- 1Center for Biomedical Technology, Laboratory of Cognitive and Computatioal Neuroscience, Complutense University of Madrid, Polytechnic University of Madrid, Madrid, Spain
- 2Department of Basic Psychology, Autonoma University of Madrid, Madrid, Spain
- 3Center for Biomedical Technology, Polytechnic University of Madrid, Madrid, Spain
Working memory (WM) is the ability to transiently maintain and manipulate internal representations beyond its external availability to the senses. This process is thought to support high level cognitive abilities and been shown to be strongly predictive of individual intelligence and reasoning abilities. While early models of WM have relied on a modular perspective of brain functioning, more recent evidence suggests that cognitive functions emerge from the interactions of multiple brain regions to generate large-scale networks. Here we will review the current research on functional connectivity of WM processes to highlight the critical role played by neural interactions in healthy and pathological brain states. Recent findings demonstrate that WM abilities are not determined solely by local brain activity, but also rely on the functional coupling of neocortical-hippocampal regions to support WM processes. Although the hippocampus has long been held to be important for long-term declarative memory, recent evidence suggests that the hippocampus may also be necessary to coordinate disparate cortical regions supporting the periodic reactivation of internal representations in WM. Furthermore, recent brain imaging studies using connectivity measures, have shown that changes in cortico-limbic interactions can be useful to characterize WM impairments observed in different neuropathological conditions. Recent advances in electrophysiological and neuroimaging techniques to model network activity has led to important insights into how neocortical and hippocampal regions support WM processes and how disruptions along this network can lead to the memory impairments commonly reported in many neuropathological populations.
Much of our knowledge about the neural substrates of working memory (WM) comes from extensive neuropsychological and neuroimaging research focused on determining the functional specialization of specific brain areas in this cognitive function. Findings from these investigations have delineated a remarkable consistent group of areas that contribute to WM processes, this is, prefrontal cortex (PFC) (Goldman-Rakic, 1995; Braver et al., 1997; Rypma and D'Esposito, 1999; D'Esposito et al., 2000), neocortical associative areas (Druzgal and D'Esposito, 2001; Postle et al., 2003; Fiebach et al., 2007), and more recently the medial temporal lobes (MTL) (Abrahams et al., 1999; Campo et al., 2005a,b, 2009; Carrozzo et al., 2005; Karlsgodt et al., 2005; Nichols et al., 2006; Olson et al., 2006; Petersson et al., 2006; Axmacher et al., 2007, 2008a; Braun et al., 2008; Cashdollar et al., 2009; Olsen et al., 2009; Schon et al., 2009; Wagner et al., 2009; Öztekin et al., 2010; Toepper et al., 2010; van Vugt et al., 2010; Faraco et al., 2011; Lee and Rudebeck, 2011). Although the involvement of MTL in WM is matter of debate (Talmi et al., 2005; Zarahn et al., 2005; Shrager et al., 2008; Tudesco Ide et al., 2010; Jeneson et al., 2011), it has called for a re-evaluation of the prevailing conception considering that MTL is exclusively dedicated to long-term memory (LTM). These findings have reactivated the proposal of proceduralist or unitary models of memory, according to which there is a continuum between WM and LTM. Proponents of this notion call into question the existence of different stores for WM and LTM and consider that both processes are governed by the same principles (Crowder, 1993; Cowan, 2001; Nairne, 2002; Oberauer, 2002; McElree, 2006; Öztekin et al., 2010; Nee and Jonides, 2011). It can also be derived from this conception that WM and LTM can be assumed to involve the activation of a common neural network, including MTL (Fuster, 1995; Cartling, 2001; Ruchkin et al., 2003; Ranganath and Blumenfeld, 2005; Cashdollar et al., 2011).
Based on the assumption that cognitive processes engage distributed neural networks, if we want to gain a clearer understanding of the functional role of MTL in WM, it cannot be considered in isolation, but evaluated within the neural context in which it takes place (McIntosh, 1999). It is, therefore, necessary to characterize that role from the perspective of the functional systems (Bullmore and Sporns, 2009). Although “it seems obvious that brain regions must cooperate to accomplish the various tasks in which they are communally required” (Gordon, 2011), it has not been till recently that a more dynamic perspective postulating that cognitive functions rely on the coordinated action of widespread brain regions has gained interest in detriment of a more localizationist perspective (Engel et al., 2001; Sporns and Tononi, 2007; Bressler and Menon, 2010). Consequently, research has recently started to focus on the study of neural networks, and now it is widely accepted that mechanisms supporting memory processes can be better explained by neural communication (Fell and Axmacher, 2011). Here we will review the current research on functional networks of WM, with a special focus on findings providing compelling evidence about the involvement of neocortical-hippocampal coupling. First, we will present data obtained across different experiments in healthy individuals by manipulating mnemonic requirements. Then, under the consideration that the degree of participation of brain regions in a functional network may depend on the operational principles governing a cognitive process (D'Esposito, 2007), we will review studies that consider the participation of the MTL-neocortical network both in WM and LTM. Finally, we will show how disturbed interactions between MTL and neocortex may underlie the WM dysfunction observed in different pathologies, and discuss the contribution of these findings to further understand the functional role of hippocampal-neocortical connectivity in WM.
Functional Brain Networks
Functional networks can be defined “by a set of elements with time-variant properties altogether that interact with each other” (Stephan and Friston, 2007). Identification of the constituents of a functional network is necessary in order to delineate it (McIntosh, 1999; Bressler and Menon, 2010). Related to brain systems, for any brain region to be considered as a part of a functional neural network, it must be shown that, interacting with a specific group of areas, is engaged in a particular function or process (Bressler and Menon, 2010). In recent years, neuroscience has witnessed the appearance of numerous approaches for modeling functional brain networks (Guye et al., 2008; Bullmore and Sporns, 2009; McIntosh, 2011). One major distinction in the current methods pertains to the nature of connectivity. Connections among elements of a network can be characterized as undirected links, this is functional connectivity, or as directed links, this is effective connectivity (Sporns and Tononi, 2007; Friston, 2009; Bressler and Menon, 2010). Functional connectivity models establish symmetrical correlations between brain regions. In functional magnetic resonance imaging (fMRI) usually involves the pairwise or partial correlation of BOLD signal among different voxels across the brain. In electrophysiology the phase-locking value (PLV) and spectral coherence refer to the synchronization of the phases of oscillatory signals or the correlation between oscillatory signals (combining power and phase), respectively (Castellanos et al., 2011; Fell and Axmacher, 2011; Cohen et al., 2011). Effective connectivity models go further, and establish how the dependencies are mediated. Let's say, whether synchronization arise via master-slave or by mutual entrainment processes (Penny et al., 2009). These methods imply the specification of a causal model including structural parameters, which is not necessary in functional connectivity (Sporns and Tononi, 2007; Stephan and Friston, 2007; Friston, 2009; McIntosh, 2011; Valdes-Sosa et al., 2011; although see Vicente et al., 2011).
Network Dynamics between MTL and Neocortex in the Healthy Brain
Most of the current conceptualizations of WM considers that it is a distributed system in which PFC and MTL interact with posterior cortices (mainly inferior temporal cortex, ITC), modulating its activity by enhancing relevant and suppressing irrelevant information-related activity (Ranganath and D'Esposito, 2005). From this perspective it is assumed that WM maintenance processes constitute the engagement of the same neural networks that support online processing (Fuster, 1995; Ruchkin et al., 2003; Pasternak and Greenlee, 2005; D'Esposito, 2007), and three core interactions can be highlighted: PFC-ITC; MTL-ITC; and PFC-MTL. Thus, a PFC-ITC interaction is modeled computationally as coupled attractor networks (i.e., “network of neurons with excitatory interconnections that can settle into a stable pattern of firing” Rolls, 2010b), in which a WM module (i.e., PFC) exerts a top-down modulatory influence in a perceptual module (i.e., ITC) (Renart et al., 1999, 2001). Interaction of hippocampus with content-specific regions in the ITC via rhinal cortex (Fransén, 2005; Chapman et al., 2008; Vitay and Hamker, 2008; Olsen et al., 2009; Zeithamova and Preston, 2010; Schwindel and McNaughton, 2011) has been also suggested as a mechanism for the reinstatement of specific information processed or stored in those areas (Rolls, 2000; Woloszyn and Sheinberg, 2009; Szatmary and Izhikevich, 2010). This interaction is proposed to be controlled by MTL based on short-term couplings from neural assemblies in MTL to corresponding assemblies in the neocortex (Cartling, 2001). Finally, recent evidence from animal studies suggest that the interplay between PFC and MTL, especially hippocampus, is key for normal WM function (Benchenane et al., 2011; for review see Colgin, 2011; Gordon, 2011), although there remains uncertainty about the functional role of this interaction (Gordon, 2011).
While the precise mechanism mediating the interaction between two brain regions is not known, synchronization of neural oscillatory activity may represent a mechanism for inter-regional communication (for a review see Fries, 2005; Wang, 2010). In networks of synchronized neurons, phase synchronization supports neural communication and induce synaptic plasticity between the interacting regions (Duzel et al., 2010; Jutras and Buffalo, 2010; Fell and Axmacher, 2011). Recent models propose that theta oscillations play an important role in the interplay among the neural populations involved in memory processes by integrating widespread local circuits, that are organized in high frequencies, through phase synchronization (Canolty and Knight, 2010; Young, 2011; for reviews see Jutras and Buffalo, 2010; Battaglia et al., 2011; Fell and Axmacher, 2011). Theta oscillations appear preferentially in the hippocampus, in the MTL and in other brain regions interconnected with the hippocampus (Düzel et al., 2010; Battaglia et al., 2011).
Here we will review neuroimaging studies focusing on characterizing the functional interactions among PFC-MTL-posterior cortex, and how their findings adjudicate between the above mentioned proposals. In order to investigate the neural interactions underlying WM operations, most of these studies typically examine how connections among the elements of the network are modified when mnemonic demands are systematically modified, mainly parametric manipulation of memory load and/or the use of delay intervals of variable length. The rationale is that if certain interaction is significantly involved in WM, then the parameters of such interaction (i.e., strength or directionality of connection) should be modulated by the systematic modification of task requirements (van Vugt et al., 2010; Colgin, 2011; Fell and Axmacher, 2011).
Load-Dependent Functional Interactions
Changes in network interactions as a function of WM load have been explored in several studies providing some hints about the dynamics of WM operations (see Table 1). That PFC exerts a top-down modulation of the activity in content-representing posterior areas as a mechanism underlying maintenance of verbal or visual information is partially supported by findings showing load-dependent functional connectivity between PFC and ITC (Fiebach et al., 2006), or enhanced phase synchronization between frontal and temporal regions in the gamma (Axmacher et al., 2008a) or theta range (Cashdollar et al., 2009; Payne and Kounios, 2009). Only a recent study made an exception to this observation (Rissman et al., 2008). Hence, this load-induced enhancement could reflect a top-down selective strengthening of representation and maintenance of relevant information (Payne and Kounios, 2009). Consistent with the role of PFC in modulating neural activity in posterior regions selectively related to relevant stimuli are findings from several studies using functional connectivity measures (Sauseng et al., 2005; Yoon et al., 2006; Gazzaley et al., 2007; Protzner and McIntosh, 2009; see also Edin et al., 2009). Importantly, these findings were causally supported by recent studies using transcranial magnetic stimulation (Feredoes et al., 2011; Zanto et al., 2011). Also related to this issue, complexity of manipulation performed on information actively maintained modulate prefrontal-posterior coupling in theta or alpha frequency ranges (Sauseng et al., 2005, 2007), further supporting the idea of a top-down control process, and suggesting that phase coupling is a probable operational mechanism (Zanto et al., 2011). As we introduced above, functional coupling between PFC and MTL is considered one of the core connections in the neural circuitry underlying WM. Crucially, increasing the number of consecutively presented faces (Rissman et al., 2008; Axmacher et al., 2009b) or letters (Finn et al., 2010) using a modified Stenberg paradigm led to a greater connectivity strength between PFC and MTL structures, either hippocampus or parahippocampus. Theta oscillation has been proposed as a potential neurophysiological mechanism mediating this interaction (Mitchell et al., 2008; Anderson et al., 2010; Battaglia et al., 2011). This proposal has been supported by studies demonstrating phase-locking of PFC spiking activity with the hippocampal theta cycle in animals during WM tasks that showed a positive correlation with performance or behavioral demands (Jones and Wilson, 2005; Siapas et al., 2005; Hyman et al., 2010; Brockmann et al., 2011; Fujisawa and Buzsaki, 2011; for a review see Colgin, 2011; Gordon, 2011). However, PFC-hippocampal communication through theta oscillations in human WM has not been directly observed thus far (Raghavachari et al., 2006; but see Anderson et al., 2010). Additionally, Axmacher and colleagues (Axmacher et al., 2008a) observed a decreased correlation of hippocampus with two contralateral frontal regions (middle and inferior frontal gyrus) with increasing memory demands. Nonetheless, an elegant study combining functional and structural connectivity measures has reported direct empirical evidence that subjects with stronger structural connections between the hippocampus and the PFC have slower PFC oscillations, which correlated with better performance in a WM task (Cohen, 2011). Consistent with this finding, connectivity strength from PFC to MTL was positively correlated with performance in a verbal WM task (Campo et al., in press). These data support the relevancy of PFC-MTL interaction in human WM by showing that individual differences in task performance could be related to differences in the strength of such interaction (Gordon, 2011). Increased WM load has also been shown to enhance connectivity strength and synchronization within MTL and between MTL, either hippocampus or parahippocampus, and ITC (Axmacher et al., 2008a; Rissman et al., 2008). A phase-modeling approach characterized this interaction as a top-down modulatory effect from MTL to ITC in high frequency range (Axmacher et al., 2008a). Furthermore, connectivity strength from MTL to ITC distinguished between healthy controls and patients with temporal lobe epilepsy of hippocampal origin during a verbal WM task (see below Campo et al., in press). MTL could participate in reinstating WM contents in ITC by means of this top-down interaction (Woloszyn and Sheinberg, 2009). These connectivity findings point to MTL-ITC inter-regional interactions, probably mediated by cross-frequency coupling of beta/gamma amplitude to theta phase (Babiloni et al., 2009; Axmacher et al., 2010; Jutras and Buffalo, 2010), as a crucial neural process for WM encoding and maintenance (Fell and Axmacher, 2011). Theta phase-locked gamma hippocampo-cortical loops may provide a coding scheme for structuring the maintenance of multiple items in WM (Lisman, 2005; Moran et al., 2010; Fell and Axmacher, 2011; Freunberger et al., 2011; Lundqvist et al., 2011). According to this scheme, individual memories represented by a cell assembly fires at a gamma subcycle in a certain theta phase. In this way, information contained in distributed populations across the neocortex can be transferred to the hippocampal networks. Crucially, two recent studies (Fuentemilla et al., 2010; Poch et al., 2011) reported evidence that the periodicity of distributed WM reactivations in the beta/gamma frequency range were phase-locked to the ongoing hipocampal and frontal theta activity, which was functionally relevant for the maintenance of configural-relational information. The interaction of theta and gamma oscillations may operates as the limited human brain resource that engenders limited WM capacity (Jensen and Lisman, 1998; Düzel et al., 2010).
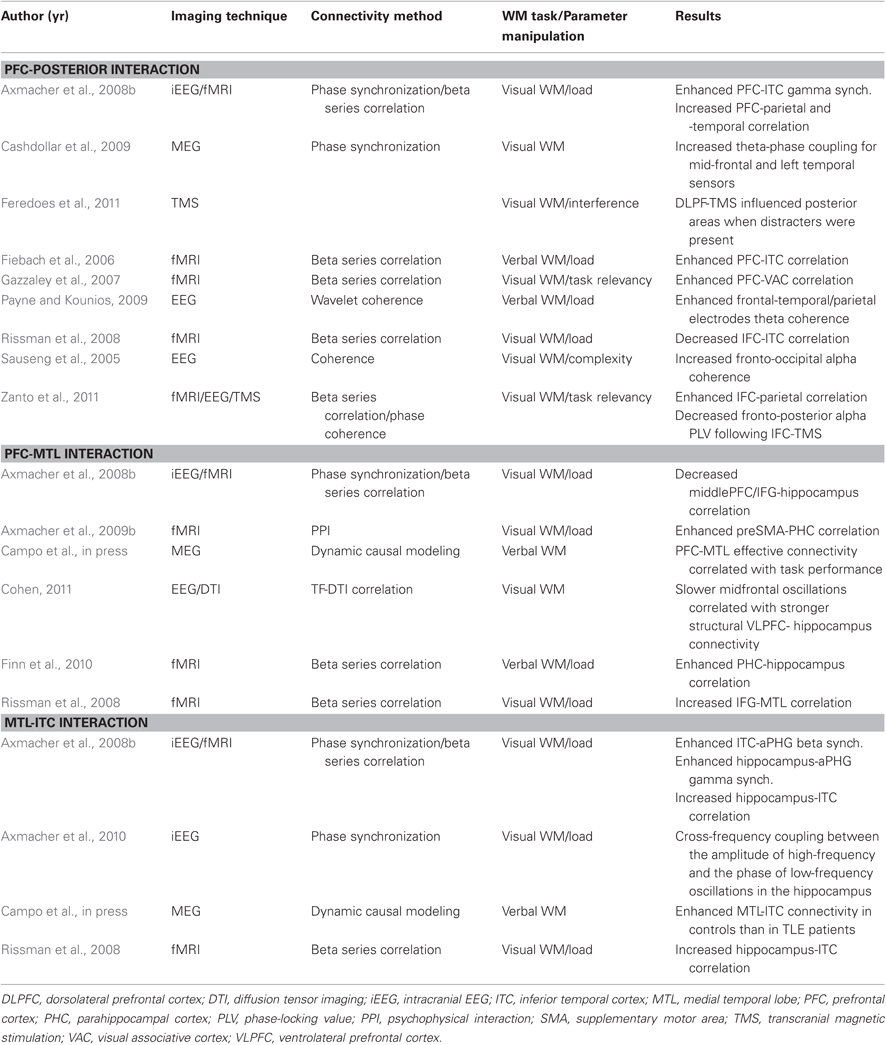
Table 1. Results from studies showing modulation among PFC-MTL-posterior cortex during the performance of a WM task as a function of parametric manipulation of mnemonic demands.
Finally, one interesting finding is that maintenance of an increasing number of items in WM led to smaller or more selectivity set of connections (Axmacher et al., 2008a; Ginestet and Simmons, 2010). Although speculatively, Axmacher et al. (Axmacher et al., 2008a) proposed that this effect could be reflecting some properties of hippocampus sparse coding (Rolls, 2010a; Schwindel and McNaughton, 2011).
Time-Dependent Functional Interactions
Data from animal and human studies have shown that neural activity in brain regions recruited during WM tasks can be influenced by the length of the mnemonic delay (Haxby et al., 1995; Owen et al., 1996; Elliott and Dolan, 1999; Lee and Kesner, 2003; Picchioni et al., 2007; Yoon et al., 2008; Buchsbaum et al., 2010). However, very few studies have explored how functional interactions among the elements of the network varied as the length of the delay interval is manipulated. McIntosh and colleagues (McIntosh et al., 1996; McIntosh, 1999; see also Grady et al., 2001) parametrically increased the duration of the delay during a WM task for faces. The authors characterized a functional circuit encompassing inferior frontal regions (IFCs), primary and multimodal sensory areas, anterior cingulate, and hippocampal gyrus. The interactions among the elements of this functional network were modulated (expressed by the sign of connections, as well as some changes in strength) by the manipulation of the temporal dimension of the task. Specifically, the cortico-limbic interactions that characterized the short-delay networks showed a shift from right to left hemisphere with the increase of delay interval, and more effects from prefrontal regions (BA47) as delay was extended. Modulation of the interactions between hippocampus and PFC as a function of temporal WM constraints could be related to differential roles played by both structures during WM operations (Yoon et al., 2008). Greater involvement of PFC in monitoring processes and prospective memory has been proposed (Floresco et al., 1997; Fuster, 2001; Gilbert, 2011), and thus extending the temporal requirements for information maintenance may require greater strength of connections from this region (Buchsbaum et al., 2010; Churchwell and Kesner, 2011).
A common Neural Network for Working Memory and Long-Term Memory?
We have seen a number of studies showing that WM depends on a neural network including PFC, MTL, and posterior neocortical regions, whose interactions are modified to accommodate the increase of mnemonic demands. Similar functional networks have been described for studies exploring patterns of connectivity during LTM processes (Rajah et al., 1999; Fell et al., 2001; Simons and Spiers, 2003; Addis and McAndrews, 2006; Summerfield et al., 2006; Kahn et al., 2008; Babiloni et al., 2009; Anderson et al., 2010). These evidences constitute further support for the emerging view that the hippocampus and related structures form part of a neural network involved in both LTM and WM processes. However, despite the similarity between neural networks, the question emerges as to whether the connectivity among the elements of these apparently analogous functional networks differ qualitatively or quantitatively, thus allowing for a distinction between memory processes (McIntosh, 1999; Rajah and McIntosh, 2005). Accordingly, direct examinations of the pattern of interactions of MTL across tasks simultaneously tapping both WM and LTM process are needed in order to determine whether these interactions are reflecting a common functional architecture or not (Axmacher et al., 2009a; Fell and Axmacher, 2011). Using tasks designed under the influence of the framework of unitary memory systems (Cowan, 2001; Oberauer, 2002; McElree, 2006) could shed light on this question (see Figure 1). These models consider that information could be represented in different states, either in the focus of attention or in an activated state with different accessibility (Lustig et al., 2009; Nee and Jonides, 2011). Whether neural networks underlying these states were similar or could be differentiated was investigated in a recent fMRI study (Nee and Jonides, 2008). In this study mechanisms of retrieval of items within the focus of attention or outside the focus (i.e., WM) were explored using functional connectivity analyses. Information considered to be within the focus of attention was associated with stronger connectivity between ITC and frontal and posterior parietal regions (see also Roth and Courtney, 2007). Crucially, retrieval dynamics of information outside the focus (Lewis-Peacock et al., 2012) were characterized by increased coupling between ventrolateral prefrontal cortex (VLPFC) and MTL, as well as with other neocortical sites, as a function of retrieval demands (see Figure 1). Results from Rissman et al. (2008), can be interpreted under this framework. While increased PFC-ITC coupling was observed during maintenance of low WM load (i.e., one face), increased involvement of MTL and PFC mediated coupling was observed at higher mnemonic loads (i.e., four faces). Hence, these data support that processes of STM are similar to those implicated in LTM, suggesting common underlying mechanisms between STM and LTM. Nonetheless, differentiation between retrieval and updating of information should be explored in order to refine the characterization of the neural bases reported by this study (Bledowski et al., 2009; although see Roth and Courtney, 2007). Additionally, whether the information outside the focus of attention is better explained by two-state models or by three-state models are in need of further functional connectivity studies (Öztekin et al., 2010; Lewis-Peacock et al., 2012; Nee and Jonides, 2011).
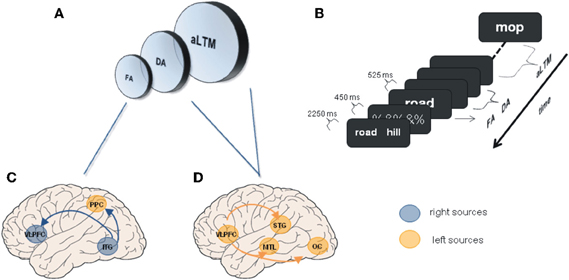
Figure 1. Unitary memory models and associated functional connectivity networks. (A) Unitary memory models posit that perception, WM and LTM do not differ in the underlying neural substrates, but in the state of information representations. Accordingly, two or three states are proposed: a focused of attention (FA); a region of direct access (DA); and other information that is hypothesized to be an activated portion of LTM (aLTM). The last two states are considered to be undistinguishable by some authors leading to two states models (Oberauer, 2002; McElree, 2006; Lustig et al., 2009; Öztekin et al., 2010; Nee and Jonides, 2011). (B) Serial position task (SP) of 12 items used to explore differences among the three hypothesized states, and to investigate underlying neural networks. SP12 corresponds to FA; SP9–11 corresponds to DA; SP1–8 corresponds to aLTM [Adapted from (Öztekin et al., 2010)]. (C) Regions demonstrating functional connectivity increases with right ITG related to the focus of attention [Adapted from Nee and Jonides (2008)]. (D) Some of the regions demonstrating enhanced connectivity with left mid-VLPFC related to aLTM [Adapted from Nee and Jonides (2008)]. Other regions were anterior STG and ventromedial PFC. ITG, inferior temporal gyrus; MTL, medial temporal lobe; OC, occipital cortex; PPC, posterior parietal cortex; STG, superior temporal gyrus; VLPFC, ventrolateral prefrontal cortex.
Another line of research exploring whether the same neural mechanism underlies WM and LTM has focused on the interaction between both types of processes. Several studies have shown that MTL WM maintenance-related activity contributes to subsequent LTM recognition (Schon et al., 2004; Ranganath et al., 2005a; Axmacher et al., 2007, 2009a; Khader et al., 2007). Using this paradigm, Ranganath and colleagues (Ranganath et al., 2005b) found that increase functional connectivity of hippocampus with a network of cortical regions including ITC and frontal areas during the delay period of a complex visual WM task led to improved LTM recognition of the maintained items. Likewise, a series of studies has refined these findings and identified (Axmacher et al., 2008b, 2009b) a parahippocampal (PHC) functional network as the hallmark of WM-LTM interaction. Using the right PHC as the seed region (Rissman et al., 2004), it was shown that it was significantly correlated with the hippocampus, after correction for number of trials, in all possible conditions relating both types of memory. Interestingly, the number of voxels which showed significant correlation with the seed region in the right PHC was reduced when successful WM performance was followed by subsequent forgetting as compared to subsequent recognition. Finally, subsequent LTM formation was facilitated by an increase in the number of regions engaged in a WM maintenance-related PHC functional network, which was independent of WM performance. However, subsequent memory performance was modulated by WM load and was inversely related to the strength of the interaction between PHC and medial frontal cortex. This is, LTM encoding and maintenance of multiple items in WM compete for the engagement of a PFC-MTL network (Axmacher et al., 2009b). Interestingly, recent findings (Cashdollar et al., 2009) propose hippocampal theta coordination of posterior regions during maintenance as a potential mechanism facilitating LTM encoding. Moreover, a recent study has found that slower oscillatory activity (i.e., theta range) during WM maintenance and stronger structural connectivity between hippocampus and PFC was predictive of LTM recognition (Cohen, 2011). Considered together, these findings provide evidence for an interaction between WM and LTM in MTL networks.
Disturbed Network Dynamics Between MTL and Neocortex
There is increasing agreement that cognitive impairments observed in neurological and neuropsychiatric disorders, whether they are characterized by localized focal lesion or not, are caused by abnormally organized or disconnected networks (Bassett et al., 2009). Therefore, further understanding of the hippocampo-cortical WM network could be gained by exploring the impact that such disorders produce on the integrity of the system (Menon, 2011). This is, how damage to one or more of its constituent elements lead to dynamic adjustments or reorganization of the remaining network in an attempt to compensate, and the efficacy of this change (McIntosh, 1999; Gazzaley et al., 2004; Rowe, 2010; Seghier et al., 2010; Castellanos et al., 2011; Gordon, 2011). Those pathological conditions in which the functioning of specific nodes of the network is disrupted, and consequently their connections, would provide the most valuable information.
Pathological disruption of the hippocampal formation has been primarily observed in Alzheimer's disease (AD) and temporal lobe epilepsy (TLE), although with different patterns of cell loss (Small et al., 2011). AD is characterized by gray matter atrophy and the formation of plaques, which are particularly prominent in the MTL and PCC (posterior cingulate cortex) (Braak and Braak, 1997; Thal et al., 2002; Sabuncu et al., 2011; Nickl-Jockschat et al., 2012). Interestingly, these areas show significantly reduced network connectivity (Zhou et al., 2008; Yao et al., 2010; Pievani et al., 2011). Moreover, abnormalities in functional connectivity have been shown to overlap with structural atrophy and beta-amyloid (β-amyloid) deposition (Buckner et al., 2009; Menon, 2011). Hence, cognitive impairments associated with AD, and particularly WM deficits, can be examined considering the disruption of these connections (Uhlhaas and Singer, 2006). When comparing functional connectivity patterns between patients with AD and healthy controls during a WM task for faces, it was found that activity in PFC correlated with hippocampus and extrastriate cortex in controls, while it was restricted to other frontal regions in patients (Grady et al., 2001). The pathological nature of this pattern of connectivity is reinforced by recent findings showing an increased functional connectivity between hippocampus and diffuse areas of PFC which was negatively correlated with performance on a memory task in amnestic mild cognitive impairment patients showing hippocampal atrophy (Bai et al., 2009). These results are consistent with studies showing an increased connectivity within anterior sites and sparing of hubs in frontal regions (Babiloni et al., 2006; Supekar et al., 2008; Bajo et al., 2010; Liang et al., 2011), and reduced fronto-posterior interaction and impairment of hubs in temporal regions (Stam et al., 2006; Supekar et al., 2008; Liang et al., 2011). TLE associated with hippocampal sclerosis (HS) (Wieser, 2004), also referred to as mesial TLE (mTLE), is typically considered a network disorder (Liao et al., 2010). Several studies in mTLE have shown abnormal patterns of functional connectivity during a resting state condition, the so-called default network (Raichle et al., 2001), revealing not only local network abnormalities within lesional MTL, but more widespread effects in network interactions (Liao et al., 2010, 2011; Pereira et al., 2010). A recent study (Bettus et al., 2009) has shown a decreased basal functional connectivity within the epileptogenic MTL which was correlated with the degree of connectivity increase in the contralateral MTL. Interestingly, the strength of basal functional connectivity in the contralesional MTL was correlated with WM performance, in what was suggested to reflect a compensatory effect. Further evidence of connectivity changes in MTL networks associated to HS comes from a study comparing dynamic causal models (Friston et al., 2003) extracted from magnetoencephalographic recordings during verbal WM encoding (Campo et al., in press). Having established that the best model across subjects (i.e., Bayesian model comparison, Penny et al., 2004) evidenced bilateral, forward and backward connections, coupling ITC, IFCs, and MTL (Figure 2), it was found that left HS patients were characterized by a decreased backward connectivity from left MTL to left ITC, and an increased forward and backward PFC-hippocampal connectivity in the contralesional hemisphere. Interestingly, performance was positively correlated with left PFC-MTL connectivity strength in controls; while it was negatively correlated with right PFC-MTL across subjects. A critical role of hippocampal-dependent theta synchronization of activity in posterior regions for maintenance of relational visual information was demonstrated after showing that patients with bilateral HS exhibited impaired performance and selective loss of such synchronization when compared to a group of patients with TLE but intact hippocampi (Cashdollar et al., 2009).
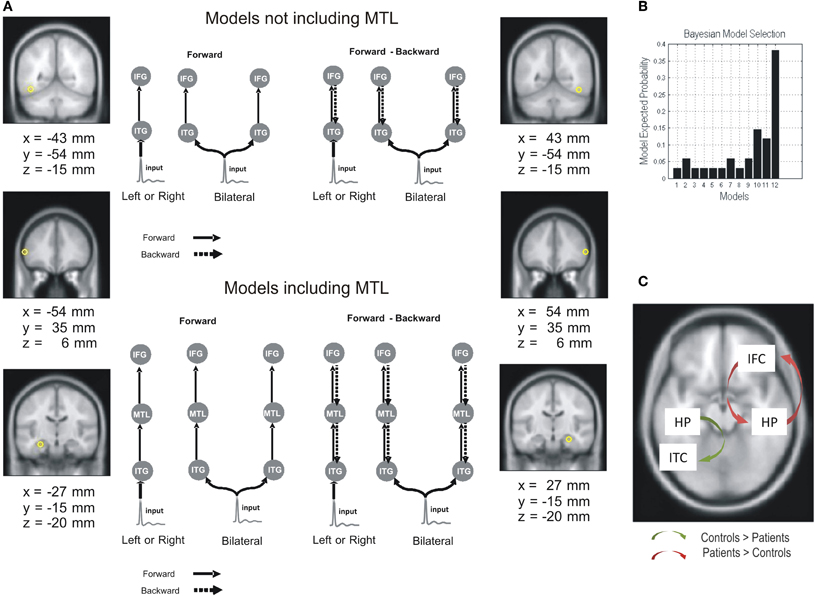
Figure 2. Effective connectivity differences between mTLE patients and controls in a verbal WM task. Dynamic causal models, extracted from magnetoencephalographic recordings during verbal WM encoding, were compared in TLE patients (with left hippocampal sclerosis) and controls. (A) Network architecture was specified on the basis of the inverse solutions for single subjects using multiple sparse priors. Twelve models were specified and inverted separately for each subject. Outlines of some of DCM models for the effective connectivity analysis are depicted. The models were constructed starting with simple architectures and adding hierarchical levels (i.e., sources and extrinsic connections). The simplest models only included the ITC and IFC sources, while more complex models included MTL sources. The sources were left unilateral, right unilateral, or bilateral. Models also differed in terms of their connections; forward only or both forward and backward. (B) Group level Bayesian selection of the 12 tested models: random fixed effects (RFX) showing model expected probability. Results indicate the best model is one with bilateral forward and backward connections comprising IFG, ITC, and MTL (Model 12). (C) Group differences in effective connectivity assessed using subject-specific (maximum a posteriori) parameter estimates. Adapted from Campo et al. (in press).
A clearly distinct neuropathological pattern can be observed in schizophrenia, which is a complex disorder mainly associated with a profound deterioration of PFC function and characterized by several cognitive impairments (Lewis and Gonzalez-Burgos, 2006; Bonilha et al., 2008; Pomarol-Clotet et al., 2010). Among these, WM dysfunction is regarded as a fundamental core of the disease (Goldman-Rakic, 1994; Lee and Park, 2005; Van Snellenberg, 2009; Haenschel and Linden, 2011) and has been typically attributed to specific alterations in the neural microcircuits of the PFC (Perlstein et al., 2001; Manoach, 2003; Arnsten et al., 2010; Banyai et al., 2011; Menon, 2011; Anticevic et al., in press). However, the disease is not considered to be caused by focal brain abnormalities (Friston, 1999; Uhlhaas and Singer, 2010). The extent to which WM impairments in schizophrenia reflect dysregulations of the underlying neural networks has begun to be exploited recently (Uhlhaas and Singer, 2006). A number of studies have reported disturbed interactions between PFC and temporal regions, including hippocampus, in patients with schizophrenia and in healthy individuals at risk of developing the disease, although the nature of the altered connectivity is complex (Winterer et al., 2003). Thus, mimicking animal models of genetic risk factors in which mutant mice showed reduced hippocampal-prefrontal connectivity during a WM task (Sigurdsson et al., 2010), functional connectivity studies have shown, for the most part, a decreased connectivity within PFC and between PFC and hippocampus and several posterior regions (Kim et al., 2003; Cho et al., 2006; Bassett et al., 2009; Benetti et al., 2009; Meda et al., 2009; Hashimoto et al., 2010; Henseler et al., 2010; Banyai et al., 2011; Kang et al., 2011). However, some studies have observed an abnormal persistent coupling between PFC and hippocampus and temporal regions while engaged in WM tasks (Meyer-Lindenberg et al., 2001, 2005; Crossley et al., 2009; Esslinger et al., 2009; Wolf et al., 2009). Several factors could be the cause of this inconsistency (i.e., chronicity, antipsychotic treatment, level of arousal, type of task; Manoach, 2003; Winterer et al., 2003), but their influence has so far not been systematically investigated. Thus, further research on the role of these factors in WM impairments and modulation of network interactions in schizophrenia is warranted. Moreover, interaction of WM-related specific connectivity deficits with generalized task-independent connectivity dysfunction should be explored (Wolf et al., 2009; Lynall et al., 2010; Cole et al., 2011; Fornito et al., 2011). Additionally, it is also conceivable that altered early perceptual and encoding processes modulate WM dysfunction. More investigation on this relationship will help to clarify the complexity of the dynamics underlying the observed deficits (Haenschel and Linden, 2011). Nonetheless, these data, along with data from structural connectivity studies (Karlsgodt et al., 2008; Spoletini et al., 2009; for a meta-analysis see Ellison-Wright and Bullmore, 2009), support the proposal that abnormal functional connectivity between PFC and temporal structures might underlie WM dysfunction in schizophrenia (Friston, 1998; Winterer et al., 2003; Stephan et al., 2006).
Concluding Remarks and Future Directions
Findings from connectivity studies we have reviewed thus far shed light on how the pattern of neural interactions of a functional network of brain regions, including MTL, varies as a result of WM dynamics. The picture that emerges is that connectivity of content-specific regions in posterior cortex with either PFC or MTL is enhanced during WM to adequate the system to mnemonic requirements. Evidence that MTL connectivity is modulated by parametric manipulations of WM task demands, along with earlier findings from lesional and activation studies, bolster the view that MTL plays a critical role in WM processes. Under the perspective of unitary models of memory it can be stated that which brain regions within the WM network are functionally linked, this is the neural context (McIntosh, 1999), depend on which specific processes are taking place. Thus, different interactions support different levels of processing, from more attentional (i.e., PFC-ITC) to more mnemonic (i.e., MTL-ITC, PFC-MTL) (Nee and Jonides, 2008; Öztekin et al., 2010; Nee and Jonides, 2011). This modulatory feedback from PFC and MTL of the neural activity in posterior regions have been observed during the encoding, maintenance and recognition phases of WM, and with different types of stimuli. We have also seen how functional interactions of MTL during WM processes are predictive of how well the information is transferred to LTM (Axmacher et al., 2008b; Cohen, 2011). Moreover, connectivity strength between parahippocampus and PFC modulated the encoding of items in LTM during simultaneous WM maintenance, thus pointing to MTL based networks as a site of WM-LTM interactions (Axmacher et al., 2009b). The majority of these findings are derived from correlational methods, and cannot be used to make strong statements about causality. Thus, future studies should explore the directionality of the information flow using effective connectivity methods (Anderson et al., 2010; Campo et al., in press). Considered together, these data suggest that MTL participates in the WM network not only when stimuli are novel or relations between stimuli must be formed (Ranganath and Blumenfeld, 2005), but when the amount or complexity of to-be remembered stimuli cannot be accomplished using attention-based maintenance process (Rissman et al., 2008). Furthermore, these findings emphasize the relevance of neocortical-hippocampal coupling. Given that distinct sensory inputs arrive to specific regions of the MTL in virtue of their differential connectivity pathways (Rolls, 2000), stimulus class effects in the pattern functional connectivity of different MTL regions (i.e., perirhinal and parahippocampal cortex) should be explored in future experiments (Witter et al., 2000; Davachi, 2006; Olsen et al., 2009). The compelling evidence provided by the studies we have reviewed about the involvement of MTL in WM should be reconciled with several demonstrations of WM-LTM dissociation and insensitivity of WM performance to hippocampal damage (Vallar and Papagano, 1986; Carlesimo et al., 2001; Shrager et al., 2008; Tudesco Ide et al., 2010; Jeneson et al., 2011). Further research about of WM-LTM interplay and the role of MTL will be needed.
Even though the neural mechanism underlying information transfer between brain areas remains unknown, a prime candidate to play a key role in this process appears to be phase synchronization of oscillatory activity (Fries, 2005; Fell and Axmacher, 2011). With regard to WM it has been shown that, consistent with neurophysiological and network models (Lisman and Idiart, 1995; Lundqvist et al., 2011), phase synchronization between slower and higher oscillations reflect the retention of information (Sauseng et al., 2009; Axmacher et al., 2010; Cohen, 2011). Interestingly, LTM processes are also governed by similar synchronization mechanisms (Nyhus and Curran, 2010; Fell and Axmacher, 2011). However, no studies have directly addressed how phase synchronization during WM modulate subsequent LTM recognition (Fell and Axmacher, 2011) or the patterns of phase synchronization during different states of information processing under proceduralist models (Nee and Jonides, 2011). Furthermore, the precise role of synchrony in support of inter-neuronal communication is far from being well understood (Aru et al., 2011; Fell and Axmacher, 2011; Gordon, 2011). Oscillatory coherence has been associated with several cognitive processes, from perceptual binding (Singer and Gray, 1995) to rule learning (Benchenane et al., 2010), and, therefore, new experiments should explore the functional role of neural synchronization in WM and in WM-LTM interplay (Jutras and Buffalo, 2010; Benchenane et al., 2011). Future studies should also explore how functional networks supporting WM interact with the anatomical network infrastructure (Guye et al., 2008; He and Evans, 2010; Cohen, 2011). Additionally, greater understanding of the functional significance of neural synchronization would be obtained by investigating the relationship between neuromodulators and oscillatory coupling (Cartling, 2001; Takahashi et al., 2008; Haenschel and Linden, 2011; Young, 2011).
Finally, we have also seen how abnormalities in functional connectivity arising from more or less localized lesions can contribute to WM dysfunction observed in mTLE, AD, and schizophrenia. Particularly, abnormal communication between MTL and PFC has been observed after damage to one of these structures, probably due to tight structural and functional connection between them (Brockmann et al., 2011). The reviewed findings add to a growing body of evidence further supporting the involvement of MTL in the neural network underlying WM. Characterizing dysfunctional networks not only provide a better understanding of a variety of disorders, but also critical inferences about the functional dynamics between particular elements of the WM network. Relating patterns of altered oscillatory synchronization with histologically mapped patterns of cell loss (i.e., different degrees of cell death in CA1 in mTLE) could help in increasing our knowledge about the mechanisms mediating neural communication during WM (Colgin et al., 2009; Small et al., 2011). Exploring the development of distinct patterns of connectivity following damage to the network underlying WM could help to develop better diagnostic and prognostic tools. Evaluation of the integrity of memory structures in the presurgical evaluation of patients with mTLE, and prognosis of surgical outcome, could benefit from network analysis. In pathologies such as AD, with a clear progress of dysfunction with time, the use of a longitudinal approach could increase our knowledge about disruptions in the network affecting certain elements in specific stages and its behavioral consequences. Finally, network analysis could provide the neurophysiological basis for evaluating the efficacy of cognitive and pharmacological treatments (Colgin, 2011; Haenschel and Linden, 2011; Kleen et al., 2011).
Conflict of Interest Statement
The authors declare that the research was conducted in the absence of any commercial or financial relationships that could be construed as a potential conflict of interest.
Acknowledgments
This work was supported by a research grant from the Spanish Ministry of Science and Innovation (Grant PSI2010–16742) to Pablo Campo. Claudia Poch was supported by Spanish Ministry of Science and Education (AP2009–4131). Pablo Campo was supported by a Ramon y Cajal Fellowship from the Spanish Ministry of Science and Innovation (RYC-2010–05748).
References
Abrahams, S., Morris, R. G., Polkey, C. E., Jarosz, J. M., Cox, T. C., Graves, M., and Pickering, A. (1999). Hippocampal involvement in spatial and working memory: a structural MRI analysis of patients with unilateral mesial temporal lobe sclerosis. Brain Cogn. 41, 39–65.
Addis, D. R., and McAndrews, M. P. (2006). Prefrontal and hippocampal contributions to the generation and binding of semantic associations during successful encoding. Neuroimage 33, 1194–1206.
Anderson, K. L., Rajagovindan, R., Ghacibeh, G. A., Meador, K. J., and Ding, M. (2010). Theta oscillations mediate interaction between prefrontal cortex and medial temporal lobe in human memory. Cereb. Cortex 20, 1604–1612.
Anticevic, A., Repovs, G., and Barch, D. M. (in press). Working memory encoding and maintenance deficits in schizophrenia: neural evidence for activation and deactivation abnormalities. Schizophr. Bull.
Arnsten, A. F., Paspalas, C. D., Gamo, N. J., Yang, Y., and Wang, M. (2010). Dynamic network connectivity: a new form of neuroplasticity. Trends Cogn. Sci. 14, 365–375.
Aru, J., Aru, J., Wibral, M., Priessemann, V., Singer, W., and Vicente, R. (2011). Analyzing possible pitfalls of cross-frequency analysis. BMC Neurosci. 12, 303.
Axmacher, N., Elger, C. E., and Fell, J. (2009a). Working memory-related hippocampal deactivation interferes with long-term memory formation. J. Neurosci. 29, 1052–1960.
Axmacher, N., Haupt, S., Cohen, M. X., Elger, C. E., and Fell, J. (2009b). Interference of working memory load with long-term memory formation. Eur. J. Neurosci. 29, 1501–1513.
Axmacher, N., Henseler, M. M., Jensen, O., Weinreich, I., Elger, C. E., and Fell, J. (2010). Cross-frequency coupling supports multi-item working memory in the human hippocampus. Proc. Natl. Acad. Sci. U.S.A. 107, 3228–3233.
Axmacher, N., Mormann, F., Fernandez, G., Cohen, M. X., Elger, C. E., and Fell, J. (2007). Sustained neural activity patterns during working memory in the human medial temporal lobe. J. Neurosci. 27, 7807–7816.
Axmacher, N., Schmitz, D. P., Wagner, T., Elger, C. E., and Fell, J. (2008a). Interactions between medial temporal lobe, prefrontal cortex, and inferior temporal regions during visual working memory: a combined intracranial EEG and functional magnetic resonance imaging study. J. Neurosci. 28, 7304–7312.
Axmacher, N., Schmitz, D. P., Weinreich, I., Elger, C. E., and Fell, J. (2008b). Interaction of working memory and long-term memory in the medial temporal lobe. Cereb. Cortex 18, 2868–2878.
Babiloni, C., Ferri, R., Binetti, G., Cassarino, A., Dal Forno, G., Ercolani, M., Ferreri, F., Frisoni, G. B., Lanuzza, B., Miniussi, C., Nobili, F., Rodriguez, G., Rundo, F., Stam, C. J., Musha, T., Vecchio, F., and Rossini, P. M. (2006). Fronto-parietal coupling of brain rhythms in mild cognitive impairment: a multicentric EEG study. Brain Res. Bull. 69, 63–73.
Babiloni, C., Vecchio, F., Mirabella, G., Buttiglione, M., Sebastiano, F., Picardi, A., Di Gennaro, G., Quarato, P. P., Grammaldo, L. G., Buffo, P., Esposito, V., Manfredi, M., Cantore, G., and Eusebi, F. (2009). Hippocampal, amygdala, and neocortical synchronization of theta rhythms is related to an immediate recall during rey auditory verbal learning test. Hum. Brain Mapp. 30, 2077–2089.
Bai, F., Zhang, Z., Watson, D. R., Yu, H., Shi, Y., Yuan, Y., Zang, Y., Zhu, C., and Qian, Y. (2009). Abnormal functional connectivity of hippocampus during episodic memory retrieval processing network in amnestic mild cognitive impairment. Biol. Psychiatry 65, 951–958.
Bajo, R., Maestu, F., Nevado, A., Sancho, M., Gutierrez, R., Campo, P., Castellanos, N. P., Gil, P., Moratti, S., Pereda, E., and Del-Pozo, F. (2010). Functional connectivity in mild cognitive impairment during a memory task: implications for the disconnection hypothesis. J. Alzheimers Dis. 22, 183–193.
Banyai, M., Diwadkar, V. A., and Erdi, P. (2011). Model-based dynamical analysis of functional disconnection in schizophrenia. Neuroimage 58, 870–877.
Bassett, D. S., Bullmore, E. T., Meyer-Lindenberg, A., Apud, J. A., Weinberger, D. R., and Coppola, R. (2009). Cognitive fitness of cost-efficient brain functional networks. Proc. Natl. Acad. Sci. U.S.A. 106, 11747–11752.
Battaglia, F. P., Benchenane, K., Sirota, A., Pennartz, C. M., and Wiener, S. I. (2011). The hippocampus: hub of brain network communication for memory. Trends Cogn. Sci. 15, 310–318.
Benchenane, K., Peyrache, A., Khamassi, M., Tierney, P. L., Gioanni, Y., Battaglia, F. P., and Wiener, S. I. (2010). Coherent theta oscillations and reorganization of spike timing in the hippocampal- prefrontal network upon learning. Neuron 66, 921–936.
Benchenane, K., Tiesinga, P. H., and Battaglia, F. P. (2011). Oscillations in the prefrontal cortex: a gateway to memory and attention. Curr. Opin. Neurobiol. 21, 475–485.
Benetti, S., Mechelli, A., Picchioni, M., Broome, M., Williams, S., and McGuire, P. (2009). Functional integration between the posterior hippocampus and prefrontal cortex is impaired in both first episode schizophrenia and the at risk mental state. Brain 132, 2426–2436.
Bettus, G., Guedj, E., Joyeux, F., Confort-Gouny, S., Soulier, E., Laguitton, V., Cozzone, P. J., Chauvel, P., Ranjeva, J. P., Bartolomei, F., and Guye, M. (2009). Decreased basal fMRI functional connectivity in epileptogenic networks and contralateral compensatory mechanisms. Hum. Brain Mapp. 30, 1580–1591.
Bledowski, C., Rahm, B., and Rowe, J. B. (2009). What “works” in working memory? Separate systems for selection and updating of critical information. J. Neurosci. 29, 13735–13741.
Bonilha, L., Molnar, C., Horner, M. D., Anderson, B., Forster, L., George, M. S., and Nahas, Z. (2008). Neurocognitive deficits and prefrontal cortical atrophy in patients with schizophrenia. Schizophr. Res. 101, 142–151.
Braak, H., and Braak, E. (1997). Frequency of stages of Alzheimer-related lesions in different age categories. Neurobiol. Aging 18, 351–357.
Braun, M., Finke, C., Ostendorf, F., Lehmann, T. N., Hoffmann, K. T., and Ploner, C. J. (2008). Reorganization of associative memory in humans with long-standing hippocampal damage. Brain 131, 2742–2750.
Braver, T. S., Cohen, J. D., Nystrom, L. E., Jonides, J., Smith, E. E., and Noll, D. C. (1997). A parametric study of prefrontal cortex involvement in human working memory. Neuroimage 5, 49–62.
Bressler, S. L., and Menon, V. (2010). Large-scale brain networks in cognition: emerging methods and principles. Trends Cogn. Sci. 277–290.
Brockmann, M. D., Poschel, B., Cichon, N., and Hanganu-Opatz, I. L. (2011). Coupled oscillations mediate directed interactions between prefrontal cortex and hippocampus of the neonatal rat. Neuron 71, 332–347.
Buckner, R. L., Sepulcre, J., Talukdar, T., Krienen, F. M., Liu, H., Hedden, T., Andrews-Hanna, J. R., Sperling, R. A., and Johnson, K. A. (2009). Cortical hubs revealed by intrinsic functional connectivity: mapping, assessment of stability, and relation to Alzheimer's disease. J. Neurosci. 29, 1860–1873.
Buchsbaum, B. R., Padmanabhan, A., and Berman, K. F. (2010). The neural substrates of recognition memory for verbal information: spanning the divide between short- and long-term memory. J. Cogn. Neurosci. 23, 978–991.
Bullmore, E., and Sporns, O. (2009). Complex brain networks: graph theoretical analysis of structural and functional systems. Nat. Rev. Neurosci. 10, 186–198.
Campo, P., Garrido, M. I., Moran, R. J., Maestu, F., Garcia-Morales, I., Gil-Nagel, A., Del Pozo, F., Dolan, R. J., and Friston, K. J. (in press). Remote effects of hippocampal sclerosis on effective connectivity during working memory encoding: a case of connectional diaschisis? Cereb. Cortex.
Campo, P., Maestu, F., Capilla, A., Fernandez, S., Fernandez, A., and Ortiz, T. (2005b). Activity in human medial temporal lobe associated with encoding process in spatial working memory revealed by magnetoencephalography. Eur. J. Neurosci. 21, 1741–1748.
Campo, P., Maestu, F., Garcia-Morales, I., Gil-Nagel, A., Strange, B., Morales, M., and Ortiz, T. (2009). Modulation of medial temporal lobe activity in epilepsy patients with hippocampal sclerosis during verbal working memory. J. Int. Neuropsychol. Soc. 15, 536–546.
Campo, P., Maestu, F., Ortiz, T., Capilla, A., Fernandez, S., and Fernandez, A. (2005a). Is medial temporal lobe activation specific for encoding long-term memories? Neuroimage 25, 34–42.
Canolty, R. T., and Knight, R. T. (2010). The functional role of cross-frequency coupling. Trends Cogn. Sci. 14, 506–515.
Carlesimo, G. A., Perri, R., Turriziani, P., Tomaiuolo, F., and Caltagirone, C. (2001). Remembering what but not where: independence of spatial and visual working memory in the human brain. Cortex 37, 519–534.
Carrozzo, M., Koch, G., Turriziani, P., Caltagirone, C., Carlesimo, G. A., and Lacquaniti, F. (2005). Integration of cognitive allocentric information in visuospatial short-term memory through the hippocampus. Hippocampus 15, 1072–1084.
Cartling, B. (2001). Neuromodulatory control of interacting medial temporal lobe and neocortex in memory consolidation and working memory. Behav. Brain Res. 126, 65–80.
Cashdollar, N., Duncan, J. S., and Düzel, E. (2011). Challenging the classical distinction between long-term and short-term memory: reconsidering the role of the hippocampus. Future Neurol. 6, 351–362.
Cashdollar, N., Malecki, U., Rugg-Gunn, F. J., Duncan, J. S., Lavie, N., and Duzel, E. (2009). Hippocampus-dependent and -independent theta-networks of active maintenance. Proc. Natl. Acad. Sci. U.S.A. 106, 20493–20498.
Castellanos, N. P., Bajo, R., Cuesta, P., Villacorta-Atienza, J. A., Paul, N., Garcia-Prieto, J., Del-Pozo, F., and Maestu, F. (2011). Alteration and reorganization of functional networks: a new perspective in brain injury study. Front. Hum. Neurosci. 5:90. doi: 10.3389/fnhum.2011.00090
Cohen, M. X. (2011). Hippocampal-prefrontal connectivity predicts midfrontal oscillations and long-term memory performance. Curr. Biol. 21, 1900–1905.
Cohen, M. X., Wilmes, K., and van andde Vijver, I. (2011). Cortical electrophysiological network dynamics of feedback learning. Trends Cogn. Sci. 15, 558–566.
Cole, M. W., Anticevic, A., Repovs, G., and Barch, D. (2011). Variable global dysconnectivity and individual differences in schizophrenia. Biol. Psychiatry 70, 43–50.
Colgin, L. L. (2011). Oscillations and hippocampal-prefrontal synchrony. Curr. Opin. Neurobiol. 21, 467–474.
Colgin, L. L., Denninger, T., Fyhn, M., Hafting, T., Bonnevie, T., Jensen, O., Moser, M. B., and Moser, E. I. (2009). Frequency of gamma oscillations routes flow of information in the hippocampus. Nature 462, 353–357.
Cowan, N. (2001). The magical number 4 in short-term memory: a reconsideration of mental storage capacity. Behav. Brain Sci. 24, 87–114; discussion 114–185.
Crossley, N. A., Mechelli, A., Fusar-Poli, P., Broome, M. R., Matthiasson, P., Johns, L. C., Bramon, E., Valmaggia, L., Williams, S. C., and McGuire, P. K. (2009). Superior temporal lobe dysfunction and frontotemporal dysconnectivity in subjects at risk of psychosis and in first-episode psychosis. Hum. Brain Mapp. 30, 4129–4137.
Chapman, C. A., Jones, R. S., and Jung, M. (2008). Neuronal plasticity in the entorhinal cortex. Neural Plast. 2008, 314785.
Cho, R. Y., Konecky, R. O., and Carter, C. S. (2006). Impairments in frontal cortical gamma synchrony and cognitive control in schizophrenia. Proc. Natl. Acad. Sci. U.S.A. 103, 19878–19883.
Churchwell, J. C., and Kesner, R. P. (2011). Hippocampal-prefrontal dynamics in spatial working memory: interactions and independent parallel processing. Behav. Brain Res. 225, 389–395.
D'Esposito, M. (2007). From cognitive to neural models of working memory. Philos. Trans. R. Soc. Lond. B Biol. Sci. 362, 761–772.
D'Esposito, M., Postle, B. R., and Rypma, B. (2000). Prefrontal cortical contributions to working memory: evidence from event-related fMRI studies. Exp. Brain Res. 133, 3–11.
Davachi, L. (2006). Item, context and relational episodic encoding in humans. Curr. Opin. Neurobiol. 16, 693–700.
Druzgal, T. J., and D'Esposito, M. (2001). Activity in fusiform face area modulated as a function of working memory load. Brain Res. Cogn. Brain Res. 10, 355–364.
Düzel, E., Penny, W. D., and Burgess, N. (2010). Brain oscillations and memory. Curr. Opin. Neurobiol. 20, 1–7.
Duzel, E., Penny, W. D., and Burgess, N. (2010). Brain oscillations and memory. Curr. Opin. Neurobiol. 20, 143–149.
Edin, F., Klingberg, T., Johansson, P., McNab, F., Tegner, J., and Compte, A. (2009). Mechanism for top-down control of working memory capacity. Proc. Natl. Acad. Sci. U.S.A. 106, 6802–6807.
Elliott, R., and Dolan, R. J. (1999). Differential neural responses during performance of matching and nonmatching to sample tasks at two delay intervals. J. Neurosci. 19, 5066–5073.
Ellison-Wright, I., and Bullmore, E. (2009). Meta-analysis of diffusion tensor imaging studies in schizophrenia. Schizophr. Res. 108, 3–10.
Engel, A. K., Fries, P., and Singer, W. (2001). Dynamic predictions: oscillations and synchrony in top-down processing. Nat. Rev. Neurosci. 2, 704–716.
Esslinger, C., Walter, H., Kirsch, P., Erk, S., Schnell, K., Arnold, C., Haddad, L., Mier, D., Opitzvon Boberfeld, C., Raab, K., Witt, S. H., Rietschel, M., Cichon, S., and Meyer-Lindenberg, A. (2009). Neural mechanisms of a genome-wide supported psychosis variant. Science 324, 605.
Faraco, C. C., Unsworth, N., Langley, J., Terry, D., Li, K., Zhang, D., Liu, T., and Miller, L. S. (2011). Complex span tasks and hippocampal recruitment during working memory. Neuroimage 55, 773–787.
Fell, J., and Axmacher, N. (2011). The role of phase synchronization in memory processes. Nat. Rev. Neurosci. 12, 105–118.
Fell, J., Klaver, P., Lehnertz, K., Grunwald, T., Schaller, C., Elger, C. E., and Fernandez, G. (2001). Human memory formation is accompanied by rhinal-hippocampal coupling and decoupling. Nat. Neurosci. 4, 1259–1264.
Feredoes, E., Heinen, K., Weiskopf, N., Ruff, C., and Driver, J. (2011). Causal evidence for frontal involvement in memory target maintenance by posterior brain areas during distracter interference of visual working memory. Proc. Natl. Acad. Sci. U.S.A. 108, 17510–17515.
Fiebach, C. J., Friederici, A. D., Smith, E. E., and Swinney, D. (2007). Lateral inferotemporal cortex maintains conceptual-semantic representations in verbal working memory. J. Cogn. Neurosci. 19, 2035–2049.
Fiebach, C. J., Rissman, J., and D'Esposito, M. (2006). Modulation of inferotemporal cortex activation during verbal working memory maintenance. Neuron 51, 251–261.
Finn, A. S., Sheridan, M. A., Kam, C. L., Hinshaw, S., and D'Esposito, M. (2010). Longitudinal evidence for functional specialization of the neural circuit supporting working memory in the human brain. J. Neurosci. 30, 11062–11067.
Floresco, S. B., Seamans, J. K., and Phillips, A. G. (1997). Selective roles for hippocampal, prefrontal cortical, and ventral striatal circuits in radial-arm maze tasks with or without a delay. J. Neurosci. 17, 1880–1890.
Fornito, A., Yoon, J., Zalesky, A., Bullmore, E. T., and Carter, C. S. (2011). General and specific functional connectivity disturbances in first-episode schizophrenia during cognitive control performance. Biol. Psychiatry 70, 64–72.
Fransén, E. A. (2005). Functional role of entorhinal cortex in working memory and information processing of the medial temporal lobe. Neural Netw. 18, 1141–1149.
Freunberger, R., Werkle-Bergner, M., Griesmayr, B., Lindenberger, U., and Klimesch, W. (2011). Brain oscillatory correlates of working memory constraints. Brain Res. 1375, 93–102.
Fries, P. (2005). A mechanism for cognitive dynamics: neuronal communication through neuronal coherence. Trends Cogn. Sci. 9, 474–480.
Friston, K. (2009). Causal modelling and brain connectivity in functional magnetic resonance imaging. PLoS Biol. 7, 220–225. doi: 10.1371/journal.pbio.1000033
Friston, K. J. (1999). Schizophrenia and the disconnection hypothesis. Acta Psychiatr. Scand. Suppl. 395, 68–79.
Friston, K. J., Harrison, L., and Penny, W. (2003). Dynamic causal modelling. Neuroimage 19, 1273–1302.
Fuentemilla, L., Penny, W. D., Cashdollar, N., Bunzeck, N., and Duzel, E. (2010). Theta-coupled periodic replay in working memory. Curr. Biol. 20, 606–612.
Fujisawa, S., and Buzsaki, G. (2011). A 4 Hz oscillation adaptively synchronizes prefrontal, VTA, and hippocampal activities. Neuron 72, 153–165.
Gazzaley, A., Rissman, J., Cooney, J., Rutman, A., Seibert, T., Clapp, W., and D'Esposito, M. (2007). Functional interactions between prefrontal and visual association cortex contribute to top-down modulation of visual processing. Cereb. Cortex 17(Suppl. 1), i125-i135.
Gazzaley, A., Rissman, J., and Desposito, M. (2004). Functional connectivity during working memory maintenance. Cogn. Affect. Behav. Neurosci. 4, 580–599.
Ginestet, C. E., and Simmons, A. (2010). Statistical parametric network analysis of functional connectivity dynamics during a working memory task. Neuroimage 55, 688–704.
Goldman-Rakic, P. S. (1994). Working memory dysfunction in schizophrenia. J. Neuropsychiatry Clin. Neurosci. 6, 348–357.
Goldman-Rakic, P. S. (1995). Architecture of the prefrontal cortex and the central executive. Ann. N.Y. Acad. Sci. 769, 71–83.
Gordon, J. A. (2011). Oscillations and hippocampal-prefrontal synchrony. Curr. Opin. Neurobiol. 21, 486–491.
Grady, C. L., Furey, M. L., Pietrini, P., Horwitz, B., and Rapoport, S. I. (2001). Altered brain functional connectivity and impaired short-term memory in Alzheimer's disease. Brain 124, 739–756.
Guye, M., Bartolomei, F., and Ranjeva, J. P. (2008). Imaging structural and functional connectivity: towards a unified definition of human brain organization? Curr. Opin. Neurol. 21, 393–403.
Haenschel, C., and Linden, D. (2011). Exploring intermediate phenotypes with EEG: working memory dysfunction in schizophrenia. Behav. Brain Res. 216, 481–495.
Hashimoto, R., Lee, K., Preus, A., McCarley, R. W., and Wible, C. G. (2010). An fMRI study of functional abnormalities in the verbal working memory system and the relationship to clinical symptoms in chronic schizophrenia. Cereb. Cortex 20, 46–60.
Haxby, J. V., Ungerleider, L. G., Horwitz, B., Rapoport, S. I., and Grady, C. L. (1995). Hemispheric differences in neural systems for face working memory: a PET-rCBF study. Hum. Brain Mapp. 3, 68–82.
He, Y., and Evans, A. (2010). Graph theoretical modeling of brain connectivity. Curr. Opin. Neurol. 23, 341–350.
Henseler, I., Falkai, P., and Gruber, O. (2010). Disturbed functional connectivity within brain networks subserving domain-specific subcomponents of working memory in schizophrenia: relation to performance and clinical symptoms. J. Psychiatr. Res. 44, 364–372.
Hyman, J. M., Zilli, E. A., Paley, A. M., and Hasselmo, M. E. (2010). Working memory performance correlates with prefrontal-hippocampal theta interactions but not with prefrontal neuron firing rates. Front. Integr. Neurosci. 4:2. doi: 10.3389/neuro.07.002.2010
Jeneson, A., Mauldin, K. N., and Squire, L. R. (2011). Intact working memory for relational information after medial temporal lobe damage. J. Neurosci. 30, 13624–13629.
Jensen, O., and Lisman, J. E. (1998). An oscillatory short-term memory buffer model can account for data on the Sternberg task. J. Neurosci. 18, 10688–10699.
Jones, M. W., and Wilson, M. A. (2005). Theta rhythms coordinate hippocampal-prefrontal interactions in a spatial memory task. PLoS Biol. 3:e402. doi: 10.1371/journal.pbio.0030402
Jutras, M. J., and Buffalo, E. A. (2010). Synchronous neural activity and memory formation. Curr. Opin. Neurobiol. 20, 150–155.
Kahn, I., Andrews-Hanna, J. R., Vincent, J. L., Snyder, A. Z., and Buckner, R. L. (2008). Distinct cortical anatomy linked to subregions of the medial temporal lobe revealed by intrinsic functional connectivity. J. Neurophysiol. 100, 129–139.
Kang, S. S., Sponheim, S. R., Chafee, M. V., and MacDonald, A. W. 3rd. (2011). Disrupted functional connectivity for controlled visual processing as a basis for impaired spatial working memory in schizophrenia. Neuropsychologia 49, 2836–2847.
Karlsgodt, K. H., Shirinyan, D., van Erp, T. G., Cohen, M. S., and Cannon, T. D. (2005). Hippocampal activations during encoding and retrieval in a verbal working memory paradigm. Neuroimage 25, 1224–1231.
Karlsgodt, K. H., van Erp, T. G., Poldrack, R. A., Bearden, C. E., Nuechterlein, K. H., and Cannon, T. D. (2008). Diffusion tensor imaging of the superior longitudinal fasciculus and working memory in recent-onset schizophrenia. Biol. Psychiatry 63, 512–518.
Khader, P., Ranganath, C., Seemuller, A., and Rosler, F. (2007). Working memory maintenance contributes to long-term memory formation: evidence from slow event-related brain potentials. Cogn. Affect. Behav. Neurosci. 7, 212–224.
Kim, J. J., Kwon, J. S., Park, H. J., Youn, T., Kang, D. H., Kim, M. S., Lee, D. S., and Lee, M. C. (2003). Functional disconnection between the prefrontal and parietal cortices during working memory processing in schizophrenia: a15(O)H2O PET study. Am. J. Psychiatry 160, 919–923.
Kleen, J. K., Wu, E. X., Holmes, G. L., Scott, R. C., and Lenck-Santini, P. P. (2011). Enhanced oscillatory activity in the hippocampal-prefrontal network is related to short-term memory function after early-life seizures. J. Neurosci. 31, 15397–15406.
Lee, A. C., and Rudebeck, S. R. (2011). Investigating the interaction between spatial perception and working memory in the human medial temporal lobe. J. Cogn. Neurosci. 22, 2823–2835.
Lee, I., and Kesner, R. P. (2003). Time-dependent relationship between the dorsal hippocampus and the prefrontal cortex in spatial memory. J. Neurosci. 23, 1517–1523.
Lee, J., and Park, S. (2005). Working memory impairments in schizophrenia: a meta-analysis. J. Abnorm. Psychol. 114, 599–611.
Lewis-Peacock, J. A., Drysdale, A. T., Oberauer, K., and Postle, B. R. (2012). Neural evidence for a distinction between short-term memory and the focus of attention. J. Cogn. Neurosci. 24, 61–79.
Lewis, D. A., and Gonzalez-Burgos, G. (2006). Pathophysiologically based treatment interventions in schizophrenia. Nat. Med. 12, 1016–1022.
Liang, P., Wang, Z., Yang, Y., Jia, X., and Li, K. (2011). Functional disconnection and compensation in mild cognitive impairment: evidence from DLPFC connectivity using resting-state fMRI. PLoS One 6:e22153. doi: 10.1371/journal.pone.0022153
Liao, W., Zhang, Z., Pan, Z., Mantini, D., Ding, J., Duan, X., Luo, C., Lu, G., and Chen, H. (2010). Altered functional connectivity and small-world in mesial temporal lobe epilepsy. PLoS One 5:e8525. doi: 10.1371/journal.pone.0008525
Liao, W., Zhang, Z., Pan, Z., Mantini, D., Ding, J., Duan, X., Luo, C., Wang, Z., Tan, Q., Lu, G., and Chen, H. (2011). Default mode network abnormalities in mesial temporal lobe epilepsy: a study combining fMRI and DTI. Hum. Brain Mapp. 32, 883–895.
Lisman, J. (2005). The theta/gamma discrete phase code occuring during the hippocampal phase precession may be a more general brain coding scheme. Hippocampus 15, 913–922.
Lisman, J. E., and Idiart, M. A. (1995). Storage of 7 +/− 2 short-term memories in oscillatory subcycles. Science 267, 1512–1515.
Lundqvist, M., Herman, P., and Lansner, A. (2011). Theta and gamma power increases and alpha/beta power decreases with memory load in an attractor network model. J. Cogn. Neurosci. 23, 3008–3020.
Lustig, C. A., Lewis, R. L., Berman, M. G., Nee, D. E., Moore, K. S., and Jonides, J. (2009). “Psychological and Neural Mechanisms of Short-term Memory,” in Handbook of Neuroscience for the Behavioral Sciences, eds G. G. Berntson and J. T. Cacioppo (New Jersey: John Wiley and Sons), 567–585.
Lynall, M. E., Bassett, D. S., Kerwin, R., McKenna, P. J., Kitzbichler, M., Muller, U., and Bullmore, E. (2010). Functional connectivity and brain networks in schizophrenia. J. Neurosci. 30, 9477–9487.
Manoach, D. S. (2003). Prefrontal cortex dysfunction during working memory performance in schizophrenia: reconciling discrepant findings. Schizophr. Res. 60, 285–298.
McElree, B. (2006). “Accessing recent events,” in The Psychology of Learning and Motivation, ed B. H. Ross (San Diego, CA: Academic Press), 155–200.
McIntosh, A. R. (1999). Mapping cognition to the brain through neural interactions. Memory 7, 523–548.
McIntosh, A. R., Grady, C. L., Haxby, J. V., Ungerleider, L. G., and Horwitz, B. (1996). Changes in limbic and prefrontal functional interactions in a working memory task for faces. Cereb. Cortex 6, 571–584.
Meda, S. A., Stevens, M. C., Folley, B. S., Calhoun, V. D., and Pearlson, G. D. (2009). Evidence for anomalous network connectivity during working memory encoding in schizophrenia: an ICA based analysis. PLoS One 4:e7911. doi: 10.1371/journal.pone.0007911
Menon, V. (2011). Large-scale brain networks and psychopathology: a unifying triple network model. Trends Cogn. Sci. 15, 483–506.
Meyer-Lindenberg, A., Poline, J. B., Kohn, P. D., Holt, J. L., Egan, M. F., Weinberger, D. R., and Berman, K. F. (2001). Evidence for abnormal cortical functional connectivity during working memory in schizophrenia. Am. J. Psychiatry 158, 1809–1817.
Meyer-Lindenberg, A. S., Olsen, R. K., Kohn, P. D., Brown, T., Egan, M. F., Weinberger, D. R., and Berman, K. F. (2005). Regionally specific disturbance of dorsolateral prefrontal-hippocampal functional connectivity in schizophrenia. Arch. Gen. Psychiatry 62, 379–386.
Mitchell, D. J., McNaughton, N., Flanagan, D., and Kirk, I. J. (2008). Frontal-midline theta from the perspective of hippocampal “theta”. Prog. Neurobiol. 86, 156–185.
Moran, R. J., Campo, P., Maestu, F., Reilly, R. B., Dolan, R. J., and Strange, B. A. (2010). Peak frequency in the theta and alpha bands correlates with human working memory capacity. Front. Hum. Neurosci. 4:200. doi: 10.3389/fnhum.2010.00200
Nairne, J. S. (2002). Remembering over the short-term: the case against the standard model. Annu. Rev. Psychol. 53, 53–81.
Nee, D. E., and Jonides, J. (2008). Neural correlates of access to short-term memory. Proc. Natl. Acad. Sci. U.S.A. 105, 14228–14233.
Nee, D. E., and Jonides, J. (2011). Dissociable contributions of prefrontal cortex and the hippocampus to short-term memory: evidence for a 3-state model of memory. Neuroimage 54, 1540–1548.
Nickl-Jockschat, T., Kleiman, A., Schulz, J. B., Schneider, F., Laird, A. R., Fox, P. T., Eickhoff, S. B., and Reetz, K. (2012). Neuroanatomic changes and their association with cognitive decline in mild cognitive impairment: a meta-analysis. Brain Struct. Funct. 215, 115–125.
Nichols, E. A., Kao, Y. C., Verfaellie, M., and Gabrieli, J. D. (2006). Working memory and long-term memory for faces: evidence from fMRI and global amnesia for involvement of the medial temporal lobes. Hippocampus 16, 604–616.
Nyhus, E., and Curran, T. (2010). Functional role of gamma and theta oscillations in episodic memory. Neurosci. Biobehav. Rev. 34, 1023–1035.
Oberauer, K. (2002). Access to information in working memory: exploring the focus of attention. J. Exp. Psychol. Learn. Mem. Cogn. 28, 411–421.
Olsen, R. K., Nichols, E. A., Chen, J., Hunt, J. F., Glover, G. H., Gabrieli, J. D., and Wagner, A. D. (2009). Performance-related sustained and anticipatory activity in human medial temporal lobe during delayed match-to-sample. J. Neurosci. 29, 11880–11890.
Olson, I. R., Moore, K. S., Stark, M., and Chatterjee, A. (2006). Visual working memory is impaired when the medial temporal lobe is damaged. J. Cogn. Neurosci. 18, 1087–1097.
Owen, A. M., Morris, R. G., Sahakian, B. J., Polkey, C. E., and Robbins, T. W. (1996). Double dissociations of memory and executive functions in working memory tasks following frontal lobe excisions, temporal lobe excisions or amygdalo-hippocampectomy in man. Brain 119(Pt 5), 1597–1615.
Öztekin, I., Davachi, L., and McElree, B. (2010). Are representations in working memory distinct from representations in long-term memory? Neural evidence in support of a single store. Psychol. Sci. 21, 1123–1133.
Pasternak, T., and Greenlee, M. W. (2005). Working memory in primate sensory systems. Nat. Rev. Neurosci. 6, 97–107.
Payne, L., and Kounios, J. (2009). Coherent oscillatory networks supporting short-term memory retention. Brain Res. 1247, 126–132.
Penny, W. D., Stephan, K. E., Mechelli, A., and Friston, K. J. (2004). Comparing dynamic causal models. Neuroimage 22, 1157–1172.
Penny, W. D., Litvak, V., Fuentemilla, L., Duzel, E., and Friston, K. (2009). Dynamic causal models for phase coupling. J. Neurosci. Methods 183, 19–30.
Pereira, F. R., Alessio, A., Sercheli, M. S., Pedro, T., Bilevicius, E., Rondina, J. M., Ozelo, H. F., Castellano, G., Covolan, R. J., Damasceno, B. P., and Cendes, F. (2010). Asymmetrical hippocampal connectivity in mesial temporal lobe epilepsy: evidence from resting state fMRI. BMC Neurosci. 11, 66.
Perlstein, W. M., Carter, C. S., Noll, D. C., and Cohen, J. D. (2001). Relation of prefrontal cortex dysfunction to working memory and symptoms in schizophrenia. Am. J. Psychiatry 158, 1105–1113.
Petersson, K. M., Gisselgard, J., Gretzer, M., and Ingvar, M. (2006). Interaction between a verbal working memory network and the medial temporal lobe. Neuroimage 33, 1207–1217.
Picchioni, M., Matthiasson, P., Broome, M., Giampietro, V., Brammer, M., Mathes, B., Fletcher, P., Williams, S., and McGuire, P. (2007). Medial temporal lobe activity at recognition increases with the duration of mnemonic delay during an object working memory task. Hum. Brain Mapp. 28, 1235–1250.
Pievani, M., de Haan, W., Wu, T., Seeley, W. W., and Frisoni, G. B. (2011). Functional network disruption in the degenerative dementias. Lancet Neurol. 10, 829–843.
Poch, C., Fuentemilla, L., Barnes, G. R., and Duzel, E. (2011). Hippocampal theta-phase modulation of replay correlates with configural-relational short-term memory performance. J. Neurosci. 31, 7038–7042.
Pomarol-Clotet, E., Canales-Rodriguez, E. J., Salvador, R., Sarro, S., Gomar, J. J., Vila, F., Ortiz-Gil, J., Iturria-Medina, Y., Capdevila, A., and McKenna, P. J. (2010). Medial prefrontal cortex pathology in schizophrenia as revealed by convergent findings from multimodal imaging. Mol. Psychiatry 15, 823–830.
Postle, B. R., Druzgal, T. J., and D'Esposito, M. (2003). Seeking the neural substrates of visual working memory storage. Cortex 39, 927–946.
Protzner, A. B., and McIntosh, A. R. (2009). Modulation of ventral prefrontal cortex functional connections reflects the interplay of cognitive processes and stimulus characteristics. Cereb. Cortex 19, 1042–1054.
Raghavachari, S., Lisman, J. E., Tully, M., Madsen, J. R., Bromfield, E. B., and Kahana, M. J. (2006). Theta oscillations in human cortex during a working-memory task: evidence for local generators. J. Neurophysiol. 95, 1630–1638.
Raichle, M. E., MacLeod, A. M., Snyder, A. Z., Powers, W. J., Gusnard, D. A., and Shulman, G. L. (2001). A default mode of brain function. Proc. Natl. Acad. Sci. U.S.A. 98, 676–682.
Rajah, M. N., and McIntosh, A. R. (2005). Overlap in the functional neural systems involved in semantic and episodic memory retrieval. J. Cogn. Neurosci. 17, 470–482.
Rajah, M. N., McIntosh, A. R., and Grady, C. L. (1999). Frontotemporal interactions in face encoding and recognition. Brain Res. Cogn. Brain Res. 8, 259–269.
Ranganath, C., and Blumenfeld, R. S. (2005). Doubts about double dissociations between short- and long-term memory. Trends Cogn. Sci. 9, 374–380.
Ranganath, C., Cohen, M. X., and Brozinsky, C. J. (2005a). Working memory maintenance contributes to long-term memory formation: neural and behavioral evidence. J. Cogn. Neurosci. 17, 994–1010.
Ranganath, C., and D'Esposito, M. (2005). Directing the mind's eye: prefrontal, inferior and medial temporal mechanisms for visual working memory. Curr. Opin. Neurobiol. 15, 175–182.
Ranganath, C., Heller, A., Cohen, M. X., Brozinsky, C. J., and Rissman, J. (2005b). Functional connectivity with the hippocampus during successful memory formation. Hippocampus 15, 997–1005.
Renart, A., Moreno, D., dela Rocha, J., Parga, N., and Rolls, E. T. (2001). A model of the IT-PF network in object working memory which includes balanced persistent activity and tuned inhibition. Neurocomputing 38–40, 1525–1531.
Renart, A., Parga, N., and Rolls, E. T. (1999). Associative memory properties of multiple cortical modules. Network 10, 237–255.
Rissman, J., Gazzaley, A., and D'Esposito, M. (2004). Measuring functional connectivity during distinct stages of a cognitive task. Neuroimage 23, 752–763.
Rissman, J., Gazzaley, A., and D'Esposito, M. (2008). Dynamic adjustments in prefrontal, hippocampal, and inferior temporal interactions with increasing visual working memory load. Cereb. Cortex 18, 1618–1629.
Rolls, E. T. (2000). Hippocampo-cortical and cortico-cortical backprojections. Hippocampus 10, 380–388.
Rolls, E. T. (2010a). A computational theory of episodic memory formation in the hippocampus. Behav. Brain Res. 215, 180–196.
Roth, J. K., and Courtney, S. M. (2007). Neural system for updating object working memory from different sources: sensory stimuli or long-term memory. Neuroimage 38, 617–630.
Rowe, J. B. (2010). Connectivity analysis is essential to understand neurological disorders. Front. Syst. Neurosci. 4:144 doi: 10.3389/fnsys.2010.00144
Ruchkin, D. S., Grafman, J., Cameron, K., and Berndt, R. S. (2003). Working memory retention systems: a state of activated long-term memory. Behav. Brain Sci. 26, 709–728; discussion 728–777.
Rypma, B., and D'Esposito, M. (1999). The roles of prefrontal brain regions in components of working memory: effects of memory load and individual differences. Proc. Natl. Acad. Sci. U.S.A. 96, 6558–6563.
Sabuncu, M. R., Desikan, R. S., Sepulcre, J., Yeo, B. T., Liu, H., Schmansky, N. J., Reuter, M., Weiner, M. W., Buckner, R. L., Sperling, R. A., and Fischl, B. (2011). The dynamics of cortical and hippocampal atrophy in Alzheimer disease. Arch. Neurol. 68, 1040–1048.
Sauseng, P., Hoppe, J., Klimesch, W., Gerloff, C., and Hummel, F. C. (2007). Dissociation of sustained attention from central executive functions: local activity and interregional connectivity in the theta range. Eur. J. Neurosci. 25, 587–593.
Sauseng, P., Klimesch, W., Doppelmayr, M., Pecherstorfer, T., Freunberger, R., and Hanslmayr, S. (2005). EEG alpha synchronization and functional coupling during top-down processing in a working memory task. Hum. Brain Mapp. 26, 148–155.
Sauseng, P., Klimesch, W., Heise, K. F., Gruber, W. R., Holz, E., Karim, A. A., Glennon, M., Gerloff, C., Birbaumer, N., and Hummel, F. C. (2009). Brain oscillatory substrates of visual short-term memory capacity. Curr. Biol. 19, 1846–1852.
Schon, K., Hasselmo, M. E., Lopresti, M. L., Tricarico, M. D., and Stern, C. E. (2004). Persistence of parahippocampal representation in the absence of stimulus input enhances long-term encoding: a functional magnetic resonance imaging study of subsequent memory after a delayed match-to-sample task. J. Neurosci. 24, 11088–11097.
Schon, K., Quiroz, Y. T., Hasselmo, M. E., and Stern, C. E. (2009). Greater working memory load results in greater medial temporal activity at retrieval. Cereb. Cortex 19, 2561–2571.
Schwindel, C. D., and McNaughton, B. L. (2011). Hippocampal-cortical interactions and the dynamics of memory trace reactivation. Prog. Brain Res. 193, 163–177.
Seghier, M. L., Zeidman, P., Neufeld, N. H., Leff, A. P., and Price, C. J. (2010). Identifying abnormal connectivity in patients using dynamic causal modeling of FMRI responses. Front. Syst. Neurosci. 4:142 doi: 10.3389/fnsys.2010.00142
Shrager, Y., Levy, D. A., Hopkins, R. O., and Squire, L. R. (2008). Working memory and the organization of brain systems. J. Neurosci. 28, 4818–4822.
Siapas, A. G., Lubenov, E. V., and Wilson, M. A. (2005). Prefrontal phase locking to hippocampal theta oscillations. Neuron 46, 141–151.
Sigurdsson, T., Stark, K. L., Karayiorgou, M., Gogos, J. A., and Gordon, J. A. (2010). Impaired hippocampal-prefrontal synchrony in a genetic mouse model of schizophrenia. Nature 464, 763–767.
Simons, J. S., and Spiers, H. J. (2003). Prefrontal and medial temporal lobe interactions in long-term memory. Nat. Rev. Neurosci. 4, 637–648.
Singer, W., and Gray, C. M. (1995). Visual feature integration and the temporal correlation hypothesis. Annu. Rev. Neurosci. 18, 555–586.
Small, S. A., Schobel, S. A., Buxton, R. B., Witter, M. P., and Barnes, C. A. (2011). A pathophysiological framework of hippocampal dysfunction in ageing and disease. Nat. Rev. Neurosci. 12, 585–601.
Spoletini, I., Cherubini, A., Di Paola, M., Banfi, G., Rusch, N., Martinotti, G., Bria, P., Rubino, I. A., Siracusano, A., Caltagirone, C., and Spalletta, G. (2009). Reduced fronto-temporal connectivity is associated with frontal gray matter density reduction and neuropsychological deficit in schizophrenia. Schizophr. Res. 108, 57–68.
Sporns, O., and Tononi, G. (2007). “Structural determinants of functional brain dynamics,” in Handbook of Brain Connectivity. Understanding Complex Systems, eds V. K. Jirsa and A. R. McIntosh (Berlin: Springer-Verlag), 117–148.
Stam, C. J., Jones, B. F., Manshanden, I., van Cappellen van Walsum, A. M., Montez, T., Verbunt, J. P., de Munck, J. C., van Dijk, B. W., Berendse, H. W., and Scheltens, P. (2006). Magnetoencephalographic evaluation of resting-state functional connectivity in Alzheimer's disease. Neuroimage 32, 1335–1344.
Stephan, K. E., Baldeweg, T., and Friston, K. J. (2006). Synaptic plasticity and dysconnection in schizophrenia. Biol. Psychiatry 59, 929–939.
Stephan, K. E., and Friston, K. J. (2007). “Models of effective connectivity in neural systems,” in Handbook of Brain Connectivity. Undertanding Complex Systems, eds V. K. Jirsa and A. R. McIntosh (Berlin: Springer-Verlag), 303–326.
Summerfield, C., Greene, M., Wager, T., Egner, T., Hirsch, J., and Mangels, J. (2006). Neocortical connectivity during episodic memory formation. PLoS Biol. 4:e128. doi: 10.1371/journal.pbio.0040128
Supekar, K., Menon, V., Rubin, D., Musen, M., and Greicius, M. D. (2008). Network analysis of intrinsic functional brain connectivity in Alzheimer's disease. PLoS Comput. Biol. 4:e1000100. doi: 10.1371/journal.pcbi.1000100
Szatmary, B., and Izhikevich, E. M. (2010). Spike-timing theory of working memory. PLoS Comput. Biol. 6. doi: 10.1371/journal.pcbi.1000879
Takahashi, H., Kato, M., Takano, H., Arakawa, R., Okumura, M., Otsuka, T., Kodaka, F., Hayashi, M., Okubo, Y., Ito, H., and Suhara, T. (2008). Differential contributions of prefrontal and hippocampal dopamine D(1) and D(2) receptors in human cognitive functions. J. Neurosci. 28, 12032–12038.
Talmi, D., Grady, C. L., Goshen-Gottstein, Y., and Moscovitch, M. (2005). Neuroimaging the serial position curve. A test of single-store versus dual-store models. Psychol. Sci. 16, 716–723.
Thal, D. R., Rub, U., Orantes, M., and Braak, H. (2002). Phases of A beta-deposition in the human brain and its relevance for the development of AD. Neurology 58, 1791–1800.
Toepper, M., Markowitsch, H. J., Gebhardt, H., Beblo, T., Thomas, C., Gallhofer, B., Driessen, M., and Sammer, G. (2010). Hippocampal involvement in working memory encoding of changing locations: an fMRI study. Brain Res. 1354, 91–99.
Tudesco Ide, S., Vaz, L. J., Mantoan, M. A., Belzunces, E., Noffs, M. H., Caboclo, L. O., Yacubian, E. M., Sakamoto, A. C., and Bueno, O. F. (2010). Assessment of working memory in patients with mesial temporal lobe epilepsy associated with unilateral hippocampal sclerosis. Epilepsy Behav. 18, 223–228.
Uhlhaas, P. J., and Singer, W. (2006). Neural synchrony in brain disorders: relevance for cognitive dysfunctions and pathophysiology. Neuron 52, 155–168.
Uhlhaas, P. J., and Singer, W. (2010). Abnormal neural oscillations and synchrony in schizophrenia. Nat. Rev. Neurosci. 11, 100–113.
Valdes-Sosa, P. A., Roebroeck, A., Daunizeau, J., and Friston, K. (2011). Effective connectivity: influence, causality and biophysical modeling. Neuroimage 58, 339–361.
Vallar, G., and Papagano, C. (1986). Phonological short-term store and the nature of the recency effect: evidence from neuropsychology. Brain Cogn. 5, 428–442.
Van Snellenberg, J. X. (2009). Working memory and long-term memory deficits in schizophrenia: is there a common substrate? Psychiatry Res. 174, 89–96.
van Vugt, M. K., Schulze-Bonhage, A., Litt, B., Brandt, A., and Kahana, M. J. (2010). Hippocampal gamma oscillations increase with memory load. J. Neurosci. 30, 2694–2699.
Vicente, R., Wibral, M., Lindner, M., and Pipa, G. (2011). Transfer entropy–a model-free measure of effective connectivity for the neurosciences. J. Comput. Neurosci. 30, 45–67.
Vitay, J., and Hamker, F. H. (2008). Sustained activities and retrieval in a computational model of the perirhinal cortex. J. Cogn. Neurosci. 20, 1993–2005.
Wagner, D. D., Sziklas, V., Garver, K. E., and Jones-Gotman, M. (2009). Material-specific lateralization of working memory in the medial temporal lobe. Neuropsychologia 47, 112–122.
Wang, X. J. (2010). Neurophysiological and computational principles of cortical rhythms in cognition. Physiol. Rev. 90, 1195–1268.
Wieser, H. G. (2004). ILAE Commission Report. Mesial temporal lobe epilepsy with hippocampal sclerosis. Epilepsia 45, 695–714.
Winterer, G., Coppola, R., Egan, M. F., Goldberg, T. E., and Weinberger, D. R. (2003). Functional and effective frontotemporal connectivity and genetic risk for schizophrenia. Biol. Psychiatry 54, 1181–1192.
Witter, M. P., Naber, P. A., van Haeften, T., Machielsen, W. C., Rombouts, S. A., Barkhof, F., Scheltens, P., and Lopes andda Silva, F. H. (2000). Cortico-hippocampal communication by way of parallel parahippocampal-subicular pathways. Hippocampus 10, 398–410.
Wolf, R. C., Vasic, N., Sambataro, F., Hose, A., Frasch, K., Schmid, M., and Walter, H. (2009). Temporally anticorrelated brain networks during working memory performance reveal aberrant prefrontal and hippocampal connectivity in patients with schizophrenia. Prog. Neuropsychopharmacol. Biol. Psychiatry 33, 1464–1473.
Woloszyn, L., and Sheinberg, D. L. (2009). Neural dynamics in inferior temporal cortex during a visual working memory task. J. Neurosci. 29, 5494–5507.
Yao, Z., Zhang, Y., Lin, L., Zhou, Y., Xu, C., and Jiang, T. (2010). Abnormal cortical networks in mild cognitive impairment and Alzheimer's disease. PLoS Comput. Biol. 6:e1001006. doi: 10.1371/journal.pcbi.1001006
Yoon, J. H., Curtis, C. E., and D'Esposito, M. (2006). Differential effects of distraction during working memory on delay-period activity in the prefrontal cortex and the visual association cortex. Neuroimage 29, 1117–1126.
Yoon, T., Okada, J., Jung, M. W., and Kim, J. J. (2008). Prefrontal cortex and hippocampus subserve different components of working memory in rats. Learn. Mem. 15, 97–105.
Young, C. K. (2011). Behavioral significance of hippocampal theta oscillations: looking elsewhere to find the right answers. J. Neurophysiol. 106, 497–499.
Zanto, T. P., Rubens, M. T., Thangavel, A., and Gazzaley, A. (2011). Causal role of the prefrontal cortex in top-down modulation of visual processing and working memory. Nat. Neurosci. 14, 656–661.
Zarahn, E., Rakitin, B., Abela, D., Flynn, J., and Stern, Y. (2005). Positive evidence against human hippocampal involvement in working memory maintenance of familiar stimuli. Cereb. Cortex 15, 303–316.
Zeithamova, D., and Preston, A. R. (2010). Flexible memories: differential roles for medial temporal lobe and prefrontal cortex in cross-episode binding. J. Neurosci. 30, 14676–14684.
Keywords: functional connectivity, effective connectivity, working memory, hippocampus, medial temporal lobe, epilepsy, dementia, schizophrenia
Citation: Poch C and Campo P (2012) Neocortical-hippocampal dynamics of working memory in healthy and diseased brain states based on functional connectivity. Front. Hum. Neurosci. 6:36. doi: 10.3389/fnhum.2012.00036
Received: 30 November 2011; Paper pending published: 19 January 2012;
Accepted: 14 February 2012; Published online: 05 March 2012.
Edited by:
Simone Rossi, Azienda Ospedalira Universitaria Senese, ItalyReviewed by:
David Bartrés-Faz, University of Barcelona, SpainPatrizia Turriziani, University of Palermo, Italy
Copyright: © 2012 Poch and Campo. This is an open-access article distributed under the terms of the Creative Commons Attribution Non Commercial License, which permits non-commercial use, distribution, and reproduction in other forums, provided the original authors and source are credited.
*Correspondence: Pablo Campo, Departamento de Psicología Básica, Universidad Autónoma de Madrid, Campus de Cantoblanco, 28049 Madrid, Spain. e-mail: pablo.campo@uam.es