- 1Marine College, Shandong University, Weihai, China
- 2State Key Laboratory of Microbial Technology, Shandong University, Qingdao, China
A Gram-stain-negative, facultatively aerobic, beige and slender bacterium, designed N1Y132T, was isolated from a sediment sample taken from coastal zone of Weihai, China (37°34′31.44″ N, 122°9′15″ E). Growth occurred at 15-33°C (optimal 25–28°C), at pH 6.5–8.5 (optimal pH 7.5), in 0–9% (w/v) NaCl (optimal 2.0–2.5%). According to the phylogenetic analysis based on the 16S rRNA gene sequences, strain N1Y132T showed the highest sequence similarity (97.9%) with Carboxylicivirga taeanensis JCM 19490T, followed by C. sediminis JR1T (97.4%), C. mesophila JCM 18290T (96.4%) and C. linearis FB218T (95.1%). Thus, strain N1Y132T was a member of the genus Carboxylicivirga. The average nucleotide identity (ANI) values between strain N1Y132T and C. sediminis JR1T, C. linearis FB218T and C. mesophila JCM 18290T were 74.8%, 71.1%, and 74.9%, respectively. Chemotaxonomic analysis showed that the sole respiratory quinone was MK-7 and the major fatty acids (> 5.0%) included iso-C15:0, anteiso-C15:0, iso-C13:0, iso-C14:0, iso-C15:0 3-OH, and C15:1ω6c. The polar lipids were consisted of a phosphatidylethanolamine, two phosphoaminolipids and six unidentified lipids. The DNA G + C content was 38.1 mol%. Based on the evidence presented in this study, strain N1Y132T represents a novel species of the genus Carboxylicivirga, for which the name Carboxylicivirga marinus sp. nov. is proposed. The type strain is N1Y132T (= KCTC 72934T = MCCC 1H00431T).
Introduction
The genus Carboxylicivirga, a member of family Marinilabiliaceae of the phylum Bacteroidetes, was originally proposed by Yang et al. (2014). Bacteria of the genus Carboxylicivirga are Gram-stain-negative and slender, and the major respiratory quinone is MK-7. At the time of writing, 5 species are assigned to the genus, including C. mesophila (Yang et al., 2014), C. taeanensis (Yang et al., 2014), C. linearis (Wang et al., 2015), C. flava (Wang et al., 2016), and C. sediminis (Wang F. Q. et al., 2018). Without exception, members of the genus Carboxylicivirga are isolated from marine sediments. The 16S rRNA gene sequencing revealed that Carboxylicivirga was the coregenera in the gut of marine invertebrates, such as the mud crab Scylla paramamosain (Wei et al., 2019) and the lobster Homarus gammarus (Holt et al., 2019). However, the function of this newly recognized genus in the environment or in the host are less known. In this study, a beige, non-motile and facultatively aerobic bacterium, strain N1Y132T, is proposed as representing a novel species of genus Carboxylicivirga. Comparative analysis of genomes of strain N1Y132T and reference strains C. sediminis JR1T, C. linearis FB218T, and C. mesophila JCM 18290T are contributed to understand the function of genus Carboxylicivirga.
Materials and Methods
Bacterial Isolation and Cultivation
Strain N1Y132T was isolated from a sediment sample taken from coastal zone of Weihai, China (37°34′31.44″ N, 122°9′15″ E), in October 2018. For the bacterial isolation, the sediment sample was homogenized and 10-fold serially diluted to 10–5 with sterile seawater and 100 μl aliquot of each dilution was spread on marine agar 2216 (MA; Becton Dickinson) and incubated at 28°C for 21 days. The strain, designed N1Y132T, was incubated on MA at 28°C and was stored at −80°C in sterile 1% (w/v) saline supplemented with 15% (v/v) glycerol. The type strains C. mesophila JCM 18290T (obtained from Japan Collection of Microorganisms, Japan), C. taeanensis JCM 19490T (obtained from Japan Collection of Microorganisms, Japan), C. sediminis JR1T (generously provided by Wang FQ, Wang F. Q. et al., 2018) and C. linearis FB218T (storage in our lab) were used as reference strains and cultivated routinely on MA at 28°C.
Phenotypic, Physiological, and Biochemical Characteristics
The morphological and physiological features of strain N1Y132T were examined after incubation at 28±C for 3 days on MA plates. Cell morphology and size were observed by light microscope (E600, Nikon) and transmission electron microscope (JEM-1200, Jeol). Gram reaction was determined using the method described by Smibert and Krieg (1994). Motility was examined on MA soft agar (0.3% agar). Gliding motility was examined according to the method described by Bowman (2000). Growth range and optimum of temperature were evaluated by observation of visible colonies on modified MA at various temperatures (0, 4, 10, 15, 20, 25, 28, 30, 33, 37, 40, and 45±C). The effects of different salt concentrations on growth were tested by using a medium comprised of 0.1% yeast extract, 0.5% peptone and 1.8% agar, prepared with artificial seawater (0.32% MgSO4, 0.23% MgCl2, 0.12% CaCl2, 0.07% KCl and 0.02% NaHCO3, all w/v) and containing different concentrations of NaCl (0, 0.5, 1, 1.5, 2, 2.5, 3, 4, 5, 6, 7, 8, 9, 10, 11, and 12%, w/v). The pH range (from pH 5.5 to 9.5, in intervals of 0.5 pH units) and optimal pH for growth was tested in marine broth 2216 (MB) medium by using the appropriate buffer solution, including MES (pH 5.5 and 6.0), PIPES (pH 6.5 and 7.0), HEPES (pH 7.5 and 8.0), Tricine (pH 8.5), and CAPSO (pH 9.0 and 9.5). The HCl or NaOH was used to adjust the pH of the medium before autoclaving. Growth under anaerobic (10% H2, 10% CO2, and 80% N2) and microaerobic (5% O2, 10% CO2, and 85% N2) conditions was examined after incubation for 2 weeks on MA in an anaerobic jar with or without 0.1% (w/v) KNO3. Catalase activity was detected by bubble production in 3% (v/v) H2O2. Oxidase activity was determined using an oxidase reagent kit (bioMérieux) according to the manufacturer’s instructions. Antibiotic susceptibility of strain N1Y132T was investigated on MA plates at 28±C for up to 3 days (0.5 McFarland standard) using the disc diffusion method as described previously (Du et al., 2014) and according to guidelines of the Clinical and Laboratory Standards Institute (CLSI, 2012). Bacterial abilities to hydrolyze agar, DNA, alginate, starch, casein, CM-cellulose and Tweens (20, 40, 60, and 80) were tested according to the methods from Smibert and Krieg (1994). Nitrogen fixation ability was tested by using a nitrogen free medium comprised of 0.1% starch and 1.8% agar, prepared with artificial seawater (0.32% MgSO4, 0.23% MgCl2, 0.12% CaCl2, 0.07% KCl and 0.02% NaHCO3, all w/v) at 28±C for 2 weeks in aerobic and anaerobic conditions (Wang H. B. et al., 2018). Additional physiological and biochemical characteristics were determined using API 20E and API ZYM kits (bioMérieux). Tests of acid production from carbohydrates were detected using the API 50CHB fermentation kit (bioMérieux). Oxidation of substrate was examined in Biolog GEN III MicroPlates. All API tests were performed according to the manufacturer’s instructions (except for salinity, which was adjusted to 3%).
16S rRNA Gene Sequence Analysis
The 16S rRNA gene was amplified by PCR using the Taq DNA polymerase with the universal primers 27F and 1492R (Lane, 1991). The purified PCR product with 3′-A overhangs was cloned into a pMD18-T vector (Takara) based on the TA cloning method, and the ligation product was transformed into Escherichia coli DH5α cells. The positive clones were selected and sequenced bi-directionally with primers M13-47 and RV-M at BGI Co. Ltd (Qingdao, China) using the ABI 3730XL system. Thus, a near-complete 16S rRNA gene sequence (1500 bp) of strain N1Y132T was gained. The determined 16S rRNA gene sequence for strain N1Y132T was submitted to the National Centre for Biotechnology Information (NCBI) GenBank database. The 16S rRNA gene similarities were calculated using the EzBioCloud Database (Yoon et al., 2017). Multiple sequences were aligned using the CLUSTAL_X program (Thompson et al., 1997). In order to determine the phylogenetic position of strain N1Y132T, phylogenetic trees were constructed using the neighbor-joining (NJ) (Saitou and Nei, 1987), maximum-likelihood (ML) (Fitch, 1971) and maximum-parsimony (MP) (Felsenstein, 1981) methods in MEGA version 7.0 (Kumar et al., 2016). The reliability of relationships was ensured by performing bootstrap analyses based on 1000 replications.
Chemotaxonomic Characterization
In order to determine the chemotaxonomic features, the cells of strain N1Y132T and C. sediminis JR1T, C. linearis FB218T, C. mesophila JCM 18290T, and C. taeanensis JCM 19490T were harvested by centrifuge and subjected to freeze-drying after cultivation in marine broth 2216 medium for 3 days at 28±C. Fatty acid methyl esters (FAMEs) were extracted from 30 mg freeze-dried biomass according to the standard protocol of MIDI (Sherlock Microbial Identification System, version 6.1). Fatty acids were prepared and analyzed by using an Agilent 6890N gas chromatograph. Peaks were automatically integrated, and fatty acid names and percentages were determined using the TSBA40 database of the Microbial Identification system (Sasser, 1990). Respiratory quinones of strain N1Y132T were obtained from 300 mg freeze-dried cells using the two-stage method described by Tindall et al. (2007) and analyzed by HPLC (Kroppenstedt, 1982). Polar lipids were extracted from 50 mg of freeze-dried cell material using a chloroform/methanol system according to the procedures described by Komagata and Suzuki (Komagata and Suzuki, 1988). Polar lipids were analyzed by two-dimensional thin layer chromatography according to Minnikin et al. (1984).
Genomic Analysis
Genomic DNAs of strain N1Y132T and reference strains C. sediminis JR1T, C. linearis FB218T, and C. mesophila JCM 18290T were extracted by using a genomic DNA extraction kit (Takara) according to the manufacturer’s recommendations. The draft genome sequence of N1Y132T, C. sediminis JR1T, C. linearis FB218T, and C. mesophila JCM 18290T were sequenced on the Illumina HiSeq PE150 platform at Beijing Novogene Bioinformatics Technology (Beijing, China). The genome sequence was deposited in the DDBJ/GenBank/EMBL database. The G + C content of the chromosomal DNA was calculated using genome sequence. Homologous genomic regions of four Carboxylicivirga species were analyzed using progressiveMauve algorithm (Darling et al., 2004). Core genes and specific genes of four strains were analyzed by the CD-HIT (Fu et al., 2012) rapid clustering of similar proteins software with a threshold of 40% pairwise identity and 0.4 length difference cutoff in amino acid. Then, the Venn figure was drawn to show their relationships among the samples.
The genes of involved in N1Y132T metabolic pathways were analyzed using the KofamKOALA (Aramaki et al., 20201) and the NCBI Prokaryotic Genome Annotation Pipeline (PGAP) (Tatusova et al., 20162). Carbohydrate-active enzymes of strain N1Y132T and three reference strains were annotated using the dbCAN2 meta server (Zhang et al., 2018). The presence of gene clusters encoding secondary metabolites of strain N1Y132T was predicted by using the antiSMASH 6.0 database (Blin et al., 2021). The average nucleotide identity (ANI) was calculated using ANI calculator (Lee et al., 20163). In addition, digital DNA-DNA hybridization (dDDH) values between strain N1Y132T and reference strains were obtained using the genome-to-genome distance calculator (GGDC) website (Meier-Kolthoff et al., 2013)4.
Results and Discission
Morphological, Physiological, and Biochemical Characteristics
Transmission electron microscopy showed that the cells of strain N1Y132T were slender and non-flagellar (Supplementary Figure 1), which were similar with the closely related strains C. sediminis JR1T, C. linearis FB218T, C. mesophila JCM 18290T, and C. taeanensis JCM 19490T. However, cells of strain N1Y132T were longer than these related strains (Table 1). Strain N1Y132T was susceptible to clarithromycin (15 μg), ampicillin (10 μg), chloramphenicol (30 μg), erythromycin (15 μg), rifampicin (5 μg), ceftriaxone (30 μg), penicillin (10 μg), cefotaxime sodium (30 μg) and carbenicillin (100 μg), but resistant to vancomycin (30 μg), gentamicin (10 μg), polymyxin B (300 μg), ofloxacin (5 μg), norfloxacin (10 μg), lincomycin (2 μg), tetracycline (30 μg), and tobramycin (10 μg). The strain N1Y132T was positive for the hydrolysis of starch and gelatin, but negative for agar, DNA, alginate, casein, CM-cellulose and Tweens (20, 40, 60, and 80). According to the results of commercial kits, all the five strains showed positive reactions for alkaline phosphatase, acid phosphatase, naphthol-AS-Biphosphohydrolase, N-acetyl-β-glucosaminidase and esculin, but negative reactions for esterase (C4), esterase lipase (C8), α-galactosidase, H2S production and D-adonitol. Given these positive results, the ability of strain N1Y132T to utilize substrate was weaker than that of these three reference strains. Detailed phenotypical features were listed in species description as well as in Table 1 and the results of commercial kits were listed in Supplementary Table 1.
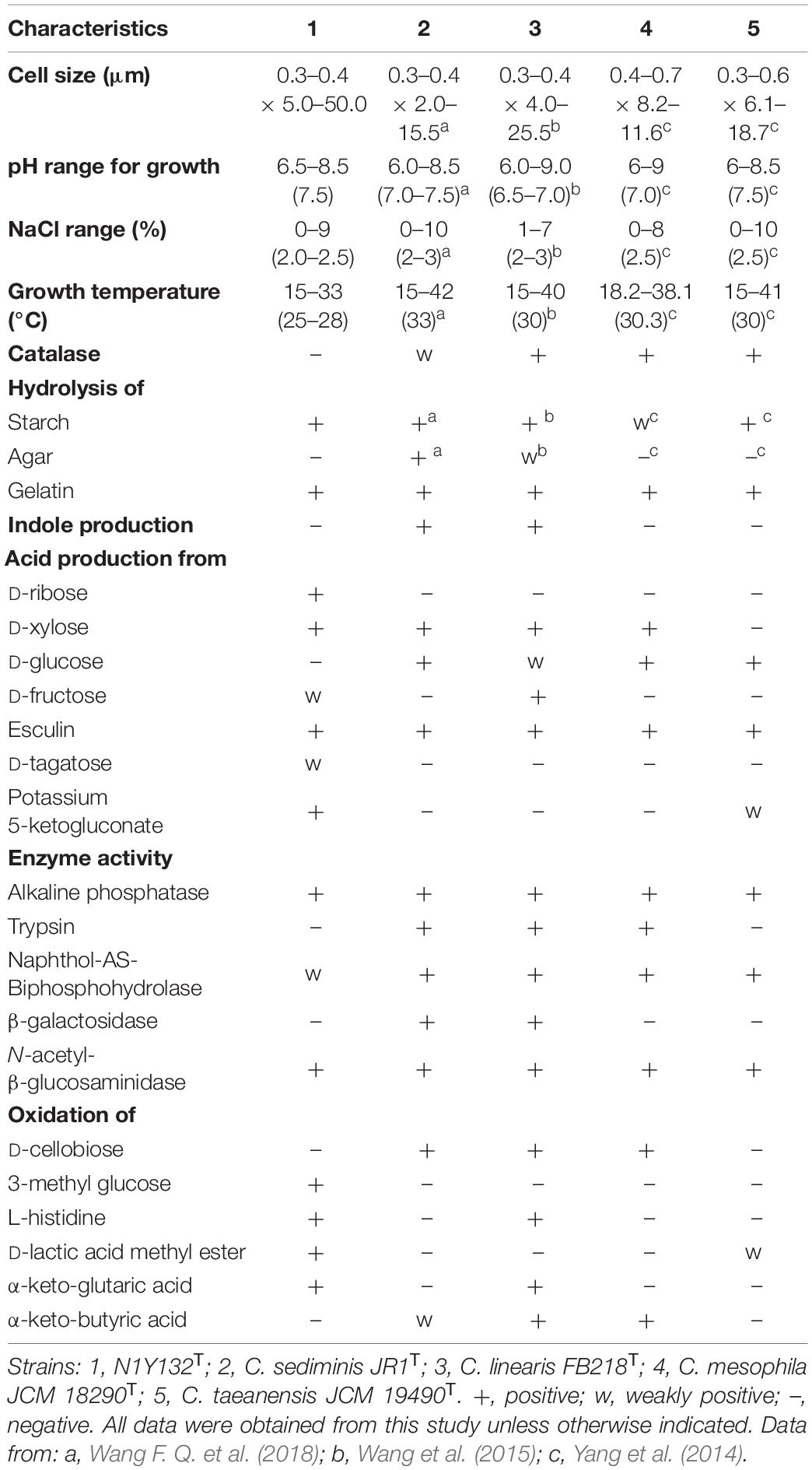
Table 1. Differential phenotypic characteristics of strain N1Y132T and the related Carboxylicivirga species.
Phylogenetic Analysis of 16S rRNA Gene Sequences
After PCR amplification and sequencing, a near-complete 16S rRNA gene sequence (1500 bp) of strain N1Y132T was obtained and subjected to comparative analysis. BLAST searches revealed that strain N1Y132T shared the highest 16S rRNA gene sequence similarity with C. taeanensis JCM 19490T (97.9%, Figure 1A). In addition, strain N1Y132T shared the 16S rRNA gene sequence similarity of 96.4% with C. mesophila JCM 18290T, which was the type species of the genus Carboxylicivirga. In the resulting NJ tree, strain N1Y132T was placed within the clade comprising the genus Carboxylicivirga and was located nearest to C. sediminis JR1T (Figure 1B). MP and ML trees also showed similar tree topologies. Both the sequence similarities and phylogenetic relationships indicated that strain N1Y132T may represent a novel species of the genus Carboxylicivirga.
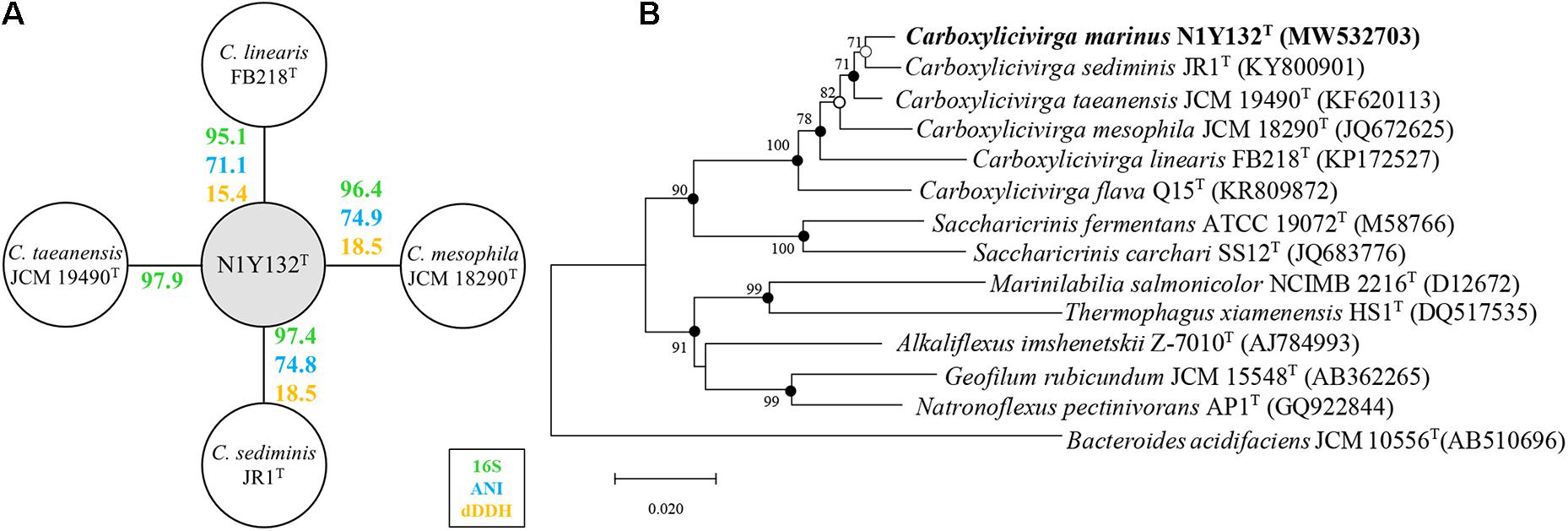
Figure 1. Phylogenetic relationship of strain N1Y132T and other members of family Marinilabiliaceae. (A) The similarities shared between strain N1Y132T, C. taeanensis JCM 19490T, C. sediminis JR1T, C. mesophila JCM 18290T, and C. linearis FB218T for 16S rRNA gene sequence similarity (16S), average nucleotide identity (ANI) and digital DNA-DNA hybridization (dDDH). The 16S rRNA gene similarities were calculated using the EzBioCloud Database. Average nucleotide identity (ANI) was calculated using ANI calculator (https://www.ezbiocloud.net/tools/ani/). The digital DNA-DNA hybridization (dDDH) were calculated using the genome-to-genome distance calculator (GGDC) website (http://ggdc.dsmz.de/distcalc2.php). (B) Neighbor-joining phylogenetic tree based on 16S rRNA gene sequences, showing the relationships of strain N1Y132T and related species. Bootstrap values ≥70% (based on 1000 replications) are shown at branching nodes. Filled circles indicate that the corresponding nodes were also recovered in the trees generated with the maximum-likelihood and maximum-parsimony algorithms. Open circles indicate that the corresponding nodes were also recovered in either the maximum-likelihood or maximum-parsimony algorithm. Bacteroides acidifaciens JCM 10556T (GenBank accession number, AB510696) was used as an outgroup. Bar, 0.02 substitutions per nucleotide position.
Chemotaxonomic Characteristics
The major fatty acids (> 5%) of strain N1Y132T were iso-C15:0 (20.7%), anteiso-C15:0 (10.2%), iso-C13:0 (6.7%), iso-C14:0 (6.6%), iso-C15:0 3-OH (6.2%), and C15:1 ω6c (6.0%), which were similar in composition to those of other species in genus Carboxylicivirga (Table 2). However, some differences between strain N1Y132T and other Carboxylicivirga species were observed. For example, C. mesophila JCM 18290T, C. linearis FB218T and C. taeanensis JCM 19490T contained C13:1 AT 12-13, which was not detected in strain N1Y132T and C. sediminis JR1T. The respiratory quinone of strain N1Y132T was determined to be MK-7 (Kroppenstedt, 1982), which was in line with the description of the genus Carboxylicivirga (Yang et al., 2014). The polar lipids of strain N1Y132T were comprised of a phosphatidylethanolamine, two phosphoaminolipids and unidentified lipids 1-6 (Supplementary Figure 2). Phosphatidylethanolamine and several unknown polar lipids were detected predominantly in tested Carboxylicivirga species, in line with the earlier report (Wang F. Q. et al., 2018).
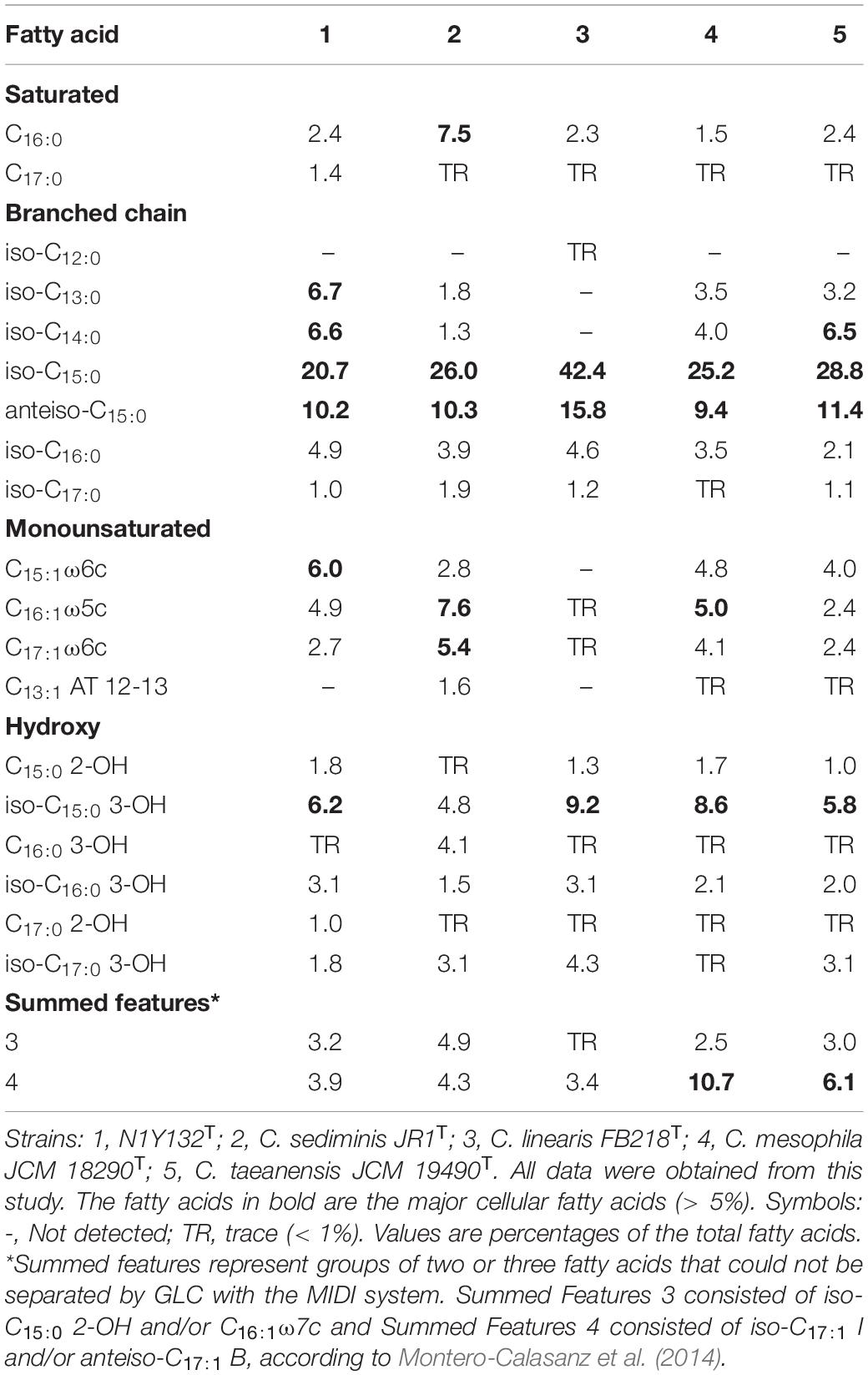
Table 2. Cellular fatty acid contents (%) of strain N1Y132T and the related Carboxylicivirga species.
Genome Insight of Four Strains Belonging to Carboxylicivirga Genus
Basic Characteristics of Four Genomes
The genome length of strain N1Y132T, C. mesophila JCM 18290T, C. linearis FB218T, and C. sediminis JR1T were less differentiating these four strains (Table 3). Among them, C. linearis FB218T harbors the largest genome (6,050,047 bp, JAGUCO000000000), while the new strain N1Y132T holds the smallest one (5,649,616 bp, JAENRR000000000). The sequencing depth of coverage and the contig numbers are different for these four draft genomes. However, some basic genome characteristics have been analyzed. The DNA G + C content of strain N1Y132T was 38.1 mol%, which was higher than that of C. linearis FB218T (36.4 mol%), but lower than that of C. mesophila JCM 18290T (41.2 mol%) and C. sediminis JR1T (40.9 mol%).
A total of 4,463 genes were predicted in the genome of strain N1Y132T by the NCBI PGAP, including 4,383 protein-coding genes, 59 tRNAs, 3 rRNAs, and 3 ncRNAs. The amino acid sequence of strain N1Y132T are annotated by KEGG (Supplementary Figure 3), and 1976 genes (39.1%) could be assigned a putative function, including carbohydrate metabolism (225 genes), signaling and cellular processing (213 genes), genetic information processing (194 genes), amino acid metabolism (126 genes), environmental information processing (115 genes) and metabolism of cofactors and vitamins (107 genes), glycan biosynthesis and metabolism (78 genes) and energy metabolism (70 genes). The results of C. sediminis JR1T, C. linearis FB218T, and C. mesophila JCM 18290T and the comparison with strain N1Y132T were shown in Supplementary Figure 3.
Four Carboxylicivirga genomes are similar in the gene number and protein function prediction. The homologous gene regions have been showed by the progressiveMauve algorithm (Supplementary Figure 4). Subsequently, core genes and specific genes of four strains were analyzed with a threshold of 40% pairwise identity and 0.4 length difference cutoff in amino acid (Figure 2). These four strains shared 2082 core genes. The genome of C. linearis FB218T seem to hold the most unique genes of 1766. The resulting dDDH values between the genomes of strain N1Y132T and C. sediminis JR1T, C. mesophila JCM 18290T, and C. linearis FB218T are 18.5%, 18.5%, and 15.4%, respectively (Figure 1A). The dDDH values between strain N1Y132T and the reference strains are less than 20%, which are far below the threshold (70%) for new species identification (Goris et al., 2007). The ANI values between strain N1Y132T and C. mesophila JCM 18290T, C. sediminis JR1T, and C. linearis FB218T are 74.9%, 74.8%, and 71.1%, respectively (Figure 1A). These values are below the proposed cut-off for a species boundary of 95-96% ANI (Richter and Rossello-Mora, 2009). These results support that the new isolate represents a novel species of the genus Carboxylicivirga.
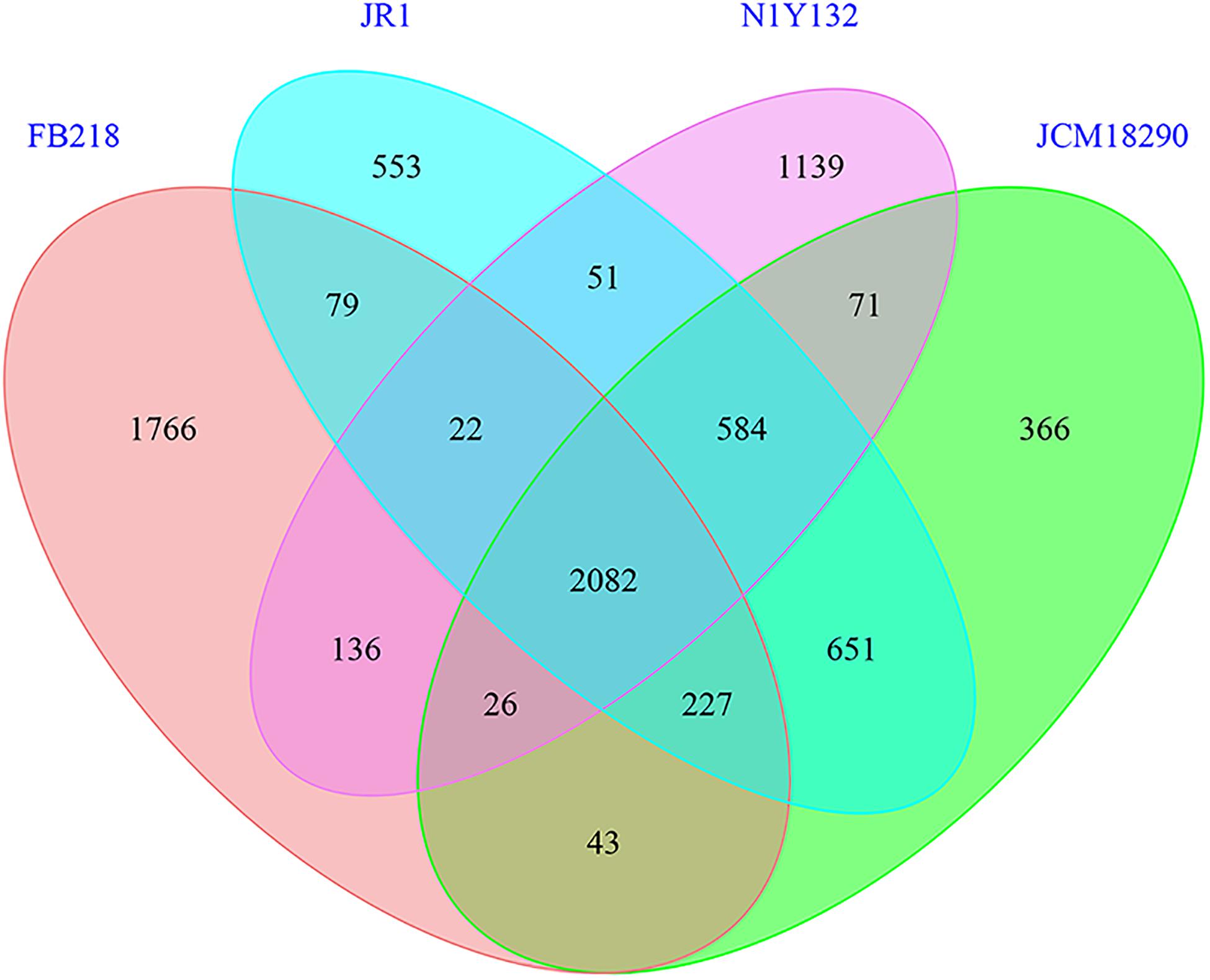
Figure 2. Distribution of orthologous genes in the genomes of the four Carboxylicivirga species (strain N1Y132T, C. mesophila JCM 18290T, C. linearis FB218T, and C. sediminis JR1T) tested by the CD-HIT rapid clustering of similar proteins software. 2082 core gene clusters that were represented in all four genomes. Strain N1Y132T, C. mesophila JCM 18290T, C. linearis FB218T, and C. sediminis JR1T contained 1139, 366, 1766, and 553 species-specific gene clusters, respectively. The clustering results were used to generate Venn’s diagram (Supplementary Data 1).
Metabolic Pathways
Many complete metabolic pathways have been found in the genomes of these four strains (Figure 3). All four strains hold the complete lipid metabolism, nucleotide metabolism and glycan metabolism. For instance, a phosphatidylethanolamine biosynthesis pathway (M00093) has been identified in these four genomes, which is in accordance with the polar lipids results of the genus Carboxylicivirga (Supplementary Figure 2). For the carbohydrate metabolism, the Carboxylicivirga strains possess complete pathways of glycolysis, gluconeogenesis, pyruvate oxidation, citrate cycle, pentose phosphate pathway (M00006), and phosphoribosyl diphosphate (PRPP) biosynthesis. However, the C. sediminis JR1T and C. linearis FB218T are deficient in the pentose phosphate pathways (M00004 and M00007), and the C. sediminis JR1T and C. mesophila JCM 18290T are devoid of the other carbohydrate metabolism (M00631D-galacturonate degradation). For the amino acid metabolism pathways, only the strain N1Y132T has the ability of lysine biosynthesis, and both C. sediminis JR1T and C. mesophila JCM 18290T contain the tryptophan biosynthesis pathway. For the metabolism of cofactors and vitamins, the strain N1Y132T is lack of riboflavin, biotin and cobalamin biosynthesis, which differentiate between strain N1Y132T and other three Carboxylicivirga strains.
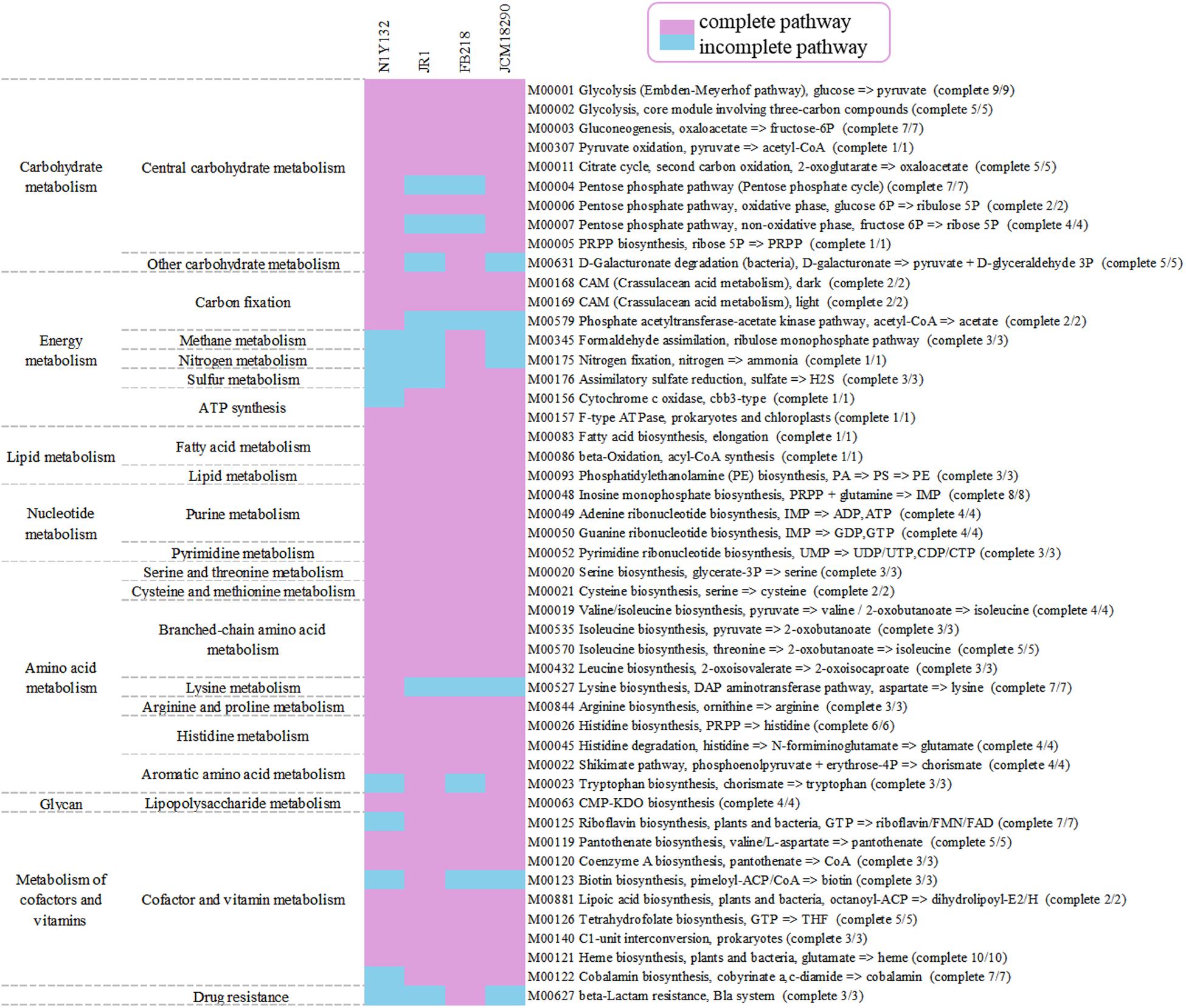
Figure 3. Heat maps of complete and incomplete metabolic pathways in the genomes of strain N1Y132T, C. sediminis JR1T, C. linearis FB218T, and C. mesophila JCM 18290T based on KEGG database.
The energy metabolism pathways exhibited the most striking difference among these four Carboxylicivirga strains. All strains have two carbon fixation metabolism pathways [CAM (Crassulacean acid metabolism), dark and light]. In many bacteria, CAM achieves carbon fixation through phosphoenolpyruvate carboxylase (EC: 4.1.1.31), malate dehydrogenase (EC:1.1.1.37), and PPDK (EC:2.7.9.1), the specific process is shown in Figure 4A. Only the strain N1Y132T has a phosphate acetyltransferase-acetate kinase pathway, which is one of carbon fixation metabolism pathways. However, both C. linearis FB218T and C. mesophila JCM 18290T have a complete assimilatory sulfate reduction pathway, wherein sulfate is reduced to cysteine and sequentially incorporated into the biomass. Assimilatory sulfate reduction of C. linearis FB218T and C. mesophila JCM 18290T is accomplished through four steps sequentially mediated by sulfate adenylyltransferase (EC 2.7.7.4; cysNC, cysN, cysD), adenylyl-sulfate kinase (EC 2.7.1.25; cysNC), PAPS reductase (EC 1.8.4.8; cysH) and assimilatory sulfite reductase (NADPH) (EC 1.8.7.2; cysJ, cysI) (Figure 4B). In the genomes of the strains N1Y132T and C. sediminis JR1T, a homologous gene cluster contain cysN, cysD, cysH, cysJ, and cysI, but lack of the cysNC gene. Additionally, C. linearis FB218T has a complete nitrogen fixation pathway (Figure 3). C. linearis FB218T could grow on the mineral salts medium plates containing starch and agar but lacking NH4+, NO3–, or organic nitrogen, while other Carboxylicivirga strains could not grow.
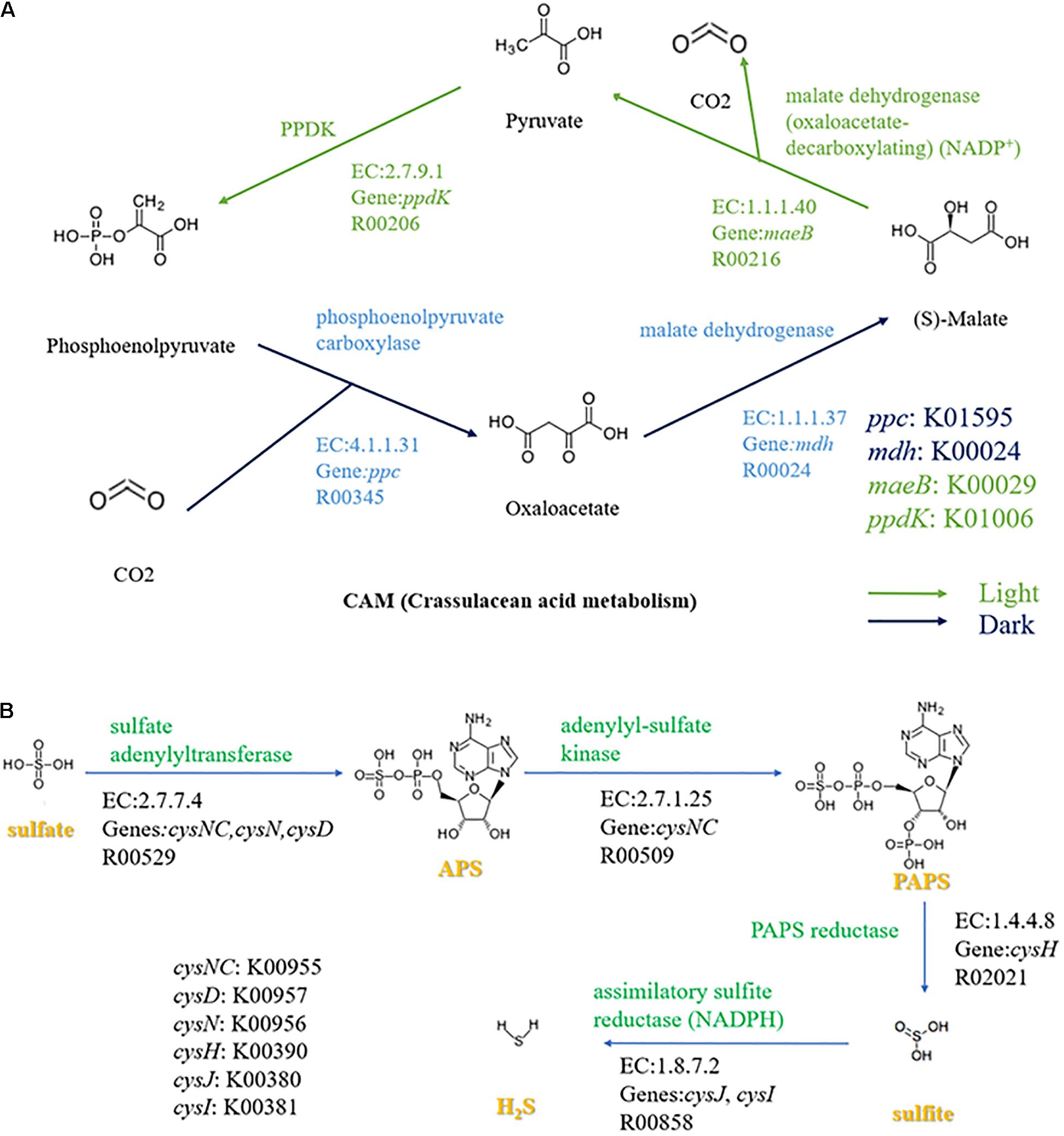
Figure 4. Carbon and sulfur metabolism of Carboxylicivirga strains N1Y132T, C. sediminis JR1T, C. linearis FB218T, and C. mesophila JCM 18290T. (A) Crassulacean acid metabolism (CAM) cycle in dark and light of four strains in genus Carboxylicivirga. The green arrows and blue arrows indicate the progression of light and dark reactions, respectively. (B) Assimilatory sulfate reduction pathway in Carboxylicivirga species based on KEGG analysis. Other reactants and products have been omitted.
Prediction of Secondary Metabolites
Based on secondary metabolite analysis predicted by antiSMASH, the genome of strain N1Y132T, C. sediminis JR1T, C. linearis FB218T and C. mesophila JCM 18290T share four gene clusters encoding for the RRE (RRE-element containing cluster), the terpene cyclase, the NRPS-like (non-ribosomal peptides) and RiPP-like (Other unspecified ribosomally synthesized and post-translationally modified peptide product) cluster (Supplementary Figure 5). The strain N1Y132T have 7 putative biosynthetic gene clusters (BGCs). These clusters include one RRE-containing protocluster, one RiPP-like biosynthetic cluster, two NRPS-like clusters, two terpene cyclase clusters and one hserlactone region. For bacteria living in coastal waters, the possession of multiple BGCs that can produce secondary metabolites may help them grow better (Lim et al., 2020). Besides, strain N1Y132T and C. linearis FB218T are found to have unique gene cluster, strain N1Y132T has a hserlactone region (Homoserine lactone cluster) and C. linearis FB218T has Type I PKS (Polyketide synthase).
Carbohydrate-Active Enzymes (CAZymes)
Given the polysaccharides utilization of marine Bacteroidetes bacteria (Krüger et al., 2019), these four Carboxylicivirga genomes have been analyzed by the CAZy database. Strain N1Y132T, C. sediminis JR1T, C. linearis FB218T and C. mesophila JCM 18290T contain 255, 296, 333 and 287 carbohydrate-active enzymes, respectively (Figure 5). Among these carbohydrate-active enzymes, glycoside hydrolases (GHs) are the greatest number of enzymes (more than 60% of the identified enzymes were assigned to the GH family in the genomes of four strains). Compared with three reference strains, strain N1Y132T harbor 255 carbohydrate-active enzymes including 172 GHs, which were obviously lower than C. sediminis JR1T, C. linearis FB218T and C. mesophila JCM 18290T. The results obtained from the CAZy database suggest that strain N1Y132T and three reference strains may have a metabolic potential for polysaccharide utilization. The experimental results show that strains N1Y132T, C. sediminis JR1T, C. linearis FB218T and C. mesophila JCM 18290T can utilize various carbon sources (Table 1 and Supplementary Table 1), which are consistent with the genome annotation results.
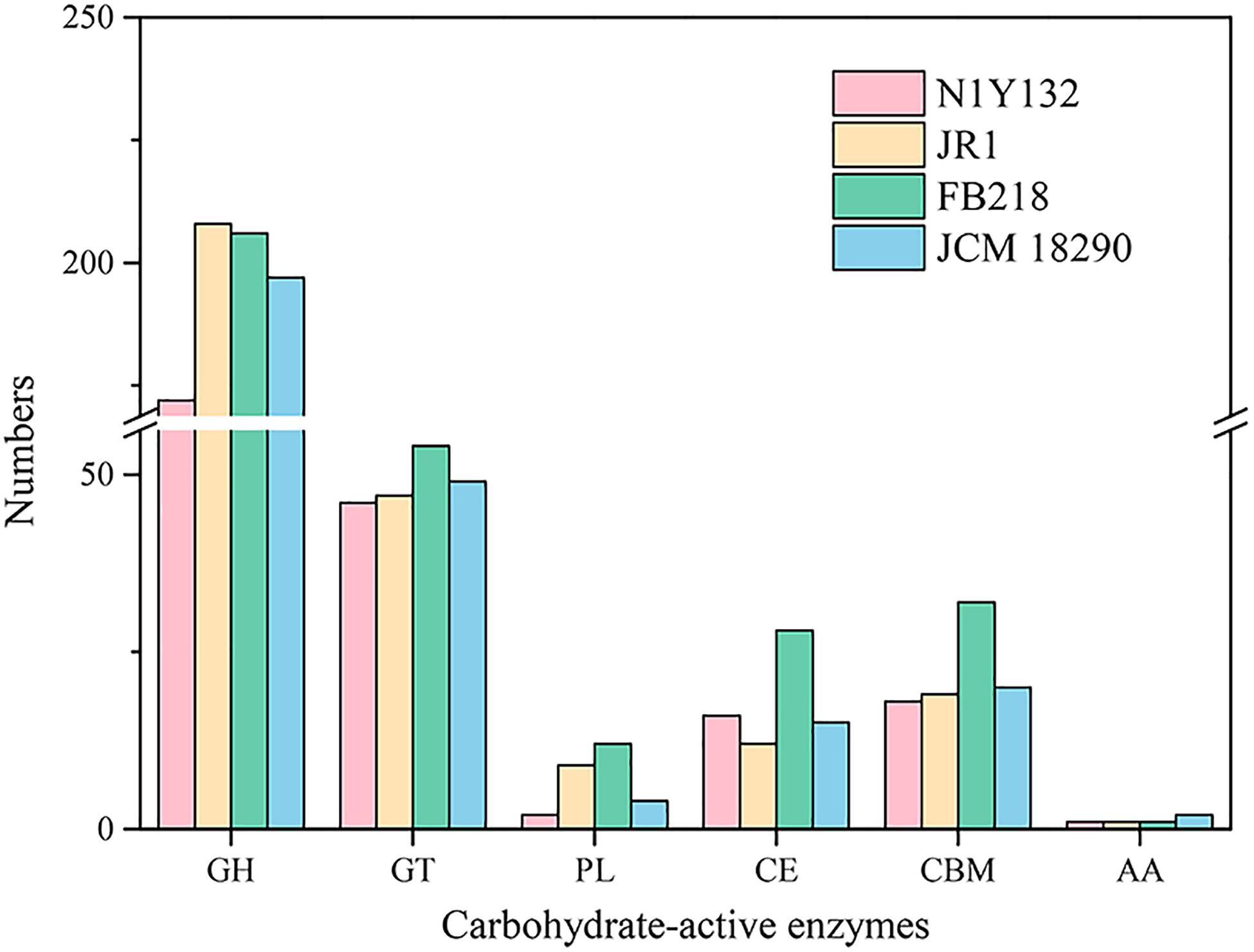
Figure 5. Histogram of predicted carbohydrate-active enzymes (CAZymes) in strain N1Y132T, C. sediminis JR1T, C. linearis FB218T, and C. mesophila JCM 18290T. Numbers of each enzyme detected in the genome were shown in the map.
According to the polyphasic taxonomic characterization results, strain N1Y132T could be classified as a new member of the genus Carboxylicivirga, for which the name Carboxylicivirga marinus sp. nov. was proposed.
Description of Carboxylicivirga marinus sp. nov.
Carboxylicivirga marinus (ma.ri’nus. L. masc. adj. marinus of or belonging to the sea, marine).
Cells of strain N1Y132T are slender, Gram-stain-negative, facultatively aerobic, non-motile and approximately 0.3–0.4 μm width and 5–50 μm length. Colonies on MA are circular, beige and smooth, and approximately 1.0 mm in diameter after 3 days of incubation at 28°C. Growth occurs at 15–33°C (optimal 25–28±C), at pH 6.5–8.5 (optimal pH 7.5), in 0–9% (w/v) NaCl (optimal 2.0–2.5%). Cells are negative for oxidase and catalase activity. Positive for hydrolysis of starch. Negative for hydrolyses of agar, DNA, alginate, casein, CM-cellulose and Tweens (20, 40, 60, and 80). Activities of alkaline phosphatase, alkaline phosphatase, leucine arylamidase, α-chymotrypsin, acid phosphatase, naphthol-AS-Biphosphohydrolase, α-glucosidase, N-acetyl-β-glucosaminidase. Acid is produced from D-ribose, D-xylose, D-fructose (weekly), esculin, starch, D-tagatose (weekly) and potassium 5-ketogluconate. In carbon source oxidation test, positive results are obtained for 3-methyl glucose, L-histidine, glucuronamide, α-keto-glutaric acid and L-malic acid. The major fatty acids are iso-C15:0, anteiso-C15:0, iso-C13:0, iso-C14:0, iso-C15:0 3-OH, and C15:1ω6c. The sole respiratory quinone is MK-7. The polar lipids consist of a phosphatidylethanolamine, two phosphoaminolipids and six unidentified lipids. The DNA G + C content of the type strain is 38.1 mol%.
The type strain, N1Y132T (= KCTC 72934T = MCCC 1H00431T) was isolated from marine sediment sample obtained from the coast of Weihai, China (37°34′31.44″ N, 122°9′15″ E). The GenBank accession number for the 16S rRNA gene sequence of strain N1Y132T is MW532703 and the draft genome data has been deposited in GenBank under the accession number JAENRR000000000.
Data Availability Statement
The GenBank accession number for the 16S rRNA gene sequence of strain N1Y132T is MW532703 and the whole genome shotgun project of strain N1Y132T, C. sediminis JR1T, C. linearis FB218T, and C. mesophila JCM 18290T have been deposited at DDBJ/ENA/GenBank under the accession number JAENRR000000000, JAGTAR000000000, JAGUCO000000000, JAGUCN000000000, respectively.
Author Contributions
D-CL isolated the Strain N1Y132T. T-SZ, Y-NZ, YG, and Z-JD performed material preparation, experimental operation, data collection, and analysis. T-SZ, YG, and Z-JD wrote the manuscript. YG and Z-JD performed project guidance and critical revision of manuscripts. All authors contributed to the article and approved the submitted version.
Funding
This work was supported by the National Natural Science Foundation of China (32070002, 31900091, and 31770002), the National Science and Technology Fundamental Resources Investigation Program of China (2019FY100700), the China Postdoctoral Science Foundation (2019M652369), and the State Key Laboratory of Microbial Technology Open Projects Fund (M2019-08).
Conflict of Interest
The authors declare that the research was conducted in the absence of any commercial or financial relationships that could be construed as a potential conflict of interest.
Publisher’s Note
All claims expressed in this article are solely those of the authors and do not necessarily represent those of their affiliated organizations, or those of the publisher, the editors and the reviewers. Any product that may be evaluated in this article, or claim that may be made by its manufacturer, is not guaranteed or endorsed by the publisher.
Supplementary Material
The Supplementary Material for this article can be found online at: https://www.frontiersin.org/articles/10.3389/fmars.2021.731212/full#supplementary-material
Abbreviations
KCTC, Korea Collection for Type Culture; MCCC, Marine Culture Collection of China; JCM, Japan Collection of Microorganisms; NJ, neighbor-joining; ME, minimum-evolution; ML, maximum-likelihood; MA, marine agar 2216; MB, marine broth 2216; ANI, average nucleotide identity; dDDH, digital DNA-DNA hybridization; HPLC, High Performance Liquid Chromatography.
Footnotes
- ^ https://www.genome.jp/tools/kofamkoala/
- ^ https://www.ncbi.nlm.nih.gov/genome/annotation_prok/
- ^ https://www.ezbiocloud.net/tools/ani/
- ^ http://ggdc.dsmz.de/distcalc2.php
References
Aramaki, T., Blanc-Mathieu, R., Endo, H., Ohkubo, K., Kanehisa, M., Goto, S., et al. (2020). KofamKOALA: KEGG Ortholog assignment based on profile HMM and adaptive score threshold. Bioinformatics 36, 2251–2252. doi: 10.1093/bioinformatics/btz859
Blin, K., Shaw, S., Kloosterman, A. M., Charlop-Powers, Z., van Wezel, G. P., Medema, M. H., et al. (2021). antiSMASH 6.0: improving cluster detection and comparison capabilities. Nucleic Acids Res. 49, W29–W35. doi: 10.1093/nar/gkab335
Bowman, J. P. (2000). Description of Cellulophaga algicola sp. nov., isolated from the surfaces of Antarctic algae, and reclassification of Cytophaga uliginosa (ZoBell and Upham 1944) Reichenbach 1989 as Cellulophaga uliginosa comb. nov. Int. J. Syst. Evol. Microbiol. 50, 1861–1868. doi: 10.1099/00207713-50-5-1861
CLSI (2012). Performance Standards for Antimicrobial Susceptibility Testing, Twenty-Second Informational Supplement CLSI Document M100-S22. Wayne, PA: Clinical and Laboratory Standards Institute.
Darling, A. C. E., Mau, B., Blattner, F. R., and Perna, N. T. (2004). Mauve: multiple alignment of conserved genomic sequence with rearrangements. Genome Res. 14, 1394–1403. doi: 10.1101/gr.2289704
Du, Z. J., Wang, Y., Dunlap, C., Rooney, A. P., and Chen, G. J. (2014). Draconibacterium orientale gen. nov., sp. nov., isolated from two distinct marine environments, and proposal of Draconibacteriaceae fam. nov. Int. J. Syst. Evol. Microbiol. 64, 1690–1696. doi: 10.1099/ijs.0.056812-0
Felsenstein, J. (1981). Evolutionary trees from DNA sequences: a maximum likelihood approach. J. Mol. Evol. 17, 368–376. doi: 10.1007/BF01734359
Fitch, W. M. (1971). Toward defining course of evolution - minimum change for a specific tree topology. Syst. Zool. 20, 406–416. doi: 10.2307/2412116
Fu, L. M., Niu, B. F., Zhu, Z. W., Wu, S. T., and Li, W. Z. (2012). CD-HIT: accelerated for clustering the next-generation sequencing data. Brief. Bioinformatics 28, 3150–3152. doi: 10.1093/bioinformatics/bts565
Goris, J., Konstantinidis, K. T., Klappenbach, J. A., Coenye, T., Vandamme, P., and Tiedje, J. M. (2007). DNA-DNA hybridization values and their relationship to whole-genome sequence similarities. Int. J. Syst. Evol. Microbiol. 57, 81–91. doi: 10.1099/ijs.0.64483-0
Holt, C. C., Van Der Giezen, M., Daniels, C. L., Stentiford, G. D., and Bass, D. (2019). Spatial and temporal axes impact ecology of the gut microbiome in juvenile European lobster (Homarus gammarus). ISME J. 14, 531–543. doi: 10.1038/s41396-019-0546-1
Komagata, K., and Suzuki, K. I. (1988). Lipid and cell-wall analysis in bacterial systematics. Methods Microbiol. 19, 161–207.
Kroppenstedt, R. M. (1982). Separation of bacterial menaquinones by HPLC using reverse phase (RP18) and a silver loaded ion exchanger as stationary phases. J. Liq. Chromatogr. 5, 2359–2367. doi: 10.1080/01483918208067640
Krüger, K., Chafee, M., Ben Francis, T., del Rio, T. G., Becher, D., Schweder, T., et al. (2019). In marine Bacteroidetes the bulk of glycan degradation during algae blooms is mediated by few clades using a restricted set of genes. ISME J. 13, 2800–2816. doi: 10.1038/s41396-019-0476-y
Kumar, S., Stecher, G., Tamura, K., Sudhir, K., and Stecher, K. (2016). MEGA7: molecular evolutionary genetics analysis version 7.0 for bigger datasets. Mol. Biol. Evol. 33, 1870–1874. doi: 10.1093/molbev/msw054
Lane, D. J. (1991). “16S/23S rRNA sequencing,” in Nucleic Acid Techniques in Bacterial Systematics, eds E. Stackebrandt and M. Goodfellow (New York, NY: Wiley), 115–175.
Lee, I., Kim, Y. O., Park, S. C., and Chun, J. (2016). OrthoANI: an improved algorithm and software for calculating average nucleotide identity. Int. J. Syst. Evol. Microbiol. 66, 1100–1103. doi: 10.1099/ijsem.0.000760
Lim, Y., Kang, I., and Cho, J. C. (2020). Genome characteristics of Kordia antarctica IMCC3317T and comparative genome analysis of the genus Kordia. Sci. Rep. 10:14715. doi: 10.1038/s41598-020-71328-9
Meier-Kolthoff, J. P., Auch, A. F., Klenk, H. P., and Göker, M. (2013). Genome sequence-based species delimitation with confidence intervals and improved distance functions. BMC Bioinformatics 14:60. doi: 10.1186/1471-2105-14-60
Minnikin, D. E., O’Donnell, A. G., Goodfellow, M., Alderson, G., Athalye, M., and Schaal, A. (1984). An integrated procedure for the extraction of bacterial isoprenoid quinones and polar lipids. J. Microbiol. Methods 2, 233–241. doi: 10.1016/0167-7012(84)90018-6
Montero-Calasanz, M. D., Goker, M., Rohde, M., Sproer, C., Schumann, P., Busse, H. J., et al. (2014). Chryseobacterium oleae sp nov., an efficient plant growth promoting bacterium in the rooting induction of olive tree (Olea europaea L.) cuttings and emended descriptions of the genus Chryseobacterium, C. daecheongense, C. gambrini, C. gleum, C. joostei, C. jejuense, C. luteum, C. shigense, C. taiwanense, C. ureilyticum and C. vrystaatense. Syst. Appl. Microbiol. 37, 342–350. doi: 10.1016/j.syapm.2014.04.004
Richter, M., and Rossello-Mora, R. (2009). Shifting the genomic gold standard for the prokaryotic species definition. Proc. Natl. Acad. of Sci. U.S.A. 106, 19126–19131. doi: 10.1073/pnas.0906412106
Saitou, N., and Nei, M. (1987). The neighbor-joining method: a new method for reconstructing phylogenetic trees. Mol. Biol. Evol. 4, 406–425. doi: 10.1093/oxfordjournals.molbev.a040454
Sasser, M. (1990). Identification of Bacteria by gas Chromatography of Cellular Fatty Acids, MIDI Technical Note 101. Newark, DE: MIDI Inc.
Smibert, R. M., and Krieg, N. R. (1994). “Phenotypic characterization,” in Methods for General and Molecular Bacteriology, eds P. Gerhardt, R. G. E. Murray, W. A. Wood, and N. R. Krieg (Washington, DC: American Society for Microbiology), 607–654.
Tatusova, T., DiCuccio, M., Badretdin, A., Chetvernin, V., Nawrocki, E. P., Zaslavsky, L., et al. (2016). NCBI prokaryotic genome annotation pipeline. Nucleic Acids Res. 44, 6614–6624. doi: 10.1093/nar/gkw569
Thompson, J. D., Gibson, T. J., Plewniak, F., Jeanmougin, F., and Higgins, D. G. (1997). The CLUSTAL_X windows interface: flexible strategies for multiple sequence alignment aided by quality analysis tools. Nucleic Acids Res. 25, 4876–4882. doi: 10.1093/nar/25.24.4876
Tindall, B. J., Sikorski, J., Smibert, R. M., and Krieg, N. R. (2007). “Phenotypic characterization and the principles of comparative systematics,” in Methods for General and Molecular Microbiology, 3rd Edn, eds C. A. Reddy, T. J. Beveridge, J. A. Breznak, G. Marzluf, T. M. Schmidt, and L. R. Snyder (Washington, DC: ASM Press), 330–393.
Wang, F. Q., Ren, L. H., Zou, R. J., Sun, Y. Z., Liu, X. J., Jiang, F., et al. (2018). Carboxylicivirga sediminis sp. nov., isolated from coastal sediment. Int. J. Syst. Evol. Microbiol. 68, 1896–1901. doi: 10.1099/ijsem.0.002761
Wang, F. Q., Zhou, Y. X., Lin, X. Z., Chen, G. J., and Du, Z. J. (2015). Carboxylicivirga linearis sp. nov., isolated from a sea cucumber culture pond. Int. J. Syst. Evol. Microbiol. 65, 3271–3275. doi: 10.1099/ijsem.0.000407
Wang, H., Qi, C., Chen, W., Dong, W., Tang, H., and Hu, X. K. (2016). Carboxylicivirga flava sp. nov., isolated from marine surface sediment. Int. J. Syst. Evol. Microbiol. 66, 5412–5416. doi: 10.1099/ijsem.0.001533
Wang, H. B., Li, H., Zhang, M. L., Song, Y. X., Huang, J., Huang, H., et al. (2018). Carbon dots enhance the nitrogen fixation activity of Azotobacter chroococcum. ACS Appl. Mater. Interfaces 10, 16308–16314. doi: 10.1021/acsami.8b03758
Wei, H. L., Wang, H., Tang, L., Mu, C. K., Ye, C. Y., Chen, L. Z., et al. (2019). High-throughput sequencing reveals the core gut microbiota of the mud crab (Scylla paramamosain) in different coastal regions of southern China. BMC Genomics 20:829. doi: 10.1186/s12864-019-6219-7
Yang, S. H., Table Seo, H. S., Woo, J. H., Oh, H. M., Jang, H., Lee, J. H., et al. (2014). Carboxylicivirga gen. nov. in the family Marinilabiliaceae with two novel species, Carboxylicivirga mesophila sp. nov. and Carboxylicivirga taeanensis sp. nov., and reclassification of Cytophaga fermentans as Saccharicrinis fermentans gen. nov., comb. nov. Int. J. Syst. Evol. Microbiol. 64, 1351–1358. doi: 10.1099/ijs.0.053462-0
Yoon, S. H., Ha, S. M., Kwon, S., Lim, J., Kim, Y., Seo, H., et al. (2017). Introducing EzBioCloud: a taxonomically united database of 16S rRNA gene sequences and whole-genome assemblies. Int. J. Syst. Evol. Microbiol. 67, 1613–1617. doi: 10.1099/ijsem.0.001755
Keywords: Carboxylicivirga, 16S rRNA gene, polyphasic taxonomy, draft genome sequencing, genome analysis
Citation: Zhang T-S, Zhang Y-N, Lu D-C, Gong Y and Du Z-J (2021) Carboxylicivirga marinus sp. nov., Isolated From Marine Sediment, and Genome Insight of the Genus Carboxylicivirga. Front. Mar. Sci. 8:731212. doi: 10.3389/fmars.2021.731212
Received: 26 June 2021; Accepted: 05 August 2021;
Published: 26 August 2021.
Edited by:
Martin W. Hahn, University of Innsbruck, AustriaReviewed by:
Ram Hari Dahal, Kyungpook National University, South KoreaAsif Hameed, National Chung Hsing University, Taiwan
Copyright © 2021 Zhang, Zhang, Lu, Gong and Du. This is an open-access article distributed under the terms of the Creative Commons Attribution License (CC BY). The use, distribution or reproduction in other forums is permitted, provided the original author(s) and the copyright owner(s) are credited and that the original publication in this journal is cited, in accordance with accepted academic practice. No use, distribution or reproduction is permitted which does not comply with these terms.
*Correspondence: Ya Gong, gongya@sdu.edu.cn; Zong-Jun Du, duzongjun@sdu.edu.cn