- 1Neurology Unit, Department of Clinical and Experimental Sciences, University of Brescia, Brescia, Italy
- 2Stroke Unit, Azienda Socio Sanitaria Territoriale Spedali Civili, Brescia, Italy
- 3U.O. Neurologia, Fondazione Poliambulanza Hospital, Brescia, Italy
- 4Neurology Unit, Movement Disorders Division, Department of Neurosciences, Biomedicine and Movement Sciences, University of Verona, Verona, Italy
Background: Functional neurological disorders are characterized by neurological symptoms that have no identifiable pathology and little is known about their underlying pathophysiology.
Objectives: To analyze motor cortex excitability and intracortical inhibitory and excitatory circuits’ imbalance in patients with flaccid functional weakness.
Methods: Twenty-one consecutive patients with acute onset of flaccid functional weakness were recruited. Single and paired-pulse transcranial magnetic stimulation (TMS) protocols were used to analyze resting motor thresholds (RMT) and intracortical inhibitory (short interval intracortical inhibition – SICI) and excitatory (intracortical facilitation – ICF) circuits’ imbalance between the affected and non-affected motor cortices.
Results: We observed a significant increase in RMT and SICI in the affected motor cortex (p < 0.001), but not for ICF, compared to the contralateral unaffected side.
Conclusion: This study extends current knowledge of functional weakness, arguing for a specific central nervous system abnormality which may be involved in the symptoms’ pathophysiology.
Introduction
Functional neurological disorders (FND) are characterized by neurological symptoms that have no identifiable, responsible pathology (American Psychiatric Association, 2013; Edwards et al., 2014; Roelofs et al., 2019; Baizabal-Carvallo and Jankovic, 2020). Functional disorders are common in neurological practice, accounting up to 15% of new referrals in neurology clinics (Stone et al., 2010). Despite their common occurrence, little is known about the underlying pathophysiology and treatment, also considering that they have a poor prognosis at long term follow-up, with high levels of physical disability (Espay et al., 2006; Avanzino et al., 2008; Quartarone et al., 2009). Individuals with FND have traditionally been left in a therapeutic void, with neither neurologist nor psychiatrics able to provide adequate strategies for improvement (Pringsheim and Edwards, 2017).
Recent studies have shown that patients with FNDs are not different psychologically from individuals with neurologic movement disorders, and that a relevant proportion of individuals with FND have no psychopathology detectable on symptom screening measures (van der Hoeven et al., 2015). These symptoms are thought to result from a distorted mind-body relationship in self-awareness, with a functional mismatch between beliefs/expectations and sensory information related to the symptom (Edwards et al., 2013), rather than an intention to obtain earning or help (Espay et al., 2018). However, researchers are continuing to explore the psychological underpinnings of FND, still debating whether to support the Freudian hypothesis of conversion or to embrace a neurologic (organic) explanation for the disorder (Pringsheim and Edwards, 2017).
Among FNDs, a great proportion is determined by patients presenting with sudden weakness, mimicking an acute stroke (stroke-mimic) (Carson et al., 2000). In functional weakness and paralysis, the motor system, from motor cortex to muscle, seems to be unaffected. To date, the diagnosis is based exclusively on ruling out organic disorders, while we still lack an objective measure of disease.
A possible marker could reside in the functional evaluation of the motor cortex, but mixed results have been reported regarding motor cortex excitability and intracortical inhibitory circuits, evaluated with transcranial magnetic stimulation (TMS) (Premi et al., 2017). These studies were performed in small groups of patients, including both flaccid and spastic-dystonic paralysis.
TMS has already been proven useful to assess non-invasively and in vivo several inhibitory and excitatory intracortical circuits, as well as several parameters of cortical plasticity (Rossini et al., 2015). These techniques have been successfully applied in several studies, which have included stroke, movement disorders and neurodegenerative diseases (Motta et al., 2018; Koch et al., 2019; Benussi et al., 2020).
Abnormalities in frontal, parietal and limbic influences on the motor system have recently emerged (Kanaan et al., 2007; Stone et al., 2007; Cojan et al., 2009; Demartini et al., 2019), suggesting for a top-down inhibition of the motor system causing weakness or paralysis (Hallett, 2016). In particular, these studies have provided novel insights into the possible neural mechanisms involved in functional paralysis, by showing that unilateral paralysis was associated not only with a suppressed activation of the motor cortex during attempted movements but also with changes in its functional connectivity, including greater recruitment of the precuneus and ventromedial prefrontal cortex regions that are critical for accessing self-related representations and memories. Moreover, no evidence has emerged that brain regions normally implicated in conscious motor inhibition (such as the inferior frontal gyrus) are responsible for the paralysis (Cojan et al., 2009).
In this view, FNDs would represent a proper neurological disorder, beyond the possible presence of a specific psychological or psychiatric trait (Edwards et al., 2013).
The objective of this study was to evaluate possible abnormalities in motor cortex excitability between the affected and unaffected motor cortices in a relatively large cohort of well characterized patients with flaccid functional weakness, mimicking an acute stroke, by applying paired-pulse TMS protocols measuring short interval intracortical inhibition (SICI) and intracortical facilitation (ICF).
Methods
Participants
Twenty-one patients were recruited from the Stroke Unit, ASST Spedali Civili Hospital, Brescia, and from the Neurology Unit, Fondazione Poliambulanza Hospital, Brescia, Italy with an acute onset functional paralysis (flaccid hemiparesis, 7 right-sided, 14 left-sided), admitted for a clinical suspect of ischemic stroke with acute hemiparesis.
Brain CT scan was unremarkable and some patients (n = 11) were treated with recombinant tissue plasminogen activator if clinical and temporal inclusion criteria were satisfied (Powers et al., 2019).
At 24 h follow-up, brain 1.5T or 3T MRI scans with T1, T2, T2-Fluid Attenuated Inversion Recovery (FLARI) and Diffusion Weighted Images (DWI) sequences resulted unremarkable and ruled out a cerebrovascular event or any other abnormality that could have possible explained the symptomatology.
At discharge (range: 4–7 days after admission), global neurological examinations showed a persistent hemiparesis. At follow-up (mean disease duration: 22.5 ± 31.5 months), considering the persistency of clinical symptoms, brain and cervical spine MRI, and EMG-ENG were performed in all patients to exclude lesions in the cervical spinal cord (i.e., myelopathy) or peripheral nerves. All tests were unremarkable and excluded an organic lesion. Central motor conduction time (CMCT) was not evaluated in all patients, thus possible subclinical myelopathies could have been undiagnosed.
The functional etiology was also defined according to the Carson scale (Carson et al., 2000) (1 = not at all; 2 = somewhat; 3 = largely; 4 = completely) based on the assessment of the premorbid psychological status by Minnesota Multiphasic Personality Inventory 2 (MMPI-2) (Tellegen and Ben-Porath, 1993) and the Italian version of the Symptom Rating Test (Fava et al., 1983). Functional etiology was confirmed by unremarkable instrumental examination, including the assessment of the premorbid psychological status, which supported the presence of a largely (n = 13) or completely (n = 8) functional etiology, in line with the Carson scale (Carson et al., 2000). None of the patients were excluded and all met criteria for a functional etiology.
Patients were screened for depression using the Zung Self-Rating Depression Scale (Zung, 1965).
None of the patients were being treated with drugs that could have altered the cerebral cortex excitability at TMS evaluation.
Informed consent was acquired from all participants in accordance to the Declaration of Helsinki. The local ethics committee of the Brescia Hospital approved the present study (05.19.2015, #NP1965).
70 healthy subjects (age 44.9 ± 16.5) who underwent TMS on the left motor cortex were included as a control group.
Transcranial Magnetic Stimulation Variables and Protocols
A TMS figure-of-eight coil (each loop diameter 70 mM – D702 coil) connected to a monophasic Magstim Bistim2 system (Magstim Company, Oxford, United Kingdom) was employed for all TMS paradigms, as previously reported (Benussi et al., 2018a). Motor evoked potentials (MEPs) were recorded from the right and left first dorsal interosseous (FDI) muscles through surface Ag/AgCl electrodes placed in a belly-tendon montage and acquired using a Biopac MP-150 electromyograph (BIOPAC Systems Inc., Santa Barbara, CA, United States). Responses were amplified and filtered at 20 Hz and 2 kHz with a sampling rate of 5 kHz and recorded on a personal computer for offline elaboration (AcqKnowledge 4.1, BIOPAC Systems Inc., Santa Barbara, CA, United States).
Resting motor threshold (RMT) was determined on both motor cortices as the minimum intensity of the stimulator required to elicit motor evoked potentials (MEPs) with a 50 μV amplitude in 50% of 10 consecutive trails, recorded form the right or left first dorsal interosseous muscles during full relaxation (Rossini et al., 2015). Moreover, MEP latencies were measured on both sides at an intensity of 120% RMT.
SICI and ICF were studied using a paired-pulse technique, employing a conditioning-test design. For all paradigms, the test stimulus (TS) was adjusted to evoke a MEP of ∼1 mv amplitude in the right and left FDI muscles (Benussi et al., 2018b).
The conditioning stimulus (CS) was adjusted at 70% of the RMT, employing multiple interstimulus intervals (ISIs), including 1, 2, 3 ms for SICI and 7, 10, 15 ms for ICF (Kujirai et al., 1993; Ziemann et al., 1996). For each ISI and for each protocol, ten different paired CS-TS stimuli and fourteen control TS stimuli were delivered in all participants in a pseudo randomized sequence, with an inter trial interval of 5 s (± 10%). Patients were stimulated on the left side first in ∼50% trails in order to reduce possible effects of attention and adaptation to the TMS pulses and thus on motor cortex excitability measures.
The conditioned MEP amplitude, evoked after delivering a paired CS-TS stimulus, was expressed as percentage of the average control MEP amplitude. Audio-visual feedback was provided to ensure muscle relaxation during the entire experiment and trials were rejected if electromyographic activity was greater that 100 μV in the 250 ms before TMS stimulus application. Less that 2% of trials were discarded for each protocol. All of the participants were capable of following instructions and reaching complete muscle relaxation; if, however, the data was corrupted by patient movement, the protocol was restarted and the initial recording was rejected.
Statistical Analysis
TMS evoked responses were compared using one-way repeated measures ANOVA (for RMT and MEP latency) or two-way repeated measures ANOVA (for SICI and ICF) with SIDE (affected vs. unaffected) and ISI (1, 2, 3, 7, 10, 15 ms) as within-subjects factor. If a significant main effect was observed, group differences were evaluated with post hoc tests (p-values are reported after Bonferroni correction for multiple comparisons). Mauchly’s test was used to check for sphericity violation. Pearson’s correlation co-efficient was used to investigate any association between differences in RMT and SICI scores between sides and with NIH Stroke Scale scores (Pezzella et al., 2009).
Data analyses were carried out using SPSS 21.0 software.
Results
Participants
Demographic and clinical characteristics are reported in Table 1. We did not observe a significant association between handedness and side of the hemiparesis, as assessed by Fisher’s exact test (p = 0.533). NIH Stroke Scale scores were 3.8 ± 1.4 and 2.4 ± 0.7 at onset and at TMS, respectively.
Transcranial Magnetic Stimulation
In one patient, RMT could not be measured on the affected side because the motor cortex was unexcitable at 80% of the maximum stimulator output (% MSO) (intensities between 80 and 100% MSO were not tested for patient discomfort), and was excluded from analysis.
No significant differences in MEP latencies between sides were observed, F(1,19) = 0.42, p = 0.524, partial η2 = 0.02 (see Table 2).
One-way repeated measures ANOVA showed a significant difference in RMT scores between the affected and the unaffected side, F(1,19) = 26.62, p < 0.001, partial η2 = 0.58, with a significantly increased RMT in the affected motor cortex (49.1 ± 9.0% MSO) compared to the unaffected one (43.3 ± 7.3% MSO) (see Table 2).
RMT was significantly increased in the affected side compared to healthy controls, F(1,89) = 5.07, p = 0.027, partial η2 = 0.05, but not in the unaffected side, F(1,89) = 0.20, p = 0.656, partial η2 < 0.01 (see Table 2).
Two-way repeated measures ANOVA highlighted a significant ISI × GROUP interaction for SICI-ICF, F(5,95) = 3.87, p = 0.003, partial η2 = 0.17. Post hoc tests, with Bonferroni corrections for multiple comparisons, showed a significant difference in MEP amplitudes between the affected and unaffected side at ISI 1, 2, 3 ms (for all p < 0.001) but not at ISI 7, 10, 15 ms (for all p > 0.005) (see Figure 1), with a significantly increased SICI in the affected (average 0.18 ± 0.05) compared to the unaffected side (average 0.39 ± 0.10) (see Table 2).
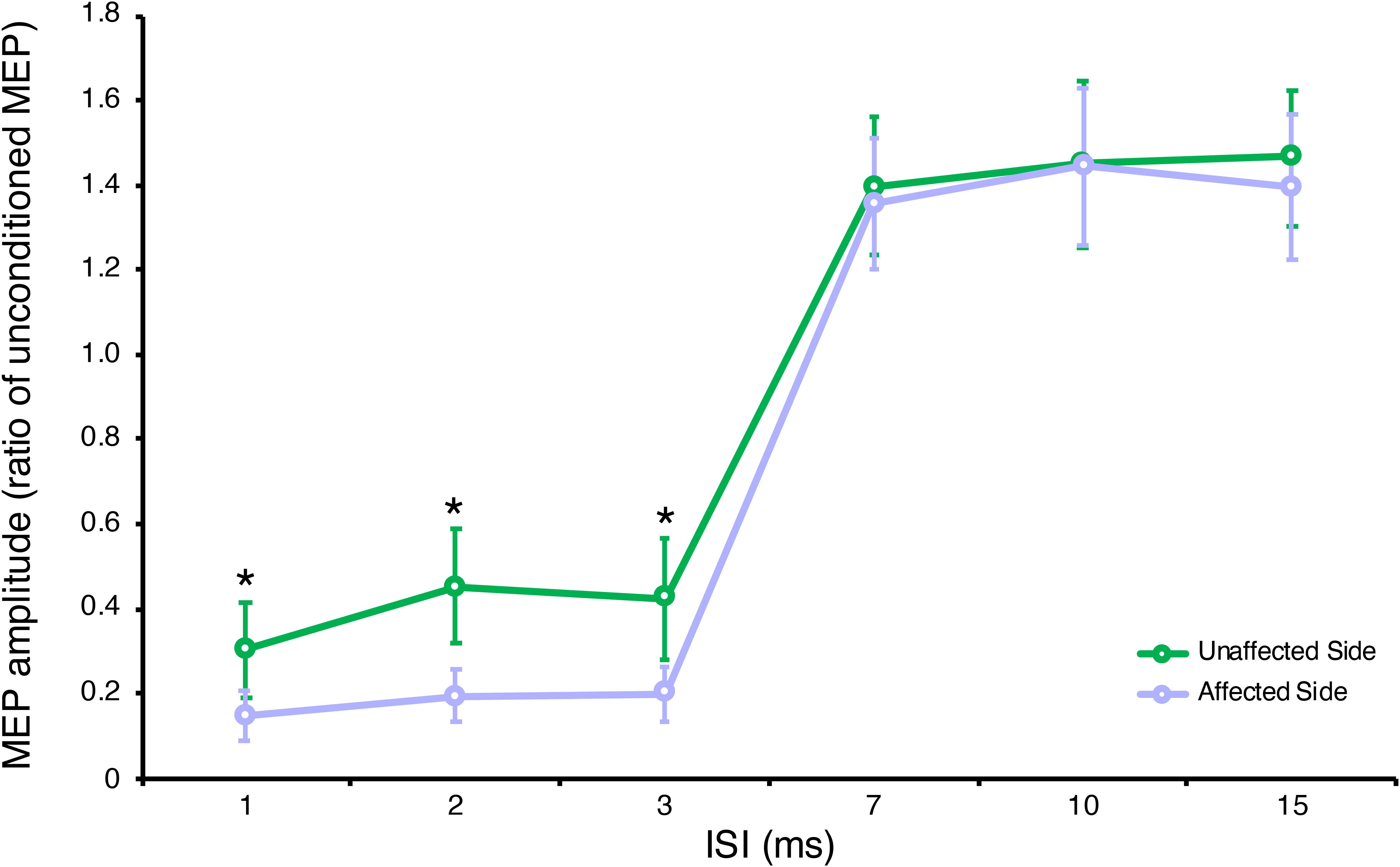
Figure 1. Neurophysiological parameters in the affected and unaffected sides. Short-interval intracortical inhibition (SICI) at ISI 1, 2, 3 ms and intracortical facilitation (ICF) at ISI 7, 10, 15 ms. Data are represented as a ratio to the unconditioned MEP amplitude; error bars represent standard errors. MEP, motor evoked potential; ISI, inter stimulus interval. *p < 0.05 vs. contralateral side using one-way ANOVA (post hoc tests with Bonferroni correction for multiple comparisons).
There was no significant correlation between the difference in RMT measures between sides and the difference in average SICI between sides (r = 0.149, p = 0.531).
We observed a significant correlation between NIH Stroke Scale scores and average SICI in the affected side (r = 0.601, p = 0.005), but not in the unaffected side, nor with RMT or SICI (both affected and unaffected sides), all p > 0.05.
Compared to healthy controls, we observed a significant ISI × GROUP interaction for SICI-ICF in the affected, F(6,528) = 8.73, p < 0.001, partial η2 = 0.09, and unaffected sides, F(6,528) = 3.41, p = 0.003, partial η2 = 0.04. Post hoc tests, with Bonferroni corrections for multiple comparisons, showed a significant increase in SICI at ISI 2 ms (p = 0.022) in the affected side compared to healthy controls, and a significant decrease in SICI at ISI 1, 2, 3 ms (all p < 0.001) in the unaffected side compared to healthy controls.
Discussion
In the present study, carried out in a well characterized group of patients with long-lasting flaccid functional paralysis, we observed an imbalance of intracortical inhibition between the affected and unaffected motor cortices. In particular we observed an increase in motor threshold and intracortical inhibition, evaluated with the SICI paired-pulse TMS protocol. SICI is thought to reflect short-lasting post-synaptic inhibition mediated through GABAergic interneurons, modulating the activity of the corticospinal output. In this case, an increase in SICI could reflect an increase of inhibitory intracortical circuits affecting corticospinal excitability, possibly inducing a decrease in voluntary movements.
Previous studies have shown that patients with functional paralysis show a paradoxical suppression of the MEP amplitude after motor imagery in the affected side, opposite to what is observed in healthy subjects, suggesting for a disturbed control of voluntary movements. Conversely, action observation has shown to induce an increase in motor cortex excitability similar to healthy controls (Liepert et al., 2011). One of these studies, performed on 3 patients with flaccid paralysis, did not observe any significant differences in SICI or ICF between the affected and unaffected side (Liepert et al., 2008). Subsequently, another study performed on 4 patients with functional paralysis showed a significant increase in SICI and a non-significant increase in RMT in the affected side compared to healthy controls (Premi et al., 2017).
Several studies using functional neuroimaging have now shown that frontal areas are dysfunctional and particularly strongly connected to the affected motor cortex (Cojan et al., 2009; Nowak and Fink, 2009; Hallett, 2016. Interestingly, long-lasting functional paralysis has been associated with greater cortical thickness/density in motor areas, suggesting that functional brain abnormalities lead to structural changes when disease is sustained over time (Aybek et al., 2014).
Interestingly, patients with depression exhibit a significant interhemispheric difference in motor cortex excitability, an imbalanced inhibitory and excitatory intracortical circuitry, and an impaired long-term potentiation-like response to paired-associative transcranial magnetic stimulation, reinforcing the possible parallelism between FND and psychological comorbidity (Cantone et al., 2017).
In line with previous studies on FND, the majority of patients in this study were represented by females. There is currently no clear scientific explanation for the differences in gender prevalence favoring females but it can be argued that neurobiological, hormonal, cultural, social, and previous history of psychological or sexual trauma may be relevant contributors (Baizabal-Carvallo and Jankovic, 2020; Edwards and Aybek, 2020).
Interestingly, in one patient we could not evoke a reliable MEP on the affected motor cortex, which is somewhat contradictory with previous studies, in which patients with psychogenic paralysis had normal MEPs, confirming the physiological integrity of motor fibers in the corticospinal tract, anterior roots and plexuses (Cantello et al., 2001).
Other than evaluating intracortical circuits and corticospinal tract integrity, TMS may be also used as a therapeutic option in patients with FND. It has been demonstrated that TMS may exert a therapeutic effect via genuine neuromodulation, via non-specific placebo effects and by demonstrating, through its immediate effects on the motor system (i.e., movement in a “paretic” limb), that symptom improvement is possible, thus directly changing higher level beliefs that may be responsible for the maintenance of the disorder (Pollak et al., 2014; Schönfeldt-Lecuona et al., 2016; Garcin et al., 2017).
We acknowledge that this study entails some limitations. Firstly, it is not known if these modifications in cortical excitability are the cause or consequence of the functional disorder. To this, further studies should assess cortical excitability in the acute phase of symptoms’ onset. Supporting these findings and arguing for a causal role of increased SICI in functional hemiparesis are previous findings observed at only 1 month after symptom onset in patients with functional paralysis (Premi et al., 2017). Moreover, these findings should be compared with patients with organic hemiparesis (i.e., after stroke), in order to shed further light on disease pathophysiology.
Moreover, it would have been interesting to assess the excitability of the motor cortex with further measures, primarily the input–output (IO) curves and TMS mapping. The IO curves can be easily measured by plotting the amplitude of motor evoked potentials (MEP) against a range of different stimulus intensities, and are sensitive measures of changes in neuronal system excitability (Ridding and Rothwell, 1997). TMS mapping, i.e., the assessing of the area (number of scalp positions from which a MEP can be elicited) and volume (the sum of the averaged MEP amplitudes for each excitable scalp site) of the cortical representation of a target muscle is relatively more time consuming but provides interesting information; in stroke, for example, hand muscles of the paretic side are underrepresented (Marconi et al., 2007). Another relevant aspect could have been to investigate interhemispheric connectivity between the two motor cortices, using a paired coil method to test interhemispheric inhibition (IHI) (Ferbert et al., 1992). In stroke survivors, a disruption of IHI from the unaffected to the affected motor cortex via glutamatergic transcallosal fibers can interfere with motor performance of the paretic limb (McDonnell and Stinear, 2017). Moreover, EEG analysis should be performed in functional paralysis, possibly providing novel aspects in disease pathophysiology (Cojan et al., 2009). Finally, the small sample size and the gender imbalance may preclude generalization of these results to larger populations.
Conclusion
The asymmetry/imbalance of SICI between the affected and unaffected motor cortices could represent a potential tool to support the diagnosis of FND in flaccid hemiparesis. To this, further studies on larger samples are warranted, combining clinical, electrophysiological and neuroimaging approaches for a more comprehensive assessment.
Data Availability Statement
The datasets generated for this study are available on request to the corresponding author.
Ethics Statement
The studies involving human participants were reviewed and approved by the local ethics committee of the Brescia Hospital. The patients/participants provided their written informed consent to participate in this study.
Author Contributions
(1) Research project: (A) EP, AB, and BB: conception; (B) EP, AB, BB, and MM: organization; (C) EP, AB, VC, SC, EM, NG, VV, ID, MG, RS, AC, MT, AP, BB, and MM: execution. (2) Statistical analysis: (A) EP and AB: design; (B) EP and AB: execution; (C) EP, AB, and BB: review and critique. (3) Manuscript preparation: (A) EP, AB, and BB: writing of the first draft; (B) EP, AB, VC, SC, EM, NG, VV, ID, MG, RS, AC, MT, AP, BB, and MM: review and critique.
Conflict of Interest
The authors declare that the research was conducted in the absence of any commercial or financial relationships that could be construed as a potential conflict of interest.
References
American Psychiatric Association (2013). Diagnostic and Statistical Manual of Mental Disorders. Maine Avenue SW: American Psychiatric Association.
Avanzino, L., Martino, D., van de Warrenburg, B. P. C., Schneider, S. A., Abbruzzese, G., Defazio, G., et al. (2008). Cortical excitability is abnormal in patients with the “fixed dystonia” syndrome. Mov. Disord. 23, 646–652. doi: 10.1002/mds.21801
Aybek, S., Nicholson, T. R. J., Draganski, B., Daly, E., Murphy, D. G., David, A. S., et al. (2014). Grey matter changes in motor conversion disorder. J. Neurol. Neurosurg. Psychiatry 85, 236–238. doi: 10.1136/jnnp-2012-304158
Baizabal-Carvallo, J. F., and Jankovic, J. (2020). Gender differences in functional movement disorders. Mov. Disord. Clin. Pract. 7, 182–187. doi: 10.1002/mdc3.12864
Benussi, A., Alberici, A., Ferrari, C., Cantoni, V., Dell’Era, V., Turrone, R., et al. (2018a). The impact of transcranial magnetic stimulation on diagnostic confidence in patients with Alzheimer disease. Alzheimers Res. Ther. 10:94. doi: 10.1186/s13195-018-0423-6
Benussi, A., Dell’Era, V., Cantoni, V., Ferrari, C., Caratozzolo, S., Rozzini, L., et al. (2018b). Discrimination of atypical parkinsonisms with transcranial magnetic stimulation. Brain Stimul. 11, 366–373. doi: 10.1016/j.brs.2017.11.013
Benussi, A., Grassi, M., Palluzzi, F., Koch, G., Di Lazzaro, V., Nardone, R., et al. (2020). Classification accuracy of transcranial magnetic stimulation for the diagnosis of neurodegenerative dementias. Ann. Neurol. 87, 394–404. doi: 10.1002/ana.25677
Cantello, R., Boccagni, C., Comi, C., Civardi, C., and Monaco, F. (2001). Diagnosis of psychogenic paralysis: the role of motor evoked potentials. J. Neurol. 248, 889–897. doi: 10.1007/s004150170075
Cantone, M., Bramanti, A., Lanza, G., Pennisi, M., Bramanti, P., and Pennisi, G. (2017). Cortical plasticity in depression: a neurochemical perspective from transcranial magnetic stimulation. ASN Neuro 9:1759091417711512. doi: 10.1177/1759091417711512
Carson, A. J., Ringbauer, B., Stone, J., McKenzie, L., Warlow, C., and Sharpe, M. (2000). Do medically unexplained symptoms matter? A prospective cohort study of 300 new referrals to neurology outpatient clinics. J. Neurol. Neurosurg. Psychiatry 68, 207–210. doi: 10.1136/jnnp.68.2.207
Cojan, Y., Waber, L., Carruzzo, A., and Vuilleumier, P. (2009). Motor inhibition in hysterical conversion paralysis. Neuroimage 47, 1026–1037. doi: 10.1016/J.NEUROIMAGE.2009.05.023
Demartini, B., Gambini, O., Uggetti, C., Cariati, M., Cadioli, M., Goeta, D., et al. (2019). Limbic neurochemical changes in patients with functional motor symptoms. Neurology 93:e52–e58. doi: 10.1212/WNL.0000000000007717
Edwards, M. J., and Aybek, S. (2020). Gender, abuse, and functional movement disorders: from his-story to the future. Mov. Disord. Clin. Pract. 7, 167–168. doi: 10.1002/mdc3.12887
Edwards, M. J., Fotopoulou, A., and Pareés, I. (2013). Neurobiology of functional (psychogenic) movement disorders. Curr. Opin. Neurol. 26, 442–447. doi: 10.1097/WCO.0b013e3283633953
Edwards, M. J., Stone, J., and Lang, A. E. (2014). From psychogenic movement disorder to functional movement disorder: it’s time to change the name. Mov. Disord. 29, 849–852. doi: 10.1002/mds.25562
Espay, A. J., Aybek, S., Carson, A., Edwards, M. J., Goldstein, L. H., Hallett, M., et al. (2018). Current concepts in diagnosis and treatment of functional neurological disorders. JAMA Neurol. 75, 1132–1141. doi: 10.1001/jamaneurol.2018.1264
Espay, A. J., Morgante, F., Purzner, J., Gunraj, C. A., Lang, A. E., and Chen, R. (2006). Cortical and spinal abnormalities in psychogenic dystonia. Ann. Neurol. 59, 825–834. doi: 10.1002/ana.20837
Fava, G. A., Kellner, R., Perini, G. I., Fava, M., Michelacci, L., Munari, F., et al. (1983). Italian validation of the symptom rating test (SRT) and symptom questionnaire (SQ). Can. J. Psychiatry 28, 117–123. doi: 10.1177/070674378302800208
Ferbert, A., Priori, A., Rothwell, J. C., Day, B. L., Colebatch, J. G., and Marsden, C. D. (1992). Interhemispheric inhibition of the human motor cortex. J. Physiol. 453, 525–546. doi: 10.1113/jphysiol.1992.sp019243
Garcin, B., Mesrati, F., Hubsch, C., Mauras, T., Iliescu, I., Naccache, L., et al. (2017). Impact of transcranial magnetic stimulation on functional movement disorders: cortical modulation or a behavioral effect? Front. Neurol. 8:338. doi: 10.3389/fneur.2017.00338
Hallett, M. (2016). Neurophysiologic Studies of Functional Neurologic Disorders, 1st Edn, Vol. 139. Amsterdam: Elsevier B.V.
Kanaan, R. A. A., Craig, T. K. J., Wessely, S. C., and David, A. S. (2007). Imaging repressed memories in motor conversion disorder. Psychosom. Med. 69, 202–205. doi: 10.1097/PSY.0b013e31802e4297
Koch, G., Bonnì, S., Casula, E. P., Iosa, M., Paolucci, S., Pellicciari, M. C., et al. (2019). Effect of cerebellar stimulation on gait and balance recovery in patients with hemiparetic stroke: a randomized clinical trial. JAMA Neurol. 76, 170–178. doi: 10.1001/jamaneurol.2018.3639
Kujirai, T., Caramia, M. D., Rothwell, J. C., Day, B. L., Thompson, P. D., Ferbert, A., et al. (1993). Corticocortical inhibition in human motor cortex. J. Physiol. 471, 501–519. doi: 10.1113/jphysiol.1993.sp019912
Liepert, J., Hassa, T., Tüscher, O., and Schmidt, R. (2008). Electrophysiological correlates of motor conversion disorder. Mov. Disord. 23, 2171–2176. doi: 10.1002/mds.21994
Liepert, J., Hassa, T., Tüscher, O., and Schmidt, R. (2011). Motor excitability during movement imagination and movement observation in psychogenic lower limb paresis. J. Psychosom. Res. 70, 59–65. doi: 10.1016/j.jpsychores.2010.06.004
Marconi, B., Pecchioli, C., Koch, G., and Caltagirone, C. (2007). Functional overlap between hand and forearm motor cortical representations during motor cognitive tasks. Clin. Neurophysiol. 118, 1767–1775. doi: 10.1016/j.clinph.2007.04.028
McDonnell, M. N., and Stinear, C. M. (2017). TMS measures of motor cortex function after stroke: a meta-analysis. Brain Stimul. 10, 721–734. doi: 10.1016/j.brs.2017.03.008
Motta, C., Di Lorenzo, F., Ponzo, V., Pellicciari, M. C., Bonnì, S., Picazio, S., et al. (2018). Transcranial magnetic stimulation predicts cognitive decline in patients with Alzheimer’s disease. J. Neurol. Neurosurg. Psychiatry 89, 1237–1242. doi: 10.1136/jnnp-2017-317879
Nowak, D. A., and Fink, G. R. (2009). Psychogenic movement disorders: aetiology, phenomenology, neuroanatomical correlates and therapeutic approaches. Neuroimage 47, 1015–1025. doi: 10.1016/J.NEUROIMAGE.2009.04.082
Pezzella, F. R., Picconi, O., De Luca, A., Lyden, P. D., and Fiorelli, M. (2009). Development of the Italian version of the national institutes of health stroke scale it-NIHSS. Stroke 40, 2557–2559. doi: 10.1161/STROKEAHA.108.534495
Pollak, T. A., Nicholson, T. R., Edwards, M. J., and David, A. S. A. (2014). systematic review of transcranial magnetic stimulation in the treatment of functional (conversion) neurological symptoms. J. Neurol. Neurosurg. Psychiatry 85, 191–197. doi: 10.1136/jnnp-2012-304181
Powers, W. J., Rabinstein, A. A., Ackerson, T., Adeoye, O. M., Bambakidis, N. C., Becker, K., et al. (2019). Guidelines for the early management of patients with acute ischemic stroke: 2019 update to the 2018 guidelines for the early management of acute ischemic stroke: a guideline for healthcare professionals from the american heart association/american stroke. Stroke 50, e344–e418. doi: 10.1161/STR.0000000000000211
Premi, E., Benussi, A., Compostella, S., Gilberti, N., Vergani, V., Delrio, I., et al. (2017). Multimodal brain analysis of functional neurological disorders: a functional stroke mimic case series. Psychother. Psychosom. 86, 317–319. doi: 10.1159/000465524
Pringsheim, T., and Edwards, M. (2017). Functional movement disorders: five new things. Neurol. Clin. Pract. 7, 141–147. doi: 10.1212/CPJ.0000000000000350
Quartarone, A., Rizzo, V., Terranova, C., Morgante, F., Schneider, S., Ibrahim, N., et al. (2009). Abnormal sensorimotor plasticity in organic but not in psychogenic dystonia. Brain 132, 2871–2877. doi: 10.1093/brain/awp213
Ridding, M. C., and Rothwell, J. C. (1997). Stimulus/response curves as a method of measuring motor cortical excitability in man. Electroencephalogr. Clin. Neurophysiol. Electromyogr. Mot. Control 105, 340–344. doi: 10.1016/S0924-980X(97)00041-6
Roelofs, J. J., Teodoro, T., and Edwards, M. J. (2019). Neuroimaging in functional movement disorders. Curr. Neurol. Neurosci. Rep. 19, 1–7. doi: 10.1007/s11910-019-0926-y
Rossini, P. M., Burke, D., Chen, R., Cohen, L. G., Daskalakis, Z., Di Iorio, R., et al. (2015). Non-invasive electrical and magnetic stimulation of the brain, spinal cord, roots and peripheral nerves: basic principles and procedures for routine clinical and research application. An updated report from an I.F.C.N. Committee. Clin. Neurophysiol. 126, 1071–1107. doi: 10.1016/j.clinph.2015.02.001
Schönfeldt-Lecuona, C., Lefaucheur, J., Lepping, P., Liepert, J., Connemann, B. J., Sartorius, A., et al. (2016). Non-invasive brain stimulation in conversion (Functional) weakness and paralysis: a systematic review and future perspectives. Front. Neurosci. 10:140. doi: 10.3389/fnins.2016.00140
Stone, J., Carson, A., Duncan, R., Roberts, R., Warlow, C., Hibberd, C., et al. (2010). Who is referred to neurology clinics?—The diagnoses made in 3781 new patients. Clin. Neurol. Neurosurg. 112, 747–751. doi: 10.1016/J.CLINEURO.2010.05.011
Stone, J., Zeman, A., Simonotto, E., Meyer, M., Azuma, R., Flett, S., et al. (2007). fMRI in patients with motor conversion symptoms and controls with simulated weakness. Psychosom. Med. 69, 961–969. doi: 10.1097/PSY.0b013e31815b6c14
Tellegen, A., and Ben-Porath, Y. S. (1993). Code-type comparability of the MMPI and MMPI-2: analysis of recent findings and criticisms. J. Per.s Assess. 61, 489–500. doi: 10.1207/s15327752jpa6103_5
van der Hoeven, R. M., Broersma, M., Pijnenborg, G. H. M., Koops, E. A., van Laar, T., Stone, J., et al. (2015). Functional (psychogenic) movement disorders associated with normal scores in psychological questionnaires: a case control study. J. Psychosom. Res. 79, 190–194. doi: 10.1016/J.JPSYCHORES.2015.06.002
Ziemann, U., Rothwell, J. C., and Ridding, M. C. (1996). Interaction between intracortical inhibition and facilitation in human motor cortex. J. Physiol. 496, 873–881. doi: 10.1113/jphysiol.1996.sp021734
Keywords: functional neurological disorders, functional paralysis, transcranial magnetic stimulation, short interval intracortical inhibition, motor threshold
Citation: Benussi A, Premi E, Cantoni V, Compostella S, Magni E, Gilberti N, Vergani V, Delrio I, Gamba M, Spezi R, Costa A, Tinazzi M, Padovani A, Borroni B and Magoni M (2020) Cortical Inhibitory Imbalance in Functional Paralysis. Front. Hum. Neurosci. 14:153. doi: 10.3389/fnhum.2020.00153
Received: 18 February 2020; Accepted: 06 April 2020;
Published: 07 May 2020.
Edited by:
Giovanni Di Pino, Campus Bio-Medico University, ItalyReviewed by:
Michele Dileone, Hospital Virgen del Puerto, SpainViviana Versace, Research Unit for Neurorehabilitation of South Tyrol, Italy
Mariagiovanna Cantone, Sant’Elia Hospital, Italy
Copyright © 2020 Benussi, Premi, Cantoni, Compostella, Magni, Gilberti, Vergani, Delrio, Gamba, Spezi, Costa, Tinazzi, Padovani, Borroni and Magoni. This is an open-access article distributed under the terms of the Creative Commons Attribution License (CC BY). The use, distribution or reproduction in other forums is permitted, provided the original author(s) and the copyright owner(s) are credited and that the original publication in this journal is cited, in accordance with accepted academic practice. No use, distribution or reproduction is permitted which does not comply with these terms.
*Correspondence: Enrico Premi, zedtower@gmail.com
†These authors have contributed equally to this work