Sci. Total Environ. 735:139509. doi: 10.1016/j.scitotenv.2020.139509
FMI (2014c). Ice Winter 2013/2014. Available online at: http://en.ilmatieteenlaitos.fi/ice-winter-2013-2014 (accessed November 29, 2017).
Hawkins, A. D., and Johnstone, A. D. F. (1978). The hearing of the Atlantic Salmon, Salmo salar. J. Fish Biol. 13, 655–673. doi: 10.1111/j.1095-8649.1978.tb03480.x
HELCOM (2010). Maritime Activities in the Baltic Sea – An Integrated Thematic Assessment on Maritime Activities and Response to Pollution at Sea in the Baltic Sea Region. Baltic Sea Environment Proceedings No. 123. Helsinki: Helsinki Commission.
HELCOM (2018). HELCOM Assessment on Maritime Activities in the Baltic Sea 2018. Baltic Sea Environment Proceedings No. 152. Helsinki: Helsinki Commission.
Hildebrand, J. A. (2009). Anthropogenic and natural sources of ambient noise in the ocean. Mar. Ecol. Prog. Ser. 395, 5–20. doi: 10.3354/meps08353
IISD (2018). Nineteenth Meeting of the United Nations Open-Ended Informal Consultative Process on Oceans and the Law of the Sea. (Winnipeg: IISD), 18–22.
Leppäranta, M., and Myrberg, K. (2009). Physical Oceanography of the Baltic Sea. Berlin: Springer. doi: 10.1007/978-3-540-79703-6
McDonald, M. A., Hildebrand, J. A., and Wiggins, S. M. (2006). Increases in deep ocean ambient noise in the Northeast Pacific west of San Nicolas Island, California. J. Acoust. Soc. Am. 120, 711–718. doi: 10.1121/1.2216565
Merchant, N. D., Faulkner, R. C., and Martinez, R. (2017). Marine noise budgets in practice. Conserv. Lett. 11:e12420. doi: 10.1111/conl.12420
Merchant, N. D., Pirotta, E., Barton, T. R., and Thompson, P. M. (2014). Monitoring ship noise to assess the impact of coastal developments on marine mammals. Mar. Pollut. Bull. 78, 85–95. doi: 10.1016/j.marpolbul.2013.10.058
Merchant, N. D., Witt, M. J., Blondel, P., Godley, B. J., and Smith, G. H. (2012a). Assessing sound exposure from shipping in coastal waters using a single hydrophone and Automatic Identification System (AIS) data. Mar. Pollut. Bull. 64, 1320–1329. doi: 10.1016/j.marpolbul.2012.05.004
Merchant, N. D., Blondel, P., Dakin, D. T., and Dorocicz, J. (2012b). Averaging underwater noise levels for environmental assessment of shipping. J. Acoust. Soc. Am. 132:EL343. doi: 10.1121/1.4754429
Mustonen, M., Klauson, A., Andersson, M., Clorennec, D., Folegot, T., Koza, R., et al. (2019). Spatial and temporal variability of ambient underwater sound in the Baltic Sea. Sci. Rep. 9:13237. doi: 10.1038/s41598-019-48891-x
National Research and Council (2003). Ocean Noise and Marine Mammals. Washington, DC: The National Academies Press. doi: 10.17226/10564
Nichols, T. A., Anderson, T. W., and Širović, A. (2015). Intermittent noise induces physiological stress in a coastal marine fish. PLoS One 10:e0139157. doi: 10.1371/journal.pone.0139157
Nikolopoulos, A., Sigray, P., Andersson, M., Carlström, J., and Lalander, E. (2016). BIAS Implementation Plan - Monitoring and Assessment Guidance for Continuous Low Frequency Sound in the Baltic Sea. Available online at: https://biasproject.files.wordpress.com/2013/11/bias-implementation-plan.pdf (accessed November 02, 2020).
Offutt, G. C. (1974). Structures for the detection of acoustic stimuli in the Atlantic codfish, Gadus morhua. J. Acoust. Soc. Am. 56, 665–671. doi: 10.1121/1.1903306
Pijanowski, B. C., Villanueva-Rivera, L. J., Dumyahn, S. L., Farina, A., Krause, B. L., Napoletano, B. M., et al. (2011). Soundscape Ecology: the science of sound in the landscape. Bioscience 61, 203–216.
Richardson, W. J., Greene, C. R., Malme, C. I., and Thomson, D. H. (1995). Marine Mammals and Noise. San Diego, CA: Academic Press. doi: 10.1016/C2009-0-02253-3
Robinson, S. P., Lepper, P. A., and Hazelwood, R. A. (2014). Good Practice Guide for Underwater Noise Measurement. NPL Good Practice Guide No. 133. Available online at: https://www.npl.co.uk/special-pages/guides/gpg133underwater (accessed November 02, 2020).
Rolland, R. M., Parks, S. E., Hunt, K. E., Castellote, M., Corkeron, P. J., Nowacek, D. P., et al. (2012). Evidence that ship noise increases stress in right whales. Proc. R. Soc. B 279, 2363–2368. doi: 10.1098/rspb.2011.2429
Sairanen, E. E. (2014). Baltic Sea Underwater Soundscape. Weather and Ship Induced Sounds and the Effect of Shipping on Harbor Porpoise (Phocoena phocoena) Activity. Master’s thesis, University of Helsinki, Helsinki.
Sigray, P., Andersson, M., Pajala, J., Laanearu, J., Klauson, A., Tegowski, J., et al. (2016). “BIAS: a regional management of underwater sound in the Baltic Sea,” in The Effects of Noise on Aquatic Life II, eds A. N. Popper and A. D. Hawkins (New York, NY: Springer), 1015–1023. doi: 10.1007/978-1-4939-2981-8
Sills, J. M., Southall, B. L., and Reichmuth, C. (2015). Amphibious hearing in ringed seals (Pusa hispida): underwater audiograms, aerial audiograms and critical ratio measurements. J. Exp. Biol. 218, 2250–2259. doi: 10.1242/jeb.120972
Slabbekoorn, H., Bouton, N., van Opzeeland, I., Coers, A., ten Cate, C., and Popper, A. N. (2010). A noisy spring: the impact of globally rising underwater sound levels on fish. Trends Ecol. Evol. 25, 419–427. doi: 10.1016/j.tree.2010.04.005
Snoeijs-Leijonmalm, P., and Andrén, E. (2017). “Why is the Baltic Sea so special to live in?,” in Biological Oceanography of the Baltic Sea, eds P. Snoeijs-Leijonmalm, H. Schubert, and T. Radziejewska (Dordrecht: Springer), 23–84. doi: 10.1007/978-94-007-0668-2
Sueur, J., Aubin, T., and Simonis, C. (2008). Seewave: a free modular tool for sound analysis and synthesis. Bioacoustics 18, 213–226. doi: 10.1080/09524622.2008.9753600
Tuszynski, J. (2014). caTools: Moving Window Statistics, GIF, Base64, ROC AUC, etc. R package version 1.17.1. Available online at: https://CRAN.R-project.org/package=caTools (accessed November 02, 2020).
Van der Graaf, A. J., Ainslie, M. A., André, M., Brensing, K., Dalen, J., Dekeling, R. P. A., et al. (2012). European Marine Strategy Framework Directive -Good Environmental Status (MSFD GES): Report of the Technical Subgroup on Underwater Noise and Other Forms of Energy. Available online at: https://ec.europa.eu/environment/marine/pdf/MSFD_reportTSG_Noise.pdf (accessed November 02, 2020).
Veirs, S., Veirs, V., and Wood, J. D. (2016). Ship noise extends to frequencies used for echolocation by endangered killer whales. PeerJ 4, e1657. doi: 10.7717/peerj.1657
Verfuß, U. K., Andersson, M., Folegot, T., Laanearu, J., Matuschek, R., Pajala, J., et al. (2015). BIAS Standards for Noise Measurements. Background Information, Guidelines and Quality Assurance. Amended version. 2015. Available online at: https://biasproject.files.wordpress.com/2016/04/bias_standards_v5_final.pdf (accessed November 02, 2020).
Wickham, H. (2017). Tidyverse: Easily Install and Load ‘Tidyverse’ Packages. R package version 1.1.1. https://CRAN.R-project.org/package=tidyverse (accessed November 02, 2020).
Wickham, H., and Grolemund, G. (2011). Dates and times made easy with lubridate. J. Stat. Softw. 40, 1–25.
WWF (2010). Future Trends in the Baltic Sea. Available online at: http://wwf.panda.org/wwf_news/?194764/Future-trends-in-the-Baltic-Sea (accessed April 27, 2020).
Keywords: underwater noise, shipping, AIS, adaptive threshold level, Baltic Sea, intermittent noise, noise threshold
Citation: Syrjälä J, Kalliola R and Pajala J (2020) Underwater Acoustic Environment of Coastal Sea With Heavy Shipping Traffic: NE Baltic Sea During Wintertime. Front. Mar. Sci. 7:589141. doi: 10.3389/fmars.2020.589141
Received: 30 July 2020; Accepted: 22 October 2020;
Published: 12 November 2020.
Edited by:
Lotfi Rabaoui, King Fahd University of Petroleum and Minerals, Saudi ArabiaReviewed by:
Giuseppa Buscaino, National Research Council (CNR), ItalySalvatore Viola, NATO Centre for Maritime Research and Experimentation, Italy
Copyright © 2020 Syrjälä, Kalliola and Pajala. This is an open-access article distributed under the terms of the Creative Commons Attribution License (CC BY). The use, distribution or reproduction in other forums is permitted, provided the original author(s) and the copyright owner(s) are credited and that the original publication in this journal is cited, in accordance with accepted academic practice. No use, distribution or reproduction is permitted which does not comply with these terms.
*Correspondence: Joonas Syrjälä, joonas.syrjala@traficom.fi; jovisy@utu.fi