- 1 Research Service, Veterans Affairs Boston Healthcare System, Boston, MA, USA
- 2 Department of Psychiatry, Harvard Medical School, Boston, MA, USA
Several studies have reported deficits in γ oscillatory activity elicited by sensory stimulation or cognitive processes in schizophrenia patients (SZ) compared to healthy control subjects (HC). However, the evidence for cortical hyperexcitability and reduced function of N-methyl-D-aspartate receptors (NMDARs) on parvalbumin-expressing inhibitory interneurons in schizophrenia leads to the prediction that γ activity should rather be increased in SZ, but data supporting this hypothesis have been lacking. One possibility is that baseline induced γ power is increased, an effect that might have gone unnoticed in studies of stimulus-locked oscillations. Here we addressed this question by re-analyzing the data from a previously published study on the 40 Hz auditory steady-state response (ASSR) in schizophrenia in which dipole source localization was used to examine γ responses in the left and right auditory cortices. Subjects were 16 HC and 18 chronic SZ, who listened to trains of clicks presented at 40 Hz during electroencephalogram recording. Independent component analysis was used to remove ocular artifacts. Power spectra were computed for the pre-stimulus baseline period. We found that baseline power was higher in SZ than HC at 40 Hz in the left auditory cortex. Baseline 40 Hz power in the left auditory cortex was also correlated with ASSR evoked power in SZ. Thus, γ oscillation abnormalities in schizophrenia may include abnormal increases in baseline power as well as deficits in evoked oscillations. These baseline increases could be the sign of NMDAR hypofunction on parvalbumin-expressing inhibitory interneurons, which would be consistent with acute NMDAR antagonism and genetic ablation models of schizophrenia.
Introduction
Oscillatory activity in the γ band (30–100 Hz) of the electroencephalogram (EEG) has been proposed to be sensitive to cortical circuit abnormalities in schizophrenia (e.g., Kwon et al., 1999; Spencer et al., 2003; Lewis et al., 2005). Fast-spiking local circuit interneurons that express the calcium-binding protein parvalbumin (PV) show several abnormalities in schizophrenia, including reduced synthesis of γ-amino-butyric acid (GABA; Lewis et al., 2011). PV interneurons play a major role in the genesis of γ oscillations in cortical circuits, as they exert powerful, precisely timed inhibition onto their target pyramidal cells and inhibitory interneurons (Whittington and Traub, 2003; Bartos et al., 2007; Sohal et al., 2009). Thus, γ oscillations may provide a sensitive biomarker for assessing the integrity of local circuit inhibition mediated by PV interneurons in schizophrenia (Woo et al., 2010).
Reduced function of N-methyl-D-aspartate receptors (NMDARs) may be an important neural substrate of schizophrenia (Coyle et al., 2003; Krystal et al., 2003; Kantrowitz and Javitt, 2010). One of the neurophysiological consequences of acute NMDAR administration in humans and animals is increased cortical excitability (e.g., Di Lazzaro et al., 2003; Homayoun and Moghaddam, 2007), which has led to the hypothesis that NMDAR antagonists produce their schizophrenia-like effects primarily by reducing the excitation of inhibitory interneurons, leading to a disinhibition of pyramidal cells and increased excitability (Olney et al., 1991; Moghaddam et al., 1997). Consistent with this hypothesis, neuropathological studies have found evidence for decreased expression of NMDARs on inhibitory interneurons in individuals with schizophrenia (Woo et al., 2004; Bitanihirwe et al., 2009), and studies utilizing transcranial magnetic stimulation have reported signs of increased cortical excitability in schizophrenia patients (SZ; e.g., Hoffman and Cavus, 2002; Daskalakis et al., 2007). Furthermore, Heckers et al. (1998) observed increased baseline activity in the hippocampus in SZ.
The hypothesis that γ oscillations reflect the integrity of local circuit inhibition predicts that schizophrenia should be associated with γ deficits, which have in fact been found in several sensory and cognitive domains (e.g., Kwon et al., 1999; Spencer et al., 2003, 2008a; Krishnan et al., 2005; Cho et al., 2006; Ferrarelli et al., 2008; Ford et al., 2008; Leicht et al., 2010). However, the evidence for increased cortical excitability and NMDAR hypofunction in schizophrenia suggests the opposite prediction, namely that SZ should show increased γ activity relative to healthy individuals. In some studies from our laboratory we have in fact found positive correlations between psychotic symptoms such as hallucinations and particular γ measures (Spencer et al., 2004, 2008b, 2009; Mulert et al., 2011), but none of these findings involved overall increases in γ oscillations in patients.
One possibility is that evoked γ responses to stimuli may be reduced in schizophrenia as a consequence of increased baseline γ activity. Evoked responses are typically measured in reference to a pre-stimulus baseline, and differences in baseline levels could account for at least part of the difference in post-stimulus responses. Furthermore, baseline oscillatory activity is unlikely to be stimulus-locked, and therefore increased baseline activity manifested as non-stimulus locked or “induced” oscillations would not be detectable with stimulus-locked measures [evoked power or phase locking factor (PLF)] unless specifically analyzed. Evidence for such an effect of increased baseline activity on post-stimulus responses in schizophrenia has been reported for low-frequency oscillations (Winterer et al., 2000) and in functional magnetic resonance imaging (Hugdahl et al., 2009). Also, ffytche et al. (1998) observed increased baseline activity and decreased visual-evoked responses in the visual cortex of hallucinators with Charles Bonnet syndrome.
The goals of the present study were to determine whether: (1) baseline γ power was increased in SZ compared to healthy control subjects (HC); and (2) stimulus-evoked γ power and phase locking was related to pre-stimulus baseline γ power. We addressed these questions by re-analyzing data from a previously published study on the 40 Hz auditory steady-state response (ASSR) in schizophrenia (Spencer et al., 2009; cf. Mulert et al., 2011). In that study we used source localization to analyze the ASSR generators in the left and right auditory cortices. Briefly, we found that: (1) ASSR phase locking in chronic SZ was reduced in the right hemisphere (RH) compared to HC. Evoked power did not differ between groups. (2) The patients’ auditory hallucination symptoms were positively correlated with ASSR phase locking in the left hemisphere (LH). (3) LH ASSR phase locking in SZ was modulated by the phase of a delta-band oscillation, such that a SZ LH ASSR deficit occurred only for a particular range of delta phases.
One of the methodological challenges in analyzing baseline oscillatory activity is in obtaining non-stimulus locked measures of γ power from scalp EEG recordings that are artifact-free, as recent methodological studies have demonstrated that induced oscillation power is easily contaminated by various muscle and ocular artifacts (Keren et al., 2010; Shackman et al., 2010). We overcame these problems by: (1) using a paradigm in which baseline γ power effects were expected to occur at a discrete frequency, the frequency of the ASSR; (2) removing ocular artifacts with independent component analysis (ICA), which can separate artifact patterns across the frequency spectrum, unlike regression- and template-based methods; and (3) using source localization to further constrain the potential sources of oscillation effects and to restrict the possibility of contamination by artifacts.
Materials and Methods
Subjects
This study was approved by the Institutional Review Boards of the Veterans Affairs Boston Healthcare System and Harvard Medical School. After a complete description of the study to the subjects, written informed consent was obtained. All subjects were paid for their participation.
The data set consisted of 18 SZ and 16 HC. Subjects were selected without regard for ethnicity, and met our standard inclusion criteria: (1) age between 18 and 55 years; (2) right-handed; (3) no history of electroconvulsive treatment; (4) no history of neurological illness, including epilepsy; (5) no history of alcohol or drug dependence, nor abuse within the last year, nor long duration ( > 1 year) of past abuse (DSM-IV criteria); (6) no present medication for medical disorders that would have deleterious EEG, neurological, or cognitive functioning consequences; (7) verbal IQ above 75; (8) no alcohol use in the 24 h prior to testing; and (9) English as a first language. SZ were diagnosed according to DSM-IV criteria.
The SZ and HC groups were matched on gender (all male), age [HC: 44.4 ± 6.8 years; SZ: 39.8 ± 10.5 years; t(32) = 1.47, p = 0.151], parental socio-economic status (Hollingshead, 1965), and handedness (all right-handed). All SZ were taking atypical antipsychotics, and one patient was also receiving a typical antipsychotic. Antipsychotic medication dosages were converted to chlorpromazine equivalents (Stoll, 2001), which ranged from 83 to 1200 (mean 450 ± 306). The mean age of onset for SZ was 26.1 years (± 8.0). The total positive and negative symptom scores on the Scale for the Assessment of Positive Symptoms (SAPS; Andreasen, 1984) and the Scale for the Assessment of Negative Symptoms (Andreasen, 1983) were 9.0 ± 4.2 and 13.9 ± 4.9, respectively.
Stimuli and Experimental Design
Subjects were seated in a quiet room in a comfortable chair in front of a computer monitor. Stimuli consisted of one hundred and fifty 40 Hz trains of 1 ms white noise clicks (500 ms duration, 1100 ms stimulus onset asynchrony), which were presented binaurally through headphones (70 dB sound pressure level). Subjects were instructed to look at the fixation cross on the monitor and listen to the stimuli.
EEG Recording and Processing
The EEG was recorded (0.05-100 Hz, 500 Hz digitization) with a Neuroscan Synamp amplifier using sintered Ag/AgCl electrodes in an electrode cap at 60 standard scalp sites (Chatrian et al., 1985), nosetip, and left earlobe, referenced to the right earlobe, and grounded at AFz. Bipolar vertical and horizontal electro-oculograms were recorded from electrodes above and below the right eye and at the left and right outer canthi, respectively. Electrode impedances were < 10kΩ. Single-trial epochs were extracted from -250 to +772 ms relative to stimulus onset and corrected for ocular artifacts (blinks and eye movements) with ICA (Makeig et al., 1996). Independent components accounting for ocular activity were identified via visual inspection. Vertical EOG components had topographies with maximal values around the most anterior sites and inverted polarity at the nosetip. Horizontal EOG components were maximal at lateral frontal sites and reversed polarity between the hemispheres.
Next, epochs containing other artifacts were removed. The artifact criteria were: (1) > ± 90 μ V change in one time point; and (2) amplitude range within an epoch exceeding 200 μ V. These criteria were visually tested and verified. Finally, the retained artifact-free single epochs were re-referenced to the average reference. The number of epochs retained per subject was (mean ± standard deviation) 145 ± 7 for HC and 140 ± 12 for SZ.
Source Localization
Complete details can be found in Spencer et al. (2009). ASSR source localization was performed with Brain Electric Source Analysis (BESA) v5.1. The HC grand average ASSR was filtered from 13 to 100 Hz to eliminate the transient auditory evoked potentials, and the 30–550 ms segment of the resulting waveform (the period of the ASSR) was used for dipole modeling with the standard BESA 4-shell (brain, scalp, skull, and cerebrospinal fluid) spherical head model (Figure 1A). The 5-dipole model consisted of two pairs of dipoles in the superior temporal plane of each hemisphere, and a deep midline dipole accounting for residual activity (Figure 1B). The residual variance of the model was 6.7%. The HC model was used for both the HC and SZ data. When the 5-dipole HC model was applied to the SZ grand average ASSR the RV was 15.2%. This model is similar to other dipole models of auditory cortex activity (e.g., Herdman et al., 2002; Poulsen et al., 2007).
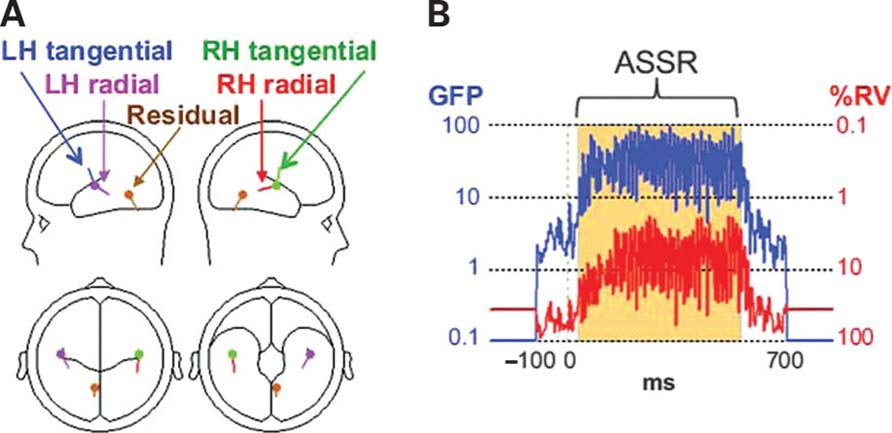
FIGURE 1. Dipole source localization was used to study baseline γ activity in schizophrenia patients (SZ) and healthy control subjects (HC). (A) Plots of the auditory steady-state response (ASSR) global field power (GFP) and the percent residual variance (RV) of the BESA dipole model during the ASSR (30–550 ms). (B) The 5-dipole model of the ASSR. The left hemisphere (LH) radial and right hemisphere (RH) tangential dipoles were the focus of this study.
The dipoles that were oriented tangentially to the lateral scalp surface (but radially to the fronto-central scalp) were termed “LH tangential” and “RH tangential”. The complementary cortical dipoles that had less tangential orientations were termed “LH radial” and “RH radial” (although their orientations were not strictly radial). The deep midline dipole was termed “Residual”.
Power Spectrum Analyses
The total spectral power of the pre-stimulus baseline period (-250 to 0 ms) was computed with the fast Fourier transform (FFT) using a 10% Hanning window. The FFT was applied to the single-trial epochs, and the single-trial power spectra were averaged to yield the baseline power spectrum (4 Hz frequency resolution). Power values were log10 transformed (Kiebel et al., 2005). Due to power line frequency (60 Hz) artifacts, the frequencies 56, 60, and 64 Hz were eliminated from analyses.
The post-stimulus ASSR evoked power and PLF measures were taken from the original study, in which those measures were computed using the Morlet wavelet transform (Tallon-Baudry et al.,1996). Evoked power and PLF were averaged within the time-frequency window of 30–550 ms and 38–50 Hz where the ASSR was maximal.
All statistical tests were two-tailed with α = 0.05. For ANOVAs, the Greenhouse–Geisser correction for inhomogeneity of variance (Keselman and Rogan, 1980) was applied for factors with more than two levels and is reflected in the reported ρ values. The non-parametric Spearman’s γ was used for correlation analyses.
Results
Previously we identified abnormalities in the RH tangential and LH radial dipoles in the SZ group, so these sources were the focus of our analyses. For the RH tangential dipole, baseline power appeared to be slightly increased in SZ compared to HC from 36 to 72 Hz (Figure 2B), but this effect was not significant [t(32) = 0.669, p = 0.509], nor did 40 Hz baseline power differ between groups [t(32) = 0.502, p = 0.619]. For the LH radial dipole, baseline power appeared to be increased in SZ compared to HC from 16 to 100 Hz (Figure 2A). This broadband increase was marginally significant [t(32) = 1.89, p = 0.069]. At 40 Hz, baseline power was significantly increased in SZ [t(32) = 2.14, < 0.05].
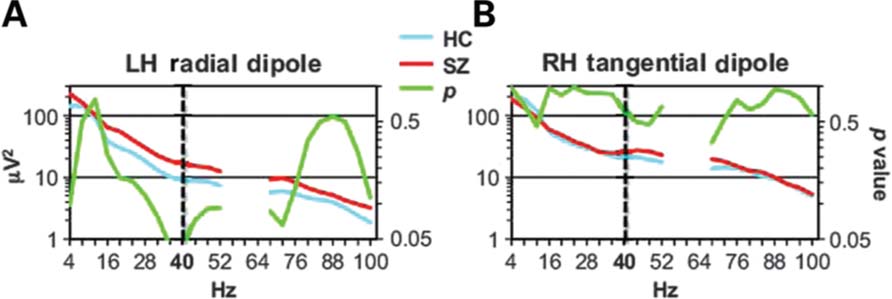
FIGURE 2. Baseline power spectra for the LH radial (A) and RH tangential (B) sources. The HC and SZ spectra are plotted, along with the p values of t-tests of the group difference at each frequency to illustrate the statistical significance of the increased SZ baseline power at 40 Hz. (Due to 60 Hz power line artifact, the frequencies 56–64 Hz were not analyzed.)
Given the evidence suggesting that increased baseline activity may be related to psychotic symptoms, particularly hallucinations, we examined correlations between LH radial dipole baseline 40 Hz power and psychotic symptom scales (Total Positive Symptoms, Global Rating of Hallucinations, and Auditory Hallucinations) of the SAPS. None of these correlations approached significance, even at uncorrected levels (ρ’s < | 0.070 |, p’s > 0.783).
To examine whether the increased LH 40 Hz baseline power in SZ developed during the course of the session, the baseline power values for the LH radial dipole were averaged for the first through fifth sets of the single trials (e.g., trials 1–30, 30–60, etc.) and analyzed in an ANOVA with the design Group × Quintile. The main effect of Group was significant [F(1,32) = 4.48, < 0.05], the main effect of Quintile was marginally significant [F(4,32) = 2.23, p = 0.089] and the Group × Quintile interaction was not significant [F(4,32) = 0.230, p = 0.878]. In exploratory post hoc tests we examined whether increased baseline power in SZ was significant in each quintile. Baseline power was significantly increased in the first and second quintiles (p’s < 0.05) and marginally significant in the fourth and fifth quintiles (p’s < 0.078), but the significant t-tests would not have survived correction for multiple comparisons.
Left hemisphere radial dipole baseline power at 40 Hz was correlated with ASSR evoked power in SZ (ρ = 0.608, p < 0.01) but not HC (ρ = 0.188, p = 0.485; Figure 3A). RH tangential dipole baseline power at 40 Hz was not correlated with ASSR evoked power in either group (HC: ρ =0.065, p = 0.812; SZ: ρ =0.116, p = 0.648; Figure 3B). ASSR PLF was not correlated with baseline power for either dipole in either group (ρ’s < | 0.314 |, p’s > 0.205). Baseline power was not correlated with age or parental socio-economic status in either subject group, nor was baseline power correlated with age of onset or medication dosage in SZ (all ρ’s < | 0.154 |, p’s > 0.583).
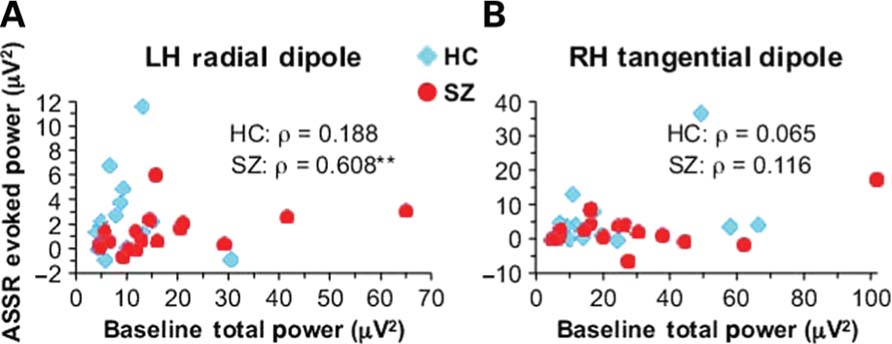
FIGURE 3. Scatterplots illustrating the correlations between 40 Hz baseline power and ASSR stimulus-evoked power in SZ for the LH radial (A) and RH tangential (B) dipoles.
Discussion
We found that pre-stimulus baseline power during auditory steady-state stimulation at 40 Hz was increased at 40 Hz in the left auditory cortex of chronicSZ relativetoHC. Baseline 40 Hz power was correlated with ASSR evoked power but not PLF in SZ. Baseline power at 40 Hz was not correlated with antipsychotic dosage, demographic measures, or positive symptoms in the SZ. While baseline power appeared to be increased in SZ across a wide frequency band (16–100 Hz), the effect was significant just at 40 Hz, the ASSR stimulation frequency. A question for future studies is whether the broadband power increase would be significant with a larger sample size.
Winterer et al. (2000) also reported an increase in non-stimulus locked oscillatory activity in schizophrenia. They found this effect at low frequencies, in the delta and theta bands. In contrast, baseline power at low frequencies was not reduced in the present study. There are several differences between the two studies that could account for these different findings, including: (1) the subjects in Winterer et al. (2000) were engaged in performing an oddball task, while the present ASSR experiment involved passive listening; and (2) auditory steady-state stimulation is designed to tap into the resonance of auditory cortex circuits in the γ band, while the oddball task is not known to engage neural circuits with specific resonant frequencies. It is an interesting question whether active task performance would change the frequency of the baseline power increase in SZ.
The finding of increased baseline γ power in SZ also adds to the growing body of evidence that schizophrenia is associated not just with deficits in γ activity, but pathological increases as well. Positive correlations between γ measures and psychotic symptoms have been reported in several of our studies (Spencer et al., 2004, 2008b, 2009; Mulert et al., 2011), and one study found increased γ phase locking in patients relative to controls during visual steady-state stimulation (Riečanský et al., 2010). However, to our surprise, we did not find any correlations between baseline γ power and psychotic symptoms, even though LH radial dipole PLF was positively correlated with auditory hallucinations in this sample (Spencer et al., 2009).
While the increase of baseline power in the present study appeared across a broad range of the EEG spectrum in SZ, it was significant just at 40 Hz, the frequency of the steady-state stimulation. This suggests that the circuits underlying the LH radial ASSR dipole continued to oscillate at the stimulation frequency even when the steady-state stimulus was not being presented, although the oscillations were no longer phase-locked to the preceding stimulus. Presumably the enhanced 40 Hz baseline activity occurred in response to multiple presentations of the steady-state stimuli, which raises the question of how this effect developed over time during the session. We found some evidence that the 40 Hz baseline power effect was present as early as the first ~30 trials of the session, but the results were not conclusive. It could be that the increase in 40 Hz baseline power occurs very rapidly and reaches ceiling within the first 30 trials.
Some other questions to be investigated are whether the increase in baseline power occurs only for stimulation in the γ band, and why an ASSR source in the LH but not the RH showed increased baseline γ. Structural and functional abnormalities of the left temporal lobe are strongly associated with auditory hallucinations, a hallmark symptom of schizophrenia (reviewed in Hugdahl et al., 2009), but few studies have examined whether auditory cortex microcircuitry abnormalities are also lateralized (e.g., Chance et al., 2008). The present data suggest that NMDAR hypofunction may be present on PV interneurons in the primary auditory cortex in the LH but not the RH in schizophrenia.
Increased baseline γ power may be due to reduced function of NMDARs on fast-spiking PV interneurons. NMDAR antagonism increases pyramidal cell activity but decreases the activity of fast-spiking interneurons (Homayoun and Moghaddam, 2007), presumably via the disinhibition of pyramidal cells by reduced NMDAR-mediated tonic excitation of fast-spiking interneurons. Increased γ due to NMDAR antagonism could be caused by the same mechanism, as suggested by a computational model of NMDAR antagonism, which showed both increased cortical excitability and increased γ activity when the NMDAR inputs to fast-spiking interneurons were reduced (Spencer, 2009). One possible explanation for these effects is that the decrease of tonic excitatory input to fast-spiking PV interneurons may increase the interneurons’ sensitivity to fast 2-amino-3-propanoic acid (AMPA) receptor-mediated excitatory input from pyramidal cells, and hence increase the synchronization of local circuits by PV interneurons in the γ frequency range. We note also that a recent study by Yizhar et al. (2011) used in vivo optogenetic methods to demonstrate that increased baseline γ power can be caused by an increase in the excitation/inhibition ratio in neurons. Such a shift in the excitation/inhibition balance could in principle also result from NMDAR antagonism.
The present finding provides a link between clinical studies and NMDAR hypofunction animal models of schizophrenia. The acute administration of NMDAR antagonists to rodents in vivo produces behavioral and cognitive abnormalities that are considered to be comparable to schizophrenia symptomatology (e.g., hyperlocomotion, attention and working memory deficits; reviewed in Amann et al., 2010), increased cortical excitability (Homayoun and Moghaddam, 2007), and increased baseline γ power (Ma and Leung, 2000, 2007; Pinault, 2008; Ehrlichman et al., 2009; Hakami et al., 2009; Lazarewicz et al., 2010). Additionally, Hong et al. (2010) found that acute ketamine administration in humans increased the power of a sensory-evoked γ oscillation, and the genetic ablation of NMDARs on PV interneurons in mice results in increased baseline γ power (Korotkova et al., 2010; Carlén et al., 2011). While acute NMDAR antagonism is an attractive animal model of schizophrenia, the absence of evidence for increased baseline γ in SZ has limited the relevance of this model to schizophrenia. The present study suggests that increased baseline γ power can in fact be detected in at least one part of the brain in SZ, and increases the convergence between the clinical literature and NMDAR hypofunction animal models of schizophrenia.
In this data set, SZ had deficits in ASSR PLF but not evoked power. Baseline 40 Hz power was not correlated with ASSR PLF, so baseline total power and stimulus-evoked phase synchronization appear to be independent. Baseline 40 Hz power was however positively correlated with ASSR evoked power for the LH radial dipole in SZ. In a similar vein, Lazarewicz et al. (2010) found that both baseline γ power and the power of a stimulus-evoked γ oscillation were increased in rats following a high dose of ketamine, although they did not report whether the baseline and evoked γ increases were correlated. Presumably, both baseline and stimulus-evoked γ increases could be due to the same disinhibition of pyramidal cells by hypoactive NMDARs on PV interneurons.
It should be noted that one of the difficulties in analyzing non-stimulus locked oscillations, particularly in the higher frequency bands, is that these oscillations are prone to contamination by muscle and ocular artifact (Keren et al., 2010; Shackman et al., 2010). Thus, a finding of increased γ power from scalp electrode recordings during baseline or resting states could be due to non-EEG sources, even with stringent artifact rejection criteria (Shackman et al.,2010). We sought to overcome this potential confound by using a paradigm in which baseline power changes were expected at a particular frequency (40 Hz). In addition, we utilized ICA to remove artifactual activity across the frequency spectrum, and employed source localization to focus on brain areas of interest. While we did find that baseline power tended to be increased across a wide frequency band in patients, the effect was significant only at 40 Hz. Therefore, we are confident in the validity of this effect.
Oscillatory activity in the γ band may be sensitive to the integrity of local circuit inhibition and factors that modulate the efficacy of this inhibition, such as NMDAR functionality. The present findings provide a link between human and animal model data in the study of the neural substrates of schizophrenia, and suggest that particular measures of γ activity may prove to be sensitive to particular cortical circuit abnormalities in neuropsychiatric disorders that can also be studied with animal models. Thus, measures of γ oscillations may prove to be useful biomarkers for translational research.
Conflict of Interest Statement
In the past 3 years, Kevin M. Spencer received consultation fees from Galenea Inc. and Bristol-Myers Squibb. Kevin M. Spencer also received a Research and Development Grant from Galenea Inc. that was administered through the Boston VA Research Institute.
Acknowledgments
This work was supported by a US Department of Veterans Affairs Merit Review grant (CX000154), US National Institutes of Health grants R03 MH076760 and R01 MH080187,and a NARSAD Young Investigator Award. The author thanks Lisa Lucia and Meredith Klump for their technical assistance.
References
Amann, L. C., Gandal, M. J., Halene, T. B., Ehrlichman, R. S., White, S. L., McCarren, H. S., and Siegel, S. J. (2010). Mouse behavioral endophenotypes for schizophrenia. Brain Res. Bull. 83, 147–161.
Andreasen, N. C. (1983). The Scale for the Assessment of Negative Symptoms (SANS). Iowa City: University of Iowa.
Andreasen, N. C. (1984). The Scale for the Assessment of Positive Symptoms (SAPS). Iowa City: University of Iowa.
Bartos, M., Vida, I., and Jonas, P. (2007). Synaptic mechanisms of synchronized gamma oscillations in inhibitory interneuron networks. Nat. Rev. Neurosci. 8, 45–56.
Bitanihirwe, B. K. Y., Lim, M. P., Kelley, J. F., Kaneko, T., and Woo, T. U. W. (2009). Glutamatergic deficits and parvalbumin-containing inhibitory neurons in the prefrontal cortex in schizophrenia. BMC Psychiatry 9, 71. doi: 10.1186/1471-244X-9-71
Carlén, M., Meletis, K., Siegle, J. H., Cardin, J. A., Futai, K., Vierling-Claassen, D., Rühlmann, C., Jones, S. R., Deisseroth, K., Sheng, M., Moore, C. I., and Tsai, L.-H. (2011). A critical role for NMDA receptors in parvalbumin interneurons for gamma rhythm induction and behavior. Mol. Psychiatry. doi: 10.1038/mp.2011.31 [Epub ahead of print].
Chance, S. A., Casanova, M. F., Switala, A. E., and Crow, T. J. (2008). Auditory cortex asymmetry, altered minicolumn spacing and absence of ageing effects in schizophrenia. Brain 131, 3178–3192.
Chatrian, G. E., Lettich, E., and Nelson, P. L. (1985). Ten percent electrode system for topographic studies of spontaneous and evoked EEG activity. Am. J. EEG Technol. 25, 83–92.
Cho, R.Y., Konecky, R. O., and Carter, C. S. (2006). Impairments in frontal cortical gamma synchrony and cognitive control in schizophrenia. Proc. Natl. Acad. Sci. U.S.A. 103, 19878–19883.
Coyle, J. T., Tsai, G., and Goff, D. (2003). Converging evidence of NMDA receptor hypofunction in the pathophysiology of schizophrenia. Ann. N.Y. Acad. Sci. 1003, 318–327.
Daskalakis, Z. J., Fitzgerald, P. B., and Christensen, B. K. (2007). The role of cortical inhibition in the pathophysiology and treatment of schizophrenia. Brain Res. Rev. 56, 427–442.
Di Lazzaro, V., Oliviero, A., Profice, P., Pennisi, M. A., Pilato, F., Zito, G., Dileone, M., Nicoletti, R., Pasqualetti, P., and Tonali, P. A. (2003). Ketamine increases motor cortex excitability to transcranial magnetic stimulation. J. Physiol. 547, 485–496.
Ehrlichman, R. S., Gandal, M. J., Maxwell, C. R., Lazarewicz, M. T., Finkel, L. H., Contreras, D., Turetsky, B. I., and Siegel, S. J. (2009). N-methyl-D-aspartic acid receptor antagonist-induced frequency oscillations in mice recreate pattern of electrophysiological deficits in schizophrenia. Neuroscience 158, 705–712.
Ferrarelli, F., Massimini, M., Peterson, M. J., Riedner, B. A., Lazar, M., Murphy, M. J., Huber, R., Rosanova, M., Alexander, A. L., Kalin, N., and Tononi, G. (2008). Reduced evoked gamma oscillations in the frontal cortex in schizophrenia patients: a TMS/EEG study. Am. J. Psychiatry 165, 996–1005.
ffytche, D. H., Howard, R. J., Brammer, M. J., David, A., Woodruff, P., and Williams, S. (1998). The anatomy of conscious vision: an fMRI study of visual hallucinations. Nat. Neurosci. 1, 738–742.
Ford, J. M., Roach, B. J., Faustman, W. O., and Mathalon, D. H. (2008). Out-of-synch and out-of-sorts: dysfunction of motor-sensory communication in schizophrenia. Biol. Psychiatry 63, 736–743.
Hakami, T., Jones, N. C., Tolmacheva, E. A., Gaudias, J., Chaumont, J., Salzberg, M., O’Brien, T. J., and Pinault, D. (2009). NMDA receptor hypofunction leads to generalized and persistent aberrant γ oscillations independent of hyperlocomotion and the state of consciousness. PLoS ONE 4, e6755. doi: 10.1371/journal.pone.0006755
Heckers, S., Rauch, S. L., Goff, D., Savage, C. R., Schacter, D. L., Fischman, A. J., and Alpert, N. M. (1998). Impaired recruitment of the hippocampus during conscious recollection in schizophrenia. Nat. Neurosci. 1, 318–323.
Herdman, A. T., Lins, O., Van Roon, P., Stapells, D. R., Scherg, M., and Picton, T. W. (2002). Intracerebral sources of human auditory steady-state responses. Brain Topogr. 15, 69–86.
Hoffman, R. E., and Cavus, I. (2002). Slow transcranial magnetic stimulation, long-term depotentiation, and brain hyperexcitability disorders. Am. J. Psychiatry 159, 1093–1102.
Hollingshead, A. B. (1965). Two Factor Index of Social Position. New Haven, CT: Yale University Press.
Homayoun, H., and Moghaddam, B. (2007). NMDA receptor hypofunction produces opposite effects on prefrontal cortex interneurons and pyramidal neurons. J. Neurosci. 27, 11496–11500.
Hong, L. E., Summerfelt, A., Buchanan, R. W., O’Donnell, P, Thaker, G. K., Weiler, M. A., and Lahti, A. C. (2010). Gamma and delta neural oscillations and association with clinical symptoms under subanesthetic ketamine. Neuropsychopharmacology 35, 632–640.
Hugdahl, K., Løberg, E.-M., and Nygård, M. (2009). Left temporal lobe structural and functional abnormality underlying auditory hallucinations in schizophrenia. Front. Neurosci. 3, 34–45.
Kantrowitz, J. T., and Javitt, D. C. (2010). N-methyl-D-aspartate (NMDA) receptor dysfunction or dysregulation: the final common pathway on the road to schizophrenia? Brain Res. Bull. 83, 108–121.
Keren, A. S., Yuval-Greenberg, S., and Deouell, L. Y. (2010). Saccadic spike potentials in gamma-band EEG: characterization, detection and suppression. Neuroimage 49, 2248–2263.
Keselman, H. J., and Rogan, J. C. (1980). Repeated measures F tests and psychophysiological research: controlling the number of false positives. Psychophysiology 17, 499–503.
Kiebel, S. J., Tallon-Baudry, C., and Friston, K. J. (2005). Parametric analysis of oscillatory activity as measured with EEG/MEG. Hum. Brain Mapp. 26, 170–177.
Korotkova, T., Fuchs, E. C., Ponomarenko, A., von Engelhardt, J., and Monyer, H. (2010). NMDA receptor ablation on parvalbumin-positive interneurons impairs hippocampal synchrony, spatial representations, and working memory. Neuron 68, 557–569.
Krishnan, G. P., Vohs, J. L., Hetrick, W. P., Carroll, C. A., Shekhar, A., Bockbrader, M. A., and O’Donnell, B. F. (2005). Steady state visual evoked potential abnormalities in schizophrenia. Clin. Neurophysiol. 116, 614–624.
Krystal, J. H., D’Souza, C., Mathalon, D., Perry, E., Belger, A., and Hoffman, R. (2003). NMDA receptor antagonist effects, cortical glutamatergic function, and schizophrenia: toward a paradigm shift in medication development. Psychopharmacology 169, 215–233.
Kwon, J. S., O’Donnell, B. F., Wallenstein, G. V., Greene, R. W., Hirayasu, Y., Nestor, P. G., Hasselmo, M. E., Potts, G. F., Shenton, M. E., and McCarley, R. W. (1999). Gamma frequency-range abnormalities to auditory stimulation in schizophrenia. Arch. Gen. Psychiatry 56, 1001–1005.
Lazarewicz, M. T., Ehrlichman, R. S., Maxwell, C. R., Gandal, M. J., Finkel, L. H., and Siegel, S. J. (2010). Ketamine modulates theta and gamma oscillations. J. Cogn. Neurosci. 22, 1452–1464.
Leicht, G., Kirsch, V., Giegling, I., Karch, S., Hantschk, I., Möller, H.-J., Pogarell, O., Hegerl, U., Rujescu, D., and Mulert, C. (2010). Reduced early auditory evoked gamma-band response in patients with schizophrenia. Biol. Psychiatry 67, 224–231.
Lewis, D. A., Fish, K. N., Arion, D., and Gonzalez-Burgos, G. (2011). Perisomatic inhibition and cortical circuit dysfunction in schizophrenia. Curr. Opin. Neurobiol. doi: 10.1016/j.conb.2011.05.013 [Epub ahead of print].
Lewis, D. A., Hashimoto, T., and Volk, D. W. (2005). Cortical inhibitory neurons and schizophrenia. Nat. Rev. Neurosci. 6, 312–324.
Ma, J., and Leung, L. S. (2000). Relation between hippocampal gamma waves and behavioral disturbances induced by phencyclidine and methamphetamine. Behav. Brain Res. 111, 1–11.
Ma, J., and Leung, L. S. (2007). The supramammillo-septal-hippocampal pathway mediates sensorimotor gating impairment and hyperlocomotion induced by MK-801 and ketamineinrats. Psychopharmacology (Berl.) 191, 961–974.
Makeig, S., Bell, A. J., Jung, T.-P., and Sejnowski, T. J. (1996). Independent component analysis of electroencephalographic data. Adv. Neural Inf. Process. Syst. 8, 145–151.
Moghaddam, B., Adams, B., Verma, A., and Daly, D. (1997). Activation of glutamatergic neurotransmission by ketamine: a novel step in the pathway from NMDA receptor blockade to dopaminergic and cognitive disruptions associated with the prefrontal cortex. J. Neurosci. 17, 2921–2927.
Mulert, C., Kirsch, V., Pascual-Marqui, R., McCarley, R. W., and Spencer, K. M. (2011). Long-range synchrony of gamma oscillations and auditory hallucination symptoms in schizophrenia. Intl. J. Psychophysiol. 79, 55–63.
Olney, J. W., Labruyere, J., Wang, G., Wozniak, D. F., Price, M. T., and Sesma, M. A. (1991). NMDA antagonist neurotoxicity: mechanism and prevention. Science 254, 1515–1518.
Pinault, D. (2008). N-methyl-D-aspartate receptor antagonists ketamine and MK-801 induce wakerelated aberrant gamma oscillations in the rat neocortex. Biol. Psychiatry 63, 730–735.
Poulsen, C., Picton, T. W., and Paus, T. (2007). Age-related changes in transient and oscillatory brain responses to auditory stimulation in healthy adults 19–45 years old. Cereb. Cortex 17, 1454–1467.
Riečanský, I., Kašpárek, T., Řehulová, J., Katina, S., and Přikryl, R. (2010). Aberrant EEG responses to gammafrequency visual stimulation in schizophrenia. Schizophr. Res. 124, 101–109.
Shackman, A. J., McMenamin, B. W., Maxwell, J. S., Greischar, L. L., and Davidson, R. J. (2010). Identifying robust and sensitive frequency bands for interrogating neural oscillations. Neuroimage 51, 1319–1333.
Sohal, V. S., Zhang, F., Yizhar, O., and Deisseroth, K. (2009). Parvalbumin neurons and gamma rhythms synergistically enhance cortical circuit performance. Nature 459, 698–702.
Spencer, K. M. (2009). The functional consequences of cortical circuit abnormalities on gamma oscillations in schizophrenia: insights from computational modeling. Front. Hum. Neurosci. 3:33. doi: 10.3389/neuro.09.033.2009
Spencer, K. M., Nestor, P. G., Niznikiewicz, M. A., Salisbury, D. F., Shenton, M. E., and McCarley, R. W. (2003). Abnormal neural synchrony in schizophrenia. J. Neurosci. 23, 7407–7411.
Spencer, K. M., Nestor, P. G., Perlmutter, R., Niznikiewicz, M. A., Klump, M. C., Frumin, M., Shenton, M. E., and McCarley, R. W. (2004). Neural synchrony indexes disordered perception and cognition in schizophrenia. Proc. Natl. Acad. Sci. U.S.A. 101, 17288–17293.
Spencer, K. M., Niznikiewicz, M. A., Nestor, P. G., Shenton, M. E., and McCarley, R. W. (2009). Left auditory cortex gamma synchronization and auditory hallucination symptoms in schizophrenia. BMC Neurosci. 10, 85. doi: 10.1186/1471-2202-10-85
Spencer, K. M., Niznikiewicz, M. A., Shenton, M. E., and McCarley, R. W. (2008a). Sensory-evoked gamma oscillations in chronic schizophrenia. Biol. Psychiatry 63, 744–747.
Spencer, K. M., Salisbury, D. F., Shenton, M. E., and McCarley, R. W. (2008b). Gamma-band auditory steady-state responses are impaired in first episode psychosis. Biol. Psychiatry 64, 369–375.
Tallon-Baudry, C., Bertrand, O., Delpuech, C., and Pernier, J. (1996). Stimulus specificity of phase-locked and nonphase-locked 40 Hz visual responses in human. J. Neurosci. 16, 4240–4249.
Whittington, M. A., and Traub, R. (2003). Inhibitory interneurons and network oscillations in vitro. Trends Neurosci. 26, 676–682.
Winterer, G., Ziller, M., Dorn, H., Frick, K., Mulert, C., Wuebben, Y., Herrmann, W. M., and Coppola, R. (2000). Schizophrenia: reduced signal-to-noise ratio and impaired phase-locking during information processing. Clin. Neurophysiol. 111, 837–849.
Woo, T.-U. W., Spencer, K., and McCarley, R. W. (2010). Gamma oscillation deficits and the onset and early progression of schizophrenia. Harv. Rev. Psychiatry 18, 173–189.
Woo, T.-U. W, Walsh, J. P., and Benes, F. M. (2004). Density of glutamic acid decarboxylase 67 messenger RNA-containing neurons that express the N-methyl-D-aspartate receptor subunit NR2A in the anterior cingulate cortex in schizophrenia and bipolar disorder. Arch. Gen. Psychiatry 61, 649–657.
Yizhar, O., Fenno, L. E., Prigge, M., Schneider, F., Davidson, T. J., O’Shea, D. J., Sohal, V. S., Goshen, I., Finkelstein, J., Paz, J. T., Stehfest, K., Fudim, R., Ramakrishnan, C., Huguenard, J. R., Hegemann, P., and Deisseroth, K. (2011). Neocortical excitation/inhibition balance in information processing and social dysfunction. Nature 477, 171–178.
Keywords: schizophrenia, gamma oscillation, auditory steady-state response, NMDA receptor
Citation: Spencer KM (2011) Baseline gamma power during auditory steady-state stimulation in schizophrenia. Front. Hum. Neurosci. 5:190. doi: 10.3389/fnhum.2011.00190
Received: 14 August 2011; Accepted: 31 December 2011;
Published online: 13 January 2012.
Edited by:
Kenneth Hugdahl, University of Bergen, NorwayReviewed by:
Ole A. Andreassen, University of Oslo, NorwayPeter Uhlhaas, Max Planck Institute for Brain Research, Germany
Copyright: © 2012 Spencer. This is an open-access article distributed under the terms of the Creative Commons Attribution Non Commercial License, which permits non-commercial use, distribution, and reproduction in other forums, provided the original authors and source are credited.
*Correspondence: Kevin M. Spencer, Research Service, Veterans Affairs Boston Healthcare System, Research 151C, 150 South Huntington Avenue, Boston, MA 02130, USA. e-mail:a2V2aW5fc3BlbmNlckBobXMuaGFydmFyZC5lZHU=