- 1Neuropharmacology Research Strength, Jeffrey Cheah School of Medicine and Health Sciences, Monash University Malaysia, Subang Jaya, Malaysia
- 2Epilepsy Program, Neurology Department, Ruber Internacional Hospital, Madrid, Spain
- 3Global Public Health, Jeffrey Cheah School of Medicine and Health Sciences, Monash University Malaysia, Subang Jaya, Malaysia
- 4Neurology Unit, Department of Medicine, Universiti Kebangsaan Malaysia Medical Centre, Kuala Lumpur, Malaysia
- 5School of Pharmacy, Monash University Malaysia, Subang Jaya, Malaysia
Background: Post-traumatic epilepsy (PTE) is a devastating neurological outcome of traumatic brain injury (TBI), which may negatively impact the quality of life of patients with TBI, and may impose a huge socioeconomic burden. This burden may be due to long-term functional outcomes associated with PTE, particularly cognitive dysfunction. To date, the relationship between TBI and PTE remains unclear, with little known about how the effect of their link on cognitive function as well.
Objective: Thus, this systematic review aimed at elucidating the relationship between PTE and cognitive impairment in adults after TBI based on available clinical studies, in hopes to aid in the development of therapeutic strategies for PTE.
Methods: A systematic literature search was performed using 6 databases; MEDLINE, Embase, CINAHL, Psych INFO, Web of Science, and Cochrane to retrieve relevant clinical studies investigating the link between PTE and cognition in the context of TBI. The Newcastle-Ottawa Scale (NOS) was used to assess the methodological quality of relevant studies.
Results: A total of six eligible studies were included for critical appraisal in this review after performing the inclusion and exclusion criteria, which involved 1,100 individuals, from 1996 to 2021. The selected studies were derived from the civilian and military population, with a follow-up period that ranged from 6 months to 35 years. The average quality of the involved studies was moderate (6.6, SD = 1.89). Five out of six studies found poorer cognitive performance in people with PTE, compared with those without PTE. Although the association between PTE and cognitive impairment was insignificant after controlling for specific covariates, there was a statistical trend toward significance.
Conclusion: This systematic review suggests that there may be a possible link between PTE and cognitive decline in TBI patients, with the latter being reported to occur up to 35 years post injury. Variations in sample sizes, follow-up periods, and neuropsychological assessment tools may be the limitations affecting the interpretation and significance of this relationship. Therefore, future studies with standard cognitive assessment tools may be warranted to solidify the link between TBI-PTE-cognitive dysfunction, prior to the development of therapeutic strategies.
Systematic Review Registration: https://www.crd.york.ac.uk/prospero/display_record.php?ID=CRD42020221702, prospero identifier: CRD42020221702.
Introduction
Traumatic brain injury (TBI) is among the leading causes of death and disability worldwide, which imposes a huge socioeconomic burden (1), with at least 10 million new cases reported each year (2, 3). TBI may be the precursor to a wide range of neurological disorders, with one of the most significantly devastating sequelae being post-traumatic epilepsy (PTE) (4–6). PTE refers to the epilepsy with unprovoked seizures that develops more than a week after TBI (7). Although the frequency may not be well known, the estimated PTE prevalence rate ranges from 1.9 to 53.3% (8, 9), depending on the age, TBI severity, and prior comorbidities. Annegers et al. (10) had earlier showed that PTE incidence ratio was only at 1.5 after mild injuries, but increased to 2.9 in moderate TBI and 17.0 in severe TBI (10), showcasing that patients with severe TBI were at a greater risk toward PTE. Moreover, a more recent study showed that veterans with penetrating TBI were at least 18 times more strongly associated with PTE development than other forms of TBI (11). PTE was deemed as the most common type of acquired epilepsy among young people, and may be closely linked to poorer functional outcomes and increased mortality (8, 9). The occurrence of PTE may impose a significant burden on patients and caregivers, mainly because of its treatment complexity (12).
Both the TBI and epilepsy elicit a wide variety of long-term consequences, independently, from physical disabilities to cognitive, emotional, and behavioral deficits, resulting in family disruptions, social restrictions, lack of earning capacity, high lifelong costs, and poor quality of life (13–15). Among them, the cognitive dysfunction may be the most well documented (16, 17). Intellectual ability, attention/concentration, processing speed, language, memory, and visual-spatial abilities are all the different domains encompassed in the term cognitive functioning (18). Thus, the functionality of these domains may be worsen in PTE, given the dual impact of the disease.
Moreover, several preclinical studies have suggested this relationship between PTE and further aggravation of cognitive impairment post-TBI. Research on mice subjected to TBI followed by the induction of seizures using electroconvulsive shock presented a worsen Barnes maze performance (learning and memory) than those with TBI only (19). In contrast, a study by Shultz et al. (20) that compared rats with or without PTE development 6 months post injury, showed no significant differences in the cognitive parameters measured by the Morris water maze. This evidence in the preclinical literature suggests that the impact of PTE on cognition may be inconclusive to date, which could be attributed to the inconsistency or variability between the PTE animal-models (spontaneous vs. induced). Hence, clinical studies on spontaneous PTE may provide better understanding on the impact of PTE on the cognition and neuropsychological performance post-TBI.
To date, there has been no systematic review to elucidate the relationship between PTE and cognitive dysfunction in the clinical context, despite the relationship of TBI and PTE or cognition being well-researched previously. Thus, this study aimed to investigate the association between PTE and cognitive dysfunction among the post-TBI population, by critical reviewing the recent publications in the clinical literature. This review may help to enlighten better therapeutic strategies to relieve cognitive dysfunction among patients with PTE and TBI, thereby improving the quality of lives of the patients, their families and caregivers.
Methodology
Protocol Registration
The review was conducted following the Preferred Reporting Items for Systematic Reviews and Meta-Analyses (PRISMA) 2020 guidelines (21) and was registered in the PROSPERO database (Registration number CRD42020221702), available at https://www.crd.york.ac.uk/prospero/display_record.php?ID=CRD42020221702.
Literature Search and Selection
The literature search was conducted using six databases: Web of Science, MEDLINE, CINAHL, EMBASE, Cochrane, and PsycINFO, with articles retrieved up to March 15, 2021. The search terms utilized encompassed variations of the word post-traumatic epilepsy, traumatic brain injury, and cognitive impairment, as detailed in Supplementary Material 1. Besides that, snowballing method from the cited references was used to identify any relevant papers.
The literature selection, which was based on the PRISMA guidelines and set inclusion and exclusion criteria, was performed by two independent researchers. A third researcher was consulted to address any potential disagreements. The inclusion criteria were: (1) studies which included adult participants/population, (2) original research published in peer-reviewed journals, (3) primary studies with the following study designs: interventional and observational studies, prospective cohorts, cross-sectional and case-control design, and (4) studies confirming the presence of PTE, performed a cognitive performance assessment and reported details on the link/relationship between PTE and cognitive function, with no restriction set on the baseline cognitive function. The exclusion criteria were: (1) non-English language articles; (2) non-original research articles; (3) articles that did not investigate PTE in the context of cognitive function; and (4) studies with inadequate information to critically evaluate the strength of the results/findings.
Data Extraction and Synthesis of Results
The study design, location of study conducted, first author and publication year, study population characteristics, assessment of PTE (seizure frequency, duration and type) and cognition, statistical analysis utilized, and significant findings pertaining to cognition in the context of PTE were extracted from each of the selected articles included in this review. It was not possible to pool the findings for a meta-analysis due to significant gaps in the measurements of cognitive function performed in the selected studies. Thus, the findings were reported qualitatively and stratified based on the principal means of cognitive assessment utilized in the studies.
Quality Assessment of Studies
The Newcastle-Ottawa Quality Assessment Scale (NOS) was used to perform the risk of bias assessment (22). A maximum of 4 points was awarded to any study in the selection section if: (i) the study represented the PTE population; (ii) the non-PTE participants were from the TBI population; (iii) PTE was identified through a record of medical evidence; and (iv) the cognitive assessment was performed at the beginning of the study (3 points if performed within the outcome) (23). Two additional points were awarded for the comparability aspect (in this review, if the TBI severity were controlled), where an extra 1 point will be awarded for consideration of other confounders. Thus, on a scale of 0–9 points, a study may be rated as having high (7–9 points), medium (4–6 points), and low quality (0–3 points) based on the points awarded (Effect of Tobacco Smoking on the Risk of Developing Community Acquired Pneumonia: A Systematic Review and Meta-Analysis, 2019). Supplementary Material 2 contains the quality assessment of selected articles included in this review.
Results
Study Selection
The initial search and retrieval of articles yielded 361 articles. Twelve articles were removed for duplication and 301 articles were excluded during title and abstract screening for non-original research articles and irrelevant studies (articles that do not fit the aim of the studies and were non-clinical studies). Out of remaining 48 articles, 44 studies, that appeared to meet the inclusion criteria, were excluded for several reasons: (1) population studied was not exclusively PTE (24, 25), (2) no ascertainment of PTE where the neuropsychological outcome could only be correlated to head injury (26, 27), and (3) no statement on the association of PTE with cognition (28, 29). Therefore, the final number of eligible and relevant full-text articles included in this systematic review for critical appraisal was four clinical studies.
As for articles retrieved through the snowballing technique, only two studies were finally included out of 12 articles identified. Thus, this brought the total number of studies included in this review to six articles (Figure 1).
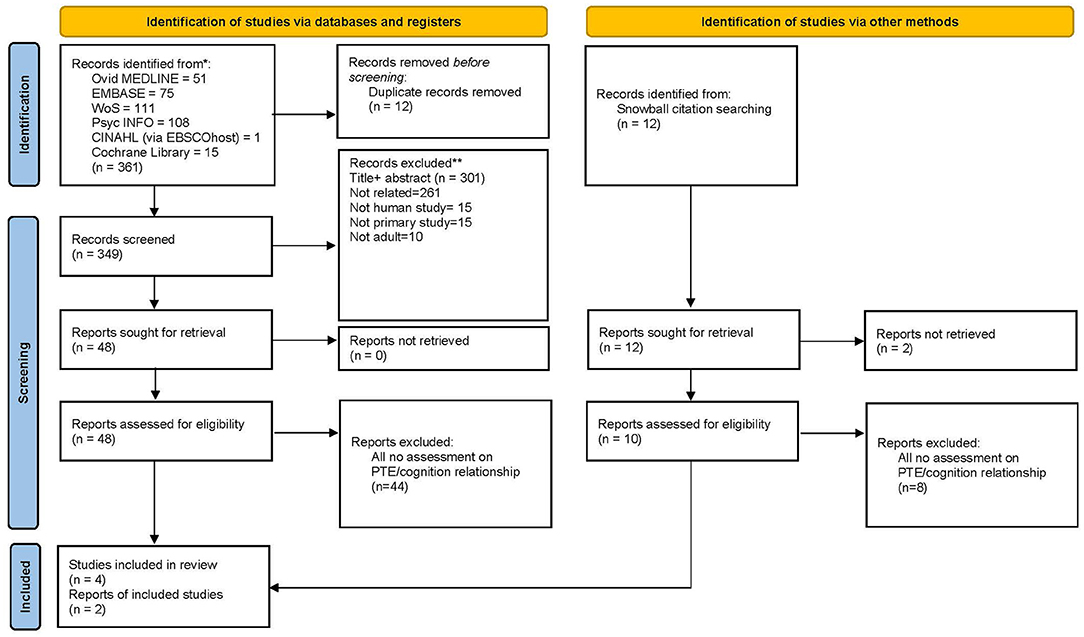
Figure 1. PRISMA 2020 flow diagram for new systematic reviews which included searches of databases and other sources used in this study.
Study Characteristics
This review selected six studies to be critically appraised, which range from 1996 to 2021. These studies were predominantly from the United States (30–32), with only 2 from Italy (33, 34). Only one study was on veterans from the Vietnam War (35). All studies were observational, a mixture of prospective and retrospective studies, with 1,100 individuals in cumulative total of participants from all six studies. The age ranges of these participants were 11–79 years old, with a higher proportion of male subjects (80.1%) in comparison with female subject represented in these studies (30–34). The follow-up period ranged from 6 months to 35 years. In three of the studies, the participants were recruited before the development of PTE (31, 33, 35), but had an incidence rate of 11.9 (31), 19 (33) and 43.7% (35), respectively. These six studies assessed cognition in three ways: by using a neuropsychological psychometric tests (31, 33), by assessing global/general cognitive ability (31, 35), and by using a cognitive rating scale (30, 32, 34). The characteristics of the reviewed studies are summarized in Table 1.
Quality Assessment
Based on the NOS assessment tool, three studies were rated as high quality (31, 33, 35), while the other three studies were classified as medium quality (30, 32, 34). All studies had good representation of the interested population (individuals with PTE) and a clear assessment on the outcome of cognitive impairment. All studies stated that the cognitive impairment was not present prior to TBI in their subject cohort. These studies have mentioned that people with a history of neurological diseases or prior cognitive dysfunction were excluded. Four studies (66.67%) confirmed the presence of PTE through the clinical diagnosis or electroencephalogram (EEG) and five studies (83.33%) performed long-term follow-up investigations which allowed the development of neuropsychological/cognitive deficits. Four out of the six selected studies (66.67%) had an adequate follow-up of cohorts with not more than 20% withdrawal during the follow-up period.
The main study design characteristics which impacted the quality scores were the lack of selection of non-exposed cohort, as two studies did not include a control group of TBI subjects without PTE (32, 34). Another characteristic that impacted the quality of the selected articles was the lack of information/assessment on confounders, where three studies did not control for the effects of head injury severity (30, 32, 35).
Assessment of PTE
Majority of the selected studies diagnosed PTE via clinical observation of involuntary movements, alterations in consciousness, or abnormal motor, or sensory function by experienced clinicians or neurologists (31, 33, 34), while some diagnosed PTE using patient-reported seizure activity through a semi-structured interview (35). The EEG monitoring was used as an adjunct diagnosis tool in some studies (31, 33).
Higher incidences of PTE were seen in the Mazzini et al. (33) study with 19% compared with the 11.9% seen in the Haltiner et al. (31) study, both at 1-year follow-up. Aligned with the other TBI study findings (4, 5, 9, 36, 37), the prevalence of PTE in both these studies were associated with the increasing injury severity and the presence and increasing frequency of neurosurgically treated subdural hematoma or intracerebral hematoma. However, all subjects in both studies had existing seizure risk factors upon enrolment. Interestingly, PTE was seen significantly more correlated with hypoperfusion in the temporal lobes, compared to those without seizures (p < 0.004), with lower Glasgow Coma Scale (GCS) on admission (31) or with longer coma duration (p < 0.007) (33).
The limitations presented in these studies in terms of PTE assessment were the knowledge transfer of previous assessment of seizures from another larger study (32, 35), and conducting follow-up seizure assessment based on the self-report method (without witness or medical report) (30, 32).
Assessment of Cognitive Impairment
We have grouped the cognitive results from the selected studies into three main assessment methods that were utilized: neuropsychological testing, global cognitive ability, and rating scale, as depicted in Table 2.
Neuropsychological Testing
Two studies which scored high methodological quality based on the NOS tool, measured cognitive performance using the established neuropsychological testing (31, 33) (Table 2). Haltiner et al. (31) used the Halstead-Reitan Neuropsychological Test Battery to determine the cognitive function of memory, motor, attention, concentration, mental flexibility, speed, problem-solving, and reasoning ability. Similarly, a study by Mazzini et al. (33) used a list of neuropsychological evaluations to assess memory, intelligence, attention, language, spatial cognition, and the perception domains.
In a study by Haltiner et al. (31), individuals with single late post-traumatic seizure (LPTS) performed slightly worse than those with no late post-traumatic seizure (NLPTS). The Haltiner et al. (31) study indicated a more significant cognitive impairment in PTE groups than the NLPTS and LPTS groups on nearly all neuropsychological measurements (p < 0.001), except for memory. However, when adjusted for injury severity factors, time to follow commands, CT-scan evidence of hematoma, neurosurgery procedure, and shunt placement, the significant differences in the neuropsychological assessments were no longer seen. This was also true when the demographics factors, such as age and education level, were adjusted. Similarly, the result of the psychometric analysis in the Mazzini et al. (33) study was skewed to suggest worsened cognitive functioning in the PTE group. Nonetheless, no significant difference in the cognition was observed between the PTE and non-PTE groups at 1 year after trauma.
Global Cognitive Ability Scale
Two high-quality studies examined cognition using global cognitive ability scales on participants, as shown in Table 2 (31, 35). Both studies reported using the Wechsler Adult Intelligence Scale (WAIS), a primary clinical instrument used to measure intelligence. Additionally, Raymont et al. (35), the only study performed on the military population, reported differences in the Armed Forces Qualification Test (38) (AFQT) results in the phase 2 (PH2) (15 years post injury) and phase 3 (PH3) (30–35 years post injury) timepoints.
The WAIS test in Haltiner et al. (31) study showed that when the TBI severity was taken into account, there were no longer significant differences in the verbal and IQ performances seen at 1-year post injury (p = 0.19). On the other hand, Raymont et al. (35) reported a highly significant difference in the WAIS mean score between the PTE and non-PTE groups at 30–35 years post-TBI (p = 0.000).
In the AFQT test reported by Raymont et al. (35), the PTE group showed a significant decline in cognition (p = 0.000) in their PH2 and PH3 scores than the non-PTE. More frequent seizures were associated with lower cognitive scores and a significant decline in intelligence (F = 5.876, df = 6, p < 0.001). Besides, a significant association between the type of last seizure and PH3 score (F = 6.010, df = 5, p = 0.000) and change in pre-TBI to PH3 scores were also reported (F = 4.140, df = 6, p = 0.000). Those with focal seizures with secondary generalization had the lowest PH3 scores and the most severe cognitive declined from pre-TBI to PH3. In contrast, those having focal seizures without impaired awareness had the highest scores at PH3 and the most negligible intelligence decline. In addition, those with parietal lesions (p = 0.001) and left insula involvement (p = 0.002) were more likely to report a history of seizures. The number of lobes involved was correlated with the occurrence of the complex partial seizure (r = 0.196, p = 0.006) post injury.
Cognitive by Uncommon Rating Scales
Three medium-quality studies reported the cognitive performance of PTE participants using less common rating scales, as in Table 2 (30, 32, 34). Bushnik et al. (30) followed 91 matched pairs of PTE and non-PTE groups at 1, 2, and 5 years post-TBI. Up to 5 years, there were no significant differences in injury severity and productivity outcomes, showing comparable cognitive improvement from the time of admission to discharge from rehabilitation. However, the Functional Independence Measure (FIM) Cognitive subscale scores showed a significant effect of seizure; the PTE group had lower cognitive scores at all 3-time points than non-PTE (F = 6.780; p < 0.01).
As for Pingue et al. (34), who retrospectively examined the clinical data of 341 adults for at least 6 months post injury, showed that at the end of the inpatient rehabilitation, the presence of seizures was linked to a lower GCS score (p < 0.05) and FIM (p < 0.01). This study had 11.9% mild TBI, 22.2% moderate, and 65.9% severe cases, with a majority (61.5%) of them having multiple site lesions, with the frontal (17.2%) and temporal lobes (13.2%) being the most affected. Early post-traumatic seizure (EPTS) was documented in 7% of cases, LPTS in 9.4%, and both EPTS and LPTS in 3% of cases. The LPTS group had a considerably higher risk of poorer neurological (p < 0.0001) and functional outcomes (p < 0.05) than EPTS group, where the risk was not significant.
Another study by Kolakowsky-Hayner et al. (32) has reported the cognitive performance scale of 25 individuals using the Craig Handicap Assessment Reporting Technique-Short Form (CHART-SF). The TBI cases in this study consisted of 16% (n = 4) mild, 32% (n = 8) moderate, 44% (n = 11) severe, while 8% (n = 2) were of unknown severity (sedated). Despite 28% of individuals having or had seizures within the last year, most experienced multiple seizures per year (range 4 to seizure events), but their mean cognitive score was still at 74.84 (SD = 25.87), which indicated no major impairment.
Discussion
This review showed that the current literature on PTE's impact on the cognitive function among patients with TBI may still be inconclusive, with some studies indicating a strong relationship between PTE and cognitive impairment, while others showcasing no significant differences in cognitive function between PTE and non-PTE groups, even after accounting for possible confounders, such as age within each study. One of the major factors that may have led to the inconclusive evidence between PTE and cognition, may be the unstandardized neuropsychological assessment of cognition as well as the non-uniformity of patient demographics utilized between the clinical studies. Nevertheless, studies, which showed that patients with PTE recorded significant cognitive impairments, such as decline in intelligence, suggested that the TBI severity, time since PTE, PTS phase, and frequency of seizures may all contribute to the severity of the cognitive impairment in patients with PTE.
Most clinical studies in the current literature were mainly conducted to determine the association of TBI severity with cognitive deficits, as found in a literature review by McInnes et al. (39), but the similar cognitive association with PTE may still be fairly scarce. Given the pathological dynamics of PTE may differ from TBI only cases and may lead to worsen quality of life, future studies should also focus on understanding the functional outcomes in patients with PTE to determine possible therapeutic strategies for them.
Although some studies suggested a potential link between PTE and cognitive impairment (31, 33), but when the severity of the TBI was controlled, no significant differences in cognitive performance was observed between the PTE and non-PTE groups. This suggest that any cognitive impairment witnessed in these studies may be solely catered to the impact of TBI itself and not PTE. In fact, most of the selected studies had patients who were majorly diagnosed with severe TBI, suggesting that the cognitive decline witnessed may be driven by the severity of the TBI rather than the development of PTE. However, it should be noted that PTE development were more strongly evident in those with more severe TBI, whereby worsen GCS score was recorded for groups that developed PTE compared with groups that were labeled with only one late seizure episode (LPTS) or no seizure at all (NLPTS), and cognitive performance between them was shown to be similar. The higher prevalence of PTE in severe TBI was supported by the evidence in the past literature (9, 10). This suggest that the interplay between injury severity and presence of seizure may not govern the cognition any differently as their independent influence on cognition. Although, one study suggested that at 1 year post injury, this interplay may be more evident, suggesting that the long-term impact of seizures on the neuronal activity and damage, in collaboration with the initial neuronal damage post-TBI may surface greater cognitive deficits and neuropsychological morbidity. However, future studies may be required to support this theory.
Particularly since these selected studies may have their limitations which may have confounded their results. One of the limitation was the very low incidence rate of PTE in some studies, Haltiner et al. (31) reported only 11.9% of PTE incidence among patients with TBI while Mazzini et al. (33) reported a slightly higher incidence rate of 19%, both of which may be too low and may have impeded the statistical significance of the neuropsychological findings. In addition, the neuropsychological tests performed in these studies were skewed, whereby in Haltiner et al. (31) study, only 44% of patients with PTE were tested compared with the larger fraction of patients with NLPTS and LPTS who were tested on the same neuropsychological evaluation, leading to missing outcome measures and possible bias interpretations. Another limitation was the low and varied occurrence of status epilepticus (SE) or refractory epilepsy at the 1-year follow-up post-TBI; null in Haltiner et al. (31) and n = 3 in Mazzini et al. (33), which were often associated with worsen functional outcomes (40). It is essential to evaluate SE and refractory epilepsy in the context of PTE since they may lead to significant neurological deterioration (41, 42). In some studies, PTE was assessed based on patient's self-reportation of seizures (30, 32), which may result in incidences of under-reporting or inaccurate/bias reporting as well. While the wide age range of patient recruitment in Mazzini's study may serve as another limitation/confounding factor, the study performed a multivariate analysis which minimized or negated the effects of age on the neurobehavioral and functional outcomes (33). Additionally, a study with a short duration of less than a year (34) may impact result interpretation, since PTE and cognitive impairment usually occurs more chronically post-TBI and the time of development may vary between patients.
Thus, a chronic studies may be more relevant, such as that performed by Raymont et al. (35), which reported a high prevalence of PTE (43.7%) in those who had sustained a TBI for over 30–35 years. In support, Bushnik et al. (30) found significantly lower cognitive scores in the LPTS individuals compared to patients with TBI alone, while Pingue et al. (34) found that LPTS individuals had worsen cognitive subscale scores than those with EPTS at the end of the 6-month inpatient rehabilitation, suggesting that long-term seizures were more detrimental to cognitive function that those with early seizure onset post-TBI. However, studies on chronic stages post-TBI may need to account for the possible effects of aging on cognition that may influence PTE's relationship with cognitive impairment (43).
Interestingly, Raymont and his colleagues concluded that PTE may be predictive of current intelligence, with a decline in IQ scores seen in military patients with PTE (35). It was worth noting that the chronic follow-up time showed that patients with increasing episodes of PTE annually (i.e., frequency of 2–10 seizures per year) were associated with a higher decline in a full-scale IQ test and intelligence (35). Furthermore, individuals with focal seizures evolving to bilateral tonic-clonic seizures had the most significant cognitive decline, suggesting the influence of type and severity of seizures on cognitive functionality.
The pattern of neurocognitive deficits may have originated from the frontal or temporal lobes, which were common place of development of seizure foci in patients with PTE (16, 44, 45). Due to the neuroanatomical location, the frontal lobes (specifically the orbitofrontal region) and temporal lobes may be particularly vulnerable to TBI lesions as well (46, 47). As clinically evidenced in this review (31, 33, 34), most PTE patients with frontal and temporal lesions reported having experienced impairment in memory, language, attention, processing speed, and executive functions (mental flexibility, problem-solving and reasoning ability) (47, 48). These findings suggest that targeting the pathology within the frontal and temporal lobes post-TBI, may prevent the PTE development and subsequent cognitive decline. The pathology of PTE's impact on cognition may also extend or propagate to other areas of the brain, such as parietal lobe (35), depending on the type of TBI/injury lesions.
Instead of investigating the pathology in the brain lesions areas, future studies should also focus on determining the causal and pathophysiological relationship of PTE and cognitive impairment via blood or biomarkers and neuroimaging techniques. There have been some robust biomarkers of epilepsy that were suggested to correlate with the pathological progression of cognition (49, 50). For example, the blood-based biomarkers (inflammatory markers), such as interleukin-6 (IL-6) and high mobility group box 1 (HMGB1) have been shown to play a role in epilepsy progression and cognitive impairment mechanism (51–53). However, these epilepsy biomarkers may still be in the early phase of the research curve (54–56). There may still be insufficient understanding on the multidimensional cascades of TBI leading to the current ineffective treatment and prevention strategies of post-TBI functional outcomes (57).
Some other strategies to lessen the impact of PTE on cognition may include controlling the severity and frequency of seizures post-TBI. Optimal seizure control may have a positive impact on the cognitive function and quality of life of patients with PTE (15, 32, 58). Consequently, cognitive screening in new-onset epilepsies post-TBI should become a standard practice in post-injury care to provide effective therapies for early seizure control, thereby preventing or slowing the cognitive decline in later years. Information about the patient's cognitive state at the onset of epilepsy and before the initiation of treatment may enable healthcare providers to assess the disease evolution and associate the possible changes to either the effectiveness of treatment, the side effects of antiseizure medications, and the dynamics of the underlying disease post injury. Both the early detection of cognitive dysfunction and optimal seizure control may have a positive impact on a patient's social function, and may reduce caregivers burden and holistically, thereby improving quality of life of patients and caregivers alike (12, 16, 59). Follow-up assessments of new-onset epilepsies post-TBI, as well as those on epilepsy treatment in future studies, may yield more prominent findings. Besides that, more assessment indicators of cognitive comorbidity should be performed in future studies, since the spectrum of cognition may be too broad to be captured by just a single type of cognitive or neuropsychological assessment (60). Furthermore, the epileptogenesis of PTE takes months to years to manifest and only in some patients with TBI, hence increasing the number of chronic investigation studies will be more beneficial to clearly witness the adverse effects of PTE, particularly on cognition.
Conclusion
This review found that the association between PTE and cognitive function among patients with TBI may be inconclusive to date, due to scarcity of the literature and significant limitations in the current literature, particularly in the non-uniformity and variations in the study designs, neuropsychological performance evaluation methods, and follow-up periods. People with TBI and PTE were reported to face the double-barreled disadvantages on cognition, which was evident up to 35 years of post injury. Therefore, more explicit treatment strategies for optimal seizure control and more transparent assessment guidelines may be required for individuals affected by PTE to manage and respond to these ongoing cognitive challenges. Further investigations may be required to narrow the knowledge gap in the etiology and pathology of neuropsychological decline in PTE individuals to provide the most effective therapeutic strategy, which may help patients with PTE to lead a good quality of life.
Data Availability Statement
The original contributions presented in the study are included in the article/Supplementary Material, further inquiries can be directed to the corresponding author/s.
Author Contributions
IN: conceptualization, data collection, investigation, literature retrieval, and writing—original draft. MS: conceptualization and supervision. AA-S: investigation and writing—review and editing. AA, CK, and WC: writing—review and editing. DM: supervision and writing—review and editing. All authors contributed to the article and approved the submitted version.
Funding
The authors were supported by Monash University Malaysia-School of Medicine Strategic Grant 2021.
Conflict of Interest
The authors declare that the research was conducted in the absence of any commercial or financial relationships that could be construed as a potential conflict of interest.
Publisher's Note
All claims expressed in this article are solely those of the authors and do not necessarily represent those of their affiliated organizations, or those of the publisher, the editors and the reviewers. Any product that may be evaluated in this article, or claim that may be made by its manufacturer, is not guaranteed or endorsed by the publisher.
Acknowledgments
We would like to thank Ms Julia Khaw; MA of Clinical Psychology; MSc Neuropsychology from Department of Psychiatry, Faculty of Health Sciences, The National University of Malaysia, Kuala Lumpur for assisting us in identifying the relevant cognitive tests in the articles.
Supplementary Material
The Supplementary Material for this article can be found online at: https://www.frontiersin.org/articles/10.3389/fneur.2022.827571/full#supplementary-material
References
1. World Health Organization. Epilepsy. (2019). Available online at: https://www.who.int/news-room/fact-sheets/detail/epilepsy (accessed July 25, 2021).
2. Beghi E, Giussani G, Abd-Allah F, Abdela J, Abdelalim A, Abraha HN, et al. Global, regional, and national burden of epilepsy, 1990–2016: a systematic analysis for the Global Burden of Disease Study 2016. Lancet Neurol. (2019) 18:357–75. doi: 10.1016/S1474-4422(18)30454-X
3. Roozenbeek B, Maas AIR, Menon DK. Changing patterns in the epidemiology of traumatic brain injury. Nat Rev Neurol. (2013) 9:231–6. doi: 10.1038/nrneurol.2013.22
4. Ferguson PL, Smith GM, Wannamaker BB, Thurman DJ, Pickelsimer EE, Selassie AW, et al. population-based study of risk of epilepsy after hospitalization for traumatic brain injury. Epilepsia. (2010) 51:891–8. doi: 10.1111/j.1528-1167.2009.02384.x
5. Xu T, Yu X, Ou S, Liu X, Yuan J, Huang H, et al. Risk factors for posttraumatic epilepsy: a systematic review and meta-analysis. Epil Behav. (2017) 67:1–6. doi: 10.1016/j.yebeh.2016.10.026
6. Yeh CC, Chen TL, Hu CJ, Chiu WT, Liao CC. Risk of epilepsy after traumatic brain injury: a retrospective population-based cohort study. J Neurol Neurosurg Psychiatry. (2013) 84:441–5. doi: 10.1136/jnnp-2012-302547
7. Fisher RS, Acevedo C, Arzimanoglou A, Bogacz A, Cross JH, Elger CE, et al. ILAE official report: a practical clinical definition of epilepsy. Epilepsia. (2014) 55:475–82. doi: 10.1111/epi.12550
8. Christensen J, Pedersen MG, Pedersen CB, Sidenius P, Olsen J, Vestergaard M. Long-term risk of epilepsy after traumatic brain injury in children and young adults: a population-based cohort study. Lancet. (2009) 373:1105–10. doi: 10.1016/S0140-6736(09)60214-2
9. Siig Hausted H, Nielsen JF, Odgaard L. Epilepsy after severe traumatic brain injury: frequency and injury severity. Brain Inj. (2020) 34:889–94. doi: 10.1080/02699052.2020.1763467
10. Annegers JF, Hauser WA, Coan SP, Rocca WA. A population-based study of seizures after traumatic brain injuries. N Engl J Med. (1998) 338:20–4. doi: 10.1056/NEJM199801013380104
11. Pugh MJ, Orman JA, Jaramillo CA, Salinsky MC, Eapen BC, Towne AR, et al. The prevalence of epilepsy and association with traumatic brain injury in veterans of the Afghanistan and Iraq wars. J Head Trauma Rehabil. (2015) 30:29–37. doi: 10.1097/HTR.0000000000000045
12. Jabbarinejad R, Cohen-Zimerman S, Wagner AK, Grafman J. Determinants of caregiver burden in male patients with epilepsy following penetrating traumatic brain injury. Epilepsy and Behavior. (2021) 116:107768. doi: 10.1016/j.yebeh.2021.107768
13. Kiraly MA, Kiraly SJ. Traumatic brain injury and delayed sequelae: a review - traumatic brain injury and mild traumatic brain injury (concussion) are precursors to later-onset brain disorders, including early-onset dementia. The Scientific World Journal. (2007) 7:1768–76. doi: 10.1100/tsw.2007.269
14. Pavlovic D, Pekic S, Stojanovic M, Popovic V. Traumatic brain injury: Neuropathological, neurocognitive and neurobehavioral sequelae. Pituitary. (2019) 22:270–82. doi: 10.1007/s11102-019-00957-9
15. Takada K, Sashika H, Wakabayashi H, Hirayasu Y. Social participation and quality-of-life of patients with traumatic brain injury living in the community: a mixed methods study. Brain Injury. (2016) 30:1590–8. doi: 10.1080/02699052.2016.1199901
16. Arciniegas DB, Held K, Wagner P. Cognitive impairment following traumatic brain injury. Curr Treat Options Neurol. (2002) 4:43–57. doi: 10.1007/s11940-002-0004-6
17. Rabinowitz AR, Levin HS. Cognitive sequelae of traumatic brain injury. Psychiatr Clin North Am. (2014) 37:1–11. doi: 10.1016/j.psc.2013.11.004
18. Sun H, Luo C, Chen X, Tao L. Assessment of cognitive dysfunction in traumatic brain injury patients: a review. Forensic Sci Res. (2017) 2:174–9. doi: 10.1080/20961790.2017.1390836
19. Chrzaszcz M, Venkatesan C, Dragisic T, Watterson DM, Wainwright MS. Minozac treatment prevents increased seizure susceptibility in a mouse “two-hit” model of closed skull traumatic brain injury and electroconvulsive shock-induced seizures. J Neurotrauma. (2010) 27:1283–95. doi: 10.1089/neu.2009.1227
20. Shultz SR, Cardamone L, Liu YR, Edward Hogan R, MacCotta L, Wright DK, et al. Can structural or functional changes following traumatic brain injury in the rat predict epileptic outcome? Epilepsia. (2013) 54:1240–50. doi: 10.1111/epi.12223
21. Page MJ, McKenzie JE, Bossuyt PM, Boutron I, Hoffmann TC, Mulrow CD, et al. The PRISMA 2020 statement: an updated guideline for reporting systematic reviews. BMJ. (2021) 372. doi: 10.1136/bmj.n71
22. Wells G, Shea B, O'Connell D, Peterson J, Welch V, Losos M, et al. The Newcastle–Ottawa Scale (NOS) for assessing the quality of non-randomized studies in meta-analysis. Appl Eng Agric. (2012) 18:727–34. Available online at: https://pdfs.semanticscholar.org/5b63/3ae0fca9ba9ddb97b4c35b8c3b264fd5104a.pdf
23. Ka-Lok Lo C, Mertz D, Loeb M. Newcastle-Ottawa Scale: Comparing reviewers' to authors' assessments. BMC Med Res Methodol. (2014) 14:45. doi: 10.1186/1471-2288-14-45
24. Binder LM, Kindermann SS, Heaton RK, Salinsky MC. Neuropsychologic impairment in patients with nonepileptic seizures. Arch Clin Neuropsychol. (1998) 13:513–22. doi: 10.1093/arclin/13.6.513
25. Cragar DE, Berry DTR, Fakhoury TA, Cibula JE, Schmitt FA. Performance of patients with epilepsy or psychogenic non-epileptic seizures on four measures of effort. Clin Neuropsychol. (2006) 20:552–66. doi: 10.1080/13854040590947380
26. Dikmen SS, Machamer JE, Winn HR, Temkin NR. Neuropsychological outcome at 1-year post head injury Sureyya. Neuropsychology. (1995) 9:80–90. doi: 10.1037/0894-4105.9.1.80
27. Himanen L, Portin R, Isoniemi H, Helenius H, Kurki T, Tenovuo O. Cognitive functions in relation to MRI findings 30 years after traumatic brain injury. Brain Injury. (2005) 19:93–100. doi: 10.1080/02699050410001720031
28. Plancher KD, Brooks-James A, Nissen CW, Diduch BK, Petterson SC. Baseline neurocognitive performance in professional lacrosse athletes. Orthopaed J Sports Med. (2014) 2. doi: 10.1177/2325967114550623
29. Scheid R, Walther K, Guthke T, Preul C, von Cramon DY. Cognitive sequelae of diffuse axonal injury. Arch Neurol. (2006) 63:418–24. doi: 10.1001/archneur.63.3.418
30. Bushnik T, Englander J, Wright J, Kolakowsky-Hayner SA. Traumatic brain injury with and without late posttraumatic seizures: What are the impacts in the post-acute phase: a NIDRR traumatic brain injury model systems study. J Head Trauma Rehabil. (2012) 27:36–44. doi: 10.1097/HTR.0b013e318273375c
31. Haltiner AM, Temkin NR, Winn HR, Dikmen SS. The impact of posttraumatic seizures on 1-year neuropsychological and psychosocial outcome of head injury. J Int Neuropsychol Soc. (1996) 2:494–504. doi: 10.1017/S1355617700001661
32. Kolakowsky-Hayner SA, Wright J, Englander J, Duong T, Ladley-O'Brien S. Impact of late PTSs on physical health and functioning for individuals with brain injury within the community. Brain Injury. (2013) 27:578–86. doi: 10.3109/02699052.2013.765595
33. Mazzini L, Cossa FM, Angelino E, Campini R, Pastore I, Monaco F. Posttraumatic epilepsy: neuroradiologic and neuropsychological assessment of long-term outcome. Epilepsia. (2003) 44:569–74. doi: 10.1046/j.1528-1157.2003.34902.x
34. Pingue V, Mele C, Nardone A. Post-traumatic seizures and antiepileptic therapy as predictors of the functional outcome in patients with traumatic brain injury. Sci Rep. (2021) 11:1–12. doi: 10.1038/s41598-021-84203-y
35. Raymont V, Salazar AM, Lipsky R, Goldman D, Tasick G, Grafman J. Correlates of posttraumatic epilepsy 35 years following combat brain injury. Neurology. (2010) 75:224–9. doi: 10.1212/WNL.0b013e3181e8e6d0
36. Annegers JF, Coan SP. The risks of epilepsy after traumatic brain injury. Seizure. (2000) 9:453–7. doi: 10.1053/seiz.2000.0458
37. Englander J, Bushnik T, Duong TT, Cifu DX, Zafonte R, Wright J, et al. Analyzing risk factors for late posttraumatic seizures: a prospective, multicenter investigation. Arch Phys Med Rehabil. (2003) 84:365–73. doi: 10.1053/apmr.2003.50022
38. Kaplan Inc. (n.d.). What is the AFQT? – Kaplan Test Prep. (2021). Available online at: https://www.kaptest.com/study/asvab/what-is-the-afqt/ (accessed July 18, 2021)
39. McInnes K, Friesen CL, MacKenzie DE, Westwood DA, Boe SG. Mild traumatic brain injury (mTBI) and chronic cognitive impairment: a scoping review. PLoS ONE. (2017) 12:e0174847. doi: 10.1371/journal.pone.0174847
40. Towne AR, Pellock JM, Ko D, DeLorenzo RJ. Determinants of mortality in status epilepticus. Epilepsia. (1994) 35:27–34. doi: 10.1111/j.1528-1157.1994.tb02908.x
41. Crawford TO, Mitchell WG, Fishman LS, Robert Snodgrass S. Very-high-dose phenobarbital for refractory status epilepticus in children. Neurology. (1988) 38:1035–40. doi: 10.1212/WNL.38.7.1035
42. Neligan A, Rajakulendran S, Walker MC. Advances in the management of generalized convulsive status epilepticus: what have we learned? Brain. (2021) 144:1336–41. doi: 10.1093/brain/awab049
43. Murman DL. The impact of age on cognition. Semin Hear. (2015) 36:111–21. doi: 10.1055/s-0035-1555115
44. Riggio S, Wong M. Neurobehavioral sequelae of traumatic brain injury. Mount Sinai J Med. (2009) 76:163–72. doi: 10.1002/msj.20097
45. Semple BD, Zamania A, Raynerc G, Shultza SR, Jonesa NC. Affective, neurocognitive and psychosocial disorders associated with traumatic brain injury and post-traumatic epilepsy. Neurobiol Dis. (2019) 123:27–41. doi: 10.1016/j.nbd.2018.07.018
46. Bigler ED. Neuropsychology and clinical neuroscience of persistent post-concussive syndrome. J Int Neuropsychol Soc. (2008) 14:1–22. doi: 10.1017/S135561770808017X
47. Phuong TH, Houot M, Méré M, Denos M, Samson S, Dupont S. Cognitive impairment in temporal lobe epilepsy: contributions of lesion, localization and lateralization. J Neurol. (2021) 268:1443–52. doi: 10.1007/s00415-020-10307-6
48. Miotto EC, Cinalli FZ, Serrao VT, Benute GG, Lucia MCS, Scaff M. Cognitive deficits in patients with mild to moderate traumatic brain injury. Arq Neuropsiquiatr. (2010) 68:862–8. doi: 10.1590/S0004-282X2010000600006
49. Dadas A, Washington J, Diaz-Arrastia R, Janigro D. Biomarkers in traumatic brain injury (TBI): a review. Neuropsychiatr Dis Treat. (2018) 14:2989–3000. doi: 10.2147/NDT.S125620
50. Morganti-Kossmann MC, Semple BD, Hellewell SC, Bye N, Ziebell JM. The complexity of neuroinflammation consequent to traumatic brain injury: from research evidence to potential treatments. Acta Neuropathol. (2019) 137:731–55. doi: 10.1007/s00401-018-1944-6
51. Paudel YN, Angelopoulou E, Piperi C, Balasubramaniam VR, Othman I, Shaikh MF. Enlightening the role of high mobility group box 1 (HMGB1) in inflammation: updates on receptor signalling. Eur J Pharmacol. (2019) 858:172487. doi: 10.1016/j.ejphar.2019.172487
52. Richard SA, Min W, Su Z, Xu H. High mobility group box 1 and traumatic brain injury. J Behav Brain Sci. (2017) 07:50–61. doi: 10.4236/jbbs.2017.72006
53. Webster KM, Shultz SR, Ozturk E, Dill LK, Sun M, Casillas-Espinosa P, et al. Targeting high-mobility group box protein 1 (HMGB1) in pediatric traumatic brain injury: chronic neuroinflammatory, behavioral, and epileptogenic consequences. Exp Neurol. (2019) 320:1–14. doi: 10.1016/j.expneurol.2019.112979
54. Laird MD, Shields JS, Sukumari-Ramesh S, Kimbler DE, Fessler RD, Shakir B, et al. High mobility group box protein-1 promotes cerebral edema after traumatic brain injury via activation of toll-like receptor 4. Glia. (2014) 62:26–38. doi: 10.1002/glia.22581
55. Pitkänen A, Xavier Ekolle Ndode-Ekane NL, Puhakka N. Epilepsy biomarkers-Toward etiology and pathology specificity. Neurobiol Dis. (2019) 123:42–58. doi: 10.1016/j.nbd.2018.05.007
56. Simonato M, Agoston DV, Brooks-Kayal A, Dulla C, Fureman B, Henshall DC, et al. Identification of clinically relevant biomarkers of epileptogenesis — a strategic roadmap. Nat Rev Neurol. (2021) 17:231–42. doi: 10.1038/s41582-021-00461-4
57. Okuma Y, Wake H, Teshigawara K, Takahashi Y, Hishikawa T, Yasuhara T, et al. Anti–high mobility group box 1 antibody therapy may prevent cognitive dysfunction after traumatic brain injury. World Neurosurg. (2019) 122:e864–71. doi: 10.1016/j.wneu.2018.10.164
58. Trinka E, Kwan P, Lee BI, Dash A. Epilepsy in Asia: disease burden, management barriers, and challenges. Epilepsia. (2019) 60:7–21. doi: 10.1111/epi.14458
59. Broodryk M. Traumatic Brain Injury Caregivers' Experiences: An Exploratory Study in the Western Cape. (2014).
Keywords: traumatic brain injury, head injury, cognitive decline, neuropsychological deficit, post-traumatic epilepsy
Citation: Ngadimon IW, Aledo-Serrano A, Arulsamy A, Mohan D, Khoo CS, Cheong WL and Shaikh MF (2022) An Interplay Between Post-Traumatic Epilepsy and Associated Cognitive Decline: A Systematic Review. Front. Neurol. 13:827571. doi: 10.3389/fneur.2022.827571
Received: 02 December 2021; Accepted: 26 January 2022;
Published: 24 February 2022.
Edited by:
Edoardo Ferlazzo, University of Catanzaro, ItalyReviewed by:
Ramon Diaz-Arrastia, University of Pennsylvania, United StatesSara Gasparini, University of Catanzaro, Italy
Iolanda Martino, University Magna Graecia, Italy
Copyright © 2022 Ngadimon, Aledo-Serrano, Arulsamy, Mohan, Khoo, Cheong and Shaikh. This is an open-access article distributed under the terms of the Creative Commons Attribution License (CC BY). The use, distribution or reproduction in other forums is permitted, provided the original author(s) and the copyright owner(s) are credited and that the original publication in this journal is cited, in accordance with accepted academic practice. No use, distribution or reproduction is permitted which does not comply with these terms.
*Correspondence: Mohd. Farooq Shaikh, farooq.shaikh@monash.edu