- IRCCS “E. Medea,” San Vito al Tagliamento, Italy
We can predict how an object would look like if we were to see it from different viewpoints. The brain network governing mental rotation (MR) has been studied using a variety of stimuli and tasks instructions. By using activation likelihood estimation (ALE) meta-analysis we tested whether different MR networks can be modulated by the type of stimulus (body vs. non-body parts) or by the type of tasks instructions (motor imagery-based vs. non-motor imagery-based MR instructions). Testing for the bodily and non-bodily stimulus axis revealed a bilateral sensorimotor activation for bodily-related as compared to non-bodily-related stimuli and a posterior right lateralized activation for non-bodily-related as compared to bodily-related stimuli. A top-down modulation of the network was exerted by the MR tasks instructions with a bilateral (preferentially sensorimotor left) network for motor imagery- vs. non-motor imagery-based MR instructions and the latter activating a preferentially posterior right occipito-temporal-parietal network. The present quantitative meta-analysis summarizes and amends previous descriptions of the brain network related to MR and shows how it is modulated by top-down and bottom-up experimental factors.
Introduction
Imagining scenes, sounds and actions, in the absence of appropriate stimuli for the relevant perception, takes place through mental imagery (Kosslyn et al., 1995, 2001). These images can also be combined and modified in novel ways. Mental rotation (hereafter MR) occurs when thinking how an object would look like if seen from a different viewpoint (Shepard and Metzler, 1971; Corballis, 1997).
Processes involved in MR have been studied extensively since Shepard and Metzler (1971) asked participants to decide whether two differently oriented three-dimensional objects were either identical, or mirror images of each other. A proportional relationship was found between the angle of rotation and the time people needed to make a decision. These results suggest that subjects form a visual image of an object and rotate this image until it is congruent with the target stimulus. This pattern has been found with three-dimensional pictures (i.e., 3D cubes), alphanumeric characters (Corballis and Sergent, 1989), abstract pictures, and body parts such as hands (Parsons, 1987; Parsons et al., 1995, 1998; Parsons and Fox, 1998). In addition, RTs for MR of body parts reflect the degree of awkwardness of the picture orientation (Parsons, 1987), because subjects imagine a spatial transformation of their own body part and report kinesthetic sensations (Parsons, 1987).
MR is a complex cognitive task, involving different sub-processes such as object orientation discrimination, visual imagery, mental representation of a stimulus, dynamic spatial transformation of this image, mental comparison, attentional and working memory stages, decision-making and implementation of this decision into a motor output (Kosslyn et al., 1995; Wexler et al., 1998).
Different factors can influence MR operations. In the present study we focused on the effect of the type of stimulus and the effect of instructions which may trigger a specific MR strategy1. With stimulus we mean the type of picture presented on the screen. With strategy we mean the instructions guiding the participants in solving the task. The strategy can be motor imagery-based or visual-imagery based instructions (see in the Method section below some examples). In Table 1 we evidenced in the column “instruction” the strategy given by the experimenters. For instance, all the studies in which the instructions explicitly required participants to imagine hand movements (e.g., “by imagining rotating their own hand into the position of the hand presented”; “simulating a motor rotation of one's own hand”; “MR as a consequence of their hand rotational movement”; see for instance in Vingerhoets et al.'s study (2002): “participants imagined moving both their hands in the hand condition, while imagining manipulating objects with their hand of preference (right hand) in the tool condition”) were included under the category “motor strategy.” All the studies in which the instructions explicitly required participants to imagine the stimulus rotating in the space (e.g., “as a consequence of an external force rotating the object”; see for instance in Barnes et al.'s study (2000): “ […] In the target phase one of the figures was offset and subjects were told to visualize it rotating in a continuous movement until it aligned with the other figure, and then to decide whether the two figures were identical or mirror images of each other”; or in: Keehner et al.'s (2006): ”[…] imagined that the table rotated while they remained stationary”) were included under the category “visual strategy.”
MR can be accomplished taking as a reference frame the object itself (i.e., allocentric view) or the viewer's position (i.e., egocentric view). We considered also the reference frame effect, where it was possible, i.e., as indicated by the authors' instructions. For instance, all the studies in which a mental change of the whole body position in space (a self-rotation) is required (e.g., “[…] after having imagined themselves to be in the figure's body position”; “subjects updated the position of one of four external objects from memory after they had performed an imagined self-rotation to a new position”; see for instance in Wraga et al.'s (2005): “[…] imagined rotating themselves to the location of the T-prompt,” or in Creem-Regehr et al.'s (2007) “[…] instructed to imagine that they were standing at the blue sphere, and from that new imagined perspective to decide whether the previously named hand part, “thumb” or “pinky,” was on their right or left”) were included under the category “egocentric.” Activation in the first group (motor strategy) is expected to be left-lateralized as it exercises processes that prepare motor movements, and it might reflect the left hemisphere dominance for action and goal-directed motor behavior (and apraxia).
The activation in the brain while solving a MR task can be modulated by the type of stimulus. For instance, neuropsychological studies indicate that different operations may be recruited in MR depending on whether the stimulus type is a body part or a two or three-dimensional object 2. In particular it has been shown that lesions in the left hemisphere impaired MR of hands, while lesions in the right hemisphere affected MR of external objects (e.g., a puppet and flag shapes) (Tomasino et al., 2003b). For instance, some authors (Kosslyn et al., 1998) directly compared different types of stimuli and showed that MR of 3D cubes enhanced bilateral activation in the right parietal lobe and in BA 19, whereas MR of hands enhanced unilateral left activation in the precentral gyrus (M1), most of the parietal lobe, the primary visual cortex, the insula, and frontal premotor cortex (BA 6) and the superior frontal cortex (BA 9). The authors proposed that MR of hands and objects can be carried out by engaging two independent mechanisms: one requiring processes that prepare motor movements, and one that does not. Lastly, it has also been shown that performing MR by imagining rotating Shepard and Metzler's stimuli as a consequence of subjects' own hand action (i.e., motor strategy) elicited activation in the left primary motor cortex—the region that in Kosslyn's PET study (Kosslyn et al., 1998) was activated in association with MR of hands only as compared to performing MR by imagining what one would see if someone else, or an external force, manipulated an object (i.e., external strategy) because they simulated a manual rotation (see also Wolbers et al., 2003; Wraga et al., 2003). In a neuropsychological study it was shown that independent of the stimulus to be rotated, patients with right hemisphere lesions were found to be selectively impaired in performing MR by using a visual strategy but were still able to perform MR based on the motor strategy. By contrast, patients with left hemisphere lesions were found to be selectively impaired in MR based on the motor strategy, with intact visual strategy based MR (Tomasino and Rumiati, 2004).
In the present study, we performed quantitative activation-likelihood-estimation (ALE) meta-analyses (Turkeltaub et al., 2002; Laird et al., 2005, 2009; Eickhoff et al., 2009) of functional neuroimaging experiments on MR. We tested a previously formulated hypotheses, the top-down and bottom up hypothesis formulated in published works (Tomasino and Rumiati, 2004, 2013; Tomasino et al., 2004, 2011; Papeo et al., 2012). We referred to bottom-up factors as the effect exerted by the type of stimulus under rot ation. Presenting body parts or external objects as stimuli might differentially contribute to the MR network. In addition, we referred to top-down factors as the effect exerted by the type of MR strategy required by MR instructions. We first identified the MR network including areas consistently activated in neuroimaging studies addressing MR abilities. In a previously published quantitative meta-analysis on MR literature (Zacks, 2008) the network related to MR included the intraparietal sulcus bilaterally, the precentral sulcus bilaterally, the left occipital lobe, and the cingulate gyrus. That meta-analysis and the present study differ in the following aspects. First, in Zack's study, aside a modest number of studies included (data from 32 articles and 320 activation foci, vs. 60 articles and 884 activation foci in the present study), the research question addressed was whether MR depends on analog spatial representations or on motor simulation, whereas here we address the influence of the type of stimulus or reference frame on the MR network. Second, in this previous meta-analysis the author reports that the study search in literature has been done in Medline till 2006. As analysis method to generate the final maps he used volume-wise probability maps (the method and software described by Turkeltaub et al., 2002). We used here a more recently revised activation likelihood estimation (ALE) method, (Eickhoff et al., 2009). Third, the aim of Zack's study was to investigate whether MR depends on analog spatial representation, and whether MR depends on motor simulation. To do so the studies were divided in omnibus and in transformation specific type of MR. On the contrary, in the present study, to address how the MR network can be modulated by the type of stimulus and strategy we divided the studies in the following subgroups: bodily and non-bodily stimuli and motor imagery based vs. visuo-spatial (non-motor)-imagery based transformations. Lastly, Zacks reported the main MR activation network; in the present study we aimed at reporting: the main MR activation network (with a higher number of studies included see above), the MR of bodily stimuli network, the MR of non-bodily stimuli network; the MR motor strategy network; and the MR visual strategy network.
Using an MR task that, through instructions, requires using a motor-imagery based strategy or a visual-imagery based strategy, might differentially contribute to the MR network, as well as the type of stimulus (bodily- and non-bodily-related MR). Based on previous neuropsychological studies (Tomasino et al., 2003a,b), we expected to find that hands and body stimuli will preferentially elicit consistent fMRI activations in the sensorimotor network, whereas 3D cubes, objects, alphanumeric characters and abstract characters will preferentially activate a right-hemisphere network of areas since the right hemisphere is held to be involved in spatial operations (e.g., Ratcliff, 1979; Farah et al., 1988). As a further issue, we addressed whether MR of body-part and MR of whole body can be distinguished in terms of fMRI activation. Whole body pictures require an imagined transformation of one's own body, whereas MR of hands do not.
Testing how the type of stimulus, i.e., bottom up modulation, can modulate the MR-related activations can shed light on some conflicting imaging results showing that MR is lateralized to the left (Alivisatos and Petrides, 1997; Zacks et al., 1999; Kosslyn et al., 2001; Vingerhoets et al., 2001) or to the right hemisphere (Harris et al., 2000; Podzebenko et al., 2002, 2005) or bilaterally (Cohen et al., 1996; Richter et al., 1997; Tagaris et al., 1997; Kosslyn et al., 1998; Carpenter et al., 1999; Jordan et al., 2001).
Testing how the type of strategy, i.e., top down modulation, can modulate the MR-related activations can shed light on the debate about whether the sensorimotor cortex is involved in MR. Finally, some neuroimaging studies explained that activation found in M1 during MR was only due to the action of subjects responding by pressing the response button (Cohen et al., 1996; Richter et al., 2000), others claimed that it provides evidence for the involvement of M1 in MR (Tagaris et al., 1998; Kosslyn et al., 1998; Carpenter et al., 1999; Ganis et al., 2000; Lamm et al., 2001; Vingerhoets et al., 2001). Still others failed to report any M1 activation when subjects performed MR tasks (Parsons and Fox, 1998; Barnes et al., 2000; Harris et al., 2000; Jordan et al., 2001). The presence or absence of M1 activation may be dependent upon the type of stimuli and strategy used and the nature of the stimulus can have a role in triggering the motor or the visual strategy.
Materials and Methods
We filtered the PubMed database (www.pubmed.org), the Web of Knowledge database (www.webofknowledge.com), and the Sleuth on-line database (http://brainmap.org), for functional neuroimaging experiments that investigated MR processes. Moreover, the literature cited in the selected papers and reviews was also searched for additional neuroimaging studies on MR. The included studies were PET or fMRI experiments carried out on healthy subjects. Studies involving pharmacological trials or clinical populations were excluded. They were analyzed by means of a random-effects analysis. Analyses based on regions of interest (ROIs) of functional localizers were excluded. All single-cases studies were excluded, too. In addition, only studies which reported the coordinates in a standard reference space (Talairach/Tournoux, MNI) were considered. Differences in coordinate spaces (MNI vs. Talairach space) were accounted for by transforming coordinates reported in Talairach space into MNI coordinates using a linear transformation (Lancaster et al., 2007).
Based on these criteria, we selected 51 fMRI papers and 9 PET papers for a total of 171 included experiments in which the MR paradigm had been used. Table 1 provides a description of all the included studies. We divided all the collected experiments into several groups: stimuli and strategies/reference frame (Table 1). In the first classification, the criteria we used to classify “body” (i.e., hands or human body) vs. “non-body” (i.e., alphanumeric characters, 3D cubes, abstract stimuli) related stimuli was how the authors described the stimuli. In particular, in the body part category, hand shapes, full bodies, hands and arm pictures were labeled and classified as body part and included. Whereas in the non-bodily category we included all the other stimuli, namely alphanumeric characters, 3D cubes, abstract stimuli. In this classification graspable tools were not included in either body part group or non-bodily stimuli group as they can be grasped thus could be thought of as related to the body, but still they are not body parts. The criteria we used to classify “motor” vs. “visual” strategy was the task instructions reported by authors in the different studies (see Table 1, column 4). For instance Study n.3 reports that authors instructed participants to “visualize it rotating in a continuous movement until it aligned with the other figure.” This type of instruction corresponds to a visual strategy of visualizing the stimulus rotating. Studies in which no explicit instructions were used were excluded from the strategy-related analysis. In the second classification, we reported the specific instructions/definition of the paradigm used as defined by the authors of the included studies. In particular, studies for which detailed instructions or paradigm definition were given, were classified as egocentric/motor imagery based MR tasks and allocentric/visuo-spatial imagery based paradigms. MR of hands was labeled as egocentric/motor imagery based MR since in literature this type of MR is by definition solved via egocentric/motor imagery transformations.
Accordingly we included studies involving bodily-related stimuli (hands, bodies: 16 studies, 220 subjects) and non-bodily related stimuli (3D cubes, alphanumeric characters and abstract shapes: 55 studies, 722 subjects), motor imagery based MR (38 studies, 500 subjects) and visuo-spatial imagery based MR (22 studies, 264 subjects). Studies included for each of these categories are indicated in Table 1 (column Stimuli and column Strategy). We excluded studies in which no coordinates were reported (N = 62), pathological subjects were included (N = 39), pharmacological treatment was performed (N = 10), ROI analyses were carried out (N = 17), children (under 18) were included (N = 9), single cases were included (N = 5) and other (N = 9: 2 engaged transsexuals participants, 1 addressing comparison of women in mid-luteal phase and men, 1 addressing MR in experts in maths, 1 involving a acustic distractor task during MR, 1 including a ROI analysis, 1 engaging a task in which the stimulus disappeared and participants had to keep the mental image active in their mind before MR, 1 involving MR of tactile stimuli, and 1 in which participants mentally visualized the stimuli (they were not visually presented) through verbal instructions).
The reported coordinates for functional activation in each category were analyzed for topographic convergence using the ALE method.
Statistical Analysis
A meta-analysis was carried out using the revised version (Eickhoff et al., 2009) of the ALE approach for coordinate-based meta-analysis of neuroimaging results (Turkeltaub et al., 2002; Laird et al., 2005). To account for the uncertainty that is technically inherent to the actual location of the peaks, the method allows to model each coordinate not as a single point, but by a three-dimensional (3D) Gaussian function with 12 mm FWHM (Laird et al., 2005, 2009; Eickhoff et al., 2009). Accordingly, the localization probability distributions describe the probability that a given focus actually lay within a particular voxel (Laird et al., 2005, 2009; Eickhoff et al., 2009, 2012). ALE probability maps were then thresholded at p < 0.05 (cluster level corrected for multiple comparisons) (Laird et al., 2005, 2009; Eickhoff et al., 2009, 2012) and a minimum cluster size of 200 mm3 was set. We performed the following ALE analysis.
ALE of All Studies Identifying the MR Network
In the first ALE analysis we addressed the “MR Network” by including all eligible studies in order to assess the general MR brain network, by determining brain areas with consistent activation across all studies on MR considered.
ALE of Studies Grouped by Stimulus Type [Bodily > Non-Bodily Related Stimuli and Reversed Contrast)]
To explore how the MR network is influenced by the type of stimulus (see above), the reported studies were grouped as i) bodily- (i.e., hands and body) and non-bodily (i.e., 3D cubes, objects, alphanumeric characters and abstract characters) related stimuli. In addition, we included an analysis comparing MR of hands and MR of body stimuli. In Table 1, we indicated the studies with body or hand stimuli in a corresponding column.
ALE of Studies Grouped by Paradigm Type [Motor Imagery-/Egocentric > Visuo-Spatial Imagery/Allocentric and Reversed Contrast]
In a third analysis, we explored how the MR network is influenced by egocentric/motor-imagery based strategies and allocentric/visual-imagery based strategies. In addition, we included an analysis by distinguishing the strategy from the reference frame variable. We thus compared motor-imagery based MR, see in Table 1 the studies indicated with M in the column Motor only and egocentric MR, see in Table 1 the studies indicated with E in the column egocentric only). An example of motor strategy can be found in Corradi-Dell'Acqua et al.'s study (2009) in which authors report that during the MR task “participants were asked to accomplish the task using different strategies, that is, either by imagining the arm stimulus rotating until this could be wedged in the human photograph (visual strategy) or by ignoring the human photograph and imagining to rotate their own arm until this reached the position depicted in the screen (motor strategy).” An example of egocentric MR can be found in Creem et al.'s study (2001) in which subjects “updated the position of one of four external objects after they had performed an imagined self-rotation to a new position.”
ALE of Studies Grouped by Single Stimulus vs. Pair of Stimuli Presentation
Lastly, we included an additional analysis comparing MR of single stimulus or pairs of stimuli (see in Table 1 the studies indicated with pair in the column Stimuli)3.
Activations were assigned using the SPM Anatomy Toolbox (Eickhoff et al., 2005).
Results
MR Network
Independent of the type of stimulus or the type of strategy, the MR network included activations in the: (i) inferior and superior parietal lobule bilaterally; (ii) left precentral gyrus; (iii) inferior frontal gyrus bilaterally; (iii) middle frontal gyrus bilaterally; (iv) SMA; (v) left insula; (vi) inferior and middle occipital gyrus bilaterally, and (vii) cerebellum bilaterally (see Table 2 and Figure 1).
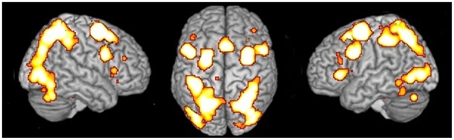
Figure 1. Network of activations underlying MR. Relative increases in neural activity associated with MR are displayed on a rendered template brain provided by spm5. Activations are significant at p < 0.05 corrected for multiple comparisons using the False Discovery Rate (FDR).
Type of Stimulus Dependent Modulation: ALE of Studies Grouped by Stimulus Type
A bilateral sensorimotor activation and a right lateralized activation were found for bodily- and non-bodily stimulus respectively.
MR of Bodily > MR of Non-Bodily Related Stimuli (and Viceversa)
MR of bodily as compared to non-bodily related stimuli included activations in the: (i) cerebellum bilaterally and left calcarine cortex, (ii) left inferior parietal lobe, right angular gyrus, left superior parietal lobe and right postcentral gyrus (Areas 2, 3b, and 4p), (iii) right insula, left superior frontal gyrus and middle cingulate cortex. The reverse contrast (non-bodily related > bodily-related stimuli) included exclusively right-lateralized activations in the: (i) middle occipital gyrus, (ii) cuneus, and (iii) superior parietal cortex (see Table 3 and Figure 2).
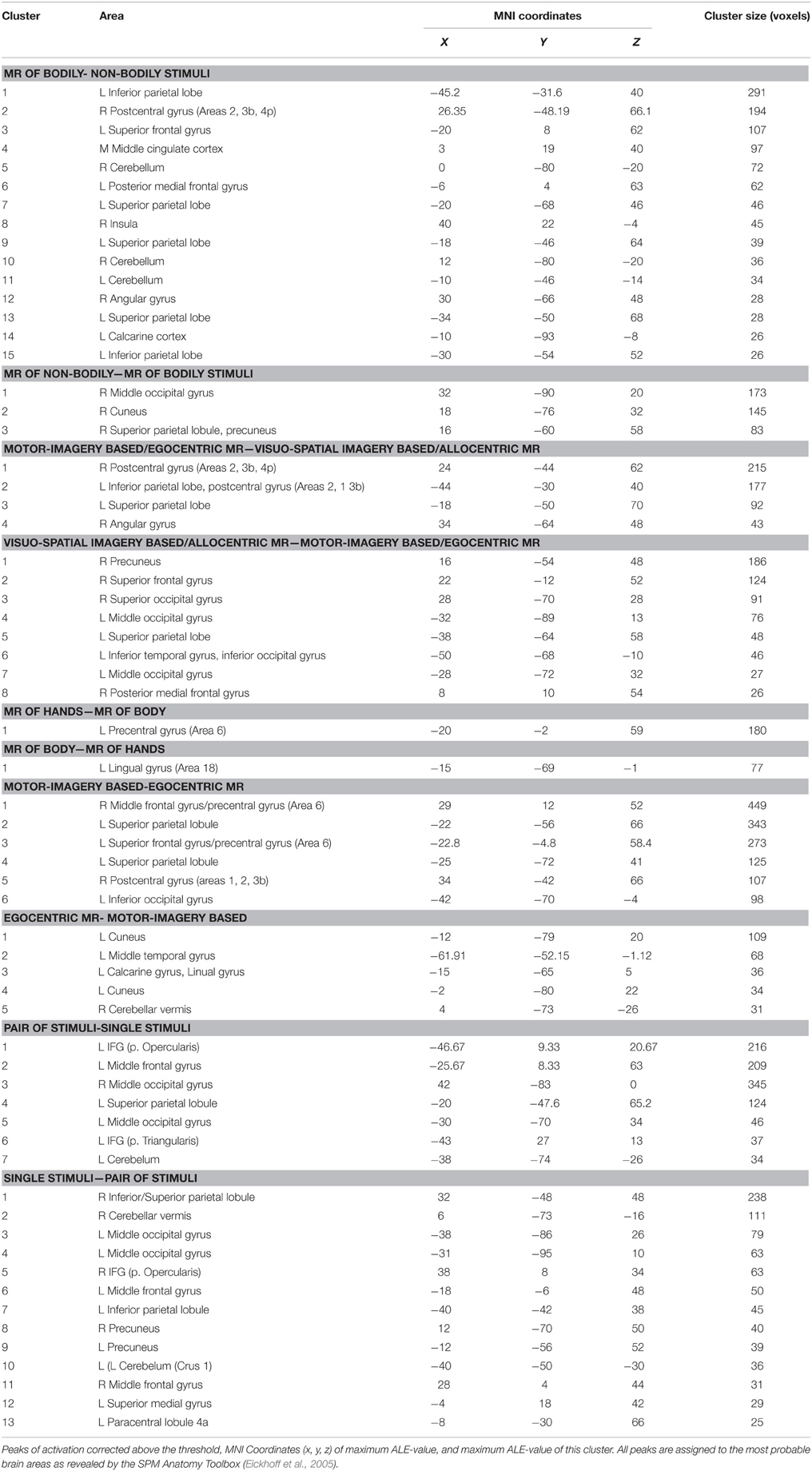
Table 3. Results of the ALE meta-analysis from the direct contrasts revealing the bottom-up modulation of the MR network exerted by the type of stimulus and strategy.
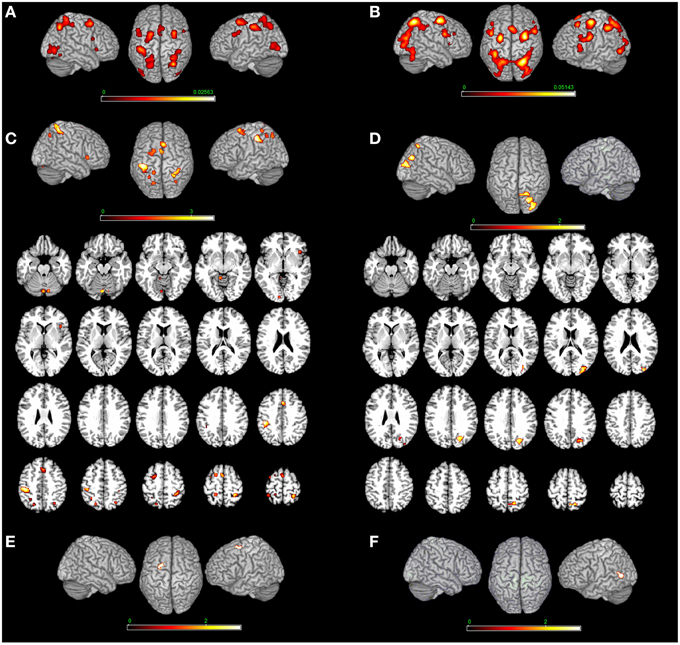
Figure 2. Bottom-up modulation of the MR network exerted by the type of stimulus. Bodily- (A) and non-bodily (B) stimulus. (C) Shows the direct contrast bodily> non-bodily stimulus and (D) shows the contrast non-bodily—bodily stimulus. For bodily related stimuli, in (E) we report MR of hands > body and in (F) we report MR of body—hands. Relative increases in neural activity associated with MR induced by different types of stimuli are displayed on a rendered template brain provided by spm5. Activations are significant at p < 0.05 corrected for multiple comparisons using the False Discovery Rate (FDR). In (A,B) color bar shows ALE value, in (C–F) color bar shows Z maps. The Z coordinates for each slices range from −24 to 66 (with incremental steps of 5 mm).
MR of Bodily Stimuli
MR of bodily stimuli included activations bilaterally in the: (i) cerebellum, middle and inferior occipital and calcarine gyrus, (ii) superior parietal lobule, postcentral gyrus (Area 2) bilaterally, left postcentral gyrus (Area 1), left inferior parietal lobe and right supramarginal gyrus, (iii) left precentral gyrus and inferior frontal gyrus (pars opercularis) bilaterally, left superior frontal gyrus, right middle frontal gyrus and posterior frontal gyrus medially, in addition to the right insula (see Table 4 and Figure 2). Regarding the comparison between hand and body stimuli, results showed that MR of hands (vs. MR of body stimuli) activated the left precentral gyrus (Area 6). MR of body stimuli (vs. MR of hands) activated the left lingual gyrus (Area 18) (see Table 3 and Figure 2).
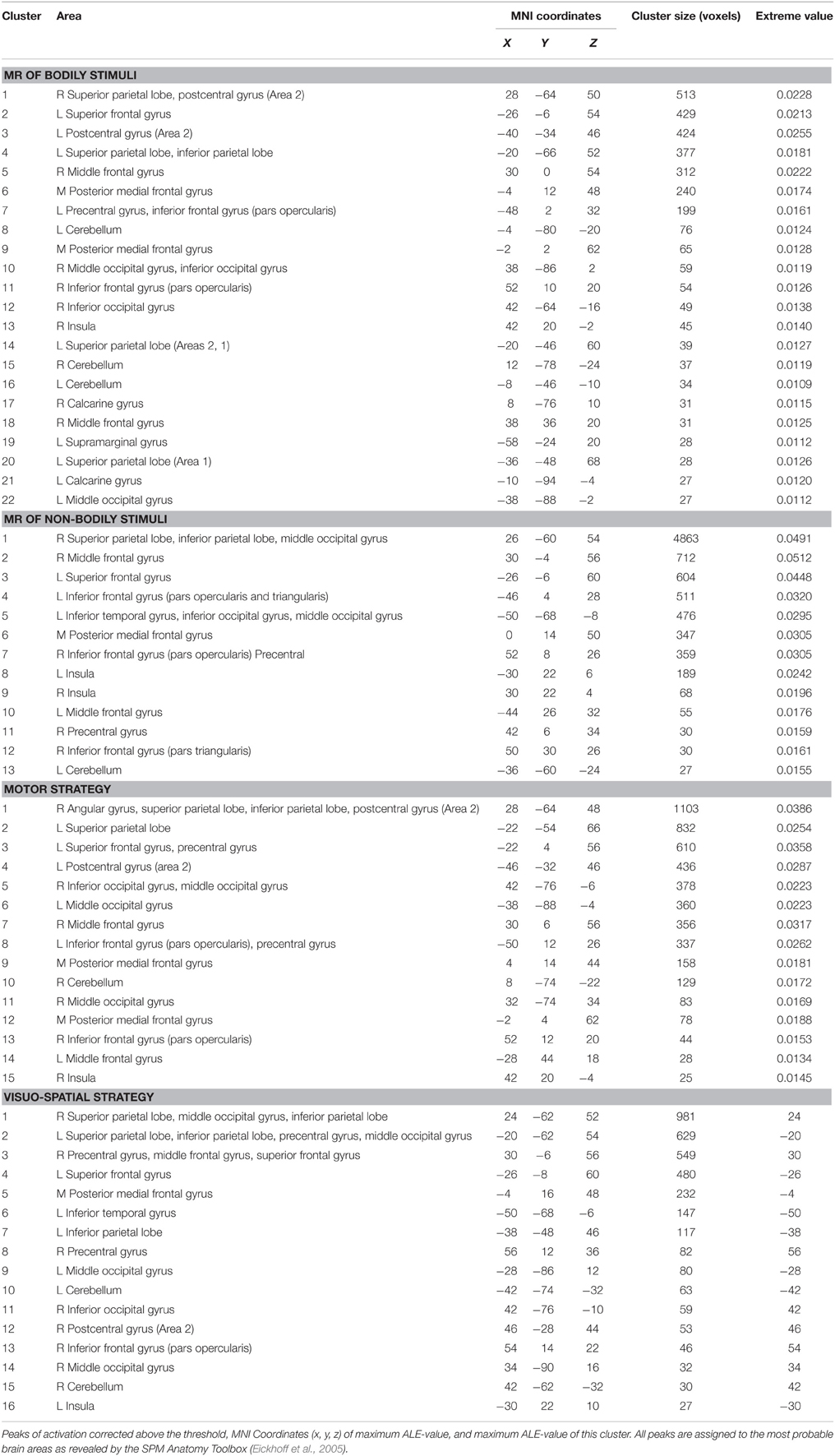
Table 4. Results of the ALE meta-analysis revealing the main effect of the type of stimulus and strategy.
MR of Non-Bodily Stimuli
MR of non-bodily related stimuli included activations in the: (i) middle occipital gyrus bilaterally, left inferior occipital gyrus, left cerebellum and left inferior temporal gyrus, (ii) right superior and inferior parietal lobe, and inferior frontal gyrus (pars opercularis and triangularis) bilaterally, in insula and the middle frontal gyrus in addition to the right precentral gyrus and the posterior frontal gyrus medially (see Table 4 and Figure 2).
Type of Strategy Dependent Modulation: ALE of Studies Grouped by Paradigm Type
A top-down modulation of the network was exerted by the MR strategy/reference frame with a preferentially sensorimotor network for motor imagery- vs. non-motor imagery-based MR and the latter activating a preferentially posterior occipito-temporal-parietal network as follows.
Motor > Visual Strategy (and Viceversa)
Motor strategy as compared to visual strategy included activations in the: (i) right postcentral gyrus (Areas 4p, 2, 3b), (ii) left postcentral gyrus (Areas 2, 1, and 3b) extending to the inferior parietal lobe, (iii) left superior parietal lobe and iv) the right angular gyrus. The reverse contrast (visual strategy as compared to motor strategy) included activations in the: (i) left middle occipital gyrus, left inferior occipital gyrus, left inferior temporal gyrus, and right superior occipital gyrus, (ii) right precuneus, (iii) left superior parietal lobe, and (iv) right posterior medial frontal gyrus and right superior frontal gyrus (see Table 3 and Figure 3).
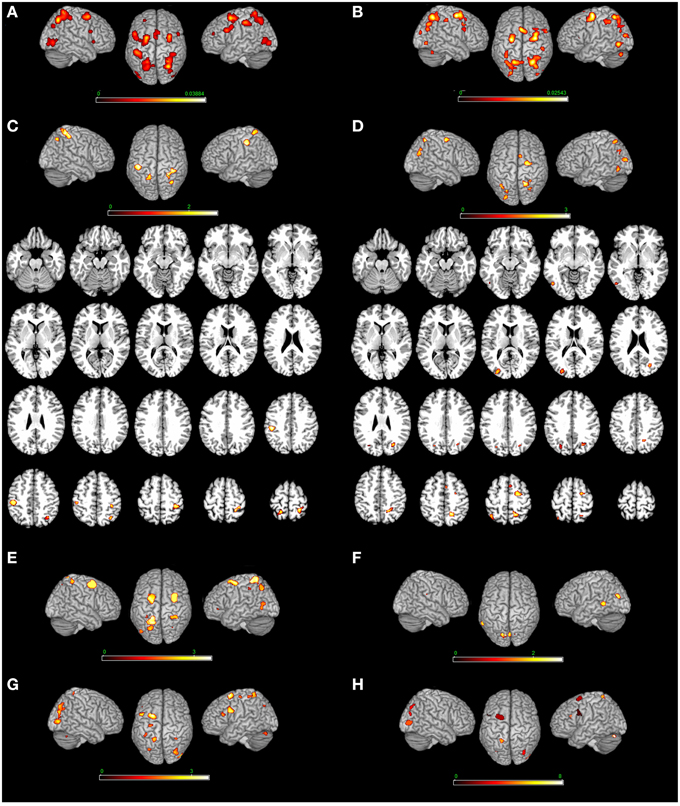
Figure 3. Top-down modulation of the MR network exerted by the type of strategy. [Motor imagery-based/egocentric (A) non-motor-imagery-based/allocentric (B) MR] and by the direct contrast [(C) motor imagery-based/egocentric > non-motor-imagery-based/allocentric and (D) non-motor-imagery-based/allocentric > motor imagery-based/egocentric]. (E) Motor-imagery based MR (vs. egocentric MR); (F) egocentric MR (vs. motor-imagery based MR); (G) Single stimulus (vs. pair of stimuli) presentation; (H) Pair of stimuli (vs. single stimulus). Relative increases in neural activity associated with MR induced by different types of strategies are displayed on a rendered template brain provided by spm5. Activations are significant at p < 0.05 corrected for multiple comparisons using the False Discovery Rate (FDR). In (A,B) color bar shows ALE value; in (C–F) color bar shows Z maps. The Z coordinates for each slices range from −24 to 66 (with incremental steps of 5 mm).
Motor Strategy
MR performed via the motor imagery based/Egocentric strategy included bilateral activations in the: (i) cerebellum bilaterally, (ii) right inferior occipital gyrus and middle occipital gyrus bilaterally, (iii) superior parietal lobe and postcentral gyrus (Area 2) bilaterally, (iv) left precentral gyrus, (v) inferior frontal gyrus (pars opercularis) bilaterally, (vi) left superior frontal gyrus and middle frontal gyrus bilaterally, (vii) posterior medial frontal gyrus, and (viii) right insula (see Table 4 and Figure 3). In addition, we performed an analysis by distinguishing the strategy from the reference frame variable. Motor-imagery based MR and egocentric MR, and we directly compared them. Results showed that motor-imagery based MR (vs. egocentric MR) activated the left superior parietal lobule, the right postcentral gyrus (Areas 1, 2, and 3b) and the precentral gyrus/middle and superior frontal gyrus bilaterally, and the left inferior occipital gyrus. Egocentric MR (vs. motor-imagery based MR) activated the left cuneus, the left middle temporal gyrus, the left lingual gyrus and calcarine sulcus, and the right cerebellum (see Table 3 and Figure 2).
Visual Strategy
MR performed via the visuo-spatial imagery based/allocentric strategy included activations in the: (i) cerebellum bilaterally, (ii) middle occipital gyrus bilaterally, right inferior occipital gyrus and left inferior temporal gyrus, (iii) superior and inferior parietal lobe, (iv) left inferior parietal lobe, (v) right postcentral gyrus (Area 2), (vi) precentral gyrus bilaterally, (vii) superior frontal gyrus bilaterally, (viii) posterior medial frontal gyrus, (ix) right inferior frontal gyrus (pars opercularis), and (x) left insula (see Table 4 and Figure 3).
Single Stimulus (vs. Pair of Stimuli) Presentation
MR of pairs of stimuli (vs. single stimulus) included activations in the: (i) left inferior and in the middle frontal gyrus, (ii) left superior parietal lobule, and (iii) middle occipital gyrus (bilaterally) and left cerebellum. MR of single stimulus (vs. pair of stimuli) included activations in the: (i) right inferior frontal gyrus and in the middle frontal gyrus (bilaterally), (ii) right and left inferior, in the right superior parietal lobule, and precuneus bilaterally, (iii) left paracentral lobule (area 4a, at x = −8, y = −30, z = 66 approximately the foot area4), and (iv) left middle occipital gyrus and cerebellum bilaterally (see Table 3 and Figure 3).
Discussion
Before addressing the implications of our main finding, that is, the differential modulation of the MR network exerted by the type of stimulus and by the type of strategy, we first discuss the neural network involved in the MR task per se. The activations encompassed areas which have been shown to be involved in MR processing by MEG, EEG, TMS, connectivity studies and neuropsychology (Kawamichi et al., 1998, 2007b; Tomasino et al., 2003a,b; Tomasino and Rumiati, 2004; Koshino et al., 2005; Feredoes and Sachdev, 2006; Mourao-Miranda et al., 2009; Seurinck et al., 2011; Lebon et al., 2012; Sack and Schuhmann, 2012; Thomas et al., 2013; Osuagwu and Vuckovic, 2014): the inferior and superior parietal lobule bilaterally, the precentral gyrus, the inferior frontal gyrus, the middle frontal gyrus, the SMA, the insula, the inferior and middle occipital gyrus and the cerebellum.
Bottom-Up Modulation of the MR Network by the Type of Stimulus
We found how the MR network can be modulated by the type of stimuli under rotation. A bilateral sensorimotor activation was found by comparing the bodily- to non-bodily stimuli. The network included the left inferior parietal lobe, right angular gyrus, left superior parietal lobe and right postcentral gyrus (Areas 2, 3b, and 4p), in addition to the left superior frontal gyrus and middle cingulate cortex. These findings are consistent with the TMS and neuropsychological literature on MR of hand shapes which seem to be related to the parietal lobe (Tomasino et al., 2003a,b; Pelgrims et al., 2005, 2009; Schwabe et al., 2009; Lebon et al., 2012; Yan et al., 2012, 2013; Thomas et al., 2013). In addition, we performed an analysis now by distinguishing MR of hands and MR of body stimuli, and we directly compared them. Results showed that MR of hands (vs. MR of body stimuli) activated the left precentral gyrus (Area 6). MR of body stimuli (vs. MR of hands) activated the left lingual gyrus (Area 18). These results confirm the view that whole body pictures and MR of hands can recruit different areas.
The reverse contrast (non-bodily related > bodily-related stimuli) included exclusively right-lateralized activations in the middle occipital gyrus, cuneus and superior parietal cortex. The literature on MR has shown that the key areas supporting MR of 3D cubes seem to be the superior parietal lobule (BA 7), together with the inferior frontal gyrus (BA 44/45) (Cohen et al., 1996; Thomsen et al., 2000), the middle frontal gyrus (BA 8), the parieto-occipital border (BAs 39/19) (Cohen et al., 1996) or –as in Richter et al. (2000)– the lateral premotor cortex (BA 6) and the supplementary motor area (medial BA 6). There are also studies showing a more intense activation in the right hemisphere (Richter et al., 1997; Thomsen et al., 2000) and others reporting a bilateral activation (Cohen et al., 1996; Tagaris et al., 1996, 1998; Carpenter et al., 1999; Richter et al., 2000). Similarly, the literature on MR of alphanumeric stimuli preferentially activated a right-lateralized network of areas involving the inferior and superior parietal lobule, the inferior temporal gyrus, the middle and inferior frontal gyrus, and the inferior and middle occipital gyrus, and MR of abstract stimuli included preferentially right-lateralized activations in the middle frontal gyrus and superior medial gyrus, the precuneus, the inferior and superior parietal lobule and the cerebellum. One cluster was found in the left hemisphere, in the left superior parietal lobule. These results are consistent with previous studies which showed that alphanumeric characters and abstract shapes enhance activation in the right superior parietal lobe (Alivisatos and Petrides, 1997; Tagaris et al., 1998; Harris et al., 2000; Podzebenko et al., 2002) together with the precentral gyrus more intensely over the right hemisphere, the extrastriate visual cortex (Tagaris et al., 1998), the left occipito-temporal junction (Podzebenko et al., 2002), the superior lateral cerebellum, the inferior frontal gyrus (BA 44/45) (Podzebenko et al., 2002) and the right posterior MFG (premotor area, BA 6) (Podzebenko et al., 2002).
Taken together, these results indicate that the type of stimulus used in MR experiments can elicit different patterns of activation and likely two types of MR mechanisms (Kosslyn et al., 1998).
A different account holds that it is the type of judgment that leads to the use of a given strategy. Tasks requiring participants to compare two simultaneously presented rotated images, in order to decide whether they are same or different, are likely to trigger object-based transformations. Whereas tasks requiring participants to judge whether a single stimulus, e.g., a body, shows the left or right arm raised are likely to trigger egocentric-based transformations (Steggemann et al., 2011). The possibility to classify studies according to this dichotomy was addressed by comparing MR of single stimulus or pairs of stimuli presentation, although the two variables, namely number of stimuli and type of transformation cannot be fully disentangled. One possibility could be comparing the ALE maps in the egocentric and one stimulus condition respectively the object-based and two stimuli condition. To perform this analysis a higher number of studies is necessary.
Top-Down Modulation of the MR Network by the Type of Strategy
The direct comparison between motor and visual strategy revealed the areas selectively modulated by one strategy or the other. In particular, when we directly compared the motor strategy to the visual strategy, we found bilateral activations in the sensorimotor areas. The reverse contrast (visual strategy as compared to the motor strategy) included bilateral activations involving the posterior occipital-temporal-parietal cortex. In addition, we performed an analysis by distinguishing the strategy from the reference frame variable. Motor-imagery based MR (vs. egocentric MR) activated the left superior parietal lobule, the right postcentral gyrus (Areas 1, 2, and 3b) and the precentral gyrus/middle and superior frontal gyrus bilaterally, and the left inferior occipital gyrus. Egocentric MR (vs. motor-imagery based MR) activated the left cuneus, the left middle temporal gyrus, the left lingual gyrus and calcarine sulcus, and the right erebellum. In a PET study (Kosslyn et al., 2001), subjects performed MR of Shepard and Metzler stimuli by imagining grasping the object and turning it with their own hand, and by mentally rotating the stimulus as if it were being rotated by a motor. The authors found an area of activation in the left superior/inferior parietal cortex, the left M1 cortex and the right parahippocampal gyrus when subjects solved the MR as a consequence of their manual activity (i.e., motor strategy). By contrast, the visual strategy activated the left inferior frontal gyrus (Area 47). The use of the motor and visual strategy in MR of 3D cubes has been further investigated in an fMRI study (Wolbers et al., 2003) by combining MR of 3D cubes and motor imagery for hands. The authors, who named the two strategies as active and passive rotations, detected an activation centered on the superior parietal lobe that was contralateral to the imagined hand.
Taken together, these results indicate that when requested by the experimenter through instructions subjects adopt one strategy or the other, and this triggers different modulation in the MR network in a top-down way.
One might argue that distinguishing visual and motor strategy is difficult even if a strategy is assigned by the authors of the original studies by means of instructions, since one cannot be sure of what the subjects do. However, in mostly all of the studies we included, authors reported behavioral data indicating that participants correctly used the motor or the visual strategy. For instance, in Papeo et al. (2012)'s study, it is reported that authors checked reliable indication that individuals used the motor or the visual strategy in RTs.
It is known that also the reference frame (egocentric or allocentric) modulates the MR processing. When directly comparing the strategy account to the reference frame variable we found that motor-imagery based MR (vs. egocentric MR) activated the left superior parietal lobule, the right postcentral gyrus (Areas 1, 2, and 3b) and the precentral gyrus/middle and superior frontal gyrus bilaterally, and the left inferior occipital gyrus, whereas the egocentric MR (vs. motor-imagery based MR) activated the left cuneus, the left middle temporal gyrus, the left lingual gyrus and calcarine sulcus, and the right cerebellum. These results indicate that these two mechanisms are different. Motor strategies engage motor behavior covertly whereas the egocentric based MR involves a (mostly spatial) judgment from another point of view (different from the perspective of the physical body).
The presentation of single stimulus vs. pairs of stimuli also influenced the MR network, however given the strength of the modulation exerted by the type of stimulus and the type of strategy we believe that it would be a very limited account considering the number of stimuli shown alone.
Lastly, the result related to the type of stimuli reporting a bilateral sensorimotor activation for bodily-related (vs. non-bodily-related stimuli) and a posterior right lateralized activation for non-bodily-related (vs. bodily-related stimuli) is consistent, as far as lateralization effects is concerned, with the result related to the type of strategy reporting a bilateral (preferentially sensorimotor left) network for motor imagery- vs. non-motor imagery-based MR and the latter activating a preferentially posterior right occipito-temporal-parietal network.
Is the M1 Cortex Involved in MR?
Activation in M1 during MR tasks is not universally accepted. In some imaging studies the activation found in M1 during MR was explained as due to the subjects responding by pressing the response button (Cohen et al., 1996; Richter et al., 2000). Other studies have failed to report M1 activation when subjects performed MR tasks (Parsons et al., 1995; Parsons and Fox, 1998; Harris et al., 2000; Jordan et al., 2001), while others found M1 activated (Kosslyn et al., 1998). Further evidence supporting a critical role of the left M1 in MR is provided by Ganis et al. (2000) who, using TMS, showed that stimulating the human hand area in the left M1 at 650 ms after stimulus onset significantly slowed down the subjects' latencies when they mentally rotated hands, but not feet (Ganis et al., 2000). The idea that the manipulation of mental images is associated with a motor process was already intrinsic to the definition of MR given by Shepard and Cooper (1982). They pointed out that stimuli under MR appear to move in imagery, as they would if they were physically rotated by the subject. This operation can be triggered implicitly by hands, as confirmed by our meta-analysis. Indeed, in our meta-analysis M1 was found to be activated in the MR of bodily vs. non-bodily stimuli contrast (cluster 2) and it was found to be sensitive to the cognitive strategy used since it was again found in the motor-imagery/egocentric based strategy vs. visuo-spatial imagery/allocentric based strategy (cluster 1). We suggest that the type of stimulus (i.e., hands or external objects) may implicitly trigger one strategy or the other (i.e., motor or visual strategy, respectively), and that the left M1 supports the former. Results of the present meta-analysis are also consistent with neuropsychological data showing that MR can be impaired in patients with a tumor affecting the hand area, providing that they imagined the rotation as a consequence of their own hand action. By contrast, lesions in the left M1 sparing the hand area did not lead to an MR deficit (Tomasino et al., 2011), which indicate that the involvement of the hand area of the left M1 cortex is strategy-driven, and that the left M1 supports the motor strategy. That activity in the M1 cortex can be suppressed during tasks tapping motor imagery is not surprising (Solodkin et al., 2004). It has been shown that inputs to M1 are suppressed during kinaesthetic imagery, suggesting the existence of a physiological mechanism whereby the motor system prevents overt movements (Solodkin et al., 2004). Other authors argued that the lack of activation in M1 during motor imagery in their task was caused by the suppression exerted by the SMA emphasizing the role of this region in suppressing movements that are represented in the motor system but not to be performed (Kasess et al., 2008).
Conclusion
The main points tested by using a quantitative meta-analytic approach (as reported more extensively in the introduction) were:
(1) Can the bottom up modulation shed light on MR-related hemispheric lateralization issue? Our results showed that bodily- (vs. non-bodily) stimuli activate a bilateral sensorimotor network, whereas non-bodily (vs. bodily)-related stimuli) included exclusively right-lateralized activations.
(2) Can the top down modulation shed light on the debate about whether the sensorimotor cortex is involved in MR? Our results showed that when we directly compared the motor to the visual strategy, we found bilateral activations in the sensorimotor areas.
(3) May the M1 activation depend upon the type of stimuli and strategy used? Our results showed that M1 was activated for motor (vs. visual) strategy and bodily- (vs. non-bodily) stimuli.
Conflict of Interest Statement
The authors declare that the research was conducted in the absence of any commercial or financial relationships that could be construed as a potential conflict of interest.
Footnotes
1 ^It is known that an additional factor influencing MR mechanisms might be the reference frame (Zacks et al., 2003).
2 ^Another account holds that it is the type of judgment that leads to the use of a specific strategy, e.g., (Steggemann et al., 2011).
3 ^In behavioral studies it is assumed that this variable can affect MR mechanisms. Two images are presented simultaneously side-by-side and participants are required to perform a same-different decision by judging whether the right stimulus is the same or a mirror-reversed (different) version of the left stimulus. This type of MR may trigger mostly object-based transformation. A body stimulus like a single human figure raising one arm (left or right) presented under different orientations may trigger mostly an egocentric transformation (Steggemann et al., 2011).
4 ^Very close to the coordinates derived from (Ehrsson et al., 2003)'s fMRI study on motor imagery and somatotopy of M1 activations: x = −8, y = −28 and z = 64 (left toe/foot) and x = 8, y = −28 and z = 64 (right toe/foot; both in MNI space).
References
Alivisatos, B., and Petrides, M. (1997). Functional activation of the human brain during mental rotation. Neuropsychologia 35, 111–118. doi: 10.1016/S0028-3932(96)00083-8
Aso, T., Hanakawa, T., Matsuo, K., Toma, K., Shibasaki, H., Fukuyama, H., et al. (2007). Subregions of human parietal cortex selectively encoding object orientation. Neurosci. Lett. 415, 225–230. doi: 10.1016/j.neulet.2007.01.051
Barnes, J., Howard, R. J., Senior, C., Brammer, M., Bullmore, E. T., Simmons, A., et al. (2000). Cortical activity during rotational and linear transformations. Neuropsychologia 38, 1148–1156. doi: 10.1016/S0028-3932(00)00025-7
Blanke, O., Ionta, S., Fornari, E., Mohr, C., and Maeder, P. (2010). Mental imagery for full and upper human bodies: common right hemisphere activations and distinct extrastriate activations. Brain Topogr. 23, 321–332. doi: 10.1007/s10548-010-0138-x
Bonda, E., Petrides, M., Frey, S., and Evans, A. (1995). Neural correlates of mental transformations of the body-in-space. Proc. Natl. Acad. Sci. U.S.A. 92, 11180–11184. doi: 10.1073/pnas.92.24.11180
Butler, A. J., Fink, G. R., Dohle, C., Wunderlich, G., Tellmann, L., Seitz, R. J., et al. (2004). Neural mechanisms underlying reaching for remembered targets cued kinesthetically or visually in left or right hemispace. Hum. Brain Mapp. 21, 165–177. doi: 10.1002/hbm.20001
Carpenter, P. A., Just, M. A., Keller, T. A., Eddy, W., and Thulborn, K. (1999). Graded functional activation in the visuospatial system with the amount of task demand. J. Cogn. Neurosci. 11, 9–24. doi: 10.1162/089892999563210
Cohen, M. S., Kosslyn, S. M., Breiter, H. C., DiGirolamo, G. J., Thompson, W. L., Anderson, A. K., et al. (1996). Changes in cortical activity during mental rotation. A mapping study using functional MRI. Brain 119(Pt 1), 89–100. doi: 10.1093/brain/119.1.89
Corballis, M. C. (1997). Mental rotation and the right hemisphere. Brain Lang. 57, 100–121. doi: 10.1006/brln.1997.1835
Corballis, M. C., and Sergent, J. (1989). Hemispheric specialization for mental rotation. Cortex 25, 15–25. doi: 10.1016/S0010-9452(89)80002-4
Corradi-Dell'Acqua, C., Tomasino, B., and Fink, G. R. (2009). What is the position of an arm relative to the body? Neural correlates of body schema and body structural description. J. Neurosci. 29, 4162–4171. doi: 10.1523/JNEUROSCI.4861-08.2009
Creem, S. H., Downs, T. H., Wraga, M., Harrington, G. S., Proffitt, D. R., and Downs, J. H. III (2001). An fMRI study of imagined self-rotation. Cogn. Affect. Behav. Neurosci. 1, 239–249. doi: 10.3758/CABN.1.3.239
Creem-Regehr, S. H., Neil, J. A., and Yeh, H. J. (2007). Neural correlates of two imagined egocentric transformations. Neuroimage 35, 916–927. doi: 10.1016/j.neuroimage.2006.11.057
de Lange, F. P., Hagoort, P., and Toni, I. (2005). Neural topography and content of movement representations. J. Cogn. Neurosci. 17, 97–112. doi: 10.1162/0898929052880039
de Lange, F. P., Helmich, R. C., and Toni, I. (2006). Posture influences motor imagery: an fMRI study. Neuroimage 33, 609–617. doi: 10.1016/j.neuroimage.2006.07.017
Ehrsson, H. H., Geyer, S., and Naito, E. (2003). Imagery of voluntary movement of fingers, toes, and tongue activates corresponding body-part specific motor representations. J. Neurophysiol. 90, 3304–3316. doi: 10.1152/jn.01113.2002
Eickhoff, S. B., Bzdok, D., Laird, A. R., Kurth, F., and Fox, P. T. (2012). Activation likelihood estimation meta-analysis revisited. Neuroimage 59, 2349–2361. doi: 10.1016/j.neuroimage.2011.09.017
Eickhoff, S. B., Laird, A. R., Grefkes, C., Wang, L. E., Zilles, K., and Fox, P. T. (2009). Coordinate-based activation likelihood estimation meta-analysis of neuroimaging data: a random-effects approach based on empirical estimates of spatial uncertainty. Hum. Brain Mapp. 30, 2907–2926. doi: 10.1002/hbm.20718
Eickhoff, S., Stephan, K. E., Mohlberg, H., Grefkes, C., Fink, G. R., Amunts, K., et al. (2005). A new SPM toolbox for combining probabilistic cytoarchitectonic maps and functional imaging data. Neuroimage 25, 1325–1335. doi: 10.1016/j.neuroimage.2004.12.034
Farah, M. J., Hammond, K. M., Levine, D. N., and Calvanio, R. (1988). Visual and spatial mental imagery: dissociable systems of representation. Cogn. Psychol. 20, 439–462. doi: 10.1016/0010-0285(88)90012-6
Feredoes, E. A., and Sachdev, P. S. (2006). Differential effects of transcranial magnetic stimulation of left and right posterior parietal cortex on mental rotation tasks. Cortex 42, 750–754. doi: 10.1016/S0010-9452(08)70413-1
Ferri, F., Frassinetti, F., Ardizzi, M., Costantini, M., and Gallese, V. (2012). A sensorimotor network for the bodily self. J. Cogn. Neurosci. 24, 1584–1595. doi: 10.1162/jocn_a_00230
Ganis, G., Keenan, J. P., Kosslyn, S. M., and Pascual-Leone, A. (2000). Transcranial magnetic stimulation of primary motor cortex affects mental rotation. Cereb. Cortex 10, 175–180. doi: 10.1093/cercor/10.2.175
Gogos, A., Gavrilescu, M., Davison, S., Searle, K., Adams, J., Rossell, S. L., et al. (2010). Greater superior than inferior parietal lobule activation with increasing rotation angle during mental rotation: an fMRI study. Neuropsychologia 48, 529–535. doi: 10.1016/j.neuropsychologia.2009.10.013
Halari, R., Sharma, T., Hines, M., Andrew, C., Simmons, A., and Kumari, V. (2006). Comparable fMRI activity with differential behavioural performance on mental rotation and overt verbal fluency tasks in healthy men and women. Exp. Brain Res. 169, 1–14. doi: 10.1007/s00221-005-0118-7
Harris, I. M., Egan, G. F., Sonkkila, C., Tochon-Danguy, H. J., Paxinos, G., and Watson, J. D. (2000). Selective right parietal lobe activation during mental rotation: a parametric PET study. Brain 123(Pt 1), 65–73. doi: 10.1093/brain/123.1.65
Hugdahl, K., Thomsen, T., and Ersland, L. (2006). Sex differences in visuo-spatial processing: an fMRI study of mental rotation. Neuropsychologia 44, 1575–1583. doi: 10.1016/j.neuropsychologia.2006.01.026
Johnston, S., Leek, E. C., Atherton, C., Thacker, N., and Jackson, A. (2004). Functional contribution of medial premotor cortex to visuo-spatial transformation in humans. Neurosci. Lett. 355, 209–212. doi: 10.1016/j.neulet.2003.11.011
Jordan, K., Heinze, H. J., Lutz, K., Kanowski, M., and Jäncke, L. (2001). Cortical activations during the mental rotation of different visual objects. Neuroimage 13, 143–152. doi: 10.1006/nimg.2000.0677
Jordan, K., Wustenberg, T., Heinze, H. J., Peters, M., and Jäncke, L. (2002). Women and men exhibit different cortical activation patterns during mental rotation tasks. Neuropsychologia 40, 2397–2408. doi: 10.1016/S0028-3932(02)00076-3
Kasess, C. H., Windischberger, C., Cunnington, R., Lanzenberger, R., Pezawas, L., and Moser, E. (2008). The suppressive influence of SMA on M1 in motor imagery revealed by fMRI and dynamic causal modeling. Neuroimage 40, 828–837. doi: 10.1016/j.neuroimage.2007.11.040
Kawamichi, H., Kikuchi, Y., Endo, H., Takeda, T., and Yoshizawa, S. (1998). Temporal structure of implicit motor imagery in visual hand-shape discrimination as revealed by MEG. Neuroreport 9, 1127–1132. doi: 10.1097/00001756-199804200-00031
Kawamichi, H., Kikuchi, Y., Noriuchi, M., Senoo, A., and Ueno, S. (2007a). Distinct neural correlates underlying two- and three-dimensional mental rotations using three-dimensional objects. Brain Res. 1144, 117–126. doi: 10.1016/j.brainres.2007.01.082
Kawamichi, H., Kikuchi, Y., and Ueno, S. (2007b). Spatio-temporal brain activity related to rotation method during a mental rotation task of three-dimensional objects: an MEG study. Neuroimage 37, 956–965. doi: 10.1016/j.neuroimage.2007.06.001
Keehner, M., Guerin, S. A., Miller, M. B., Turk, D. J., and Hegarty, M. (2006). Modulation of neural activity by angle of rotation during imagined spatial transformations. Neuroimage 33, 391–398. doi: 10.1016/j.neuroimage.2006.06.043
Koshino, H., Carpenter, P. A., Keller, T. A., and Just, M. A. (2005). Interactions between the dorsal and the ventral pathways in mental rotation: an fMRI study. Cogn. Affect. Behav. Neurosci. 5, 54–66. doi: 10.3758/CABN.5.1.54
Kosslyn, S. M., Behrmann, M., and Jeannerod, M. (1995). The cognitive neuroscience of mental imagery. Neuropsychologia 33, 1335–1344. doi: 10.1016/0028-3932(95)00067-D
Kosslyn, S. M., DiGirolamo, G. J., Thompson, W. L., and Alpert, N. M. (1998). Mental rotation of objects versus hands: neural mechanisms revealed by positron emission tomography. Psychophysiology 35, 151–161. doi: 10.1111/1469-8986.3520151
Kosslyn, S. M., Thompson, W. L., Wraga, M., and Alpert, N. M. (2001). Imagining rotation by endogenous versus exogenous forces: distinct neural mechanisms. Neuroreport 12, 2519–2525. doi: 10.1097/00001756-200108080-00046
Kucian, K., von Aster, M., Loenneker, T., Dietrich, T., Mast, F. W., and Martin, E. (2007). Brain activation during mental rotation in school children and adults. J. Neural Transm. 114, 675–686. doi: 10.1007/s00702-006-0604-5
Laird, A. R., Eickhoff, S. B., Kurth, F., Fox, P. M., Uecker, A. M., Turner, J. A., et al. (2009). ALE meta-analysis workflows via the brainmap database: progress towards a probabilistic functional brain atlas. Front. Neuroinformatics 3:23. doi: 10.3389/neuro.11.023.2009
Laird, A. R., Fox, P. M., Price, C. J., Glahn, D. C., Uecker, A. M., Lancaster, J. L., et al. (2005). ALE meta-analysis: controlling the false discovery rate and performing statistical contrasts. Hum. Brain Mapp. 25, 155–164. doi: 10.1002/hbm.20136
Lambrey, S., Doeller, C., Berthoz, A., and Burgess, N. (2012). Imagining being somewhere else: neural basis of changing perspective in space. Cereb. Cortex 22, 166–174. doi: 10.1093/cercor/bhr101
Lamm, C., Windischberger, C., Leodolter, U., Moser, E., and Bauer, H. (2001). Evidence for premotor cortex activity during dynamic visuospatial imagery from single-trial functional magnetic resonance imaging and event-related slow cortical potentials. Neuroimage 14, 268–283. doi: 10.1006/nimg.2001.0850
Lamm, C., Windischberger, C., Moser, E., and Bauer, H. (2007). The functional role of dorso-lateral premotor cortex during mental rotation: an event-related fMRI study separating cognitive processing steps using a novel task paradigm. Neuroimage 36, 1374–1386. doi: 10.1016/j.neuroimage.2007.04.012
Lancaster, J. L., Tordesillas-Gutiérrez, D., Martinez, M., Salinas, F., Evans, A., Zilles, K., et al. (2007). Bias between MNI and Talairach coordinates analyzed using the ICBM-152 brain template. Hum. Brain Mapp. 28, 1194–1205. doi: 10.1002/hbm.20345
Lebon, F., Lotze, M., Stinear, C. M., and Byblow, W. D. (2012). Task-dependent interaction between parietal and contralateral primary motor cortex during explicit versus implicit motor imagery. PLoS ONE 7:e37850. doi: 10.1371/journal.pone.0037850
Levin, S. L., Mohamed, F. B., and Platek, S. M. (2005). Common ground for spatial cognition? A behavioral and fMRI study of sex differences in mental rotation and spatial working memory. Evol. Psychol. 3, 227–254. doi: 10.1177/147470490500300116
Logie, R. H., Pernet, C. R., Buonocore, A., and Della Sala, S. (2011). Low and high imagers activate networks differentially in mental rotation. Neuropsychologia 49, 3071–3077. doi: 10.1016/j.neuropsychologia.2011.07.011
Milivojevic, B., Hamm, J. P., and Corballis, M. C. (2009). Functional neuroanatomy of mental rotation. J. Cogn. Neurosci. 21, 945–959. doi: 10.1162/jocn.2009.21085
Mourao-Miranda, J., Ecker, C., Sato, J. R., and Brammer, M. (2009). Dynamic changes in the mental rotation network revealed by pattern recognition analysis of fMRI data. J. Cogn. Neurosci. 21, 890–904. doi: 10.1162/jocn.2009.21078
Ng, V. W., Bullmore, E. T., de Zubicaray, G. I., Cooper, A., Suckling, J., and Williams, S. C. (2001). Identifying rate-limiting nodes in large-scale cortical networks for visuospatial processing: an illustration using fMRI. J. Cogn. Neurosci. 13, 537–545. doi: 10.1162/08989290152001943
Osuagwu, B. A., and Vuckovic, A. (2014). Similarities between explicit and implicit motor imagery in mental rotation of hands: an EEG study. Neuropsychologia 65, 197–210. doi: 10.1016/j.neuropsychologia.2014.10.029
Papeo, L., Rumiati, R. I., Cecchetto, C., and Tomasino, B. (2012). On-line changing of thinking about words: the effect of cognitive context on neural responses to verb reading. J. Cogn. Neurosci. 24, 2348–2362. doi: 10.1162/jocn_a_00291
Parsons, L. M. (1987). Imagined spatial transformations of one's hands and feet. Cogn. Psychol. 19, 178–241. doi: 10.1016/0010-0285(87)90011-9
Parsons, L. M., and Fox, P. T. (1998). The neural basis of implicit movements used in recognizing hand shape. Cogn. Neuropsychol. 15, 583–615.
Parsons, L. M., Fox, P. T., Downs, J. H., Glass, T., Hirsch, T. B., Martin, C. C., et al. (1995). Use of implicit motor imagery for visual shape discrimination as revealed by PET. Nature 375, 54–58. doi: 10.1038/375054a0
Parsons, L. M., Gabrieli, J. D. E., Phelps, E. A., and Gazzaniga, M. S. (1998). Cerebrally lateralized mental representations of hand shape and movement. J. Neurosci. 18, 6539–6548.
Paschke, K., Jordan, K., Wüstenberg, T., Baudewig, J., and Leo, M. J. (2012). Mirrored or identical - Is the role of visual perception underestimated in the mental rotation process of 3D-objects? A combined fMRI-eye tracking-study. Neuropsychologia 50, 1844–1851. doi: 10.1016/j.neuropsychologia.2012.04.010
Pelgrims, B., Andres, M., and Olivier, E. (2005). Motor imagery while judging object-hand interactions. Neuroreport 16, 1193–1196. doi: 10.1097/00001756-200508010-00012
Pelgrims, B., Andres, M., and Olivier, E. (2009). Double dissociation between motor and visual imagery in the posterior parietal cortex. Cereb. Cortex 19, 2298–2307. doi: 10.1093/cercor/bhn248
Podzebenko, K., Egan, G. F., and Watson, J. D. (2002). Widespread dorsal stream activation during a parametric mental rotation task, revealed with functional magnetic resonance imaging. Neuroimage 15, 547–558. doi: 10.1006/nimg.2001.0999
Podzebenko, K., Egan, G. F., and Watson, J. D. (2005). Real and imaginary rotary motion processing: functional parcellation of the human parietal lobe revealed by fMRI. J. Cogn. Neurosci. 17, 24–36. doi: 10.1162/0898929052879996
Ratcliff, G. (1979). Spatial thought, mental rotation and the right cerebral hemisphere. Neuropsychologia 17, 49–54. doi: 10.1016/0028-3932(79)90021-6
Richter, W., Somorjai, R., Summers, R., Jarmasz, M., Menon, R. S., Gati, J. S., et al. (2000). Motor area activity during mental rotation studied by time-resolved single-trial fMRI. J. Cogn. Neurosci. 12, 310–320. doi: 10.1162/089892900562129
Richter, W., Ugurbil, K., Georgopoulos, A., and Kim, S. G. (1997). Time-resolved fMRI of mental rotation. Neuroreport 8, 3697–3702. doi: 10.1097/00001756-199712010-00008
Sack, A. T., and Schuhmann, T. (2012). Hemispheric differences within the fronto-parietal network dynamics underlying spatial imagery. Front. Psychol. 3:214. doi: 10.3389/fpsyg.2012.00214
Schendan, H. E., and Stern, C. E. (2007). Mental rotation and object categorization share a common network of prefrontal and dorsal and ventral regions of posterior cortex. Neuroimage 35, 1264–1277. doi: 10.1016/j.neuroimage.2007.01.012
Schöning, S., Engelien, A., Kugel, H., Schäfer, S., Schiffbauer, H., Zwitserlood, P., et al. (2007). Functional anatomy of visuo-spatial working memory during mental rotation is influenced by sex, menstrual cycle, and sex steroid hormones. Neuropsychologia 45, 3203–3214. doi: 10.1016/j.neuropsychologia.2007.06.011
Schwabe, L., Lenggenhager, B., and Blanke, O. (2009). The timing of temporoparietal and frontal activations during mental own body transformations from different visuospatial perspectives. Hum. Brain Mapp. 30, 1801–1812. doi: 10.1002/hbm.20764
Seurinck, R., de Lange, F. P., Achten, E., and Vingerhoets, G. (2011). Mental rotation meets the motion aftereffect: the role of hV5/MT+ in visual mental imagery. J. Cogn. Neurosci. 23, 1395–1404. doi: 10.1162/jocn.2010.21525
Seurinck, R., Vingerhoets, G., de Lange, F. P., and Achten, E. (2004). Does egocentric mental rotation elicit sex differences? Neuroimage 23, 1440–1449. doi: 10.1016/j.neuroimage.2004.08.010
Seurinck, R., Vingerhoets, G., Vandemaele, P., Deblaere, K., and Achten, E. (2005). Trial pacing in mental rotation tasks. Neuroimage 25, 1187–1196. doi: 10.1016/j.neuroimage.2005.01.010
Shepard, R. N., and Cooper, L. A. (1982). Mental Images and Their Transformations. Cambridge, MA: MIT Press/Bradford Books.
Shepard, R. N., and Metzler, J. (1971). Mental rotation of three-dimensional objects. Science 171, 701–703. doi: 10.1126/science.171.3972.701
Sluming, V., Brooks, J., Howard, M., Downes, J. J., and Roberts, N. (2007). Broca's area supports enhanced visuospatial cognition in orchestral musicians. J. Neurosci. 27, 3799–3806. doi: 10.1523/JNEUROSCI.0147-07.2007
Solodkin, A., Hlustik, P., Chen, E. E., and Small, S. L. (2004). Fine modulation in network activation during motor execution and motor imagery. Cereb. Cortex 14, 1246–1255. doi: 10.1093/cercor/bhh086
Steggemann, Y., Engbert, K., and Weigelt, M. (2011). Selective effects of motor expertise in mental body rotation tasks: comparing object-based and perspective transformations. Brain Cogn. 76, 97–105. doi: 10.1016/j.bandc.2011.02.013
Stoodley, C. J., Valera, E. M., and Schmahmann, J. D. (2012). Functional topography of the cerebellum for motor and cognitive tasks: an fMRI study. Neuroimage 59, 1560–1570. doi: 10.1016/j.neuroimage.2011.08.065
Suchan, B., Botko, R., Gizewski, E., Forsting, M., and Daum, I. (2006). Neural substrates of manipulation in visuospatial working memory. Neuroscience 139, 351–357. doi: 10.1016/j.neuroscience.2005.08.020
Suchan, B., Yágüez, L., Wunderlich, G., Canavan, A. G., Herzog, H., Tellmann, L., et al. (2002). Hemispheric dissociation of visual-pattern processing and visual rotation. Behav. Brain Res. 136, 533–544. doi: 10.1016/S0166-4328(02)00204-8
Tagaris, G. A., Kim, S. G., Strupp, J. P., Andersen, P., Ugurbil, K., and Georgopoulos, A. P. (1996). Quantitative relations between parietal activation and performance in mental rotation. Neuroreport 7, 773–776. doi: 10.1097/00001756-199602290-00022
Tagaris, G. A., Kim, S. G., Strupp, J. P., Andersen, P., Ugurbil, K., and Georgopoulos, A. P. (1997). Mental rotation studied by functional magnetic resonance imaging at high field (4 tesla): performance and cortical activation. J. Cogn. Neurosci. 9, 419–432. doi: 10.1162/jocn.1997.9.4.419
Tagaris, G. A., Richter, W., Kim, S. G., Pellizzer, G., Andersen, P., Ugurbil, K., et al. (1998). Functional magnetic resonance imaging of mental rotation and memory scanning: a multidimensional scaling analysis of brain activation patterns. Brain Res. Brain Res. Rev. 26, 106–112. doi: 10.1016/S0165-0173(97)00060-X
Thomas, M., Dalecki, M., and Abeln, V. (2013). EEG coherence during mental rotation of letters, hands and scenes. Int. J. Psychophysiol. 89, 128–135. doi: 10.1016/j.ijpsycho.2013.06.014
Thomsen, T., Hugdahl, K., Ersland, L., Barndon, R., Lundervold, A., Smievoll, A. I., et al. (2000). Functional magnetic resonance imaging (fMRI) study of sex differences in a mental rotation task. Med. Sci. Monit. 6, 1186–1196.
Tomasino, B., and Rumiati, R. I. (2004). Effects of strategies on mental rotation and hemispheric lateralization: neuropsychological evidence. J. Cogn. Neurosci. 16, 878–888. doi: 10.1162/089892904970753
Tomasino, B., and Rumiati, R. I. (2013). At the mercy of strategies: the role of motor representations in language understanding. Front. Psychol. 4:27. doi: 10.3389/fpsyg.2013.00027
Tomasino, B., Rumiati, R. I., and Umiltà, C. (2003a). Selective deficit of motor imagery as tapped by a left-right decision of visually presented hands. Brain Cogn. 53, 376–380. doi: 10.1016/S0278-2626(03)00147-7
Tomasino, B., Skrap, M., and Rumiati, R. I. (2011). Causal role of the sensorimotor cortex in action simulation: neuropsychological evidence. J. Cogn. Neurosci. 23, 2068–2078. doi: 10.1162/jocn.2010.21577
Tomasino, B., Toraldo, A., and Rumiati, R. I. (2003b). Dissociation between the mental rotation of visual images and motor images in unilateral brain-damaged patients. Brain Cogn. 51, 368–371. doi: 10.1016/S0278-2626(02)00570-5
Tomasino, B., Vorano, L., Skrap, M., Gigli, G., and Rumiati, R. I. (2004). Effects of strategies of mental rotation performed by unilateral brain damaged patients. Cortex 40, 197–199. doi: 10.1016/S0010-9452(08)70949-3
Turkeltaub, P. E., Eden, G. F., Jones, K. M., and Zeffiro, T. A. (2002). Meta-analysis of the functional neuroanatomy of single-word reading: method and validation. Neuroimage 16, 765–780. doi: 10.1006/nimg.2002.1131
Vanrie, J., Béatse, E., Wagemans, J., Sunaert, S., and Van Hecke, P. (2002). Mental rotation versus invariant features in object perception from different viewpoints: an fMRI study. Neuropsychologia 40, 917–930. doi: 10.1016/S0028-3932(01)00161-0
Vingerhoets, G., de Lange, F. P., Vandemaele, P., Deblaere, K., and Achten, E. (2002). Motor imagery in mental rotation: an fMRI study. Neuroimage 17, 1623–1633. doi: 10.1006/nimg.2002.1290
Vingerhoets, G., Santens, P., Van Laere, K., Lahorte, P., Dierckx, R. A., and De, R. J. (2001). Regional brain activity during different paradigms of mental rotation in healthy volunteers: a positron emission tomography study. Neuroimage 13, 381–391. doi: 10.1006/nimg.2000.0690
Wartenburger, I., Heekeren, H. R., Preusse, F., Kramer, J., and van der Meer, E. (2009). Cerebral correlates of analogical processing and their modulation by training. Neuroimage 48, 291–302. doi: 10.1016/j.neuroimage.2009.06.025
Weiss, E., Siedentopf, C. M., Hofer, A., Deisenhammer, E. A., Hoptman, M. J., Kremser, C., et al. (2003). Sex differences in brain activation pattern during a visuospatial cognitive task: a functional magnetic resonance imaging study in healthy volunteers. Neurosci. Lett. 344, 169–172. doi: 10.1016/S0304-3940(03)00406-3
Weiss, M. M., Wolbers, T., Peller, M., Witt, K., Marshall, L., Buchel, C., et al. (2009). Rotated alphanumeric characters do not automatically activate frontoparietal areas subserving mental rotation. Neuroimage 44, 1063–1073. doi: 10.1016/j.neuroimage.2008.09.042
Wexler, M., Kosslyn, S. M., and Berthoz, A. (1998). Motor processes in mental rotation. Cognition 68, 77–94. doi: 10.1016/S0010-0277(98)00032-8
Wilson, K. D., and Farah, M. J. (2006). Distinct patterns of viewpoint-dependent BOLD activity during common-object recognition and mental rotation. Perception 35, 1351–1366. doi: 10.1068/p5571
Wolbers, T., Weiller, C., and Büchel, C. (2003). Contralateral coding of imagined body parts in the superior parietal lobe. Cereb. Cortex 13, 392–399. doi: 10.1093/cercor/13.4.392
Wraga, M., Boyle, H. K., and Flynn, C. M. (2010a). Role of motor processes in extrinsically encoding mental transformations. Brain Cogn. 74, 193–202. doi: 10.1016/j.bandc.2010.07.005
Wraga, M., Flynn, C. M., Boyle, H. K., and Evans, G. C. (2010b). Effects of a Body-oriented Response Measure on the Neural Substrate of Imagined Perspective Rotations. J. Cogn. Neurosci. 22, 1782–1793. doi: 10.1162/jocn.2009.21319
Wraga, M., Shephard, J. M., Church, J. A., Inati, S., and Kosslyn, S. M. (2005). Imagined rotations of self versus objects: an fMRI study. Neuropsychologia 43, 1351–1361. doi: 10.1016/j.neuropsychologia.2004.11.028
Wraga, M., Thompson, W. L., Alpert, N. M., and Kosslyn, S. M. (2003). Implicit transfer of motor strategies in mental rotation. Brain Cogn. 52, 135–143. doi: 10.1016/S0278-2626(03)00033-2
Yan, J., Guo, X., Jin, Z., Sun, J., Shen, L., and Tong, S. (2012). Cognitive alterations in motor imagery process after left hemispheric ischemic stroke. PLoS ONE 7:e42922. doi: 10.1371/journal.pone.0042922
Yan, J., Sun, J., Guo, X., Jin, Z., Li, Y., Li, Z., et al. (2013). Motor imagery cognitive network after left ischemic stroke: study of the patients during mental rotation task. PLoS ONE 8:e77325. doi: 10.1371/journal.pone.0077325
Zacks, J. M. (2008). Neuroimaging studies of mental rotation: a meta-analysis and review. J. Cogn. Neurosci. 20, 1–19. doi: 10.1162/jocn.2008.20013
Zacks, J. M., Ollinger, J. M., Sheridan, M. A., and Tversky, B. (2002). A parametric study of mental spatial transformations of bodies. Neuroimage 16, 857–872. doi: 10.1006/nimg.2002.1129
Zacks, J. M., Vettel, J. M., and Michelon, P. (2003). Imagined viewer and object rotations dissociated with event-related FMRI. J. Cogn. Neurosci. 15, 1002–1018. doi: 10.1162/089892903770007399
Keywords: ALE meta-analysis, mental rotation, mental imagery, fMRI
Citation: Tomasino B and Gremese M (2016) Effects of Stimulus Type and Strategy on Mental Rotation Network: An Activation Likelihood Estimation Meta-Analysis. Front. Hum. Neurosci. 9:693. doi: 10.3389/fnhum.2015.00693
Received: 11 August 2015; Accepted: 07 December 2015;
Published: 07 January 2016.
Edited by:
Lutz Jäncke, University of Zurich, SwitzerlandReviewed by:
Fred W. Mast, University of Bern, SwitzerlandPetra Jansen, University of Regensburg, Germany
Copyright © 2016 Tomasino and Gremese. This is an open-access article distributed under the terms of the Creative Commons Attribution License (CC BY). The use, distribution or reproduction in other forums is permitted, provided the original author(s) or licensor are credited and that the original publication in this journal is cited, in accordance with accepted academic practice. No use, distribution or reproduction is permitted which does not comply with these terms.
*Correspondence: Barbara Tomasino, YnRvbWFzaW5vQHVkLmxuZi5pdA==