- 1Department of Chemistry, Nara Medical University, Kashihara, Japan
- 2Faculty of Pharmacy, Keio University, Tokyo, Japan
- 3Department of Pediatrics, Asahikawa Medical University, Asahikawa, Japan
Hemoglobin (Hb) is the most abundant protein in blood, with concentration of about 12–15 g/dl. The highly concentrated Hb solution (35 g/dl) is compartmentalized in red blood cells (RBCs). Once Hb is released from RBCs by hemolysis during blood circulation, it induces renal and cardiovascular toxicities. To date, hemoglobin-based oxygen carriers of various types have been developed as blood substitutes to mitigate the Hb toxicities. One method is Hb encapsulation in phospholipid vesicles (liposomes). Although the Hb toxicity can be shielded, it is equally important to ensure the biocompatibility of the liposomal membrane. We have developed Hb-vesicles (HbV). A new encapsulation method using a rotation-revolution mixer which enabled efficient production of HbV with a high yield has considerably facilitated R&D of HbV. Along with our academic consortium, we have studied the preclinical safety and efficacy of HbV extensively as a transfusion alternative, and finally conducted a phase I clinical trial. Moreover, carbonyl-HbV and met-HbV are developed respectively for an anti-inflammatory and anti-oxidative agent and an antidote for poisons. This review paper specifically presents past trials of liposome encapsulated Hb, biocompatible lipid bilayer membranes, and efficient HbV preparation methods, in addition to potential clinical applications of HbV based on results of our in vivo studies.
Introduction
Blood donation and transfusion are routinely practiced for sustaining human health and welfare. Their history informs us of the important endeavors of researchers and clinicians at establishing present modes of safer blood transfusion, and we have to continue our endeavors to challenge the remaining difficulties. Screening of the donated blood for hepatitis viruses B, C and E, HIV, west Nile virus etc. by nucleic acid amplification testing (NAT) has mostly eliminated transfusion-related infections. Nevertheless, emergent infectious viruses threaten humanity continuously. Concentrates of donated red blood cells (RBCs) can be stored in a refrigerator for 6 weeks in the US and EU, but for only 3 weeks in Japan. Rare blood-type RBCs are frozen with a cryoprotectant for long-term storage, but the cryoprotectant must be removed before infusion. Such limited storage conditions impose burdens on logistics, especially for remote islands and rural areas and stockpiling for emergency needs. Crossmatching and blood-type testing immediately before urgent transfusion are particularly time-consuming for life-saving practices. Even in economically developed countries, clinicians experience urgent situations in which blood transfusion is not available to treat patients. These difficulties have spurred us to develop artificial red cells that can eventually be substituted for RBC transfusion where blood transfusion is not available (1). Worldwide deaths from hemorrhage are estimated as 1.9 million per year, with 1.5 million resulting from physical trauma (2).
Hemoglobin (Mw. 64,500) is the most abundant protein in blood. About two million Hb molecules are compartmentalized in a single red blood cell (RBC). The intracellular Hb concentration, which is as high as 35 g/dl, makes the Hb concentration of blood as high as 12–15 g/dl. Because blood type antigens are present on the outer surface of RBCs, an early idea was to use purified Hb as an oxygen-carrying fluid that is free of any blood type. Nevertheless, that effort was unsuccessful because of various toxic effects. In spite of its abundance in blood, Hb becomes toxic when it is released from RBCs. Dissociation of tetramer Hb subunits into two dimers occurs, which proceeds to induce renal toxicity. Entrapment of a gaseous messenger molecule, NO, induces vasoconstriction, hypertension, neurological disturbances, and malfunction of esophageal motor functions (3–6). An aqueous solution of chemically modified Hb-based oxygen carriers (HBOCs) exhibits a colloid osmotic pressure that sometimes exceeds the physiological value (20–25 mmHg) in spite of its low Hb content, thereby having a potential to cause volume overload (7). Some first generation chemically modified HBOCs presented side effects in clinical trials: higher risks of myocardial infarction and death (8, 9). These side effects of molecular Hb imply the importance of the cellular structure and larger particle size of HBOCs (10).
Encapsulation of hemoglobin into liposomes to mimic red blood cells
Concept of hemoglobin encapsulation in liposomes
Pioneering Hb microencapsulation work was first performed by Chang in 1957 (11) using a polymer membrane. Japanese groups followed his attempt to test Hb encapsulation with gelatin, gum Arabic, silicone, etc. (12). Nevertheless, regulating the particle size to be appropriate for blood flow in the capillaries and obtaining sufficient biocompatibility were extremely difficult. Bangham and Horne reported in 1964 that phospholipids assemble to form vesicles in water (13), suggesting that the vesicles (liposomes) can encapsulate water-soluble functional materials in their inner phase (14). The lipid membrane of liposomes somewhat resembles a biomembrane. It should be more biocompatible than a synthetic polymer membrane. Djordjevici and Miller in 1977 first reported a liposome-encapsulated Hb (LEH) as an artificial oxygen carrier (15). Following their trial, many laboratories tested Hb encapsulation using liposomes with various lipid compositions and preparation methods (Table 1) (16–44). The addition of cholesterol to phospholipid is a standard method of stabilizing the packing of the lipid membrane and of reducing its curvature to produce larger liposomes. Inclusion of a negatively charged lipid is also a standard recipe to provide negative charges on the liposomal surface, which is effective to reduce the lamellarity of liposomes and to increase the volume of inner aqueous phase, thereby leading to higher Hb encapsulation efficiency (33, 45). Exceptionally, the trials of entry Nos. 3, 5, 16, 21, and 22 did not use negatively charged lipids (18, 20, 42, 43). It is noteworthy that not only liposomes but also polymersomes and other submicrometer capsules made of synthetic biodegradable polymers are tested extensively for Hb encapsulation (46–48). Detailed results of their safety and efficacy studies are awaited.
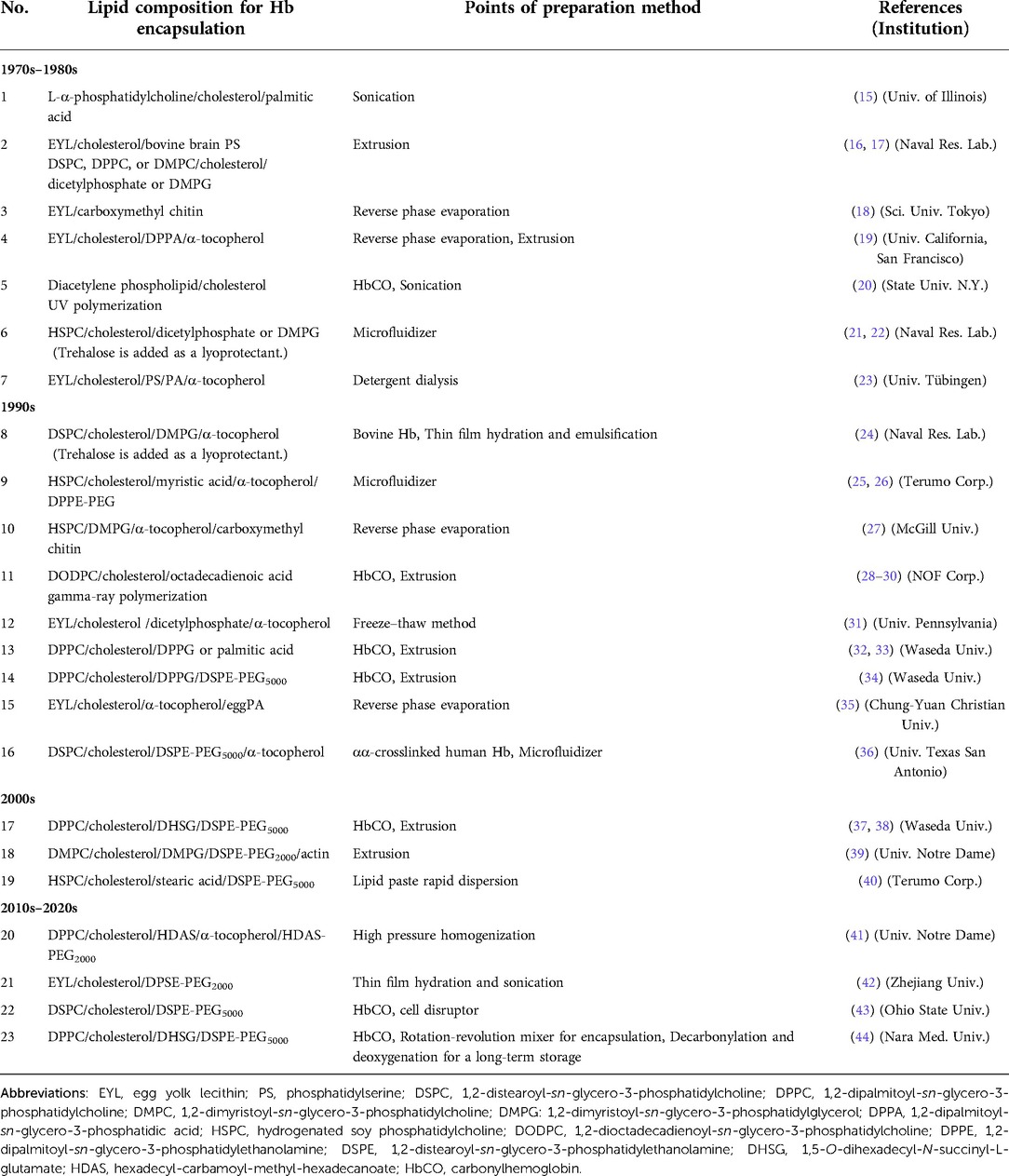
Table 1. Trials of liposome encapsulated Hb with various lipid compositions and preparation methods.
Optimal lipid compositions for stability and safety of liposome encapsulated hemoglobin
Liposome is categorized as a molecular assembly of lipids formed through hydrophobic interaction among lipids. It is generally regarded as an unstable and fragile capsule, especially when using unsaturated phospholipids such as egg yolk lecithin. To stabilize LEH for long-term storage, polymerizable phospholipids that included diacetylene or diene groups in the phospholipid molecules (entry Nos. 5 & 11) were once tested (5, 28–30). After Hb encapsulation, the lipid was polymerized by UV irradiation or gamma-ray irradiation. In the case of diene-containing phospholipid, the obtained gamma-ray irradiated LEH was so stable that it was able to be frozen and thawed or freeze-dried and rehydrated without structural damage (49). However, one difficulty was clarified from animal experiments: the polymerized liposome could not be metabolized in the reticuloendothelial system. It remained in the liver and spleen for a long time (30). Other trials include surface coverage with polymer chains, such as carboxymethyl chitin (entry No. 3) and actin (entry No. 18) (18, 39). Rudolph et al. (23) tested freeze-drying and rehydration procedures of LEH in the presence of trehalose as a cryoprotectant as well as a lyoprotectant (entry No. 7). Since Yoshioka et al. reported PEGylation of LEH in 1989, it has become a standard method to stabilize the dispersion state during storage and during blood circulation (Entry No. 9) (50). In our research of so-called Hb-vesicles (HbV), we confirmed that deoxygenated HbV can be stored for over two years at room temperature using a saturated phospholipid, DPPC, to avoid lipid peroxidation and the combination of PEGylation (44) (Figure 1). PEGylation of liposomes is generally intended to prolong their circulation half-lives by the stealth effect (51). An additional benefit of PEGylation is to improve the dispersion state of liposomes during storage and in blood plasma or in plasma expanders (52, 53).
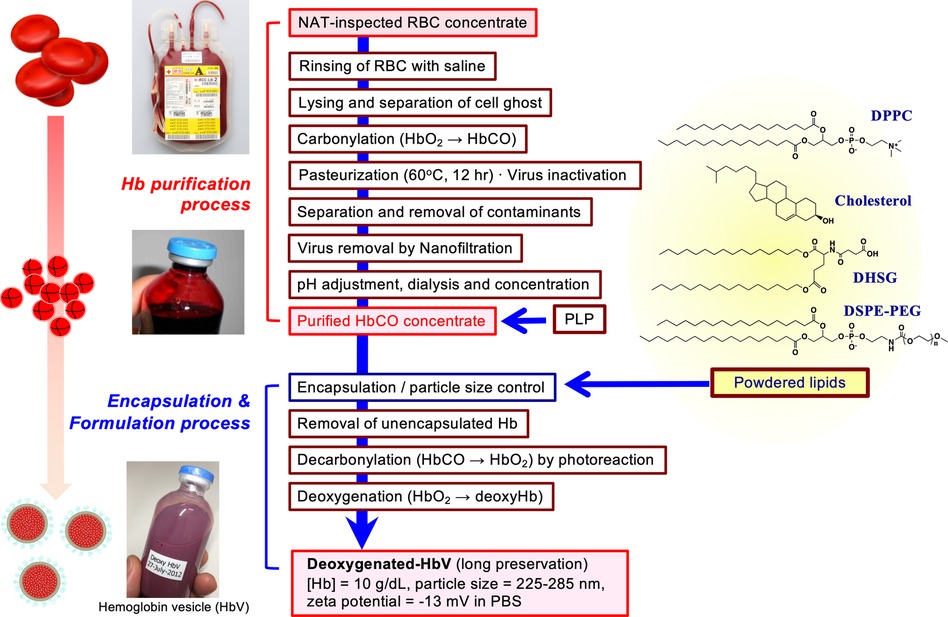
Figure 1. Outline of production scheme of HbV. HbCO solution was purified from nucleic acid amplification test (NAT)-inspected human red blood cells concentrate obtained from Japanese Red Cross Kinki Block Blood Center. Cells were rinsed with saline and lysed by gentle osmotic shock. The erythrocyte ghost was separated by ultrafiltration. After carbonylation, the resulting HbCO was pasteurized at 60 °C for 12 h, nano-filtrated, dialyzed, passed through anion-exchanging resins, and ultrafiltered to concentrate to 40 g/dl. Pyridoxal 5′-phosphate (PLP) was added to HbCO at the molar ratio of 1.0. The powdered lipids comprised of 1,2-dipalmitoyl-sn-glycero-3-phosphatidylcholine (DPPC), cholesterol, 1,5-O-dihexadecyl-N-succinyl-L-glutamate (DHSG), and 1,2-distearoyl-sn-glycero-3-phosphatidylethanolamine-N-poly(ethylene glycol) (PEG5000, DSPE-PEG) at a molar ratio of 5:4:0.9:0.03. The HbCO encapsulation and particle size control were performed using the kneading method with a rotation–revolution mixer (44). The unencapsulated Hb was removed by ultrafiltration; HbCO in the vesicles suspended in saline solution was converted to oxyhemoglobin by photoillumination and oxygen flow. The suspension was deoxygenated completely, purged in plastic bags, and sealed with an oxygen adsorber in aluminum bags for long-term storage at 2–8 °C.
The toxicities of Hb can be shielded by liposomal encapsulation to mimic the physiological structure of erythrocytes. However, another important point is whether the liposomal lipid membrane is sufficiently biocompatible. The surface property of liposome is an important factor to assess safety. Reducing interactions with negatively charged plasma proteins and vascular endothelial cells is important. As shown in Table 1, many laboratories use negatively charged phospholipid components such as phosphatidic acid (PA) (19), phosphatidyl serine (PS) (16, 17), phosphatidyl glycerol (PG), and fatty acids (32–34). Later, it was clarified that such negatively charged lipids induce complement activation or platelet activation (40, 54–58). In our case, we use DHSG as a negatively charged synthetic lipid for HbV, which does not induce complement activation or platelet activation in preclinical animal experiments (37, 38, 44, 56–58) (Figure 1). Numerous selections of lipid species and compositions are present to make liposomes. Fortunately, the present lipid compositions of HbV: DPPC/cholesterol/DHSG/DSPE-PEG5000 show sufficient stability and biocompatibility.
Preparation methods of liposome encapsulated hemoglobin
Because liposomal drugs for cancer and antifungal therapies are approved and because liposomes are used experimentally as a model of biomembrane, various preparation methods have been reported (14). Traditional methods include (i) sonication, (ii) reverse phase-evaporation method using an organic solvent such as diethyl ether, ethanol, or tertial butanol, and (iii) solubilization with detergent following dialysis. However, these methods would not be appropriate for Hb encapsulation for in vivo use because of the possibility of denaturation of Hb as a protein and incomplete removal of an organic solvent or a detergent. Another method is (iv) extrusion (32, 38). One parameter of LEH to express the oxygen-carrying capacity is the weight ratio of Hb to total lipid: Hb/Lipid. This parameter takes in increasingly larger value with encapsulation of larger amounts of Hb in liposomes with a smaller amount of lipids. Accordingly, the concentration of Hb for encapsulation is expected to be as high as 35–45 g/dl, which is similar to the corpuscular Hb concentration in erythrocytes (35 g/dl). Such a concentrated Hb solution becomes exponentially viscous (50 cP or more) (44, 59). For encapsulation, it must be mixed with lipids (thin lipid films prepared on the inner surface of flask, or freeze-dried lipid powder) (38). The resulting highly viscous mixture is extruded through membrane filters to regulate the particle size. Here the difficulty is clogging of the filter because of the large amount of Hb and lipids. To prevent filter clogging, the amount of lipids had to be reduced to about 6 g/dl, resulting in insufficient encapsulation efficiency to only about 20%. (v) Microfluidizer was often used to reduce the liposome size by a head-on collision of fast fluid flows (21). However, the fluids require lower viscosity for the fast flow and the amount of lipid to be added to Hb is limited, resulting in lower encapsulation efficiency.
To overcome such difficulties in preparation, we developed (vi) a new kneading method with a rotation-revolution mixer (44). The Hb encapsulation efficiency was increased dramatically to about 70%, which is nearly the closest packing factor of 74%, because the kneading method enabled mixing of a highly concentrated carbonylhemoglobin (HbCO) solution (40 g/dl) and a considerably large amount of powdered lipids in only 10–20 min. The high viscosity of the Hb-lipid mixture paste (ca. 103–105 cP) favorably induces frictional heat by kneading and increases the paste temperature (ca. 60 °C), which facilitates lipid dispersion and liposome formation. During the kneading operation using a thermostable HbCO solution, Hb denaturation was prevented. After HbCO in HbV is converted to HbO2 by photolysis, HbO2 is converted to deoxyHb for the long-term storage of HbV. The kneading method is apparently the most suitable for the preparation of HbV made of a viscous paste of the Hb and lipids. Actually, it has enabled scaling up of HbV production and has facilitated preclinical and clinical studies in our project (60).
Safety studies of hemoglobin vesicles
The volumes of blood donation are 200 or 400 ml in Japan. It means that a hemorrhage of 400 ml blood loss does not affect physiological performance to any considerable degree. Therefore, a situation in which a blood substitute is necessary is estimated at a massive hemorrhage of 1,000 ml or more. The Hb concentration of blood is 12–15 g/dl. Blood is a concentrated RBC dispersion suspended in an aqueous solution of plasma proteins and other various solutes. Blood has physiologically adjusted viscosity, and crystalloid and colloid osmotic pressures. A blood substitute should also possess a compatible oxygen-carrying capacity and Hb concentration. In the case of HbV, the Hb concentration is adjusted to 10 g/dl, which is slightly higher than the transfusion trigger: 6–7 g/dl. Not RBCs but plasma proteins show colloid osmotic pressure of whole blood. The HbV is suspended in a physiological saline solution and the suspension does not possess colloid osmotic pressure. Therefore, HbV infusion requires co-injection or addition of plasma expanders such as human serum albumin, hydroxyethyl starch, modified fluid gelatin, or dextran depending on the situation, to adjust the colloid osmotic pressure, as does RBC transfusion (53, 61). Unlike conventional liposomal drugs used for cancer therapy, the volume of HbV for injection should be large: 16 ml/kg or more. Because of the necessity for massive infusion for HbV with high Hb and lipid concentrations, its safety in preclinical stages has been scrutinized in terms of blood compatibility, immunological (62), hematological (57), and cardiovascular effects, vital organ function, biodistribution (63, 64), excretion, etc. No hemolysis of HbV occurs during blood circulation. However, HbV is finally phagocytosed by macrophages, especially in the spleen and liver (65), which causes the main side effect of transient hepatosplenomegaly in rodent models, although the HbV in macrophage phagosomes disappears completely in two weeks; it is excreted through urine and feces (64, 66). Hemosiderin deposition, which is often observed in patients receiving repeated blood transfusion, was confirmed after repeated infusion of HbV in rats (65). All the relevant toxicological data have been reported and summarized elsewhere (67). One important physicochemical characteristic of HbV is that the HbV particles do not form any sediment after conventional centrifugation and show interference effect of “hemolysis” and “lipidemia” on blood clinical chemistry, but they do in the presence of a high molecular weight dextran. This feature enables easy separation of HbV from blood plasma specimen and contributes to accurate analyses in blood clinical chemistry to examine organ function (52).
Potential clinical applications of hemoglobin vesicles
Hemoglobin vesicles as an oxygen carrier
The efficacy of storable and ready-to-use HbV as a transfusion alternative has been tested by many animal models in our academic consortium, as presented in Table 2 (34, 53, 68–116). The ultimate purpose for R&D of HbV is to use the HbV fluid as a transfusion alternative, especially in urgent situations when RBC transfusion is not available. In fact, HbV has demonstrated effectiveness as a resuscitative fluid in animal models of hemorrhagic shock (72–80), peri-operational or injured uncontrolled massive hemorrhage (81, 84–86), and obstetric hemorrhage (82, 83). Under such circumstances, the level of blood exchange should exceed 50%. Co-injection of a plasma expander is necessary for such extreme conditions to maintain colloid osmotic pressure and the resulting blood volume. Hagisawa et al. clarified that intraosseous infusion of HbV is possible because of its smaller particle size than RBC. It easily enters osseous blood vessels to whole body blood circulation (79). This feature is particularly beneficial when peripheral blood vessels are collapsed and inaccessible. Moreover, HbV can replace packed RBCs as a priming solution for an extracorporeal membrane oxygenator during cardiovascular surgery. As an oxygen carrier, HbV can also be used for oxygen therapeutics for local ischemic diseases, brain protection at apnea, oxygenation of tumors for sensitization (94), and as an 15O carrier for positron emission tomography (95, 96), a perfusate for transplant organs (97–100), and an oxygen carrying medium for cell culturing (101). As a target dye, HbV is useful for dye laser irradiation therapy of port-wine stains (102, 103).
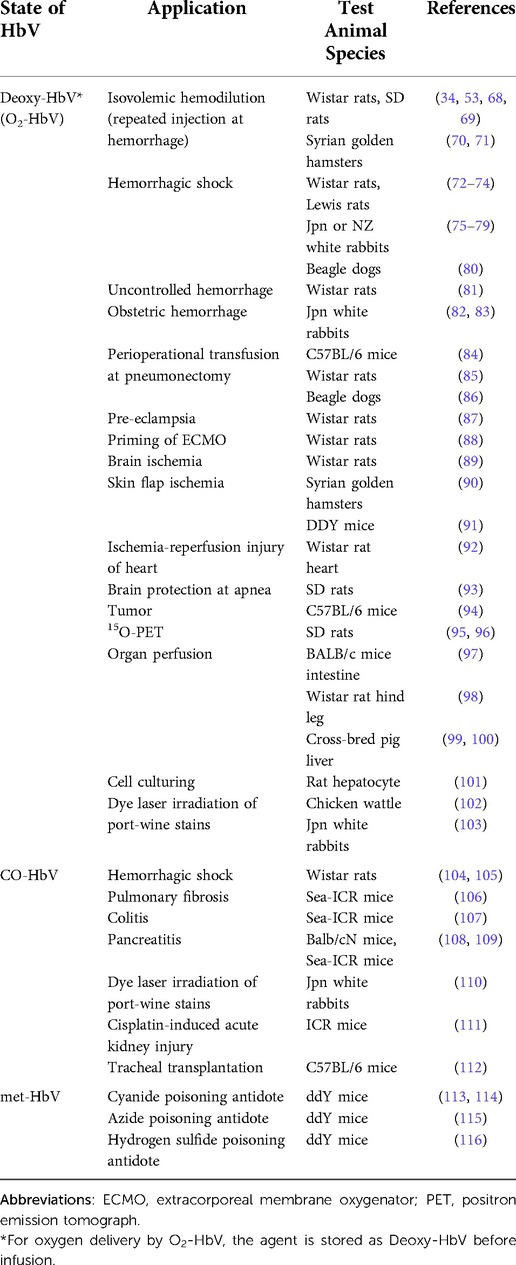
Table 2. Potential clinical application of HbV, not only as an O2-carrier, but also a CO carrier and an antidote, evidenced by academic consortium.
Hemoglobin vesicles as a carbon monoxide carrier
Carbon monoxide has been tested as an anti-oxidative and anti-inflammatory agent. Direct inhalation of diluted CO gas has been reported, as have the intravenous infusion of CO-bound RBCs and various CO-releasing nanocarriers and molecules (117, 118). Studies have confirmed that CO-bound HbV (CO-HbV) is effective for resuscitation from hemorrhagic shock mitigating ischemia reperfusion injury (104, 105). Moreover, Nagao and Taguchi et al. (106–109) have clarified CO-HbV as a therapeutic agent for colitis, pancreatitis, and cisplatin-induced acute kidney injury (Table 2). After releasing CO, HbV becomes an O2 carrier (Figure 2). For all experiments, the target molecule of CO should be enzymatic heme proteins related to generation of reactive oxygen species (ROS), such as NADPH oxidase, and cytochrome C oxidase. CO should prevent the activities of such ROS-generating enzymes. CO is well known as a poisonous gas but here, poisoning of ROS is treated by another poison: CO. It is noteworthy that histopathological studies of rat brain have clarified that CO-HbV administration did not lead to marked hippocampal damage (105). Up to 32 ml/kg dosage of CO-HbV did not induce any behavioral abnormalities and the effect of CO-HbV on the central nervous system seems minimal (119).
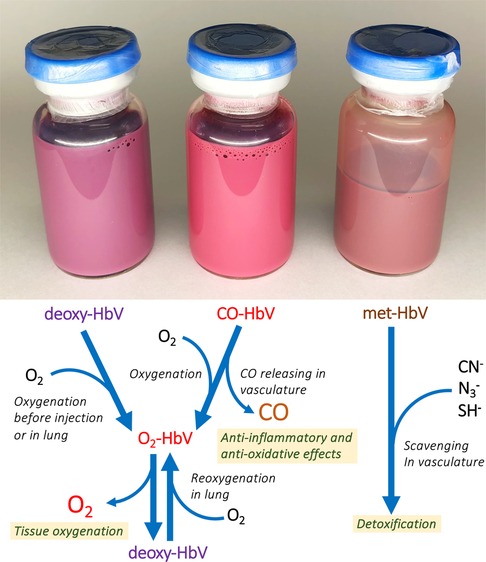
Figure 2. Glass vials containing deoxy-HbV, CO-HbV and met-HbV. The colors differ depending on the state of encapsulated Hb. Deoxy-HbV is for an O2-carrier, CO-HbV for a CO carrier, and metHbV for an antidote of poisonous materials. They can all be stored for years at room temperature.
Hemoglobin vesicles as an antidote
HbO2 in HbV gradually autoxidizes and becomes metHb, thereby losing its oxygen-carrying capacity. Stoichiometric addition of sodium nitrate to HbO2 spontaneously converts it to metHb. Suzuki and Taguchi et al. (113–116) clarified that vesicles containing metHb (metHbV) are useful as an antidote for poisons such as cyanide, azide, and hydrogen sulfide, all of which bind strongly to metHb (Table 2, Figure 2). They confirmed the efficacies of metHbV as antidotes using rodent models. For example, the efficacy as antidotes for cyanide poisoning is higher than conventional treatments with hydroxocobalamin and nitrous acid compounds. Although such an agent would not be required frequently in normal times, it is expected to be indispensable for emergency medicine in some accident-related or disaster-affected situations.
Phase 1 study of hemoglobin vesicles as a transfusion alternative
Many laboratories have attempted Hb encapsulation using liposomes, as shown in Table 1. Because of difficulty in ascertaining and developing optimal lipid compositions and efficient production methods, most groups eventually terminated their development. We have continued R&D of HbV and have confirmed their safety and efficacy through abundant preclinical studies. Our new kneading method to encapsulate Hb has facilitated their large-scale production and has supported our R&D projects overall. After full consultation with the Pharmaceuticals and Medical Devices Agency (PMDA), items of evaluations using rodents and dogs under GLP guideline were set in 2016. Subsequently, GLP non-clinical safety evaluations were conducted by private contracting laboratories and were completed in 2019 (60). From 2020, we started a small-scale GMP production of HbV at the Nara Medical University Cell Processing Center. Our academic consortium initiated a phase 1 (first-in-human) study of HbV in 2020 (Registration number, jRCT2011200004) (120) with the close assistance of clinical research organizations of Asahikawa Medical University Hospital Clinical Research Support Center and Hokkaido University Hospital Clinical Research and Medical Innovation Center.
The study examined three cohorts #1, #2, and #3 (n = 4), respectively, of 10, 50, and 100 ml dosage. Subjective symptoms, vital signs, electrocardiography, and hematological and biochemical parameters were observed. No severe adverse event was observed for any subject. No hypertension was observed. Some infusion reactions such as fever, fatigue, and conjunctivitis were observed in cohorts #1 and #2 (120), but they resolved promptly without medication. Premedication (dexamethasone, acetaminophen, and famotidine) was introduced for the safety of subjects of cohort #3. No adverse event was observed in the first two subjects. The third subject showed local rash with wheal, which resolved soon without medication after cessation of infusion. Because the coronavirus pandemic affected project rescheduling and because the investigational agent expired, cohort #3 was terminated. No problematic clinical sign was observed from any hematological or biochemical analyte, vital sign, electrocardiograph, etc. Blood specimens of cohort #3 provided concentration profiles of HbV in plasma and revealed that HbV circulate in the bloodstream with half-life of approximately 8 h. The circulation half-life of HbV is known dose-dependent, and it will become longer at a practically higher dosage. Based on the results, we are planning the next clinical trial starting from a 100 ml dose. For that purpose, additional GLP preclinical studies, including developmental toxicity studies, are underway in the ongoing project.
Conclusion
Since the first report of the liposome encapsulated Hb, 45 years have passed. After numerous trials of various lipid compositions and preparation methods, we have ascertained the optimal lipid composition and GMP production method of HbV, and have completed its GLP preclinical toxicological studies and the phase 1 clinical study. Based on the results, we are planning the next clinical trial starting from a 100 ml dose. As shown in Table 2, HbV is useful not only as a transfusion alternative and as an O2 carrier, but also as a CO carrier and an antidote for poisoning, which were not expected at the beginning of R&D of HBOCs. Such vast availability to various clinical situations is expected to enhance the contributions of HbV to future human health and welfare. Both CO-HbV and met-HbV are the derivatives of O2-HbV but different agents. Their preclinical safety and efficacy studies are the target of our research before starting clinical studies. Other HBOCs are expected to have some potential for use in the same manner.
Author contributions
All authors contributed to the article and approved the submitted version.
Funding
This research was supported partly by a Grant-in-Aid for Scientific Research from the Japan Society for the Promotion of Sciences (21H03824), Health and Labour Science Grants (Health Science Research Including Drug Innovation) from the Ministry of Health, Labour and Welfare, Japan (H24-Soyakusougou-Ippan009), and the Japan Agency for Medical Research and Development (AMED, project numbers JP15lk0201034, JP18lk1403022, JP21ym0126034).
Acknowledgments
The authors acknowledge all collaborators in the academic research consortium who contributed considerably to the production and the preclinical and the clinical studies of HbV.
Conflict of interest
HS and KT hold patents related to the production and utilization of hemoglobin vesicles. The remaining authors declare that the research was conducted in the absence of any commercial or financial relationships that could be construed as a potential conflict of interest.
Publisher's note
All claims expressed in this article are solely those of the authors and do not necessarily represent those of their affiliated organizations, or those of the publisher, the editors and the reviewers. Any product that may be evaluated in this article, or claim that may be made by its manufacturer, is not guaranteed or endorsed by the publisher.
References
1. Chang TMS, Bulow L, Jahr J, Sakai H, Yang C. Nanobiotherapeutic based blood substitutes. Singapore: World Scientific Publishing Co. Pte. Ltd (2022).
3. Doherty DH, Doyle MP, Curry SR, Vali RJ, Fattor TJ, Olson JS, et al. Rate of reaction with nitric oxide determines the hypertensive effect of cell-free hemoglobin. Nat Biotechnol. (1998) 16(7):672–6. doi: 10.1038/nbt0798-672
4. Murray JA, Ledlow A, Launspach J, Evans D, Loveday M, Conklin JL. The effects of recombinant human hemoglobin on esophageal motor function in humans. Gastroenterology. (1995) 109:1241–8. doi: 10.1016/0016-5085(95)90584-7
5. Sakai H, Hara H, Yuasa M, Tsai AG, Takeoka S, Tsuchida E, et al. Molecular dimensions of hb-based O2 carriers determine constriction of resistance arteries and hypertension. Am J Physiol Heart Circ Physiol. (2000) 279:H908–15. doi: 10.1152/ajpheart.2000.279.3.H908
6. Sakai H, Sato A, Masuda K, Takeoka S, Tsuchida E. Encapsulation of concentrated hemoglobin solution in phospholipid vesicles retards the reaction with NO, but not CO, by intracellular diffusion barrier. J Biol Chem. (2008) 283:1508–17. doi: 10.1074/jbc.M707660200
7. Sakai H, Yuasa M, Onuma H, Takeoka S, Tsuchida E. Synthesis and physicochemical characterization of a series of hemoglobin-based oxygen carriers: objective comparison between cellular and acellular types. Bioconjugate Chem. (2000) 11:56–64. doi: 10.1021/bc9900789
8. Natanson C, Kern S, Lurie P, Banks SM, Wolfe SM. Cell-free hemoglobin-based blood substitutes and risk of myocardial infarction and death: a meta-analysis. JAMA. (2008) 299:2304–12. doi: 10.1001/jama.299.19.jrv80007
9. Burhop K, Gordon D, Estep T. Review of hemoglobin-induced myocardial lesions. Artif Cells Blood Subst Biotechnol. (2004) 32(3):353–74. doi: 10.1081/BIO-200027429
10. Matsuhira T, Sakai H. Artificial oxygen carriers, from nano- to micron-sized particles of hemoglobin composites substituting for red blood cells. Particuology. (2022) 64:43–55. doi: 10.1016/j.partic.2021.08.010
12. Kimoto S, Hori M, Toyoda T, Sekiguchi W. Artificial red cells. Gekachiryou. (1968) 19(3):324–32 (in Japanese).
13. Bangham AD, Horne RW. Negative staining of phospholipids and their structure modification by surface-active agents as observed in the electron microscope. J Mol Biol. (1964) 8:660–8. doi: 10.1016/S0022-2836(64)80115-7
14. Pattni BS, Chupin VV, Torchilin VP. New developments in liposomal drug delivery. Chem Rev. (2015) 115(19):10938–66. doi: 10.1021/acs.chemrev.5b00046
15. Djordjevich L, Miller IF. Lipid encapsulated hemoglobin as a synthetic erythrocyte. Fed Proc. (1977) 36:567.
16. Gaber BP, Yager P, Sheridan JP, Chang EL. Encapsulation of hemoglobin in phospholipid vesicles. FEBS Lett. (1983) 153:285–8. doi: 10.1016/0014-5793(83)80625-5
17. Farmer MC, Gaber BP. Liposome-encapsulated hemoglobin as an artificial oxygen carrying system. Methods Enzymol. (1987) 149:184–200. doi: 10.1016/0076-6879(87)49056-3
18. Kato A, Arakawa M, Kondo T. Liposome-type artificial red blood cells stabilized with carboxymethylchitin. Nippon Kagaku Kaishi. (1984) 6:987–91 (in Japanese). doi: 10.1246/nikkashi.1984.987
19. Hunt CA, Burnette RR, MacGregor RD, Strubbe AE, Lau DT, Taylor N, et al. Synthesis and evaluation of prototypal artificial red cells. Science. (1985) 230:1165–8. doi: 10.1126/science.4071041
20. Hayward JA, Levine DM, Neufeld L, Simon SR, Johnston DS, Chapman D. Polymerized liposomes as stable oxygen-carriers. FEBS Lett. (1985) 187:261–6. doi: 10.1016/0014-5793(85)81255-2
21. Beissinger RL, Farmer MC, Gossage JL. Liposome-encapsulated hemoglobin as a red cell surrogate. ASAIO Trans. (1986) 32:58–63.3778774
22. Rudolph AS. The freeze-dried preservation of liposome encapsulated hemoglobin: a potential blood substitute. Cryobiology. (1988) 25:277–84. doi: 10.1016/0011-2240(88)90036-3
23. Jopski B, Pirkl V, Jaroni H-W, Schubert R, Schmidt K-H. Preparation of hemoglobin-containing liposomes using octyl glucoside and octyltetraoxyethylene. Biochim Biophys Acta. (1989) 978:79–84. doi: 10.1016/0005-2736(89)90501-4
24. Rabinovici R, Rudolph AS, Vernick J, Feuerstein G. A new salutary resuscitative fluid: liposome encapsulated hemoglobin/hypertonic saline solution. J Trauma. (1993) 35:121–7. doi: 10.1097/00005373-199307000-00019
25. Yoshioka H. Surface modification of haemoglobin-containing liposomes with poly(ethylene glycol) prevents liposome aggregation in blood plasma. Biomaterials. (1991) 12:861–4. doi: 10.1016/0142-9612(91)90075-L
26. Takahashi A. Characterization of neo red cells (NRCs), their function and safety in vivo tests. Artif Cells Blood Substitutes Immobil Biotechnol. (1995) 23:347–54. doi: 10.3109/10731199509117951
27. Mobed M, Chang TMS. Preparation and surface characterization of carboxymethylchitin-incorporated submicron bilayer-lipid membrane artificial cells (liposomes) encapsulating hemoglobin. Biomater Artif Cells Immobilization Biotechnol. (1991) 19:731–44. doi: 10.3109/10731199109117851
28. Sato T, Kobayashi K, Sekiguchi S, Tsuchida E. Characteristics of artificial red cells: hemoglobin-encapsulated in poly-lipid vesicles. ASAIO J. (1992) 38:M580–4. doi: 10.1097/00002480-199207000-00102
29. Sakai H, Takeoka S, Yokohama H, Nishide H, Tsuchida E. Encapsulation of Hb into unsaturated lipid vesicles and gamma-ray polymerization. Polymer Adv Technol. (1992) 3:389–94. doi: 10.1002/pat.1992.220030704
30. Akama K, Awai K, Yano Y, Tokuyama S, Nakano Y. In vitro and in vivo stability of polymerized mixed liposomes composed of 2,4-octadecadienoyl groups of phospholipids. Polymer Adv Technol. (2000) 11:280–7. doi: 10.1002/1099-1581(200006)11:6%3C280::AID-PAT942%3E3.0.CO;2-C
31. Liu L, Yonetani T. Preparation and characterization of liposome-encapsulated haemoglobin by a freeze–thaw method. J Microencapsulation. (1994) 11:409–21. doi: 10.3109/02652049409034258
32. Sakai H, Hamada K, Takeoka S, Nishide H, Tsuchida E. Physical properties of hemoglobin vesicles as red cell substitutes. Biotechnol Progr. (1996) 12:119–25. doi: 10.1021/bp950068w
33. Takeoka S, Ohgushi T, Terase K, Tsuchida E. Layer-controlled hemoglobin vesicles by interaction of hemoglobin with a phospholipid assembly. Langmuir. (1996) 12:1755–9. doi: 10.1021/la940936j
34. Sakai H, Takeoka S, Park SI, Kose T, Izumi Y, Yoshizu A, et al. Surface-modification of hemoglobin vesicles with poly(ethylene glycol) and effects on aggregation, viscosity, and blood flow during 90%-exchange transfusion in anesthetized rats. Bioconjugate Chem. (1997) 8:23–30. doi: 10.1021/bc960069p
35. Chung TW, Peng IH. An engineering model to characterize oxygen transfer rates for liposome encapsulated hemoglobin (LEH). Artif Cells Blood Substit Immobil Biotechnol. (1998) 26(4):389–98. doi: 10.3109/10731199809117680
36. Phillips WT, Klipper RW, Awasthi VD, Rudolph AS, Cliff R, Kwasiborski V, et al. Polyethylene glycol-modified liposome-encapsulated hemoglobin: a long circulating red cell substitute. J Pharmacol Exp Ther. (1999) 288:665–70. https://jpet.aspetjournals.org/content/288/2/665.long9918573
37. Sakai H, Takeoka S, Wettstein R, Tsai AG, Intaglietta M, Tsuchida E. Systemic and microvascular responses to the hemorrhagic shock and resuscitation with hb-vesicles. Am J Physiol Heart Circ Physiol. (2002) 283:H1191–9. doi: 10.1152/ajpheart.00080.2002
38. Sou K, Naito Y, Endo T, Takeoka S, Tsuchida E. Effective encapsulation of proteins into size-controlled phospholipid vesicles using freeze-thawing and extrusion. Biotechnol Progr. (2003) 19:1547–52. doi: 10.1021/bp0201004
39. Li S, Nickels J, Palmer AF. Liposome-encapsulated actin-hemoglobin (LEAcHb) artificial blood substitutes. Biomaterials. (2005) 26:3759–69. doi: 10.1016/j.biomaterials.2004.09.015
40. Pape A, Kertscho H, Meier J, Horn O, Laout M, Steche M, et al. Improved short-term survival with polyethylene glycol modified hemoglobin liposomes in critical normovolemic anemia. Intensive Care Med. (2008) 34:1534–43. doi: 10.1007/s00134-008-1082-z
41. Yadav VR, Nag O, Awasthi V. Biological evaluation of liposome-encapsulated hemoglobin surface-modified with a novel PEGylated nonphospholipid amphiphile. Artif Organs. (2014) 38(8):625–33. doi: 10.1111/aor.12304
42. Li W, Yang J, Luo L, Jiang M, Qin B, Yin H, et al. Targeting photodynamic and photothermal therapy to the endoplasmic reticulum enhances immunogenic cancer cell death. Nat Commun. (2019) 10(1):3349. doi: 10.1038/s41467-019-11269-8
43. Banerjee U, Wolfe S, O'Boyle Q, Cuddington C, Palmer AF. Scalable production and complete biophysical characterization of poly(ethylene glycol) surface conjugated liposome encapsulated hemoglobin (PEG-LEH). PLoS ONE. (2022) 17(7):e0269939. doi: 10.1371/journal.pone.0269939
44. Kure T, Sakai H. Preparation of artificial red blood cells (hemoglobin vesicles) using the rotation-revolution mixer for high encapsulation efficiency. ACS Biomater Sci Eng. (2021) 7(6):2835–44. doi: 10.1021/acsbiomaterials.1c00424
45. Sou K. Electrostatics of carboxylated anionic vesicles for improving entrapment capacity. Chem Phys Lipids. (2011) 164(3):211–5. doi: 10.1016/j.chemphyslip.2011.01.002
46. Rameez S, Bamba I, Palmer AF. Large scale production of vesicles by hollow fiber extrusion: a novel method for generating polymersome encapsulated hemoglobin dispersions. Langmuir. (2010) 26(7):5279–85. doi: 10.1021/la9036343
47. Coll-Satue C, Jansman MMT, Thulstrup PW, Hosta-Rigau L. Optimization of hemoglobin encapsulation within PLGA nanoparticles and their investigation as potential oxygen carriers. Pharmaceutics. (2021) 13(11):1958. doi: 10.3390/pharmaceutics13111958
48. Mittal N, Rogers S, Dougherty S, Wang Q, Moitra P, Brummet M, et al. Erythromer (EM), a nanoscale bio-synthetic artificial red cell. In: Liu H, Kaue AD, Jahr JS, editors. Blood substitutes and oxygen biotherapeutics. Cham, Switzerland: Springer Nature Switzerland AG (2022). p. 253–65.
49. Wang L, Takeoka S, Tsuchida E, Tokuyama S, Mashiko T, Satoh T. Preparation of dehydrated powder of hemoglobin vesicles. Polymers Adv Technol. (1992) 3:17–21. doi: 10.1002/pat.1992.220030103
50. Yoshioka H, Suzuki K, Miyauchi Y. Preventing aggregation of liposomes to use for artificial RBC in plasma by surface modification with PEG. Proceeding of the 11th annual meeting of Japanese membrane association (1989). p. 49
51. Klibanov AL, Maruyama K, Torchilin VP, Huang L. Amphipathic polyethyleneglycols effectively prolong the circulation time of liposomes. FEBS Lett. (1990) 268(1):235–7. doi: 10.1016/0014-5793(90)81016-H
52. Sakai H, Tomiyama K, Masada Y, Takeoka S, Horinouchi H, Kobayashi K, et al. Pretreatment of serum containing hb-vesicels (oxygen carriers) to prevent their interference in laboratory tests. Clin Chem Lab Med. (2003) 41:222–31. doi: 10.1515/CCLM.2003.036
53. Sakai H, Miyagawa N, Horinouchi H, Takeoka S, Takaori M, Tsuchida E, et al. Intravenous injection of hb-vesicles (artificial oxygen carriers) after hemodilution with a series of plasma expanders (water-soluble biopolymers) in a rat repeated hemorrhage model. Polymers Adv Technol. (2011) 22:1216–22. doi: 10.1002/pat.1964
54. Phillips WT, Klipper R, Fresne D, Rudolph AS, Javors M, Goins B. Platelet reactivity with liposome-encapsulated hemoglobin in the rat. Exp Hematol. (1997) 25(13):1347–56. https://pubmed.ncbi.nlm.nih.gov/9406994/
55. Szebeni J, Fontana JL, Wassef NM, Mongan PD, Morse DS, Dobbins DE, et al. Hemodynamic changes induced by liposomes and liposome-encapsulated hemoglobin in pigs: a model for pseudoallergic cardiopulmonary reactions to liposomes. Role of complement and inhibition by soluble CR1 and anti-C5a antibody. Circulation. (1999) 99(17):2302–9. doi: 10.1161/01.CIR.99.17.2302
56. Sakai H, Suzuki Y, Sou K, Kano M. Cardiopulmonary hemodynamic responses to the small injection of hemoglobin vesicles (artificial oxygen carriers) in miniature pigs. J Biomed Mater Res A. (2012) 100(10):2668–77. doi: 10.1002/jbm.a.34208
57. Abe H, Azuma H, Yamaguchi M, Fujihara M, Ikeda H, Sakai H, et al. Effects of hemoglobin vesicles, a liposomal artificial oxygen carrier, on hematological responses, complement and anaphylactic reactions in rats. Artif Cells Blood Substit Immobil Biotechnol. (2007) 35(2):157–72. doi: 10.1080/10731190601188224
58. Sou K, Tsuchida E. Electrostatic interactions and complement activation on the surface of phospholipid vesicle containing acidic lipids: effect of the structure of acidic groups. Biochim Biophys Acta. (2008) 1778(4):1035–41. doi: 10.1016/j.bbamem.2008.01.006
59. Takeoka S, Terase K, Yokohama H, Sakai H, Nishide H, Tsuchida E. Interaction between phospholipid assemblies and hemoglobin (Hb). J Macromol Sci Pure Appl Chem. (1994) A31(1):97–108. doi: 10.1080/10601329409349720
60. Sakai H, Kobayashi N, Kure T, Okuda C. Translational research of hemoglobin vesicles as a transfusion alternative. Curr Med Chem. (2022) 29(3):591–606. doi: 10.2174/0929867328666210412130035
61. Sakai H, Sato A, Takeoka S, Tsuchida E. Rheological properties of hemoglobin vesicles (artificial oxygen carriers) suspended in a series of plasma substitute solutions. Langmuir. (2007) 23:8121–8. doi: 10.1021/la7004503
62. Azuma H, Fujihara M, Sakai H. Biocompatibility of HbV: liposome-encapsulated hemoglobin molecules-liposome effects on immune function. J Funct Biomater. (2017) 8(3):E24. doi: 10.3390/jfb8030024
63. Sou K, Klipper R, Goins B, Tsuchida E, Phillips WT. Circulation kinetics and organ distribution of hb-vesicles developed as a red blood cell substitute. J Pharmacol Exp Ther. (2005) 312(2):702–9. doi: 10.1124/jpet.104.074534
64. Taguchi K, Urata Y, Anraku M, Maruyama T, Watanabe H, Sakai H, et al. Pharmacokinetic study of enclosed hemoglobin and outer lipid component after the administration of hemoglobin-vesicles as an artificial oxygen carrier. Drug Metab Dispos. (2009) 37:1456–63. doi: 10.1124/dmd.109.027094
65. Sakai H, Masada Y, Horinouchi H, Ikeda E, Sou K, Takeoka S, et al. Physiologic capacity of reticuloendothelial system for degradation of hemoglobin-vesicles (artificial oxygen carriers) after massive intravenous doses by daily repeated infusions for 14 days. J Pharmacol Exp Therap. (2004) 311:874–84. doi: 10.1124/jpet.104.073049
66. Sakai H, Horinouchi H, Tomiyama K, Ikeda E, Takeoka S, Kobayashi K, et al. Hemoglobin-vesicles as oxygen carriers: influence on phagocytic activity and histopathological changes in reticuloendothelial system. Am J Pathol. (2001) 159(3):1079–88. doi: 10.1016/S0002-9440(10)61783-X
67. Sakai H, Horinouchi H, Azuma H, Otagiri M, Kobayashi K. Safety evaluation of artificial red cells (hemoglobin-vesicles) as a transfusion alternative. Artif Blood. (2013) 21(1):36–48 (in Japanese).
68. Sakai H, Horinouchi H, Yamamoto M, Ikeda E, Takeoka S, Takaori M, et al. Acute 40 percent exchange-transfusion with hemoglobin-vesicles (HbV) suspended in recombinant human serum albumin solution: degradation of HbV and erythropoiesis in a rat spleen for 2 weeks. Transfusion. (2006) 46(3):339–47. doi: 10.1111/j.1537-2995.2006.00727.x
69. Takase B, Higashimura Y, Asahina H, Masaki N, Kinoshita M, Sakai H. Intraosseous infusion of liposome-encapsulated hemoglobin (HbV) acutely prevents hemorrhagic anemia-induced lethal arrhythmias, and its efficacy persists with preventing proarrhythmic side effects in the subacute phase of severe hemodilution model. Artif Organs. (2022) 46(6):1107–21. doi: 10.1111/aor.14170
70. Sakai H, Tsai AG, Kerger H, Park SI, Takeoka S, Nishide H, et al. Subcutaneous microvascular responses to hemodilution with a red cell substitute consisting of polyethyleneglycol-modified vesicles encapsulating hemoglobin. J Biomed Mater Res. (1998) 40(1):66–78. doi: 10.1002/(SICI)1097-4636(199804)40:1%3C66::AID-JBM8%3E3.0.CO;2-P
71. Sakai H, Tsai AG, Rohlfs RJ, Hara H, Takeoka S, Tsuchida E, et al. Microvascular responses to hemodilution with hb vesicles as red blood cell substitutes: influence of O2 affinity. Am J Physiol Heart Circ Physiol. (1999) 276(2):H553–62. doi: 10.1152/ajpheart.1999.276.2.H553
72. Sakai H, Masada Y, Horinouchi H, Yamamoto M, Ikeda E, Takeoka S, et al. Hemoglobin-vesicles suspended in recombinant human serum albumin for resuscitation from hemorrhagic shock in anesthetized rats. Crit Care Med. (2004) 32(2):539–45. doi: 10.1097/01.CCM.0000109774.99665.22
73. Sakai H, Seishi Y, Obata Y, Takeoka S, Horinouichi H, Tsuchida E, et al. Fluid resuscitation with artificial oxygen carriers in hemorrhaged rats: profiles of hemoglobin-vesicle degradation and hematopoiesis for 14 days. Shock. (2009) 31(2):192–200. doi: 10.1097/SHK.0b013e31817d4066
74. Tokuno M, Taguchi K, Yamasaki K, Sakai H, Otagiri M. Long-Term stored hemoglobin-vesicles, a cellular type of hemoglobin-based oxygen carrier, has resuscitative effects comparable to that for fresh red blood cells in a rat model with massive hemorrhage without post-transfusion lung injury. PLoS One. (2016) 11(10):e0165557. doi: 10.1371/journal.pone.0165557
75. Yoshizu A, Izumi Y, Park S, Sakai H, Takeoka S, Horinouchi H, et al. Hemorrhagic shock resuscitation with an artificial oxygen carrier, hemoglobin vesicle, maintains intestinal perfusion and suppresses the increase in plasma tumor necrosis factor-alpha. ASAIO J. (2004) 50(5):458–63. doi: 10.1097/01.MAT.0000136508.51676.EF
76. Terajima K, Tsueshita T, Sakamoto A, Ogawa R. Fluid resuscitation with hemoglobin vesicles in a rabbit model of acute hemorrhagic shock. Shock. (2006) 25(2):184–9. doi: 10.1097/01.shk.0000192118.68295.5d
77. Hagisawa K, Kinoshita M, Takase B, Hashimoto K, Saitoh D, Seki S, et al. Efficacy of resuscitative transfusion with hemoglobin vesicles in the treatment of massive hemorrhage in rabbits with thrombocytopenic coagulopathy and its effect on hemostasis by platelet transfusion. Shock. (2018) 50(3):324–30. doi: 10.1097/SHK.0000000000001042
78. Hagisawa K, Kinoshita M, Takikawa M, Takeoka S, Saitoh D, Seki S, et al. Combination therapy using fibrinogen γ-chain peptide-coated, ADP-encapsulated liposomes and hemoglobin vesicles for trauma-induced massive hemorrhage in thrombocytopenic rabbits. Transfusion. (2019) 59(10):3186–96. doi: 10.1111/trf.15427
79. Hagisawa K, Kinoshita M, Saitoh D, Morimoto Y, Sakai H. Intraosseous transfusion of hemoglobin vesicles in the treatment of hemorrhagic shock with collapsed vessels in a rabbit model. Transfusion. (2020) 60(7):1400–9. doi: 10.1111/trf.15915
80. Yamamoto M, Horinouchi H, Kobayashi K, Seishi Y, Sato N, Itoh M, et al. Fluid resuscitation of hemorrhagic shock with hemoglobin vesicles in beagle dogs: pilot study. Artif Cells Blood Substit Immobil Biotechnol. (2012) 40(1–2):179–95. doi: 10.3109/10731199.2011.637929
81. Seishi Y, Horinouchi H, Sakai H, Kobayashi K. Effect of the cellular-type artificial oxygen carrier hemoglobin vesicle as a resuscitative fluid for prehospital treatment: experiments in a rat uncontrolled hemorrhagic shock model. Shock. (2012) 38(2):153–8. doi: 10.1097/SHK.0b013e31825ad7cf
82. Yuki Y, Hagisawa K, Kinoshita M, Ishibashi H, Kaneko K, Ishida O, et al. Efficacy of resuscitative infusion with hemoglobin vesicles in rabbits with massive obstetric hemorrhage. Am J Obstet Gynecol. (2021) 224(4):398.e1–398.e11. doi: 10.1016/j.ajog.2020.09.010
83. Ishibashi H, Hagisawa K, Kinoshita M, Yuki Y, Miyamoto M, Kure T, et al. Resuscitative efficacy of hemoglobin vesicles for severe postpartum hemorrhage in pregnant rabbits. Sci Rep. (2021) 11(1):22367. doi: 10.1038/s41598-021-01835-w
84. Kohno M, Ikeda T, Hashimoto R, Izumi Y, Watanabe M, Horinouchi H, et al. Acute 40% exchange-transfusion with hemoglobin-vesicles in a mouse pneumonectomy model. PLoS One. (2017) 12(6):e0178724. doi: 10.1371/journal.pone.0178724
85. Hashimoto R, Kohno M, Oiwa K, Onozawa H, Watanabe M, Horinouchi H, et al. Immediate effects of systemic administration of Normal and high O2-affinity haemoglobin vesicles as a transfusion alternative in a rat pneumonectomy model. BMJ Open Respir Res. (2020) 7(1):e000476. doi: 10.1136/bmjresp-2019-000476
86. Oiwa K, Kohno M, Onozawa H, Hashimoto R, Masuda R, Watanabe M, et al. Histological evaluation for the efficacy of hemoglobin vesicles in a canine pneumonectomy 30% hemorrhage model. Artif Blood. (2021) 29:15 (in Japanese).
87. Li H, Ohta H, Tahara Y, Nakamura S, Taguchi K, Nakagawa M, et al. Artificial oxygen carriers rescue placental hypoxia and improve fetal development in the rat pre-eclampsia model. Sci Rep. (2015) 5:15271. doi: 10.1038/srep15271
88. Yamazaki M, Aeba R, Yozu R, Kobayashi K. Use of hemoglobin vesicles during cardiopulmonary bypass priming prevents neurocognitive decline in rats. Circulation. (2006) 114(1 Suppl):I220–5. doi: 10.1161/CIRCULATIONAHA.105.000562
89. Komatsu H, Furuya T, Sato N, Ohta K, Matsuura A, Ohmura T, et al. Effect of hemoglobin vesicle, a cellular-type artificial oxygen carrier, on middle cerebral artery occlusion and arachidonic acid-induced stroke models in rats. Neurosci Lett. (2007) 421(2):121–5. doi: 10.1016/j.neulet.2007.04.080
90. Plock JA, Tromp AE, Contaldo C, Spanholtz T, Sinovcic D, Sakai H, et al. Hemoglobin vesicles reduce hypoxia-related inflammation in critically ischemic hamster flap tissue. Crit Care Med. (2007) 35(3):899–905. doi: 10.1097/01.CCM.0000257463.71761.97
91. Plock JA, Rafatmehr N, Sinovcic D, Schnider J, Sakai H, Tsuchida E, et al. Hemoglobin vesicles improve wound healing and tissue survival in critically ischemic skin in mice. Am J Physiol Heart Circ Physiol. (2009) 297(3):H905–10. doi: 10.1152/ajpheart.00430.2009
92. Nakajima J, Bessho M, Adachi T, Yamagishi T, Tokuno S, Horinouchi H, et al. Hemoglobin vesicle improves recovery of cardiac function after ischemia-reperfusion by attenuating oxidative stress in isolated rat hearts. J Cardiovasc Pharmacol. (2011) 58(5):528–34. doi: 10.1097/FJC.0b013e31822de06e
93. Naito Y, Sakai H, Inoue S, Kawaguchi M. Hemoglobin vesicles prolong the time to circulatory collapse in rats during apnea. BMC Anesthesiol. (2017) 17(1):44. doi: 10.1186/s12871-017-0338-y
94. Yamamoto M, Izumi Y, Horinouchi H, Teramura Y, Sakai H, Kohno M, et al. Systemic administration of hemoglobin vesicle elevates tumor tissue oxygen tension and modifies tumor response to irradiation. J Surg Res. (2009) 151(1):48–54. doi: 10.1016/j.jss.2007.12.770
95. Kobayashi M, Mori T, Kiyono Y, Tiwari VN, Maruyama R, Kawai K, et al. Cerebral oxygen metabolism of rats using injectable 15O-oxygen with a steady-state method. J Cereb Blood Flow Metab. (2012) 32(1):33–40. doi: 10.1038/jcbfm.2011.125
96. Tiwari VN, Kiyono Y, Kobayashi M, Mori T, Kudo T, Okazawa H, et al. Automatic labeling method for injectable 15O-oxygen using hemoglobin-containing liposome vesicles and its application for measurement of brain oxygen consumption by PET. Nucl Med Biol. (2010) 37(1):77–83. doi: 10.1016/j.nucmedbio.2009.08.004
97. Verdu EF, Bercik P, Huang XX, Lu J, Al-Mutawaly N, Sakai H, et al. The role of luminal factors in the recovery of gastric function and behavioral changes after chronic Helicobacter pylori infection. Am J Physiol Gastrointest Liver Physiol. (2008) 295(4):G664–70. doi: 10.1152/ajpgi.90316.2008
98. Araki J, Sakai H, Takeuchi D, Kagaya Y, Tashiro K, Naito M, et al. Normothermic preservation of the rat hind limb with artificial oxygen-carrying hemoglobin vesicles. Transplantation. (2015) 99(4):687–92. doi: 10.1097/TP.0000000000000528
99. Shonaka T, Matsuno N, Obara H, Yoshikawa R, Nishikawa Y, Ishihara Y, et al. Impact of human-derived hemoglobin based oxygen vesicles as a machine perfusion solution for liver donation after cardiac death in a pig model. PLoS One. (2019) 14(12):e0226183. doi: 10.1371/journal.pone.0226183
100. Iwata H, Toriumi A, Ishii D, Yamamoto A, Sato Y, Takahashi H, et al. Applicability of hemoglobin vesicles as transplantable liver perfusion preservation solution. Artif Blood. (2021) 29(1):43–9.
101. Montagne K, Huang H, Ohara K, Matsumoto K, Mizuno A, Ohta K, et al. Use of liposome encapsulated hemoglobin as an oxygen carrier for fetal and adult rat liver cell culture. J Biosci Bioeng. (2011) 112(5):485–90. doi: 10.1016/j.jbiosc.2011.07.004
102. Rikihisa N, Watanabe S, Satoh K, Saito Y, Sakai H. Photosensitizer effects of artificial red cells on dye Laser irradiation in an animal model assuming port-wine stain treatment. Plast Reconstr Surg. (2017) 139(3):707e–16e. doi: 10.1097/PRS.0000000000003082
103. Shimanouchi K, Rikihisa N, Saito Y, Iuchi K, Tsumura N, Sakai H, et al. Artificial red blood cells increase large vessel wall damage and decrease surrounding dermal tissue damage in a rabbit auricle model after subsequent flashlamp-pumped pulsed-dye laser treatment. J Dermatol. (2021) 48(5):600–12. doi: 10.1111/1346-8138.15805
104. Sakai H, Horinouchi H, Tsuchida E, Kobayashi K. Hemoglobin vesicles and red blood cells as carriers of carbon monoxide prior to oxygen for resuscitation after hemorrhagic shock in a rat model. Shock. (2009) 31(5):507–14. doi: 10.1097/SHK.0b013e318188f83d
105. Okuda C, Sakai H. Effect of carbon monoxide administration using haemoglobin-vesicles on the hippocampal tissue. Artif Cells Nanomed Biotechnol. (2022) 50(1):1–9. doi: 10.1080/21691401.2022.2027428
106. Nagao S, Taguchi K, Sakai H, Tanaka R, Horinouchi H, Watanabe H, et al. Carbon monoxide-bound hemoglobin-vesicles for the treatment of bleomycin-induced pulmonary fibrosis. Biomaterials. (2014) 35(24):6553–62. doi: 10.1016/j.biomaterials.2014.04.049
107. Nagao S, Taguchi K, Miyazaki Y, Wakayama T, Chuang VT, Yamasaki K, et al. Evaluation of a new type of nano-sized carbon monoxide donor on treating mice with experimentally induced colitis. J Control Release. (2016) 234:49–58. doi: 10.1016/j.jconrel.2016.05.016
108. Nagao S, Taguchi K, Sakai H, Yamasaki K, Watanabe H, Otagiri M, et al. Carbon monoxide-bound hemoglobin vesicles ameliorate multiorgan injuries induced by severe acute pancreatitis in mice by their anti-inflammatory and antioxidant properties. Int J Nanomedicine. (2016) 11:5611–20. doi: 10.2147/IJN.S118185
109. Taguchi K, Nagao S, Maeda H, Yanagisawa H, Sakai H, Yamasaki K, et al. Biomimetic carbon monoxide delivery based on hemoglobin vesicles ameliorates acute pancreatitis in mice via the regulation of macrophage and neutrophil activity. Drug Deliv. (2018) 25(1):1266–74. doi: 10.1080/10717544.2018.1477860
110. Rikihisa N, Shimanouchi K, Saito Y, Sakai H, Mitsukawa N. Carbon monoxide combined with artificial blood cells acts as an antioxidant for tissues thermally damaged by dye laser irradiation. Burns. (2022):S0305-4179(22)00063-8. in press. doi: 10.1016/j.burns.2022.03.009. [Online ahead of print]35410695
111. Taguchi K, Suzuki Y, Tsutsuura M, Hiraoka K, Watabe Y, Enoki Y, et al. Liposomal artificial red blood cell-based carbon monoxide donor is a potent renoprotectant against cisplatin-induced acute kidney injury. Pharmaceutics. (2021) 14(1):57. doi: 10.3390/pharmaceutics14010057
112. Watabe Y, Taguchi K, Sakai H, Enoki Y, Maruyama T, Otagiri M, et al. Bioinspired carbon monoxide delivery using artificial blood attenuates the progression of obliterative bronchiolitis via suppression of macrophage activation by IL-17A. Eur J Pharm Biopharm. (2022) 170:43–51. doi: 10.1016/j.ejpb.2021.11.011
113. Suzuki Y, Taguchi K, Kure T, Sakai H, Enoki Y, Otagiri M, et al. Liposome-encapsulated methemoglobin as an antidote against cyanide poisoning. J Control Release. (2021) 337:59–70. doi: 10.1016/j.jconrel.2021.07.015
114. Suzuki Y, Taguchi K, Kure T, Enoki Y, Otagiri M, Sakai H, et al. Long-term pharmaceutical stability of liposome-encapsulated methemoglobin as an antidote for cyanide poisoning. Int J Pharm. (2021) 610:121260. doi: 10.1016/j.ijpharm.2021.121260
115. Suzuki Y, Taguchi K, Hanyu S, Kure T, Enoki Y, Otagiri M, et al. Oxidized artificial red blood cell rescues the lethal toxidrome of azide poisoning mice via recovering cytochrome c oxidase activity. J Drug Delivery Sci Technol. (2022) 71:103282. doi: 10.1016/j.jddst.2022.103282
116. Suzuki Y, Taguchi K, Kure T, Enoki Y, Otagiri M, Sakai H, et al. Liposomal methemoglobin as a potent antidote for hydrogen sulfide poisoning. Toxicol Appl Pharmacol. (2022) 450:116159. doi: 10.1016/j.taap.2022.116159
117. Siracusa R, Schaufler A, Calabrese V, Fuller PM, Otterbein LE. Carbon monoxide: from poison to clinical trials. Trends Pharmacol Sci. (2021) 42(5):329–39. doi: 10.1016/j.tips.2021.02.003
118. Misra H, Lickliter J, Kazo F, Abuchowski A. PEGylated carboxyhemoglobin bovine (SANGUINATE): results of a phase I clinical trial. Artif Organs. (2014) 38(8):702–7. doi: 10.1111/aor.12341
119. Sakai H, Yasuda S, Okuda C, Yamada T, Owaki K, Miwa Y. Examination of central nervous system by functional observation battery after massive intravenous infusion of carbon monoxide-bound and oxygen-bound hemoglobin vesicles in rats. Curr Res Pharmacol Drug Discovery. (2022) 3:100135. doi: 10.1016/j.crphar.2022.100135
Keywords: artificial oxygen carriers, blood substitutes, translational research, encapsulation, liposome, carbonylhemoglobin, methemoglobin (metHb)
Citation: Sakai H, Kure T, Taguchi K and Azuma H (2022) Research of storable and ready-to-use artificial red blood cells (hemoglobin vesicles) for emergency medicine and other clinical applications. Front. Med. Technol. 4:1048951. doi: 10.3389/fmedt.2022.1048951
Received: 20 September 2022; Accepted: 11 November 2022;
Published: 23 December 2022.
Edited by:
Binglan Yu, Harvard Medical School, United StatesReviewed by:
Jingxiao Chen, Jiangnan University, ChinaAntony P. McNamee, Griffith University, Australia
© 2022 Sakai, Kure, Taguchi and Azuma. This is an open-access article distributed under the terms of the Creative Commons Attribution License (CC BY). The use, distribution or reproduction in other forums is permitted, provided the original author(s) and the copyright owner(s) are credited and that the original publication in this journal is cited, in accordance with accepted academic practice. No use, distribution or reproduction is permitted which does not comply with these terms.
*Correspondence: Hiromi Sakai aGlyb3Nha2FpQG5hcmFtZWQtdS5hYy5qcA==
Specialty Section: This article was submitted to Regenerative Technologies, a section of the journal Frontiers in Medical Technology