- 1Department of Radiology, Huaxi MR Research Center, West China Hospital of Sichuan University, Chengdu, China
- 2Psychoradiology Research Unit of Chinese Academy of Medical Sciences, Functional and Molecular Imaging Key Laboratory of Sichuan Province, West China Hospital of Sichuan University, Chengdu, China
- 3Center for Psychiatric Neuroscience, Feinstein Institute for Medical Research, Manhasset, NY, United States
- 4Division of Psychiatry Research, Zucker Hillside Hospital, Glen Oaks, NY, United States
Introduction: Tourette syndrome (TS) is a neuropsychiatric disorder with multiple motor and vocal tics whose neural basis remains unclear. Diffusion tensor imaging (DTI) studies have demonstrated white matter microstructural alternations in TS, but the findings are inconclusive. In this study, we aimed to elucidate the most consistent white matter deficits in patients with TS.
Method: By systematically searching online databases up to December 2020 for all DTI studies comparing fractional anisotropy (FA) between patients with TS and healthy controls (HCs), we conducted anisotropic effect size-signed differential mapping (AES-SDM) meta-analysis to investigate FA differences in TS, as well as performed meta-regression analysis to explore the effects of demographics and clinical characteristics on white matter abnormalities among TS.
Results: A total of eight datasets including 168 patients with TS and 163 HCs were identified. We found that TS patients showed robustly decreased FA in the corpus callosum (CC) and right inferior longitudinal fasciculus (ILF) compared with HCs. These two regions preserved significance in the sensitivity analysis. No regions of increased FA were reported. Meta-regression analysis revealed that age, sex, tic severity, or illness duration of patients with TS were not linearly correlated with decreased FA.
Conclusion: Patients with TS display deficits of white matter microstructure in the CC and right ILF known to be important for interhemispheric connections as well as long association fiber bundles within one hemisphere. Because the results reported in the primary literature were highly variable, future investigations with large samples would be required to support the identified white matter changes in TS.
Introduction
Tourette syndrome (TS) is a common neuropsychiatric disorder characterized by rigid, repetitive, and intermittent movements and vocalizations termed tics (1). The prevalence of TS is 0.3–1% in school-aged children (2, 3). TS patients with behavioral comorbidities [e.g., attention deficit hyperactivity disorder (ADHD) or/and obsessive compulsive disorder (OCD)] are associated with impaired social function and reduced overall life quality (2). A large number of neuroimaging studies have detected brain functional and structural abnormalities of TS especially involving cortico-basal ganglia-thalamo-cortical network (4–8), which were linked to the emergence of tics (6). In addition, widespread dysconnectivity involving parietal, temporal, and occipital lobes and interhemispheric brain abnormalities have also been demonstrated in TS, which may relate to mental and behavioral changes in TS patients (9–16). Since normal structure of white matter tracts is critical for maintaining connections between distant brain regions, studying white matter alterations is crucial to elucidate potential neurobiological mechanisms underlying TS. In addition, it may also highlight potential neuroimaging markers related to tic severity and neurobehavioral abnormalities in TS patients (17).
Diffusion tensor imaging (DTI) is a particularly useful technique for investigating white matter abnormalities (18), while fractional anisotropy (FA) is widely used to measure the degree of directionality of cellular structures within the fiber tracts which reflect microstructural integrity of the brain (19–21). The voxel-based analysis (VBA) and tract-based spatial statistics (TBSS) method are commonly used to investigate whole-brain FA differences. The VBA technique measures contiguous clusters of significant white matter voxels with the correction of multiple comparisons and noise. The TBSS calculates significant clusters within white matter skeletons after isolating the central core of white matter tracts with the highest FA (22). Further meta-analysis has successfully combined both methods in one study (23). Widespread white matter abnormalities of TS have been revealed by DTI studies, but the results have been inconclusive. Specifically, while some studies revealed decreased FA in corpus callosum (CC) (11, 24), bilateral superior longitudinal fascicle (11), bilateral frontal lobe (11, 25), and left external or internal capsule region (11, 24), others identified increased FA in the left postcentral gyrus (26) or no significant FA differences between TS patients and healthy controls (HCs) (17, 27–29). This inconsistency might be due to sample size, sample heterogeneity, and/or methodological differences. Therefore, a whole-brain meta-analysis identifying reliable neurobiological markers of TS is of particular importance.
In this study, we performed a meta-analysis to determine white matter abnormalities in patients with TS via anisotropic effect size-signed differential mapping (AES-SDM) (30), which is an effective meta-analytic technique to quantify reproducibility of neuroimaging findings by weighting results from individual studies and controlling for multiple moderators. This method has been successfully applied to the study of major depressive disorder (23), childhood maltreatment (31), and bipolar disorder (32). For significant findings, we then conducted subgroup meta-analysis on TBSS results and performed a meta-regression analysis to examine effects of demographic and clinical characteristics on the discovered white matter microstructural alterations. We hypothesized that decreased FA was manifest in TS patients when compared with HCs, especially in motor- or vocal-related tracts.
Method
Inclusion of Studies
We searched relevant studies from PubMed, Medline, and Web of Science published up to December 2020 with keywords “Tourette syndrome” or “Gilles de la Tourette syndrome” plus “diffusion tensor imaging” or “DTI” or “fractional anisotropy” or “FA” or “white matter.” We also manually checked the reference lists of the retrieved articles for additional relevant studies. All studies included in this meta-analysis are according to the Preferred Reporting Items for Systematic Reviews and Meta-Analyses (PRISMA) guidelines (33).
The inclusion criteria were as follows: (1) studies in which patients were diagnosed according to Diagnostic and Statistical Manual of Mental Disorders, 4th Edition (DSM-IV); (2) studies that compared whole-brain FA alteration between patients with TS and HCs; (3) studies that used the VBA or TBSS approach for DTI data analysis; (4) studies whose results were based on Montreal Neurological Institute (MNI) or Talairach coordinates; and (5) studies that were published in English in a peer-reviewed journal.
The exclusion criteria were as follows: (1) case reports or reviews; (2) studies with < 10 participants in either TS or HC group; and (3) peak coordinates could not be retrieved from the published article or after contacting the authors.
Quality Assessment and Data Extraction
Two authors (C.M.Y. and L.Y.) independently searched the literature, assessed the quality of the retrieved articles, and extracted and cross-checked the data. In cases of disagreement, a third author helped to reach consensus. We assessed study quality using a 12-point checklist (see Supplementary Material) which was divided into three categories: participants (items 1–4), methods for image acquisition and analysis (items 5–10), and results and conclusions (items 11 and 12). Each item was given a score of 1, 0.5, or 0 to indicate whether the criteria were fully met, partially met, or unfulfilled, respectively, and any study scoring > 6.0 was included in the meta-analysis.
We extracted the following variables from each selected study: the characteristics of the participants and their illness (sample size, mean age of participants, sex, symptom severity, and drug status); magnetic resonance methodology (magnetic field strength, acquisition voxel size, number of diffusion directions, and type of analysis); statistical methodology (statistical threshold and correction methods for multiple comparisons); and 3D coordinates (for voxel-level quantitative meta-analyses).
Voxel-Wise Meta-Analysis: AES-SDM
We analyzed FA differences in white matter between patients with TS and HCs following the standardized process of AES-SDM (www.sdmproject.com) (30) and the detail flow chart was showed in Supplementary Figure 1. Briefly, the AES-SDM technique uses effect size to combine reported peak coordinates that are extracted from statistical parametric maps, which recreates original maps of the effect size of FA difference in white matter between patients and controls. The created maps were visualized by MRIcron software (www.mricro.com/mricron/) overlaid on a high-resolution brain template generated by the International Consortium for Brain Mapping. To allow combination of VBA and TBSS studies, we adopted the TBSS template included in AES-SDM, which has been used and described by Wise and colleagues (32). All analytical processes followed the SDM tutorial (https://www.sdmproject.com/software/tutorial.pdf) and related publication (30). We adopted the default AES-SDM thresholds (anisotropy = 1.0; full width at half maximum = 20 mm, voxel p = 0.005, peak height Z = 1, cluster extent = 10 voxels) (34). The AES-SDM results are represented on a 3D-rendered brain, removing part of the left or right hemisphere and highlighting areas of the brain with significant FA alterations (35).
Jackknife Sensitivity Analysis and Subgroup Analysis
To assess the robustness of the findings, we conducted a systematic whole-brain voxel-based jackknife analysis, in which we iteratively repeated the analysis, excluding one dataset at a time to establish the extent to which the results could be replicated. If a brain region remained significant in all or most of the combinations of studies, we considered the finding to be highly replicable (36). This was done both for combining studies with TBSS and VBA and for studies with TBSS alone. We did not perform meta-analysis in other subgroups (i.e., VBA method, adults, adolescents, medicated patients, and drug-free patients) due to the lack of sufficient samples to draw reliable conclusions.
Analysis of Heterogeneity and Publication Bias
Heterogeneity refers to between-study variations. We conducted a between-study heterogeneity analysis of individual clusters using a random-effects model with Q statistics, with thresholds of p = 0.005, peak Z = 1.00, and a cluster extent of 10 voxels. Areas showing significant heterogeneity overlapping with the main findings were explored using meta-regression analyses to search for the sources of between-study variability. We also assessed publication bias by testing funnel plots via the Egger test in which any result showing p < 0.05 was regarded as having a significant publication bias.
Meta-Regression Analysis
To characterize potential effects of key demographic and clinical variables on white matter, we performed meta-regression analysis using age, percentage of male patients, symptom severity [Yale Global Tic Severity Scale (YGTSS) (37)], and illness duration as independent variables in each study. The results were weighted by the square root of the sample sizes. To minimize spurious relationships, we selected a more conservative threshold of p = 0.0005 as used in previous studies (23, 30), requiring abnormalities to be detected both in the slope and in one of the extremes of the regressors, and discarded findings in regions other than those detected in the main analysis. We displayed the main output for each variable in a map of regression slope. Finally, we visually inspected regression plots to discard fittings that were obviously driven by too few studies (36).
Fiber Tracking
The DTI query software allows placement and interactive manipulation of box-shaped region of interest (ROI) to display pathways passing through specific anatomic areas, making it easier to explore and interpret white matter pathways (38). We used this technique to display the most probable white matter tracts passing through clusters of voxels that showed significant FA group differences with an atlas of human white matter anatomy (39). The white matter tracts were mapped using streamline tracking techniques as well as filtered by tract length and ROI centered on the coordinates of significant clusters.
Results
Included Studies and Sample Characteristics
The search strategy identified 310 potentially relevant studies, eight of which met our inclusion criteria. The flow chart for study inclusion is shown in Figure 1. A total of 168 patients with TS (mean age 20.0 years) and 163 HCs (mean age 20.1 years) were included. The clinical and demographic data from all included studies are summarized in Table 1. Among eight studies, five recruited 106 patients who were either never medicated or without medication at the recruitment point. Due to high comorbidity rate, only 45 patients in three studies had one diagnosis, and the others were simultaneously diagnosed with ADHD, OCD, anxiety disorder, or depression. FA alterations involved a total of 33 coordinates in the included studies. The quality scores, ranging from 10.5 to 12 (mean score 11; Table 1; Supplementary Table 1), demonstrated that the included studies were of high quality, ensuring a more exhaustive and accurate meta-analysis. The technical details and main findings of all included studies are summarized in Table 2.
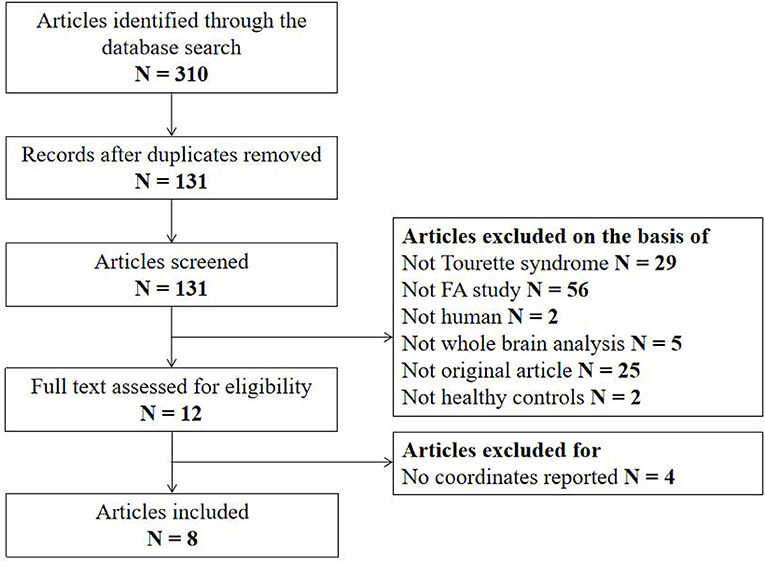
Figure 1. Flow diagram for the identification and exclusion of studies. N, number; FA, fractional anisotropy.
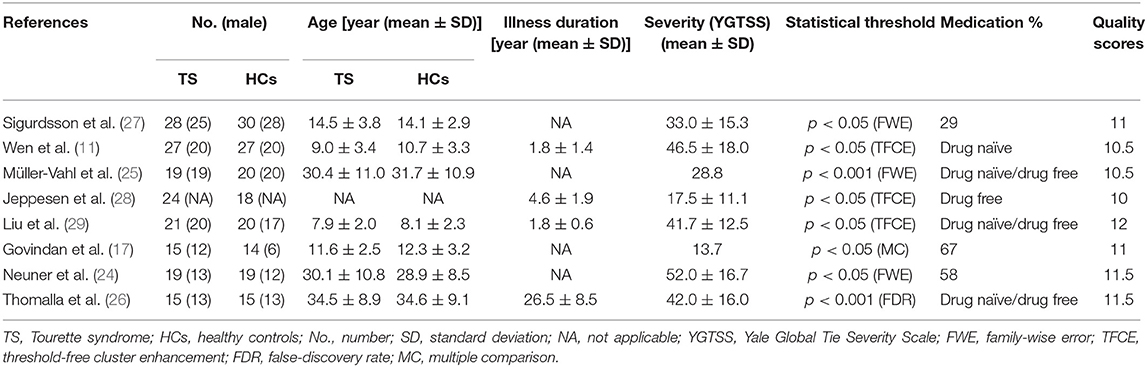
Table 1. Demographic and clinical characteristics of the participants in the eight studies included in the meta-analysis.
Pooled Meta-Analysis of All Included Studies
Pooled meta-analysis revealed two regions of decreased FA in TS patients compared with HCs: the white matter of the CC [MNI −10/−24/26, splenium of CC (SCC) Z = −1.573, p < 0.001] and right inferior longitudinal fasciculus (ILF, MNI 40/−76/−2, Z = −1.594, p < 0.001) (Table 3; Figure 2). White matter tracts traversing through these voxels were displayed in a bounding box size of 6.0 × 6.0 × 6.0 mm3 with DTI query software (Figure 2). No significant FA increase was found in patients with TS compared with HCs.
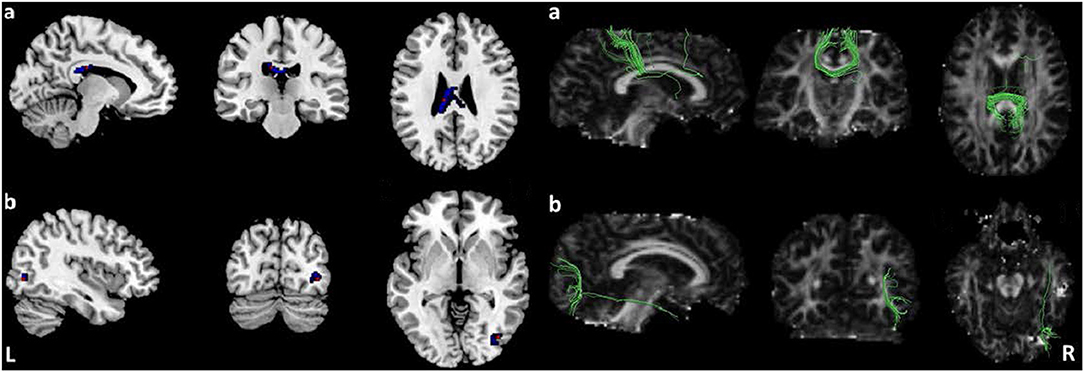
Figure 2. Results of pooled meta-analysis. Decreased FA of the CC (a) and right ILF (b) in TS patients compared with HCs and cluster-related white mater diffusion tensor tracts. Regions with blue color area showed lower FA in TS, red dots represent the peak coordinate. FA, fractional anisotropy; TS, Tourette syndrome; HCs, healthy controls; CC, corpus callosum; ILF, inferior longitudinal fasciculus.
Subgroup Meta-Analysis
The subgroup meta-analysis of TBSS method included six datasets that compared 134 patients with TS to 128 HCs. Decreased FA in the CC was significant in the subgroup analysis (MNI −10/−22/26, Z = −1.605, p < 0.001), while changes of the right ILF was not found (Table 4).
Jackknife, Heterogeneity, and Publication Bias Analysis
Whole-brain jackknife sensitivity analysis showed that decreased FA in CC in patients was highly replicable, being preserved in all but one combination (24); decreased FA in the right ILF remained significant in all but two combinations (11, 25) (Table 5). In the TBSS subgroup, the FA reduction in the CC was preserved in all combinations (Supplementary Table 2).
In the pooled meta-analysis, none of the regions with altered FA (Table 3) showed statistically significant heterogeneity between studies. Funnel plots demonstrated that the main findings were driven by at least six studies (Figure 3). Analysis of publication bias showed that the Egger test was non-significant for the CC (p = 0.832) and right ILF (p = 0.747).
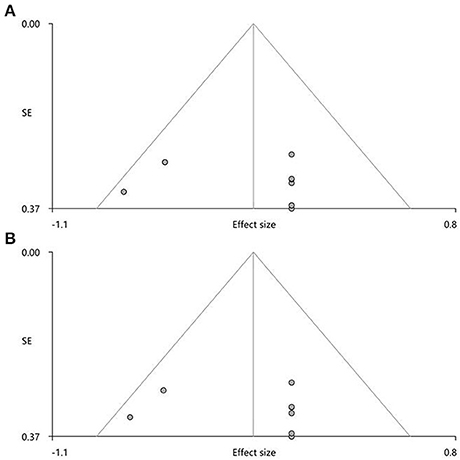
Figure 3. Results of funnel plot analysis to test for publication bias. For the pooled meta-analysis, the Egger's test and funnel plots revealed no significant publication bias (A) in the corpus callosum (Z = 0.75, t = 0.22, df = 6, p = 0.832) and (B) in the right inferior network, inferior longitudinal fasciculus (Z = 1.14, t = 0.34, df = 6, p = 0.747).
Meta-Regression Analysis
Age, sex, tic symptom severity (YGTSS), and illness duration were not significantly associated with TS-related white matter FA changes from the meta-regression analysis.
Discussion
To our knowledge, this is the first quantitative meta-analysis integrating DTI studies in patients with TS. Voxel-wise meta-analysis using AES-SDM in the present study supported that patients were associated with decreased FA in CC and right ILF. These results were robust under jackknife analysis. Clinical parameters including age, sex, tic severity, and illness duration, did not show significant association with white matter microstructural alterations.
The decreased FA in CC (mainly SCC) was found in TS patients compared with HCs in the present study, which is consistent with numerous previous studies (9–11). For example, it has previously been shown that FA values in the posterior portions of the CC (including SCC) were significantly lower in monozygotic twins of TS patients (9). Another structural study for deep white matter tracts revealed decreased FA in right SCC, and greater alterations were correlated with more severe tic symptoms in patients (11). The CC is the largest fiber bundle of the human brain connecting the left and right cerebral hemispheres while the SCC predominantly connects bilateral parietal and temporal areas (40). In line with our findings, Worbe et al. (14) suggested that patients with complex tics were associated with cortical thinning in parietal regions by showing that cortical thinning in parietal and temporal cortices was correlated negatively with the tic severity. Moreover, temporo-parietal junction (TPJ) is a functionally defined region pivotal to social cognition (41), and the dysfunction of TPJ could bring negative influence on conscious human experience and impact mental health (42). Task-fMRI studies (12, 13) on mental state judgments have demonstrated abnormal task-related activity in the right TPJ in patients, which was further shown to be related to the tic symptom. Therefore, the FA reduction in the SCC in the current study suggested impaired parietal and temporal interhemispheric connectivity, which might lead to neurocognitive deficits in mental and physical aspects of individuals with TS.
As the primary inhibitory neurotransmitter in the brain, g-aminobutyric acid (GABA) has been increasingly recognized to be involved in the pathophysiology of TS (43). Current evidence suggested that the tic symptom of TS was associated with dysfunction of GABA (44, 45), while GABAergic drugs have been shown to improve tics in a safe and effective way (46, 47). With the measurement of in vivo brain GABA non-invasively, a magnetic resonance spectroscopy (MRS) study demonstrated that GABA concentrations within the supplementary motor area (SMA) was linked to the pathogenesis of tics in TS and correlated with FA values within the CC (48). Furthermore, GABA concentrations within the SMA could be predicted by tic severity and FA values in the CC. Based on these findings, we suggested that decreased FA in the CC observed in our meta-analysis might provide new insight into treatment target in patients with TS. Future studies are needed to articulate the specific role of CC in GABA-related mechanisms underlying TS.
The present meta-analysis also identified significantly decreased FA in the right ILF in patients with TS compared with HCs. Previous DTI study combined both TBSS and atlas-based ROI analysis demonstrated a reduction of FA in the right ILF in TS patients, which was negatively associated with tic severity (11). The ILF is widely accepted as a direct connection between the occipital cortex and the temporal lobe (49) and is functionally correlated with thought disorders, visual emotion, and cognitive impairments (50). In addition, Latini and colleagues demonstrated that the ILF played an important role in integrating information of visual, memory, and emotions (51), where deficits of visual memory in TS were found to be related to occipital dysfunction (15), and cognitive disturbances were correlated with abnormal brain activity in temporal areas (16). The peak of altered FA located in the right middle and inferior occipital gyrus. There were several studies that provided evidence of gray matter alteration in the occipital brain region in patients with TS, including changed cortical thickness, cortical sulcus, and cortical curvature in occipital gyrus (52), and the abnormal functional connectivity was also found in the occipital area in patients with TS (53). In sum, our finding of the decreased FA in ILF (mainly in the occipital part) might underlie the deficits in visual emotional modulation and cognitive processing in patients with TS.
We conducted subgroup analysis confined to the TBSS method. Compared with the pooled meta-analysis, the subgroup revealed FA reduction only in the CC with larger clusters, but not in the right ILF. This finding was consistent with the previous study showing that TBSS may detect white matter abnormalities more accurately than VBA (32). The VBA method was not conducted in the subgroup analysis because there were only two original studies. Nevertheless, it was still worth noting that one of the VBA study also showed abnormal FA involving the posterior part of the CC (26). Hence, the FA reduction in the CC might be regarded as a stable indicator in TS and the TBSS technique was recommended to explored white matter microstructural abnormalities in this disorder.
The meta-regression analysis revealed that there were no significant associations between white matter microstructural alterations and examined demographic and clinical variables. Consistent with our findings, Plessen et al. have investigated the small size (on the midsagittal slice) and reduced FA values of CC in children with TS, respectively (10, 54). The results were not influenced by medication or comorbid illnesses. Furthermore, there were no significant correlations between reduction of FA in TS and the severities of tic symptoms (10). However, some studies provided evidence for a close relationship between the white matter microstructure of CC and tic severity (25, 55). Considering the small sample size for the included studies and potential confounding factors, the meta-regression analysis may result in negative results and future studies are needed for replication.
Limitation
This study has some limitations. Firstly, like most voxel-based meta-analyses, our study was based on summarized data (i.e., coordinates and effect sizes from published studies) rather than raw image files from original data (34), which may limit the precision of the spatial location of the observed effects. Second due to the high comorbidity rate of the included samples, the influences of medication treatment and comorbidities (e.g., ADHD or/and OCD) cannot be removed. As a result, future meta-analysis research is warranted when sufficient data on medication-naive patients without comorbidities are available in the literature. Thirdly, subgroup analysis was not able to be conducted comparing adults vs. adolescents and medicated patients vs. drug-free subjects, and therefore specific effects of age and medication status on the white matter microstructure need to be investigated in the future.
Conclusion
The present meta-analysis of DTI studies identified robust white matter deficits in the CC and right ILF in patients with TS, providing a strong evidence for disturbances in the interhemispheric connections and long association fiber bundles in the right hemisphere in TS. Because of limited studies in the literature, future investigations with larger sample size are warranted to provide further evidence for the white matter changes in TS.
Data Availability Statement
The original contributions presented in the study are included in the article/Supplementary Material, further inquiries can be directed to the corresponding author/s.
Author Contributions
SL contributed to the conception and design of the study, as well as the supervision of all the work of this review. CY and LY contributed to literature searching and drafting of the manuscript. All authors made critical revision of the manuscript for important intellectual content and gave final approval of the version to be submitted.
Funding
This study was supported by the National Natural Science Foundation of China (Grant Nos. 82120108014, 82071908, 81621003, 81671664, and 82102007), 1.3.5 Project for Disciplines of Excellence, West China Hospital, Sichuan University (Project Nos. ZYYC08001 and ZYJC18020), Sichuan Science and Technology Program (Grant Nos. 2021JDTD0002, 2020YJ0018, and 2021YFS0077), and Science and Technology Project of the Health Planning Committee of Sichuan (Project Nos. 19PJ078 and 20PJ010).
Conflict of Interest
WZ consulted to VeraSci.
The remaining authors declare that the research was conducted in the absence of any commercial or financial relationships that could be construed as a potential conflict of interest.
Publisher's Note
All claims expressed in this article are solely those of the authors and do not necessarily represent those of their affiliated organizations, or those of the publisher, the editors and the reviewers. Any product that may be evaluated in this article, or claim that may be made by its manufacturer, is not guaranteed or endorsed by the publisher.
Acknowledgments
SL acknowledges the support from Humboldt Foundation Friedrich Wihelm Bessel Research Award and Chang Jiang Scholars (Program No. T2019069).
Supplementary Material
The Supplementary Material for this article can be found online at: https://www.frontiersin.org/articles/10.3389/fneur.2021.659250/full#supplementary-material
References
1. Foltynie T. Vocal tics in Tourette's syndrome. Lancet Neurol. (2016) 15:e1. doi: 10.1016/S1474-4422(16)00021-1
3. Robertson MM, Eapen V, Cavanna AE. The international prevalence, epidemiology, and clinical phenomenology of Tourette syndrome: a cross-cultural perspective. J Psychosom Res. (2009) 67:475–83. doi: 10.1016/j.jpsychores.2009.07.010
4. Martino D, Ganos C, Worbe Y. Neuroimaging applications in Tourette's syndrome. Int Rev Neurobiol. (2018) 143:65–108. doi: 10.1016/bs.irn.2018.09.008
5. Conceição VA, Dias Â, Farinha AC, Maia TV. Premonitory urges and tics in Tourette syndrome: computational mechanisms and neural correlates. Curr Opin Neurobiol. (2017) 46:187–99. doi: 10.1016/j.conb.2017.08.009
6. Ganos C. Tics and Tourette's: update on pathophysiology and tic control. Curr Opin Neurol. (2016) 29:513–8. doi: 10.1097/WCO.0000000000000356
7. Hashemiyoon R, Kuhn J, Visser-Vandewalle V. Putting the pieces together in Gilles de la Tourette syndrome: exploring the link between clinical observations and the biological basis of dysfunction. Brain Topogr. (2017) 30:3–29. doi: 10.1007/s10548-016-0525-z
8. Wang L, Lee DY, Bailey E, Hartlein JM, Gado MH, Miller MI, et al. Validity of large-deformation high dimensional brain mapping of the basal ganglia in adults with Tourette syndrome. Psychiatry Res. (2007) 154:181–90. doi: 10.1016/j.pscychresns.2006.08.006
9. Cavanna AE, Stecco A, Rickards H, Servo S, Terazzi E, Peterson B, et al. Corpus callosum abnormalities in Tourette syndrome: an MRI-DTI study of monozygotic twins. J Neurol Neurosurg Psychiatry. (2010) 81:533–5. doi: 10.1136/jnnp.2009.173666
10. Plessen KJ, Grüner R, Lundervold A, Hirsch JG, Xu D, Bansal R, et al. Reduced white matter connectivity in the corpus callosum of children with Tourette syndrome. J Child Psychol Psychiatry Allied Disciplines. (2006) 47:1013–22. doi: 10.1111/j.1469-7610.2006.01639.x
11. Wen H, Liu Y, Wang J, Rekik I, Zhang J, Zhang Y, et al. Combining tract- and atlas-based analysis reveals microstructural abnormalities in early Tourette syndrome children. Hum Brain Mapp. (2016) 37:1903–19. doi: 10.1002/hbm.23146
12. Eddy CM, Cavanna AE, Hansen PC. Empathy and aversion: the neural signature of mentalizing in Tourette syndrome. Psychol Med. (2017) 47:507–17. doi: 10.1017/S0033291716002725
13. Eddy CM, Cavanna AE, Rickards HE, Hansen PC. Temporo-parietal dysfunction in Tourette syndrome: insights from an fMRI study of Theory of Mind. J Psychiatr Res. (2016) 81:102–11. doi: 10.1016/j.jpsychires.2016.07.002
14. Worbe Y, Gerardin E, Hartmann A, Valabrégue R, Chupin M, Tremblay L, et al. Distinct structural changes underpin clinical phenotypes in patients with Gilles de la Tourette syndrome. Brain. (2010) 133:3649–60. doi: 10.1093/brain/awq293
15. Rasmussen C, Soleimani M, Carroll A, Hodlevskyy O. Neuropsychological functioning in children with Tourette syndrome (TS). J Can Acad Child Adolesc Psychiatry. (2009) 18:307–15.
16. Zykov VP, Begasheva OI. Cognitive disturbances in patients with tics and Tourette's syndrome and their correction with encephabol. Zhurnal nevrologii i psikhiatrii imeni SS Korsakova. (2003) 103:10–14.
17. Govindan RM, Makki MI, Wilson BJ, Behen ME, Chugani HT. Abnormal water diffusivity in corticostriatal projections in children with Tourette syndrome. Hum Brain Mapp. (2010) 31:1665–74. doi: 10.1002/hbm.20970
18. Kubicki M, Westin CF, Maier SE, Mamata H, Frumin M, Ersner-Hershfield H, et al. Diffusion tensor imaging and its application to neuropsychiatric disorders. Harvard Rev Psychiatry. (2002) 10:324–36. doi: 10.1080/10673220216231
19. Ahn S, Lee SK. Diffusion tensor imaging: exploring the motor networks and clinical applications. Korean J Radiol. (2011) 12:651–61. doi: 10.3348/kjr.2011.12.6.651
20. Le Bihan D, Mangin JF, Poupon C, Clark CA, Pappata S, Molko N, et al. Diffusion tensor imaging: concepts and applications. JMRI. (2001) 13:534–46. doi: 10.1002/jmri.1076
21. Taylor WD, Hsu E, Krishnan KR, MacFall JR. Diffusion tensor imaging: background, potential, and utility in psychiatric research. Biol Psychiatry. (2004) 55:201–7. doi: 10.1016/j.biopsych.2003.07.001
22. Smith SM, Jenkinson M, Johansen-Berg H, Rueckert D, Nichols TE, Mackay CE, et al. Tract-based spatial statistics: voxelwise analysis of multi-subject diffusion data. Neuroimage. (2006) 31:1487–505. doi: 10.1016/j.neuroimage.2006.02.024
23. Jiang J, Zhao YJ, Hu XY, Du MY, Chen ZQ, Wu M, et al. Microstructural brain abnormalities in medication-free patients with major depressive disorder: a systematic review and meta-analysis of diffusion tensor imaging. J Psychiatry Neurosci. (2017) 42:150–63. doi: 10.1503/jpn.150341
24. Neuner I, Kupriyanova Y, Stocker T, Huang R, Posnansky O, Schneider F, et al. White-matter abnormalities in Tourette syndrome extend beyond motor pathways. Neuroimage. (2010) 51:1184–93. doi: 10.1016/j.neuroimage.2010.02.049
25. Müller-Vahl KR, Grosskreutz J, Prell T, Kaufmann J, Bodammer N, Peschel T. Tics are caused by alterations in prefrontal areas, thalamus and putamen, while changes in the cingulate gyrus reflect secondary compensatory mechanisms. Bmc Neurosci. (2014) 15:6. doi: 10.1186/1471-2202-15-6
26. Thomalla G, Siebner HR, Jonas M, Baumer T, Biermann-Ruben K, Hummel F, et al. Structural changes in the somatosensory system correlate with tic severity in Gilles de la Tourette syndrome. Brain. (2009) 132:765–77. doi: 10.1093/brain/awn339
27. Sigurdsson HP, Pepes SE, Jackson GM, Draper A, Morgan PS, Jackson SR. Alterations in the microstructure of white matter in children and adolescents with Tourette syndrome measured using tract-based spatial statistics and probabilistic tractography. Cortex. (2018) 104:75–89. doi: 10.1016/j.cortex.2018.04.004
28. Jeppesen SS, Debes NM, Simonsen HJ, Rostrup E, Larsson HB, Skov L. Study of medication-free children with Tourette syndrome do not show imaging abnormalities. Mov Disord. (2014) 29:1212–6. doi: 10.1002/mds.25858
29. Liu Y, Miao W, Wang J, Gao P, Yin G, Zhang L, et al. Structural abnormalities in early Tourette syndrome children: a combined voxel-based morphometry and tract-based spatial statistics study. PLoS ONE. (2013) 8:e76105. doi: 10.1371/journal.pone.0076105
30. Radua J, Rubia K, Canales-Rodríguez EJ, Pomarol-Clotet E, Fusar-Poli P, Mataix-Cols D. Anisotropic kernels for coordinate-based meta-analyses of neuroimaging studies. Front Psychiatry. (2014) 5:13. doi: 10.3389/fpsyt.2014.00013
31. Lim L, Radua J, Rubia K. Gray matter abnormalities in childhood maltreatment: a voxel-wise meta-analysis. Am J Psychiatry. (2014) 171:854–863. doi: 10.1176/appi.ajp.2014.13101427
32. Wise T, Radua J, Nortje G, Cleare AJ, Young AH, Arnone D. Voxel-based meta-analytical evidence of structural disconnectivity in major depression and bipolar disorder. Biol Psychiatry. (2016) 79:293–302. doi: 10.1016/j.biopsych.2015.03.004
33. Moher D, Liberati A, Tetzlaff J, Altman DG. Preferred reporting items for systematic reviews and meta-analyses: the PRISMA statement. BMJ. (2009) 339:b2535. doi: 10.1136/bmj.b2535
34. Radua J, Mataix-Cols D, Phillips ML, El-Hage W, Kronhaus DM, Cardoner N, et al. A new meta-analytic method for neuroimaging studies that combines reported peak coordinates and statistical parametric maps. Eur Psychiatry. (2012) 27:605–11. doi: 10.1016/j.eurpsy.2011.04.001
35. Du M, Liu J, Chen Z, Huang X, Li J, Kuang W, et al. Brain grey matter volume alterations in late-life depression. J Psychiatry Neurosci. (2014) 39:397–406. doi: 10.1503/jpn.130275
36. Radua J, Mataix-Cols D. Voxel-wise meta-analysis of grey matter changes in obsessive-compulsive disorder. Br J Psychiatry. (2009) 195:393–402. doi: 10.1192/bjp.bp.108.055046
37. Leckman JF, Riddle MA, Hardin MT, Ort SI, Swartz KL, Stevenson J, et al. The Yale Global Tic Severity Scale: initial testing of a clinician-rated scale of tic severity. J Am Acad Child Adolesc Psychiatry. (1989) 28:566–73. doi: 10.1097/00004583-198907000-00015
38. Sherbondy A, Akers D, Mackenzie R, Dougherty R, Wandell B. Exploring connectivity of the brain's white matter with dynamic queries. IEEE Trans Visual Comput Graph. (2005) 11:419–30. doi: 10.1109/TVCG.2005.59
39. Wakana S, Jiang H, Nagae-Poetscher LM, van Zijl PC, Mori S. Fiber tract-based atlas of human white matter anatomy. Radiology. (2004) 230:77–87. doi: 10.1148/radiol.2301021640
40. Knyazeva MG. Splenium of corpus callosum: patterns of interhemispheric interaction in children and adults. Neural Plast. (2013) 2013:12. doi: 10.1155/2013/639430
41. Mars RB, Sallet J, Schüffelgen U, Jbabdi S, Toni I, Rushworth MF. Connectivity-based subdivisions of the human right “temporoparietal junction area”: evidence for different areas participating in different cortical networks. Cerebral Cortex. (2012) 22:1894–903. doi: 10.1093/cercor/bhr268
42. Eddy CM. The junction between self and other? Temporo-parietal dysfunction in neuropsychiatry. Neuropsychologia. (2016) 89:465–77. doi: 10.1016/j.neuropsychologia.2016.07.030
43. Leckman JF, Bloch MH, Smith ME, Larabi D, Hampson M. Neurobiological substrates of Tourette's disorder. J Child Adolesc Psychopharmacol. (2010) 20:237–47. doi: 10.1089/cap.2009.0118
44. Di Lazzaro V, Pilato F, Dileone M, Ranieri F, Ricci V, Profice P, et al. GABAA receptor subtype specific enhancement of inhibition in human motor cortex. Journal Physiol. (2006) 575:721–6. doi: 10.1113/jphysiol.2006.114694
45. Lerner A, Bagic A, Simmons JM, Mari Z, Bonne O, Xu B, et al. Widespread abnormality of the γ-aminobutyric acid-ergic system in Tourette syndrome. Brain. (2012) 135:1926–36. doi: 10.1093/brain/aws104
46. Müller-Vahl KR. The treatment of Tourette's syndrome: current opinions. Expert Opin Pharmacother. (2002) 3:899–914. doi: 10.1517/14656566.3.7.899
47. Singer HS, Wendlandt J, Krieger M, Giuliano J. Baclofen treatment in Tourette syndrome: a double-blind, placebo-controlled, crossover trial. Neurology. (2001) 56:599–604. doi: 10.1212/WNL.56.5.599
48. Draper A, Stephenson MC, Jackson GM, Pépés S, Morgan PS, Morris PG, et al. Increased GABA contributes to enhanced control over motor excitability in Tourette syndrome. Curr Biol. (2014) 24:2343–7. doi: 10.1016/j.cub.2014.08.038
49. Ashtari M. Anatomy and functional role of the inferior longitudinal fasciculus: a search that has just begun. Dev Med Child Neurol. (2012) 54:6–7. doi: 10.1111/j.1469-8749.2011.04122.x
50. Chanraud S, Zahr N, Sullivan EV, Pfefferbaum A. MR diffusion tensor imaging: a window into white matter integrity of the working brain. Neuropsychol Rev. (2010) 20:209–25. doi: 10.1007/s11065-010-9129-7
51. Latini F, Mårtensson J, Larsson EM, Fredrikson M, Åhs F, Hjortberg M, et al. Segmentation of the inferior longitudinal fasciculus in the human brain: a white matter dissection and diffusion tensor tractography study. Brain Res. (2017) 1675:102–15. doi: 10.1016/j.brainres.2017.09.005
52. Kong L, Lv B, Wu T, Zhang J, Fan Y, Ouyang M, et al. Altered structural cerebral cortex in children with Tourette syndrome. Eur J Radiol. (2020) 129:109119. doi: 10.1016/j.ejrad.2020.109119
53. Rae CL, Polyanska L, Gould van Praag CD, Parkinson J, Bouyagoub S, Nagai Y, et al. Face perception enhances insula and motor network reactivity in Tourette syndrome. Brain. (2018) 141:3249–3261. doi: 10.1093/brain/awy254
54. Plessen KJ, Wentzel-Larsen T, Hugdahl K, Feineigle P, Klein J, Staib LH, et al. Altered interhemispheric connectivity in individuals with Tourette's disorder. Am J Psychiatry. (2004) 161:2028–2037. doi: 10.1176/appi.ajp.161.11.2028
Keywords: Tourette syndrome, diffusion tensor imaging, fractional anisotropy, magnetic resonance imaging, meta-analysis
Citation: Yang C, Yao L, Liu N, Zhang W, Tao B, Cao H, Gong Q and Lui S (2021) Microstructural Abnormalities of White Matter Across Tourette Syndrome: A Voxel-Based Meta-Analysis of Fractional Anisotropy. Front. Neurol. 12:659250. doi: 10.3389/fneur.2021.659250
Received: 28 January 2021; Accepted: 07 June 2021;
Published: 09 September 2021.
Edited by:
Wenbin Guo, Central South University, ChinaReviewed by:
Zhipeng Yang, Chengdu University of Information Technology, ChinaBo Gao, Affiliated Hospital of Guizhou Medical University, China
Copyright © 2021 Yang, Yao, Liu, Zhang, Tao, Cao, Gong and Lui. This is an open-access article distributed under the terms of the Creative Commons Attribution License (CC BY). The use, distribution or reproduction in other forums is permitted, provided the original author(s) and the copyright owner(s) are credited and that the original publication in this journal is cited, in accordance with accepted academic practice. No use, distribution or reproduction is permitted which does not comply with these terms.
*Correspondence: Su Lui, lusuwcums@tom.com
†These authors have contributed equally to this work