- 1Department of Neurorehabilitation, Department of Neurology, Tianjin Huanhu Hospital, Tianjin Key Laboratory of Cerebrovascular and Neurodegenerative Diseases, Tianjin, China
- 2Department of Rehabilitation Medicine, Tianjin Huanhu Hospital, Tianjin Key Laboratory of Cerebrovascular and Neurodegenerative Diseases, Tianjin, China
- 3Neurological Disease Biobank, Tianjin Neurosurgical Institute, Tianjin Huanhu Hospital, Tianjin Key Laboratory of Cerebrovascular and Neurodegenerative Diseases, Tianjin, China
Background: Constraint-induced movement therapy (CIMT) promotes upper extremity recovery post stroke, however, it is difficult to implement clinically due to its high resource demand and safety of the restraint. Therefore, we propose that modified CIMT (mCIMT) be used to treat individuals with acute subcortical infarction.
Objective: To evaluate the therapeutic effects of mCIMT in patients with acute subcortical infarction, and investigate the possible mechanisms underlying the effect.
Methods: The role of mCIMT was investigated in 26 individuals experiencing subcortical infarction in the preceding 14 days. Patients were randomly assigned to either mCIMT or standard therapy. mCIMT group was treated daily for 3 h over 10 consecutive working days, using a mitt on the unaffected arm for up to 30% of waking hours. The control group was treated with an equal dose of occupational therapy and physical therapy. During the 3-month follow-up, the motor functions of the affected limb were assessed by the Wolf Motor Function Test (WMFT) and Motor Activity Log (MAL). Altered cortical excitability was assessed via transcranial magnetic stimulation (TMS).
Results: Treatment significantly improved the movement in the mCIMT group compared with the control group. The mean WMF score was significantly higher in the mCIMT group compared with the control group. Further, the appearance of motor-evoked potentials (MEPs) were significantly higher in the mCIMT group compared with the baseline data. A significant change in ipsilesional silent period (SP) occurred in the mCIMT group compared with the control group. However, we found no difference between two groups in motor function or electrophysiological parameters after 3 months of follow-up.
Conclusions: mCIMT resulted in significant functional changes in timed movement immediately following treatment in patients with acute subcortical infarction. Further, early mCIMT improved ipsilesional cortical excitability. However, no long-term effects were seen.
Introduction
Stroke significantly increases the mortality and morbidity in the developed as well as developing world (Sudlow and Warlow, 1997; Terént, 2003; Truelsen et al., 2003; Mehndiratta et al., 2015). Despite varying levels of functional recovery, substantial sensorimotor and cognitive deficits persist in more than 50% of survivors, resulting in significant socioeconomic burden (Hendricks et al., 2002; Kim, 2014). Approximately 80% of stroke survivors manifest motor impairments associated with the upper limb (Langhorne et al., 2009; Momosaki et al., 2016). The degree of upper limb paresis is correlated with the basic activities of daily living (ADL) after stroke (Veerbeek et al., 2011; van Mierlo et al., 2016).
Constraint-induced movement therapy (CIMT) promotes movement of upper extremities affected by paralytic stroke. The major components of CIMT include intense repetitive (task-oriented) training and behavioral sharping of the impaired limb with immobilization of the unimpaired arm. Animal studies suggest that increased use of the affected limb overcame the reduced motor activity associated with cortical lesions (Nudo et al., 1996; Kleim et al., 1998). Evidence supports the effectiveness of CIMT in improving dexterity and motor function in individuals with chronic hemiplegia (Wolf et al., 1989; van der Lee et al., 1999; Taub, 2000). There are some limitations to widespread use of CIMT in stroke rehabilitation: first, original CIMT protocol requires constant supervision, therefore, it is more expensive than customary care. Second, original CIMT protocol need constraint of the unaffected hand for approximately 90% of waking hours, but some individuals with hemiplegia cannot tolerate this long limit, and there are also some security issues, especially in acute stroke patients. Compared with original CIMT protocol, the modified CIMT (mCIMT) protocols were feasible and well tolerated in acute stroke patients (Souza et al., 2015).
Although stroke damage can be devastating, many patients survive the initial event and undergo some spontaneous recovery, which can be further augmented by rehabilitative therapy. The first few weeks after stroke are vital for neuroplasticity and relearning of impaired activities (Dobkin, 2004; Kwakkel et al., 2006). Randomized controlled studies have demonstrated mCIMT could improve more affected limb use and function in acute or sub-acute cerebrovascular accident (Page et al., 2005; Singh and Pradhan, 2013).
The recovery of motor function in cortical injury varies from that of subcortical injury (Liu et al., 2015) and the effects of mCIMT in early subcortical ischemic stroke is not established. This study is undertaken to determine if mCIMT is effective in the early phase of subcortical ischemic stroke, and investigate the possible mechanisms underlying the effect. We hypothesized that mCIMT could improve functional outcomes of hemiplegic upper limb in patients with acute subcortical ischemic stroke compared with conventional occupational and physical therapy, and increase ipsilateral cortical excitability.
Materials and Methods
Study Setting and Trial Registration
In this single-center randomized controlled clinical trial, patients were recruited from November 2013 to January 2016. This study was approved by the ethics committee of Tianjin Huanhu Hospital. All the procedures involving human participants were approved by the ethics committee of Tianjin Huanhu Hospital and were in accordance with the 1964 Helsinki Declaration and its later amendments, or comparable ethical standards. All participants provided informed consent. In this single-center randomized clinical trial, we compared the upper extremity function between the group exposed to mCIMT and a dose-equivalent control group immediately after intervention and 3 months later. Transcranial magnetic stimulation (TMS) was used to assess changes in cortical excitability after treatment and follow-up. The study was registered with the Chinese Clinical Trial Registry (Registration number: ChiCTR-IOR-15005770).
Design and Participants
The inclusion criteria were: (1) stroke within 2 weeks of onset; (2) MRI showing subcortical ischemic stroke; (3) ability to raise two fingers with the forearm pronated on the table or lift the wrist 10° or more starting from a fully bent position; (4) respond to a 2-step command; and (5) a Mini Mental State Examination score exceeding 20. The exclusion criteria were: (1) inability to provide informed consent; (2) a history of stroke; (3) deviation greater than 2 cm on the line bisection test; (4) morbidity of the affected upper extremity resulting in functional limitation prior to stroke; (5) life expectancy less than 1 year; or (6) other neurological conditions affecting motor function or assessment (Thrane et al., 2015). Following informed consent, the patients were assigned to mCIMT or the control group using random odd- and even-numbered tickets in sealed envelopes. Patients selected one of the 60 sealed envelopes. Patients who selected tickets with even numbers represented the control group while those with odd numbers were allocated to mCIMT.
Interventions
The hemiplegic upper extremities in the mCIMT group were trained for 10 days by a licensed occupational therapist. All participants underwent 3 h per day of adaptive task practice and task training of the paretic limb (Wolf et al., 1989; van der Lee et al., 1999; Taub, 2000). Behavioral therapy comprised basic ADL together with skilled functional activities under supervision to improve motor performance. Positive feedback and increased gradations of difficulty were provided. Error data were provided after task training. Tasks with increasing levels of difficulty were assigned. In addition, patients carried a constraining mitt on the unaffected arm for nearly a third of their waking hours.
The control group was exposed to equal doses of traditional occupational therapy and physical therapy using a combination of neurodevelopmental techniques: bimanual tasks for the upper limbs, compensatory techniques for ADLs, strength and range of motion, positioning and mobility training.
Outcome Measurements
Primary outcomes included upper extremity motor function (tested with Wolf Motor Function Test (WMFT)) and a structured interview of real-world arm use with Motor Activity Log (MAL). The WMFT comprises 15 timed and two strength tasks (lifting the weighted limb and grip strength). The maximum time to complete a task was 120 s. If a trial was incomplete, the result was recorded as 121 s. The median time of all 15 tasks was used for analysis (Morris et al., 2001). The validity and reliability of the test had been demonstrated in stroke populations (Wolf et al., 2001, 2005; Nijland et al., 2010). The MAL was a structured interview comprising 30 standardized questions encompassing various ADL, which was used to assess the subjects’ subjective report of 30 common daily tasks. It included two assessment subscales that rate the more affected upper extremity: an amount of use (AOU) scale and a quality of movement (QOM) scale (Bonifer et al., 2005). The MAL was characterized by stability over a 2-week period with high internal consistency, high inter-rater and test-retest reliability (Taub, 2000). The tool was used extensively in CIMT studies. All participants were assessed after inclusion but before randomization, after 2 weeks and after 3 months.
Secondary outcome was the change of cortical excitability. Motor-evoked potentials (MEPs) and cortical silent period (SP) were examined by Dantec Keypoint 4c eletromyography (EMG; Medtronic A/S, Skovlunde, Denmark) with Danish Medtronic MagPro R30 (Medtronic A/S, Skovlunde, Denmark) magnetic stmulator and a focal figure-eight-shaped coil (outer diameter 4.5 cm). The maximum intensity of the magnetic field was 2.5 tesla. All patients were seated comfortably in the supine position. Surface EMG electrodes (filter bandpass: 20–10 kHz) were attached 3 cm apart over the muscle bellies of the abductor pollicis brevis. The 10–20 International electrode system was used for positioning of the TMS coil which located the electrodes on the scalp using standard cranial landmarks. Five stimulation positions were C4/C3, FC4/FC3, C5/C6, CP4/CP3 and C2/C1 according to the 10–20 International electrode system for measurements of cortical excitability. They were marked with an EEG cap, and stimulated with 90% of maximum stimulator output. The position at which stimuli at slightly suprathreshold intensity consistently yielded maximal MEPs in the contralateral abductor pollicis brevis was defined as “hot spot” (Bergmann et al., 2012). Subsequently, rest motor threshold (RMT) was defined according to the guidelines of the International Federation of Clinical Neurophysiology (IFCN) Committee as the minimum stimulus intensity eliciting MEPs of 0.50 mV in the resting muscle in at least 5 of 10 consecutive trials (Chen et al., 2008). The procedures were performed before the intervention and repeated similarly after the intervention and follow-up. Central motor conduction time (CMCT) was a neurophysiological measure that reflected conduction between the primary motor cortex and spinal cord. CMCT was calculated by subtracting the conduction time from the spinal roots to the muscle from the latency of MEPs evoked magnetically by transcranial cortical stimulation (Heald et al., 1993). In our previous study, cortical SP was an useful tool to predict outcome of acute stroke patients (Zhang et al., 2016). The SP had been proposed as an additional factor to the MEP for predicting motor recovery (van Kuijk et al., 2005). The length of the SP was measured from MEP onset until the return of uninterrupted voluntary EMG activity (Uozumi et al., 1991; Trompetto et al., 2000; Figure 1). When TMS was applied during isometric muscle contraction, cortical SP could be evoked following the MEP, which would be lasting up to 100–300 ms (Braune and Fritz, 1995). The intensity of stimulation was 120% RMT. The altered ipsilesional or contralesional MEPs and SPs, were calculated using a change ratio (Δ) as follows:
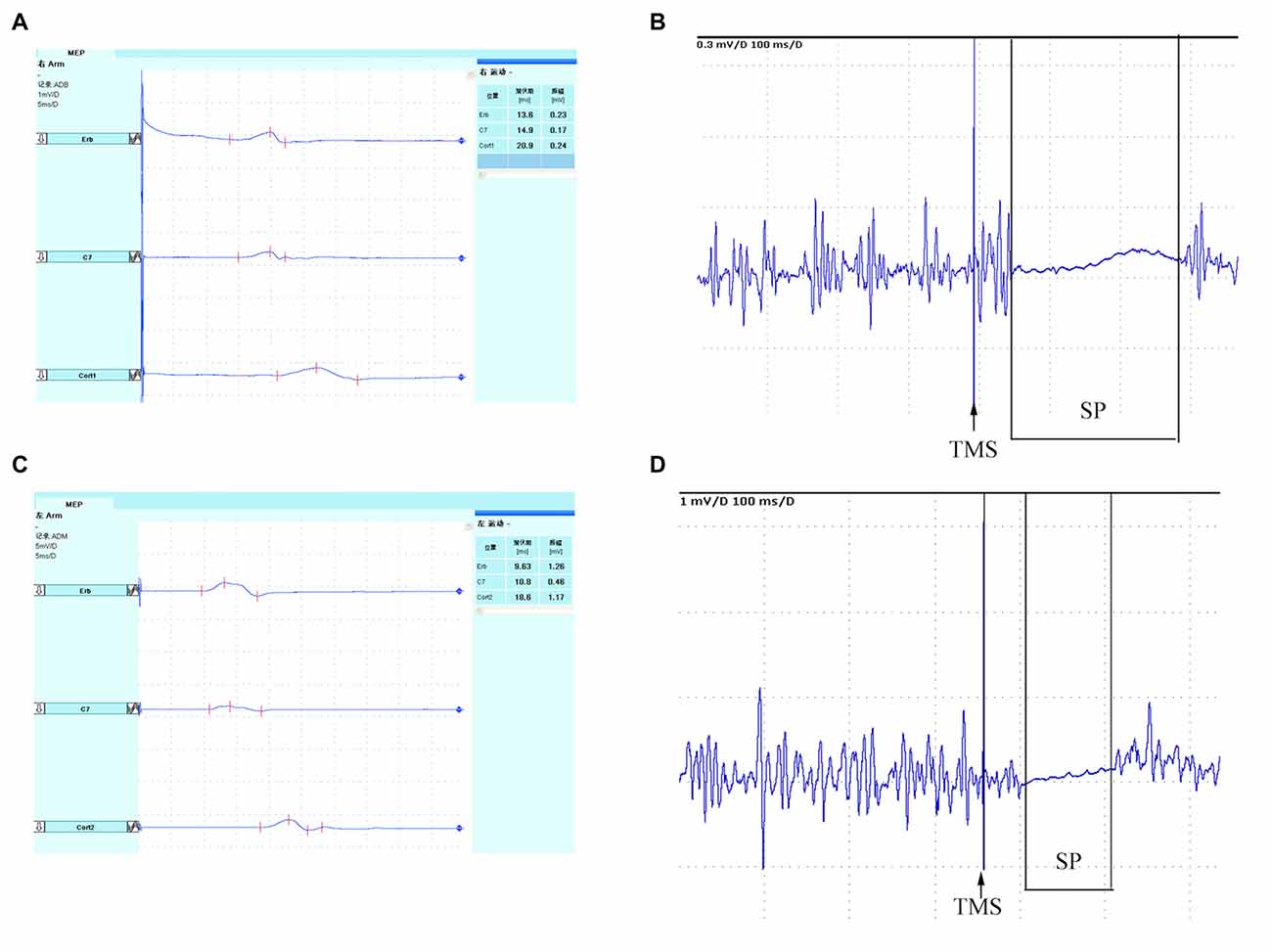
Figure 1. Schematic diagram of TMS-induced MEP. Bilateral MEPs and SP recorded in abductor pollicis brevis. Ipsilesional cortical (A) MEP and (B) SP, and Contralesional cortical (C) MEP and (D) SP. TMS, transcranial magnetic stimulation; MEP, motor-evoked potential; SP, silent period.
Statistical Analysis
SPSS version 21.0 package for Windows was used for all statistical analyses. Categorical variables were reported as proportions and continuous variables were reported as median values (interquartile range) or means ± standard deviations (SD). Baseline demographic variables were tested using independent t-test or Chi-square test. Differences between within-group inter-group or within-group analysis were determined by Mann-Whitney U-test or one-way analysis of variance (ANOVA) followed by Bonferroni multiple comparisons test. The level of statistical significance was set at P = 0.05.
Results
A total of 297 patients were screened and 29 eligible participants were selected between November 2013 and January 2016. Fifteen patients were assigned to mCIMT and 14 were enrolled in standard therapy. All the participants were inpatients, and no participant dropped out of the post-treatment assessments. One patient refused the 3-month follow-up, and another patient was lost to follow-up in the mCIMT group. Another patient was also lost to follow-up in the standard therapy group after 3 months. The flowchart outlining patient selection is presented in Figure 2.
Demographic Data
A total of 26 patients (22 men, 4 women) were enrolled and successfully followed up. No significant differences were seen between groups with medical comorbidities: 22 (88.5%) patients with hypertension, 15 (57.7%) with diabetes, three (11.5%) with atrial fibrillation, six (23.1%) with high homocysteine, and seven (27.9%) cases of stenosis of cerebral artery. The disease in the standard therapy group lasted from 2 days to 14 days with a mean of 6.15 ± 3.98 days. In contrast, the CIMT group lasted from 2 days to 14 days with a mean of 7.31 ± 3.86 days. Patient demographic and baseline data are described in Table 1.
Clinical Assessment
No significant differences in baseline data (pretreatment) were observed (Table 2). After 2 weeks of intervention, both groups showed an increase in ipsilateral upper limb motor function in the WMFT and MAL compared with baseline. A greater improvement in WMFT scores was observed in the CIMT group than in the standard therapy group (P < 0.001), and also in the extent of arm use (P = 0.038). However, other items of assessment scales were no different between the two groups. At 3-month follow-up, the scores of the QOM (MAL-QOM) and degree of arm use (MAL-AOU) were no different between mCIMT and standard therapy groups. The WMFT analysis yielded no differences in the functional ability between the groups.
Electrophysiology
TMS revealed similarities between the two groups with respect to baseline data (Table 3). After 2 weeks of intervention, MEPs were present in 10 (76.9%) patients in the CIMT group, with a significant improvement compared with baseline (P = 0.047). In the standard therapy group, the MEPs were observed in seven (53.8%) patients, with no difference compared with baseline (P = 0.695). Despite the absence of significant differences between the two groups, the presence of MEPs in mCIMT group were significantly higher than the pre-treatment levels. Concurrently, we found that the ipsilesional SP declined 21% compared with the baseline, which was statistically significant compared with the standard therapy group (P = 0.029). Other TMS parameters including contralesional SP and CMCT showed no significant changes from the standard therapy group. At 3 months of follow-up, both groups showed significant changes in ipsilesional SP compared with baseline (mCIMT p < 0.001; Control group P = 0.047). However, no differences were observed compared with each other.
Discussion
CIMT and mCIMT are most effective improving functional outcomes of the upper paretic limb (Kwakkel et al., 2015). Intermediate level of evidence supports mCIMT as an effective intervention for upper extremity hemiparesis after stroke (Uswatte et al., 2005). A single clinical trial involving hospitalized patients demonstrated significantly a higher total scores of the Action Research Arm Test and pinch subscale scores in the CIMT group immediately after therapy without follow-up assessment (Dromerick et al., 2000).
In this study, we used two standard clinical tests to assess upper motor function in patients with acute subcortical infarction. We observed a significant increase (of 1.18) in mean WMFT score (P < 0.001) in the mCIMT group after intervention (post-treatment) compared with the standard therapy, indicating that mCIMT promoted faster recovery. Although the mean WMFT time was not significantly improved in the mCIMT group after treatment, a downward trend was observed in the mean WMFT time. MAL scores below 0.27 in patients before intervention suggested occasional usage of their more affected arms for ADL tasks. Following intervention, subjects in the mCIMT group showed changes exceeding 2.0 points in the amount of use (AOU-MAL). The results suggested increased use of the affected upper limb for ADL tasks. The AOU scale scores were comparable to the results of previous mCIMT studies (Page et al., 2005; Wu et al., 2007). This study demonstrated that CIMT improved immediate motor function in patients with acute subcortical infarction.
After a follow-up of 3 months, no differences were seen in the WMFT and MAL scores between patients receiving mCIMT and standard therapies. Our result was consistent with a randomized controlled trial, which did not find a favorable effect of CIMT during the 6-month follow-up (Thrane et al., 2015). Recently, a home-based CIMT in patients with upper limb dysfunction after stroke showed that patients in both the groups showed improvement in the QOM. The home CIMT group outscored patients in the standard therapy group at 3 months, which was not consistent with our study. The patients in the home-based CIMT were recruited at least 6 months after stroke, and received 5 h of professional therapy in 4 weeks. Our study recruited patients at an earlier phase of stroke and all patients underwent shorter therapy.
A series of studies demonstrated increased cortical neuroplasticity in the subacute and chronic post-stroke phases of the brain (Liepert et al., 1998; Ro et al., 2006; Boake et al., 2007; Sawaki et al., 2008; Laible et al., 2012). However, studies conducted on patients with subcortical lesions are rare (Jang, 2007). Our study of patients with subcortical infarction displayed improved MEPs and ipsilesional SP in the mCIMT group after treatment, suggesting significant enhancement in cortical excitability of the lesion side. Studies showed that reduced ipsilesional SP level was a prognostic factor for spasticity in chronic stroke (Uozumi et al., 1992; Cruz Martínez et al., 1998). However, it may play a different role in acute stroke. Our earlier study demonstrated decreased ipsilesional SP levels during the first few days after acute cerebral infarction and significantly predicted the outcome within 3 months (Zhang et al., 2016). Patients with an SP value more than 217.05 showed a 7.69-fold increased risk of unfavorable outcomes compared with patients reporting an SP value less than 217.05. In the present study, we found that ipsilesional SP levels were significantly reduced immediately post-mCIMT compared with the control group. We speculate that reduced SP levels may reflect increased muscle tone and improved functional recovery in the acute phase of cerebral infarction.
Previous studies showed that recruitment of supplementary motor areas on the ipsilesional side enhanced the recovery. However, persistent activation of the contralesional cortex was associated with a slower and less complete recovery (Murphy and Corbett, 2009; Xerri, 2012). The present study found no changes in the SP, and CMCT in the contralesional sides of both groups. Our results suggested that the functional improvement of affected upper limb after treatment were associated with enhanced ipsilesional cortical excitability. At 3 months follow-up, significant change in ipsilesional SP was detected in mCIMT group compared with pre-treatment, and we also the similarly change in standard therapy groups. The reason for those changes may be that patients were not required to adhere to similar training at home (Table 4).
Our study limitations are as follows. First, the present study was a single-center clinical trial, and it was difficult to recruit eligible patients. Multi-centered trials are needed to increase the sample size. Second, using non-navigated TMS was a major limitation to the present study. It was well known that navigational systems allow TMS within a spatial deviation of few millimeters to a desired region of the cortex (Rossini and Rossi, 2007; Sparing et al., 2008). Using the International 10–20 electrode system for positioning of the TMS coil was not as accurate as navigational systems, but it was easily applicable in its practicable use. Moreover, using 10–20 electrode system enabled us not only to quickly retrieve the cortical region of interest but also shorten pre-evaluation related time, and avoid some security concerns resulting from navigated TMS in acute stroke patients. Third, we assessed changes of cortical excitability using TMS. However, single TMS pulses were unlikely to distinguish between stimulation of cortico-spinal, intra-cortical and trans-cortical elements, but instead target all three to varying degrees (Bestmann and Krakauer, 2015). We could not determine the contribution of other areas of the brain to the resultant output. Combination of TMS and brain functional imaging or specific stimulation protocols, such as paired-pulse stimulation could facilitate our understanding of neurophysiological mechanisms of stroke recovery.
Conclusion
Compared with standard therapy, mCIMT induced significant functional changes in acute subcortical ischemic stroke patients. Early intervention with mCIMT promotes ipsilesional cortical reorganization, without any long-term effect.
Author Contributions
JW contributed to the conception and design of the work; contributed in revising the work for important intellectual content. CY, WW, YZ, YW, WH, SL, CG, CW and LM contributed in the data acquisition. CY, WW and JW contributed in the analysis and interpretation of data for the work. CY and WW contributed in drafting the work. All authors approved the final version to be published, and agree to be accountable for all aspects of the work in ensuring that questions related to the accuracy or integrity of any part of the work are appropriately investigated and resolved.
Conflict of Interest Statement
The authors declare that the research was conducted in the absence of any commercial or financial relationships that could be construed as a potential conflict of interest.
Acknowledgments
This study was generously supported by Grants from Tianjin Public Health Bureau (2013KG122, to JW), and General Administration of Sport of China (2015B098, to JW), and Program to Establish Scientific Research Resources by Tianjin Municipal Health bureau (Establishment and Quality Control for biobanking of Neurological Diseases).
References
Bergmann, T. O., Möle, M., Schmidt, M. A., Lindner, C., Marshall, L., Born, J., et al. (2012). EEG-guided transcranial magnetic stimulation reveals rapid shifts in motor cortical excitability during the human sleep slow oscillation. J. Neurosci. 32, 243–253. doi: 10.1523/JNEUROSCI.4792-11.2012
Bestmann, S., and Krakauer, J. W. (2015). The uses and interpretations of the motor-evoked potential for understanding behaviour. Exp. Brain Res. 233, 679–689. doi: 10.1007/s00221-014-4183-7
Boake, C., Noser, E. A., Ro, T., Baraniuk, S., Gaber, M., Johnson, R., et al. (2007). Constraint-induced movement therapy during early stroke rehabilitation. Neurorehabil. Neural Repair 21, 14–24. doi: 10.1177/1545968306291858
Bonifer, N. M., Anderson, K. M., and Arciniegas, D. B. (2005). Constraint-induced therapy for moderate chronic upper extremity impairment after stroke. Brain Inj. 19, 323–330. doi: 10.1080/02699050400004302
Braune, H. J., and Fritz, C. (1995). Transcranial magnetic stimulation-evoked inhibition of voluntary muscle activity (silent period) is impaired in patients with ischemic hemispheric lesion. Stroke 26, 550–553. doi: 10.1161/01.str.26.4.550
Chen, R., Cros, D., Curra, A., Di Lazzaro, V., Lefaucheur, J. P., Magistris, M. R., et al. (2008). The clinical diagnostic utility of transcranial magnetic stimulation: report of an IFCN committee. Clin. Neurophysiol. 119, 504–532. doi: 10.1016/j.clinph.2007.10.014
Cruz Martínez, A., Muñoz, J., and Palacios, F. (1998). The muscle inhibitory period by transcranial magnetic stimulation. Study in stroke patients. Electromyogr. Clin. Neurophysiol. 38, 189–192.
Dobkin, B. H. (2004). Neurobiology of rehabilitation. Ann. N Y Acad. Sci. 1038, 148–170. doi: 10.1196/annals.1315.024
Dromerick, A. W., Edwards, D. F., and Hahn, M. (2000). Does the application of constraint-induced movement therapy during acute rehabilitation reduce arm impairment after ischemic stroke? Stroke 31, 2984–2988. doi: 10.1161/01.str.31.12.2984
Heald, A., Bates, D., Cartlidge, N. E., French, J. M., and Miller, S. (1993). Longitudinal study of central motor conduction time following stroke. 2. Central motor conduction measured within 72 h after stroke as a predictor of functional outcome at 12 months. Brain 116, 1371–1385. doi: 10.1093/brain/116.6.1371
Hendricks, H. T., van Limbeek, J., Geurts, A. C., and Zwarts, M. J. (2002). Motor recovery after stroke: a systematic review of the literature. Arch. Phys. Med. Rehabil. 83, 1629–1637. doi: 10.1053/apmr.2002.35473
Jang, S. H. (2007). A review of motor recovery mechanisms in patients with stroke. NeuroRehabilitation 22, 253–259.
Kim, J. S. (2014). Stroke in Asia: a global disaster. Int. J. Stroke 9, 856–857. doi: 10.1111/ijs.12317
Kleim, J. A., Barbay, S., and Nudo, R. J. (1998). Functional reorganization of the rat motor cortex following motor skill learning. J. Neurophysiol. 80, 3321–3325.
Kwakkel, G., Kollen, B., and Twisk, J. (2006). Impact of time on improvement of outcome after stroke. Stroke 37, 2348–2353. doi: 10.1161/01.str.0000238594.91938.1e
Kwakkel, G., Veerbeek, J. M., van Wegen, E. E., and Wolf, S. L. (2015). Constraint-induced movement therapy after stroke. Lancet Neurol. 14, 224–234. doi: 10.1016/S1474-4422(14)70160-7
Laible, M., Grieshammer, S., Seidel, G., Rijntjes, M., Weiller, C., and Hamzei, F. (2012). Association of activity changes in the primary sensory cortex with successful motor rehabilitation of the hand following stroke. Neurorehabil. Neural Repair 26, 881–888. doi: 10.1177/1545968312437939
Langhorne, P., Coupar, F., and Pollock, A. (2009). Motor recovery after stroke: a systematic review. Lancet Neurol. 8, 741–754. doi: 10.1016/S1474-4422(09)70150-4
Liepert, J., Miltner, W. H. R., Bauder, H., Sommer, M., Dettmers, C., Taub, E., et al. (1998). Motor cortex plasticity during constraint-induced movement therapy in stroke patients. Neurosci. Lett. 250, 5–8. doi: 10.1016/s0304-3940(98)00386-3
Liu, G., Dang, C., Peng, K., Xie, C., Chen, H., Xing, S., et al. (2015). Increased spontaneous neuronal activity in structurally damaged cortex is correlated with early motor recovery in patients with subcortical infarction. Eur. J. Neurol. 22, 1540–1547. doi: 10.1111/ene.12780
Mehndiratta, P., Wasay, M., and Mehndiratta, M. M. (2015). Implications of female sex on stroke risk factors, care, outcome and rehabilitation: an Asian perspective. Cerebrovasc. Dis. 39, 302–308. doi: 10.1159/000381832
Momosaki, R., Yasunaga, H., Kakuda, W., Matsui, H., Fushimi, K., and Abo, M. (2016). Very early versus delayed rehabilitation for acute ischemic stroke patients with intravenous recombinant tissue plasminogen activator: a nationwide retrospective cohort study. Cerebrovasc. Dis. 42, 41–48. doi: 10.1159/000444720
Morris, D. M., Uswatte, G., Crago, J. E., Cook, E. W. III, and Taub, E. (2001). The reliability of the wolf motor function test for assessing upper extremity function after stroke. Arch. Phys. Med. Rehabil. 82, 750–755. doi: 10.1053/apmr.2001.23183
Murphy, T. H., and Corbett, D. (2009). Plasticity during stroke recovery: from synapse to behaviour. Nat. Rev. Neurosci. 10, 861–872. doi: 10.1038/nrn2735
Nijland, R., van Wegen, E., Verbunt, J., van Wijk, R., van Kordelaar, J., and Kwakkel, G. (2010). A comparison of two validated tests for upper limb function after stroke: the wolf motor function test and the action research arm test. J. Rehabil. Med. 42, 694–696. doi: 10.2340/16501977-0560
Nudo, R. J., Wise, B. M., SiFuentes, F., and Milliken, G. W. (1996). Neural substrates for the effects of rehabilitative training on motor recovery after ischemic infarct. Science 272, 1791–1794. doi: 10.1126/science.272.5269.1791
Page, S. J., Levine, P., and Leonard, A. C. (2005). Modified constraint-induced therapy in acute stroke: a randomized controlled pilot study. Neurorehabil. Neural Repair 19, 27–32. doi: 10.1177/1545968304272701
Ro, T., Noser, E., Boake, C., Johnson, R., Gaber, M., Speroni, A., et al. (2006). Functional reorganization and recovery after constraint-induced movement therapy in subacute stroke: case reports. Neurocase 12, 50–60. doi: 10.1080/13554790500493415
Rossini, P. M., and Rossi, S. (2007). Transcranial magnetic stimulation: diagnostic,therapeutic and research potential. Neurology 68, 484–488. doi: 10.1212/01.wnl.0000250268.13789.b2
Sawaki, L., Butler, A. J., Leng, X., Wassenaar, P. A., Mohammad, Y. M., Blanton, S., et al. (2008). Constraint-induced movement therapy results in increased motor map area in subjects 3 to 9 months after stroke. Neurorehabil. Neural Repair 22, 505–513. doi: 10.1177/1545968308317531
Singh, P., and Pradhan, B. (2013). Study to assess the effectiveness of modified constraint-induced movement therapy in stroke subjects: a randomized controlled trial. Ann. Indian Acad. Neurol. 16, 180–184. doi: 10.4103/0972-2327.112461
Souza, W. C., Conforto, A. B., Orsini, M., Stern, A., and André, C. (2015). Similar effects of two modified constraint-induced therapy protocols on motor impairment, motor function and quality of life in patients with chronic stroke. Neurol. Int. 7:5430. doi: 10.4081/ni.2015.5430
Sparing, R., Buelte, D., Meister, I. G., Paus, T., and Fink, G. R. (2008). Transcranial magnetic stimulation and the challenge of coil placement: a comparison of conventional and stereotaxic neuronavigational strategies. Hum. Brain Mapp. 29, 82–96. doi: 10.1002/hbm.20360
Sudlow, C. L., and Warlow, C. P. (1997). Comparable studies of the incidence of stroke and its pathological types: results from an international collaboration. International Stroke Incidence Collaboration. Stroke 28, 491–499. doi: 10.1161/01.str.28.3.491
Taub, E. (2000). Constraint-induced movement therapy and massed practice. Stroke 31, 983–991. doi: 10.1161/01.STR.31.4.983-c
Terént, A. (2003). Trends in stroke incidence and 10-year survival in Söderhamn, Sweden, 1975–2001. Stroke 34, 1353–1358. doi: 10.1161/01.str.0000074038.71700.1c
Thrane, G., Askim, T., Stock, R., Indredavik, B., Gjone, R., Erichsen, A., et al. (2015). Efficacy of constraint-induced movement therapy in early stroke rehabilitation: a randomized controlled multisite trial. Neurorehabil. Neural Repair 29, 517–525. doi: 10.1177/1545968314558599
Trompetto, C., Assini, A., Buccolieri, A., Marchese, R., and Abbruzzese, G. (2000). Motor recovery following stroke: a transcranial magnetic stimulation study. Clin. Neurophysiol. 111, 1860–1867. doi: 10.1016/s1388-2457(00)00419-3
Truelsen, T., Mähönen, M., Tolonen, H., Asplund, K., Bonita, R., Vanuzzo, D., et al. (2003). Trends in stroke and coronary heart disease in the WHO MONICA Project. Stroke 34, 1346–1352. doi: 10.1161/01.str.0000069724.36173.4d
Uozumi, T., Ito, Y., Tsuji, S., and Murai, Y. (1992). Inhibitory period following motor potentials evoked by magnetic cortical stimulation. Electroencephalogr. Clin. Neurophysiol. 85, 273–279. doi: 10.1016/0168-5597(92)90116-s
Uozumi, T., Tsuji, S., and Murai, Y. (1991). Motor potentials evoked by magnetic stimulation of the motor cortex in normal subjects and patients with motor disorders. Electroencephalogr. Clin. Neurophysiol. 81, 251–256. doi: 10.1016/0168-5597(91)90010-u
Uswatte, G., Foo, W. L., Olmstead, H., Lopez, K., Holand, A., and Simms, L. B. (2005). Ambulatory monitoring of arm movement using accelerometry: an objective measure of upper-extremity rehabilitation in persons with chronic stroke. Arch. Phys. Med. Rehabil. 86, 1498–1501. doi: 10.1016/j.apmr.2005.01.010
van der Lee, J. H., Wagenaar, R. C., Lankhorst, G. J., Vogelaar, T. W., Devillé, W. L., and Bouter, L. M. (1999). Forced use of the upper extremity in chronic stroke patients: results from a single-blind randomized clinical trial. Stroke 30, 2369–2375. doi: 10.1161/01.str.30.11.2369
van Kuijk, A. A., Pasman, J. W., Geurts, A. C., and Hendricks, H. T. (2005). How salient is the silent period? The role of the silent period in the prognosis of upper extremity motor recovery after severe stroke. J. Clin. Neurophysiol. 22, 10–24. doi: 10.1097/01.WNP.0000150975.83249.71
van Mierlo, M. L., van Heugten, C. M., Post, M. W., Hajós, T. R., Kappelle, L. J., and Visser-Meily, J. M. (2016). Quality of life during the first two years post stroke: the restore 4 stroke cohort study. Cerebrovasc. Dis. 41, 19–26. doi: 10.1159/000441197
Veerbeek, J. M., Kwakkel, G., van Wegen, E. E., Ket, J. C., and Heymans, M. W. (2011). Early prediction of outcome of activities of daily living after stroke: a systematic review. Stroke 42, 1482–1488. doi: 10.1161/STROKEAHA.110.604090
Wolf, S. L., Catlin, P. A., Ellis, M., Archer, A. L., Morgan, B., and Piacentino, A. (2001). Assessing Wolf motor function test as outcome measure for research in patients after stroke. Stroke 32, 1635–1639. doi: 10.1161/01.str.32.7.1635
Wolf, S. L., Lecraw, D. E., Barton, L. A., and Jann, B. B. (1989). Forced use of hemiplegic upper extremities to reverse the effect of learned nonuse among chronic stroke and head-injured patients. Exp. Neurol. 104, 125–132. doi: 10.1016/s0014-4886(89)80005-6
Wolf, S. L., Thompson, P. A., Morris, D. M., Rose, D. K., Winstein, C. J., Taub, E., et al. (2005). The EXCITE trial: attributes of the Wolf motor function test in patients with subacute stroke. Neurorehabil. Neural Repair 19, 194–205. doi: 10.1177/1545968305276663
Wu, C. Y., Chen, C. L., Tsai, W. C., Lin, K. C., and Chou, S. H. (2007). A randomized controlled trial of modified constraint-induced movement therapy for elderly stroke survivors: changes in motor impairment, daily functioning, and quality of life. Arch. Phys. Med. Rehabil. 88, 273–278. doi: 10.1016/j.apmr.2006.11.021
Xerri, C. (2012). Plasticity of cortical maps: multiple triggers for adaptive reorganization following brain damage and spinal cord injury. Neuroscientist 18, 133–148. doi: 10.1177/1073858410397894
Keywords: constraint-induced movement therapy, rehabilitation, motor evoked potentials, cortical reorganization, acute subcortical stroke
Citation: Yu C, Wang W, Zhang Y, Wang Y, Hou W, Liu S, Gao C, Wang C, Mo L and Wu J (2017) The Effects of Modified Constraint-Induced Movement Therapy in Acute Subcortical Cerebral Infarction. Front. Hum. Neurosci. 11:265. doi: 10.3389/fnhum.2017.00265
Received: 13 July 2016; Accepted: 04 May 2017;
Published: 18 May 2017.
Edited by:
Srikantan S. Nagarajan, University of California, San Francisco, United StatesReviewed by:
Sandro M. Krieg, Technische Universität München, GermanyFilippo Brighina, University of Palermo, Italy
Copyright © 2017 Yu, Wang, Zhang, Wang, Hou, Liu, Gao, Wang, Mo and Wu. This is an open-access article distributed under the terms of the Creative Commons Attribution License (CC BY). The use, distribution or reproduction in other forums is permitted, provided the original author(s) or licensor are credited and that the original publication in this journal is cited, in accordance with accepted academic practice. No use, distribution or reproduction is permitted which does not comply with these terms.
*Correspondence: Jialing Wu, d3l3amwyMDA5QGhvdG1haWwuY29t
† These authors have contributed equally to this work.