- 1I-EaT Swallowing Research Laboratory, Speech Language and Hearing Sciences, Purdue University, West Lafayette, IN, United States
- 2Libraries and School of Information Studies, Purdue University, West Lafayette, IN, United States
- 3Psychological and Brain Sciences, Imaging Research Facility, Indiana University, Bloomington, IN, United States
- 4Weldon School of Biomedical Engineering, Purdue University, West Lafayette, IN, United States
Background: Swallowing disorders (dysphagia) can negatively impact quality of life and health. For clinicians and researchers seeking to improve outcomes for patients with dysphagia, understanding the neural control of swallowing is critical. The role of gray matter in swallowing control has been extensively documented, but knowledge is limited regarding the contributions of white matter. Our aim was to identify, evaluate, and summarize the populations, methods, and results of published articles describing the role of white matter in neural control of swallowing.
Methods: We completed a systematic review with a multi-engine search following PRISMA-P 2015 standards. Two authors screened articles and completed blind full-text review and quality assessments using an adapted U.S. National Institute of Health's Quality Assessment. The senior author resolved any disagreements. Qualitative synthesis of evidence was completed.
Results: The search yielded 105 non-duplicate articles, twenty-two of which met inclusion criteria. Twenty were rated as Good (5/22; 23%) or Fair (15/22; 68%) quality. Stroke was the most represented diagnosis (n = 20; 91%). All studies were observational, and half were retrospective cohort design. The majority of studies (13/22; 59%) quantified white matter damage with lesion-based methods, whereas 7/22 (32%) described intrinsic characteristics of white matter using methods like fractional anisotropy. Fifteen studies (68%) used instrumental methods for swallowing evaluations. White matter areas commonly implicated in swallowing control included the pyramidal tract, internal capsule, corona radiata, superior longitudinal fasciculus, external capsule, and corpus callosum. Additional noteworthy themes included: severity of white matter damage is related to dysphagia severity; bilateral white matter lesions appear particularly disruptive to swallowing; and white matter adaptation can facilitate dysphagia recovery. Gaps in the literature included limited sample size and populations, lack of in-depth evaluations, and issues with research design.
Conclusion: Although traditionally understudied, there is sufficient evidence to conclude that white matter is critical in the neural control of swallowing. The reviewed studies indicated that white matter damage can be directly tied to swallowing deficits, and several white matter structures were implicated across studies. Further well-designed interdisciplinary research is needed to understand white matter's role in neural control of normal swallowing and in dysphagia recovery and rehabilitation.
Introduction
Swallowing is an essential biological function governed by both peripheral and central sensorimotor pathways. Damage in these pathways can cause swallowing disorders, also known as dysphagia. Dysphagia is a frequent consequence of many neurological and anatomical conditions or diseases (e.g., stroke, cerebral palsy, Parkinson's disease, dementia, head and neck cancer, trauma, etc.), and is very common. In the US alone, four percent of adults are reported to experience dysphagia per year (Bhattacharyya, 2014). For those individuals, the impact can be profound. Dysphagia affects quality of life (Leow et al., 2010), nutrition (Namasivayam and Steele, 2015), hydration (Reber et al., 2019), respiratory function, and overall health (Langmore et al., 1998). Because of its impact and relatively high prevalence, developing effective interventions for the management of dysphagia has been a longstanding goal of clinicians and researchers. Central in these efforts has been the attempt to increase our knowledge and understanding of the underlying physiological and neurophysiological mechanisms that govern swallowing, and which can be targeted in treatment.
This knowledge base has been growing over the past 100 years, with much of the literature focused on the role of cortical and brainstem gray matter areas involved in the neural control of swallowing. In the early 1900's, neuroscience research relied heavily on animal models and focused on the reflexive nature of swallowing (Miller and Sherrington, 1915). This animal work revealed the essential role that brainstem nuclei, specifically a group of medullary nuclei, play in triggering the pharyngeal response (Doty, 1951, 1968; Car and Roman, 1969; Jean et al., 1975; Amri et al., 1984; Kessler and Jean, 1985). In the mid 1900's, some attention was directed to the cortex (Car, 1970; Sumi, 1972), as researchers found that swallowing or mastication were evoked when specific cortical regions (i.e., the lateral pericentral and superior sylvian cortex) were stimulated with electrical pulses in patients under seizure evaluation (Penfield and Welch, 1949; Penfield, 1955). This same response was also seen in animals during intracranial microelectrode stimulation (Sumi, 1972; Martin et al., 1999). Despite these findings, the theory that swallowing is primarily reflexive (i.e., brainstem mediated) predominated from the early 1900's even into the 1980's (Bosma, 1957; de Lama Lazzara et al., 1986). During this time period, this notion started being challenged with the advent of new imaging techniques that allowed researchers to non-invasively look at changes in brain structures in living humans.
Clinical studies reporting swallowing deficits in patients with cortical and subcortical lesions provided the first clear support that the role of the cerebrum was essential in swallowing (Meadows, 1973; Gordon et al., 1987; Martin and Sessle, 1993; Robbins et al., 1993; Daniels et al., 1996). This was then further delineated through novel neuroimaging techniques that enabled the study of metabolic correlates of brain activation during swallowing in vivo (e.g., Hamdy et al., 1999; Martin et al., 2001, 2004; Suzuki et al., 2003; Toogood et al., 2005; Malandraki et al., 2009, 2011). This growing body of literature was critical in shifting our appreciation of swallowing from a simple brainstem mediated reflex to a highly complex sensorimotor function relying on all levels of the central nervous system (CNS) (Malandraki et al., 2011).
Undoubtedly, identifying the gray matter regions that play a role in swallowing was a significant contribution. However, the specifics on how these regions communicate and connect with each other to achieve this complex control remains largely unexplored, i.e., there is little insight on the role of white matter tracts. One early computed tomography (CT) study reported that damage to subcortical white matter (the internal capsule and within the brainstem) caused dysphagia, likely due to disruption in the sensorimotor pathways of the corticobulbar tract (Logemann et al., 1993). Additional early CT/MRI work showed that lingual discoordination and dysphagia were common in patients with periventricular white matter lesions (Daniels et al., 1999).
Despite the relatively limited focus on the role of white matter for swallowing, it is evident from broader neurophysiology work that white matter is highly relevant to the study of all human functions. White matter is the CNS component composed primarily of myelinated axons of neurons, provides the connections between cells, and functions as the information highway between distinct brain regions. These connections bundle together to form three primary types of white matter tracts. First, association tracts connect areas of the cortex within the same hemisphere (Schmahmann et al., 2007). One prominent association tract is the superior longitudinal fasciculus which contains many branches, most notably the arcuate fasciculus, which connects the Broca's and Wernicke's areas in the left hemisphere (Breier et al., 2008). Damage to these structures in each hemisphere can lead to different symptoms, due to the lateralization of functions. Secondly, commissural tracts are the tracts that connect the right and left hemispheres, and include the corpus callosum, and the anterior and posterior commissures. Damage to these inter-hemispheric structures can cause frontal lobe dysfunction and spatial deficits (Buklina, 2005). Lastly, projection tracts connect areas in the cortex with lower centers such as deep nuclei or the brainstem. An example of projection tracts/structures are the internal capsules, which carry motor and sensory information between the cortex and sub-cortical areas. Damage to projection fibers can result in motor or sensory deficits throughout the body (Puig et al., 2011; Emos and Agarwal, 2020).
Scientific and clinical interest in these white matter tracts has been increasing. In a PubMed search using the key word “white matter,” we identified a 400% increase in relevant articles since 1999. This increase is most likely due to the emergence of a new field devoted to understanding the full network of these tracts in humans, known as the human connectome (Sporns et al., 2005). This field has evolved through imaging advancements in diffusion weighted imaging (DWI), and in analysis techniques, such as tractography (Huisman, 2010). Diffusion weighted imaging senses the diffusion of water across tissue materials. Notably, white matter tracts have a unique diffusion property; they are anisotropic, i.e., water diffuses predominantly along the fiber (Frank, 2001). This characteristic (captured with DWI) allows us to identify and describe white matter structures and their properties with simple metrics such as fractional anisotropy (Alexander et al., 2007).
Although our understanding of the role of white matter for many biological functions is increasing, our understanding of these pathways in the neural control of swallowing remains scarce. Further, it is unclear to what extent newer imaging techniques such as DWI/DTI have been used for the study of the swallowing control. Identifying and addressing these gaps will provide critical insight on the structural neural connections involved in swallowing and has the potential to improve our ability to accurately identify and treat patients with neurological disease and dysphagia. Further, white matter is highly adaptable as shown by studies on recovery of sensorimotor functions after neurotrauma (Schlaug et al., 2009; Kou and Iraji, 2014; Sampaio-Baptista and Johansen-Berg, 2017), and may hold potential for maximizing swallowing recovery, but it is unclear to what extent this has been investigated. As a first step to informing future research in this line of work, it is necessary to systematically evaluate the quality of existing evidence and compare results across studies. Therefore, this systematic review aimed to identify all published research articles describing the role of white matter in the neural control of swallowing, and summarize and evaluate them to determine answers to four primary research questions:
(1) What patient populations are represented in the available evidence?
(2) What white matter imaging techniques and swallowing evaluation techniques have been utilized to investigate the role of white matter in the neural control of swallowing?
(3) Does the available evidence provide definitive information on specific white matter tracts that are implicated in the neural control of swallowing and their role?
(4) What are the main gaps in the investigation of the role of white matter tracts in the neural control of swallowing that need to be addressed in future research?
Methods
Systematic Review Protocol
This review was conducted systematically and the detailed protocol was developed a priori in accordance with the PRISMA-P 2015 (Preferred Reporting Items for Systematic Reviews and Meta-Analyses) guidelines (Moher et al., 2009). Further, it was registered with the international prospective register of systematic reviews (PROSPERO ID: CRD 42020191453). Throughout the process, Rayyan data management software was utilized for blinding and tracking (Ouzzani et al., 2016).
Literature Search Strategy
A health sciences librarian (third author, BM) performed literature searches from May 2020 through July 2020, in the following databases: MEDLINE (via PubMed), Cochrane Library Database of Systematic Reviews, CINAHL, and Web of Science. Searches included a combination of controlled vocabulary terms, when applicable, and free text keywords. No filters were used during the search process. The literature was searched using combinations of terms including the following: “magnetic resonance imaging,” OR “MRI,” OR “white matter,” AND “deglutition disorders,” OR “dysphagia.” Terms were nominated by the senior/last author (GAM) and were further discussed and agreed upon with the entire team, including an MRI physicist and experienced imager (fourth author; H.C.). Both initial and final searches included a back-chained search of the reference lists of all identified articles. The final search was executed in November 2020, just before manuscript submission, to capture new publications. The precise search strategies, databases searched, and the number of results retrieved per database, are available in Supplementary Table A.
Inclusion and Exclusion Criteria
Studies were included in this review if they met the following criteria: (1) included human subjects of all ages, (2) were peer-reviewed research articles, scientific abstracts, case studies, or reviews, (3) were written in English language, (4) reported aberration of white matter microstructure/pathways and/or documentation of damage to white matter resulting in dysphagia, (5) used one or more of the following methods: MRI, diffusion MRI (dMRI), diffusion-weighted imaging (DWI), diffusion tensor imaging (DTI), tractography, and/or structural brain network (connectome), and (6) included swallowing/dysphagia as a primary outcome.
Consequently, studies were excluded if they: (1) included only animal models, (2) were not in English, (3) did not specifically document white matter aberration, or (4) did not include swallowing as a primary outcome measure.
The two first authors (AA and RHA) independently screened all titles and abstracts identified with the search strategy for inclusion/exclusion, and any disagreements were resolved by the senior/last author (GAM). Then, the same two authors (AA and RHA) reviewed the full text of all qualifying articles to make the final decision of eligibility, with disagreements, again, resolved by the senior/last author (GAM).
Bias Assessment
In order to compare results across studies, it is essential to assess study quality and risk of bias. Because the majority of the studies identified in this review were observational cohort studies, study quality was assessed using an adapted version of the U.S. Department of Health and Human Services National Institute of Health's (NIH) Quality Assessment protocol for observational cohort and cross-sectional studies (National Heart, Lung, and Blood Institute, 2019). This 12–item tool was developed to assist reviewers in critically appraising the internal validity of studies through a structured evaluation of sources of bias, diagnosis and outcome measures, and statistical components. For the purpose of this study, three of 12 original assessment items in the protocol were not considered in the quality assessment, because they were consistently not reported or not applicable in the studies reviewed (these were: sufficient timeframe to see exposure effect, repeated exposure assessment, and follow-up rate). See Table 1 for a full list of items assessed, and Supplementary Table B for details on the final adapted quality assessment tool.
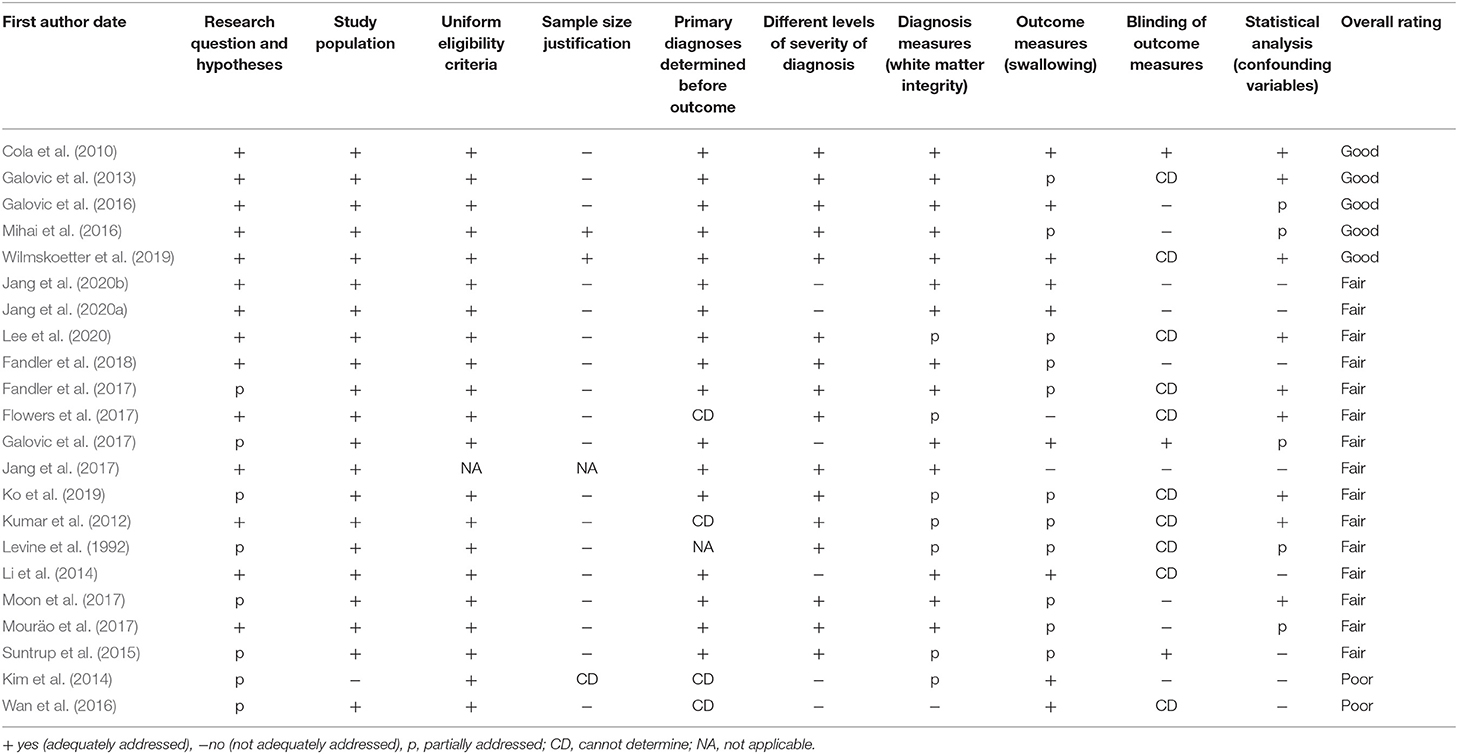
Table 1. Study quality parameters rated using the modified NIH quality assessment for observational cohort and cross-sectional studies.
Each study was independently evaluated by the two first authors (AA and RHA) using the NIH Quality Assessment protocol and was given a cumulative rating of Good, Fair, or Poor, that summarized the risk of bias in the study. According to the NIH Quality Assessment guidelines for the cumulative ratings, research studies are rated as “Good” if they have the least risk of bias, although they may not be free from all potential biases (Study Quality Assessment Tools | NHLBI, NIH, 2019). Bias in papers rated as “Good” is minimal and is discussed and accounted for in analysis. A study rated as “Fair” is still considered valid, with useful information, but may have some clear risk of bias that is not addressed. For example, a fair study may be underpowered due to limited sample size and not including blinding, both of which increase risk of bias. Finally, studies rated as “Poor” have substantial methodological limitations across multiple categories that limit interpretation of results. This tool does not determine precise cut-offs between these quality categories, but instead helps the evaluators rate the overall risk of internal bias based on key items/questions (see Supplementary Table B). To maximize objectivity, we used two independent raters (first two authors, AA and RHA), who participated in a 3-h practice training on this tool led by the senior author (GAM), before starting its use. Any disagreements in quality ratings were planned to be resolved through discussion with the senior/last author (GAM), though no disagreements occurred.
Data Extraction and Qualitative Synthesis
After completion of the bias assessment, the two first authors (AA and RHA) independently extracted data from all articles. Information extracted from each paper included study type and population characteristics, white matter techniques and information, swallowing evaluation methods, outcome measurements, and main findings from each study.
Extracted data on study type and population characteristics included: study design [classified in accordance with (Mann, 2003)], number of patients, number of participants in control group (if applicable), underlying diagnosis/disease of patient group or subgroups, severity/state of disease, age, sex, race, and ethnicity. Data extracted on white matter measurement included: scan type, scanner model and strength, head coil type, b-values, and number of directions (for DWI scans), scan settings, analysis type, and analysis method.
We also extracted details on how the primary outcome variable (swallowing) was assessed in order to compare clinical findings across studies. Data extracted included swallowing measurement method (e.g., instrumental or clinical assessment) and analysis of swallowing components (e.g., use of Penetration Aspiration Scale (Rosenbek et al., 1996), temporal measures, binary clinical ratings, etc.). Finally, information was extracted on the studies' main findings, which included implicated white matter areas and their suggested role in swallowing (including the statistical descriptions of that relationship), and limitations of each study.
Due to the wide variety of study types, patient populations, and imaging and swallowing evaluation methods, it was not possible to analyze the data across studies quantitatively at this time. Instead, a qualitative synthesis of the findings across studies was completed while critically evaluating the risk of bias of their methods and results.
Results
Study Selection and Data Extraction
The initial search of four databases in May-July 2020 retrieved a total of 182 titles, including 78 duplicates and 104 unique titles. Two articles were identified via backward citation chaining, and two additional articles (Jang et al., 2020b; Lee et al., 2020) were identified in a search verification in September 2020. A final search was conducted on November 8th, before submission, which revealed three novel articles, none of which met inclusion criteria. In total, this resulted in a subset of 108 non-duplicate articles, which all had full abstracts. Two independent reviewers (first two authors; AA and RHA) reviewed the titles and abstracts, and after this review, 27 articles met our inclusion criteria for full text review. Following a detailed full text review, 22 articles were selected for inclusion (see Figure 1 for CONSORT diagram and exclusion reasons). There was disagreement on three articles at the screening stage, which was resolved by the senior/last author (GAM), and there were no other author disagreements on article review and inclusion. After study selection, the two first authors (AA and RHA) extracted data independently with agreement on 91% of extracted items and reached consensus on the remaining 9% of items extracted.
Study Types and Characteristics
Of the 22 articles included in the review, one was a case study (Jang et al., 2017), and 21 included multiple participants and were observational, i.e., there was no intervention assessed (Table 2). The most frequent observational study design employed was retrospective cohort (n = 11), followed by three case control studies, and one prospective cohort study. Six studies did not neatly fit into one of the main observational study categories (Mann, 2003). Four of these were closest to a prospective cohort design (Li et al., 2014; Suntrup et al., 2015; Galovic et al., 2016, 2017). However, instead of examining whether an outcome of interest (i.e., dysphagia) would develop over time, these four studies included only participants who had already failed a dysphagia screening or were diagnosed with dysphagia. One study identified a convenience sample with a common diagnosis (cerebral palsy, CP) and used observational methods to determine dysphagia status (Mouräo et al., 2017), and one study recruited healthy individuals to look at both a risk factor (i.e., aberrations in white matter) and changes in swallowing (Levine et al., 1992). Because these six studies did not fit into a specific pre-defined study type, but all involved carefully defined groups of subjects, we identified them broadly as “cohort design.”
Participant Characteristics and Clinical Classifications
Demographic and clinical diagnosis data (Research Question 1) of all reviewed studies are also included in Table 2. Ages of participants ranged from five to 96 years old, and only one study included patients under 18, children with CP, ages 5.11-17.6 (Mouräo et al., 2017). One study did not report age of the participants (Kim et al., 2014). Only two studies reported race (Cola et al., 2010; Wilmskoetter et al., 2019), and one also reported ethnicity (Wilmskoetter et al., 2019). The number of participants across studies ranged from a single subject to 322 (mean = 92.77), and 40.88% of all subjects were female, though one study did not report sex (Kim et al., 2014). One article included exclusively healthy participants, and 21 articles included patients. Of these 21 articles, seven also included groups of healthy adults, four as control groups and three for white matter mapping and modeling, not for comparison.
The majority of subjects were adult patients post stroke (20 articles) and, as already mentioned, one study focused on children with CP (Mouräo et al., 2017). Thirteen studies included a clinical rating of the underlying diagnosis of their subjects (Table 2). For stroke, the most frequent clinical rating scale used was the National Institute for Health Stroke Scale (NIHSS; n = 10). Three studies used the Rankin Scale (n = 3), one used the Canadian Neurological Scale (n = 1), one used the Korean mini mental (n = 1), and one used the motility index of limbs (n = 1) (Table 2). The clinical ratings used for CP were the Gross Motor Function Classification System (GMFCS) and the Manual Ability Classification System (MACS) (Mouräo et al., 2017).
Notably, within the studies that focused on stroke (20/22), inclusion criteria varied substantially. One study listed broad criteria of stroke diagnosis without further specification, eleven required that it was the first stroke, six required damage to a specific region, such as the middle cerebral artery, and nine required varying degrees of dysphagia severity for inclusion (Table 2).
Imaging Parameters and Analysis
Data Acquisition
Imaging specifications (Research Question 2) used in all reviewed studies are detailed in Table 3. All studies used MR imaging, however, specifics on scanner models and strength, scan types, settings, and analysis methods varied.
Scanner strength is reported in tesla (T), and scanners with higher tesla values allow for a stronger MR signal and may increase the speed of scan acquisition. Fourteen of the 22 reviewed studies provided information on scanner strength (Table 3). Of those 14, three reported using exclusively 3T MRI scanners, two of which were Siemens models, and one GE. Eight reported using exclusively 1.5T, including models from GE (2), Siemens (1), and Philips (4). Three studies utilized scans from both 1.5 and 3T Siemens scanners. Finally, eight studies did not report scanner strength, brand, or model. Only five studies reported information on head coils utilized. Two reported using a 6 channel coil, two reported using a 32 channel coil, and one reported using a quadrature head coil.
In addition to scanner strength and types, a wide variety of scan sequences and parameters were also reported across studies (Table 3), with three studies not reporting scan types at all. Of the studies that did report scan types (19/22), ten acquired a T2-weighted scan (most commonly a T2 FLAIR sequence), and seven a T1-weighted scan. Eighteen studies reported acquiring a DWI/DTI sequence, i.e., the state-of-the-art non-invasive technique to explore white matter integrity (Chanraud et al., 2010). However, it is noteworthy that DWI/DTI scans were not necessarily collected for the studies' white matter analysis, but as standard-of-care protocols for stroke patients (Leiva-Salinas and Wintermark, 2010).
Of the 18 papers that acquired a DWI/DTI sequence, eleven reported their b values (i.e., the diffusion-sensitive gradient factor that helps create different types of contrast between tissues). The most frequently reported b-value was 1,000 s/mm2 which has been reported to be the optimal b-value for assessing stroke in the acute phase (Kingsley and Monahan, 2004). Additionally, one pediatric study (CP) reported a b-value of 800 s/mm2 (Mouräo et al., 2017).
Another important parameter to consider when acquiring DWI scans is the number of directions in which diffusion is measured (i.e., the greater the number of directions, the greater the number of details that can be mapped in tractography) (Vos et al., 2016). Of the eighteen studies that reported acquiring DWI scans, only four reported the number of directions. Two reported 64 directions, one reported 15, and one three directions (see Table 3).
Data Analysis
As expected, given the variety of scan models and specifications utilized, there was also considerable variability in the methods used for white matter data analysis. However, some common themes emerged. The major observation was that researchers followed one of two paths for analysis. Either they examined the damage/lesions to white matter by investigating the size and characteristics of these lesions; or they evaluated white matter integrity by measuring and describing characteristics of the tissue. Most studies followed the first approach and used qualitative rating scales or quantitative measurements to measure lesions. Studies using this (lesion-based) approach are discussed first followed by studies using the second option (white-matter integrity).
Lesion-based analysis: qualitative methods
Eight studies utilized qualitative scales to describe damage to white matter. The most commonly cited scale (n = 6) (Fandler et al., 2017; Flowers et al., 2017; Moon et al., 2017; Ko et al., 2019; Jang et al., 2020b; Lee et al., 2020) was the 4-point Fazekas scale (Fazekas et al., 1987). To determine ratings using the Fazekas scale, clinicians or researchers visually examine the amount and size of white matter hyperintensities [i.e., brighter spots on T2-weighted scans that relate to damage to small blood vessels or decrease in myelination (Wardlaw et al., 2015)] in two domains: the periventricular white matter and the deep white matter, and provide a rating of these hyperintensities. An earlier study (Levine et al., 1992) used another 4-point/grade scale developed by Awad et al. using size, multiplicity, and location to rate subcortical incidental lesions (Awad et al., 1986). Further, one study employed a rather indirect approach (Kim et al., 2014), using an adapted scale which categorizes damage to the brain's vascular territories (Rovira et al., 2005), to indirectly infer lesions to white matter.
Lesion-based analysis: quantitative methods
Ten of the 22 reviewed studies used a quantitative volume and voxel-based approach to record the location and size of lesions. These studies quantified damage by measuring lesion volume with voxels or mm2 lesioned (Galovic et al., 2013, 2016, 2017; Suntrup et al., 2015; Mihai et al., 2016; Flowers et al., 2017; Fandler et al., 2018; Ko et al., 2019; Wilmskoetter et al., 2019; Jang et al., 2020a) or they used these units to derive the amount of area or proportion (%) of an area that is lesioned (Suntrup et al., 2015). Three studies used a technique called voxel-based lesion symptom mapping (VLSM) (Bates et al., 2003), which enables calculation of correlations between locations of the “damaged” voxels and behavioral scores (Galovic et al., 2016, 2017; Wilmskoetter et al., 2019).
White matter integrity analysis
Seven of the 22 studies included in this review utilized analysis techniques that provided information on the structural integrity of the white matter, instead of focusing only on lesions. The main quantitative measure reported was fractional anisotropy (FA; n = 5) (Table 3). Higher FA values are associated with greater white matter integrity, due to more coherent diffusion of water across tissue (De Erausquin and Alba-Ferrara, 2013). Six studies generally reported using tractography, an analysis method that creates a 3D map of the white matter tracts in the brain, and two of the seven studies measured tract volumes (TV). Of those six, only one reported additional diffusion-based analysis measures, including radial diffusivity, mean diffusivity, and fibers count (Mouräo et al., 2017). These measures give more in-depth information on the integrity of white matter, such as strength of connection and anisotropy of the diffusion (Mori and Zhang, 2006; Clark et al., 2011; de Figueiredo et al., 2011). One study used a different quantitative approach that indirectly informs us about connections in the brain, known as functional connectivity (Li et al., 2014), using the synchrony of the blood oxygen level dependent signals between areas of gray matter to give us indirect insight about how these areas are connected.
Swallowing Evaluation Methods and Analysis
Swallowing Evaluation Methods
All, but one study (Jang et al., 2017), reported details on how swallowing was evaluated (Research Question 2; Table 4). It is commonly accepted that the gold standard for comprehensively evaluating swallowing involves radiologic or endoscopic imaging, i.e., Videofluoroscopic Swallow Studies (VFSS) or Fiberoptic Endoscopic Evaluations of Swallowing (FEES). These evaluation methods allow differing degrees of visualization of the oropharyngeal area, upper airway and upper esophagus, and allow clinicians to make subjective judgments or objective measurements of symptoms, and/or kinematic, temporal and bolus flow events. Fifteen of the 21 studies that reported swallowing evaluation methods used some imaging modality (VFSS and/or FEES; Table 4). Specifically, nine reported use of VFSS for all patient participants, and four reported using VFSS for a subset of subjects (e.g., they included some patients who had received a VFSS while hospitalized post-stroke and others who had only received clinical bedside swallowing evaluations). FEES was used in three studies: one used only FEES (Suntrup et al., 2015), one used FEES in addition to VFSS (Wan et al., 2016), and a third study used FEES for a subset of subjects (Galovic et al., 2017).
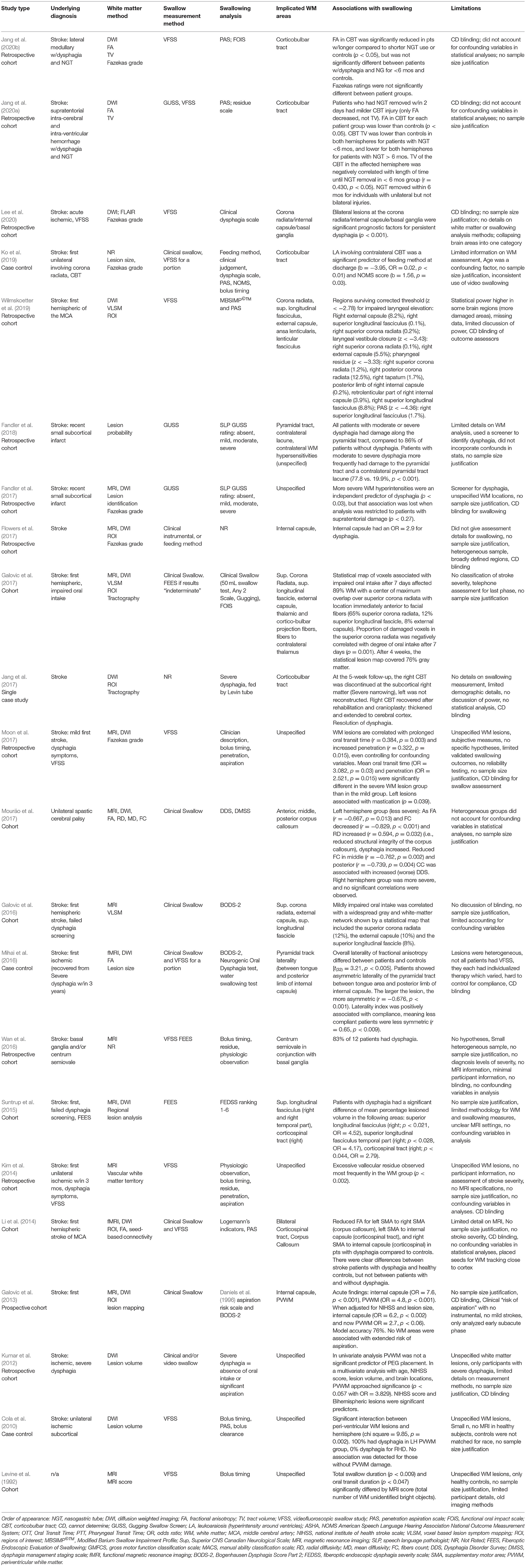
Table 4. Summary of methods, major findings and limitations of studies included in qualitative synthesis (n = 20 studies).
Eight studies used a clinical (bedside) swallowing evaluation (CSE), which typically includes a case history, a detailed cranial nerve assessment, oropharyngeal mechanism exam, and oral trials of foods and liquids. One study used a CSE in addition to VFSS (Li et al., 2014), four studies used a CSE for all patients, while reporting that a portion of subjects also received instrumental assessments (Kumar et al., 2012; Mihai et al., 2016; Galovic et al., 2017; Ko et al., 2019), and three studies used only a CSE (Galovic et al., 2013, 2016; Mouräo et al., 2017). Lastly, in two studies the researchers performed only a swallow screening, i.e., a brief evaluation determining the risk for a diagnosis of dysphagia (Fandler et al., 2017, 2018).
Analysis of Swallowing Parameters
Table 4 also summarizes each study's swallowing analysis methods (see Supplementary Table C for more extensive detail). Of the studies that included VFSS for all subjects (n = 9), six used the Penetration Aspiration Scale (PAS) which rates the level of airway invasion and patients' response to penetration or aspiration events (Rosenbek et al., 1996), five studies employed temporal/timing measures, and Wilmskoetter et al. (2019) used the Modified Barium Swallow Impairment Profile (MBSImP©™; Martin-Harris et al., 2008), a standardized protocol that enables clinicians to quantify physiological swallowing impairments. Of the studies that used FEES, one study used a tool to rate dysphagia severity from FEES, the fiberoptic endoscopic dysphagia severity scale (FEDSS).
Five of the eight studies that included CSEs used standardized tools to interpret the assessment. Mouräo et al. (2017) used the Dysphagia Disorder Survey, a validated clinical assessment of swallowing and feeding function for individuals with intellectual and developmental disability (Sheppard et al., 2014); three studies used the Bogenhausen Dysphagia Score, Part 2 (BODS-2) (Bartolome, 2006), which is a German assessment of oral intake; and two used the Functional Oral Intake Scale (FOIS) (Crary et al., 2005), i.e., a description of levels of oral intake, retrospectively. Three studies reported mixed methods for swallowing analysis, such as a variety of different clinical scales (see details on all methods in Supplementary Table C).
White Matter Areas Implicated in the Neural Control of Swallowing
Quality of Evidence and Bias Assessment
In order to determine if there is definitive evidence implicating specific white matter areas in the control of swallowing (Research Question 3), we first critically assessed the quality of the available evidence using the modified NIH quality assessment (results in last column of Table 1). Five articles were rated as “Good,” fifteen articles were classified as “Fair,” and two articles were classified as “Poor.”
The most common risk of bias (found in 20 of the 21 studies with greater than one participant) involved failing to justify sample size (i.e., not reporting power analysis or variance/effect estimates) (Table 1). Sample size was frequently limited by the clinical setting and/or by the retrospective design. The second most frequent item impacting quality assessment was blinding. Twenty of the 22 studies did not describe blinding of assessors (e.g., whether swallowing assessors were blinded to MRI results/diagnoses). Other elements negatively affecting quality ratings were related to the measurement methods used to evaluate swallowing and/or white matter. For example, studies frequently included the use of a non-validated and/or non-standardized swallowing assessment tool (15 of 22 studies) or reported limited details on MRI methodology/imaging. Lastly, for 13 studies, ratings were affected by not including confounding variables in statistical analysis. Although handling of missing data was not quantified by the NIH quality assessment tool we used, we noted that few studies described whether they had missing data and how they handled it.
Robust statistical analysis was a common element among all papers that received a quality rating of “Good.” Although only five papers received this highest rating, 20 of 22 papers were of at least “Fair” quality. Therefore, to answer Research Question 3 we qualitatively synthesized the findings of these 20 studies. The two studies rated as “Poor” were not included in this synthesis, due to significant risk of bias, but their information is presented in the tables for the sake of completeness.
Specific White Matter Tracts of Interest and Their Roles
Six of the twenty “Good” or “Fair” quality studies discussed “periventricular white matter” without further location specificity, and fourteen provided information on specific white matter regions implicated in swallowing control (Table 4; see Supplementary Table C for more detailed summary), albeit with differing levels of specificity. The most commonly implicated white matter tracts across studies were the pyramidal tracts (n = 8), followed by more specific tract sections, such as the internal capsule (n = 4), the superior longitudinal fasciculus (n = 3), the corona radiata (n = 3), the corpus callosum (n = 2), the external capsule (n = 2), and the ansa lenticularis/lenticular fasciculus (n = 1).
Pyramidal tracts and subdivisions
Eight studies identified the pyramidal tracts as important in swallowing control. The pyramidal tracts are the projection fibers carrying motor information from the cortex to the brainstem and spinal cord. Although these fibers are frequently subdivided into the corticobulbar and corticospinal tracts (Lohia and McKenzie, 2020a), two studies referred to the pyramidal tract as a whole. Specifically, Fandler et al. (2018) examined 243 patients with dysphagia and found that all patients (100%) with moderate or severe dysphagia had damage along the pyramidal tracts, compared to 86% of patients without dysphagia (Fandler et al., 2018). Further, patients with moderate to severe dysphagia more frequently presented with damage to one pyramidal tract (left or right) and a white matter hyperintensity on the contralateral pyramidal tract than those without dysphagia (77.8 vs. 19.9% respectively, p < 0.001). In a study by Mihai et al. (2016), both clinical swallowing assessments and task-based fMRI and DWI scans were performed in 18 patients who had recovered from clinically determined post-stroke dysphagia and 18 healthy controls. Results of the DWI-based FA analysis revealed that, in comparison with the control group, the patient group had an asymmetric laterality index of the pyramidal tract FA with reduced FA mostly ipsilesionally. This indicated involvement of pyramidal tract lesions in the development of dysphagia, but also some neuroplastic capacity that played a role in recovery.
Two additional studies implicated one division of the pyramidal tract, the corticospinal tract. Li et al. (2014) found that stroke patients with dysphagia had reduced FA in the corticospinal tracts bilaterally when compared to healthy controls, but their FA was not statistically different than stroke patients without dysphagia. This finding may have been influenced by the small sample size (n = 12 in each group) (Li et al., 2014). In a larger study (n = 200), patients with damage to the right corticospinal tract had significantly higher odds of being diagnosed with dysphagia than patients without damage to the same area (OR = 2.79, p < 0.044; Suntrup et al., 2015).
The other division of the pyramidal tract, the corticobulbar tract (CBT), is critical for bulbar functions such as swallowing and speech and was implicated in four papers. Three of these papers directly aimed to investigate the contribution of the CBT in swallowing control. Two studies evaluated the predictive value of CBT damage on prognosis for dysphagia recovery (Jang et al., 2020a,b). Specifically, Jang et al. (2020a) used tractography to measure FA and TV of the CBT in 42 patients with intracerebral hemorrhage and subsequent dysphagia requiring nasogastric tube (NGT) placement. Patients who recovered swallowing within 2 days had relatively minor damage to the CBT (only reduced FA, not reduced TV), whereas patients with longer NGT placement had more extensive damage to the CBT (both reduced FA and TV). In patients with longer NGT placement (2 days to 6 months), CBT volume in the affected hemisphere was negatively correlated (r = −0.430, p < 0.05) with length of time until NGT removal. Finally, none of the patients who had bilateral damage to the CBT were able to have their NGT removed within 6 months (Jang et al., 2020a). In a separate study using similar methodology, Jang et al. (2020b) examined 20 patients with lateral medullary infarctions and found that CBT FA was significantly lower in patients with prolonged NGT placement (<6 months) compared to controls and to patients with shorter NGT placement durations (Jang et al., 2020b, p. 20). Ko et al. (2019) examined the impact of unilateral vs. bilateral damage to the corticobulbar tract (CBT) on swallowing (Ko et al., 2019). They investigated two groups of stroke patients with lesions involving the CBT: one group with unilateral CBT damage and one group with bilateral CBT involvement [defined as damage to the CBT in one hemisphere and additional CBT leukoaraiosis (i.e., white matter hyperintensity) contralaterally]. As expected, bilateral CBT involvement independently predicted worse performance on functional swallowing measures (Ko et al., 2019).
Finally, the involvement of the CBT in swallowing was also reported in a single case study including a stroke patient with damage to the middle cerebral artery and subsequent intracerebral hemorrhage (Jang et al., 2017). At 5 weeks post-stroke, the patient was reported to exhibit severe dysphagia and extensive brain swelling, accompanied by a severely narrowed right CBT, which was not extending to the cortex, and no identifiable left CBT. After decompressive craniotomy at 8 weeks post-stroke, dysphagia symptoms resolved and imaging showed decreased swelling, and a more “normal appearing” right CBT that now extended to the cortex. This indirectly suggests the role of CBT fibers in connecting areas of the swallowing network.
Internal capsule
The internal capsule is a white matter structure which contains both ascending (i.e., thalamocortical) and descending (i.e., pyramidal) fibers, and therefore carries both sensory and motor information. Damage to the internal capsule was associated with dysphagia or aspiration risk in four studies. Flowers et al. (2017) retrospectively reviewed 160 stroke patients to examine neuroanatomical factors that predict the diagnosis of dysphagia, aphasia, and/or dysarthria. They identified seventy-six patients with post stroke dysphagia, and they reported that damage to the internal capsule increased odds of being diagnosed with dysphagia by an average of 3 times (OR = 2.9; 95% CI 1.2–6.6) (Flowers et al., 2017). Galovic et al. (2013) examined lesion location as a predictor of aspiration risk [assessed using the Daniels' et al. clinical evaluation method (Daniels et al., 2000)] in 94 patients within 48 h post stroke and at ~1-week post-stroke. They found that patients with internal capsule lesions had increased odds of aspiration risk in the acute phase (OR = 4.8, p < 0.001), but not at 1-week post-stroke [OR = 1.3, p = 1.0; (Galovic et al., 2013; Flowers et al., 2017)]. Further evidence for the involvement of the internal capsule in swallowing control derives from Mihai et al. DWI-based FA analysis (Mihai et al., 2016). Similar to the results involving the pyramidal tracts, their patient group had an asymmetric laterality index of the posterior limb of the internal capsule FA with reduced FA ipsilesionally, also suggesting involvement of this specific white matter area in the recovery of swallowing function. Lastly, Lee et al. (2020) found that bilateral internal capsule/corona radiata/basal ganglia lesions (all grouped together) were significant prognosticators for persistent dysphagia (p < 0.001) (Lee et al., 2020).
Superior longitudinal fasciculus
The superior longitudinal fasciculus, an association tract, connects multiple brain regions including the frontal, occipital, parietal, and temporal lobes, creating the networks needed for the regulation of motor behavior and conveyance of somatosensory information. Involvement of the superior longitudinal fasciculus was reported in three studies. In two of these, the Galovic group used VLSM to examine lesion locations and connectivity patterns as predictors of impaired oral intake in the acute stroke phase (~2 days post-stroke) (Galovic et al., 2016) and at ~1 and 4 weeks post stroke (Galovic et al., 2017). Their results showed that the statistical map of voxels associated with impaired oral intake involved the superior longitudinal fasciculus to a small extent (8 and 12% of voxels), in the acute and ~1-week phases, respectively (Galovic et al., 2016, 2017). Further, Suntrup et al. (2015) also used voxel-based imaging analysis to examine whether stroke location is associated with dysphagia in 200 acute stroke patients. They reported that damage to the right superior longitudinal fasciculus or the temporal part of the right superior longitudinal fasciculus increased the odds of dysphagia diagnosis by ~4 times. This tract was also reported in a more recent retrospective study that investigated the association between lesion location (using VLSM) and physiological aspects of swallowing (rated using the MBSIMP©TM and the PAS) in 68 acute stroke patients (Wilmskoetter et al., 2019). After controlling for age, time between measurements, and lesion volume, this study found that lesions including the superior longitudinal fasciculus were associated (to a small extent) with impairment in three physiological components. Specifically, the superior longitudinal fasciculus was implicated in 1.7% of lesioned voxels associated with increased PAS scores, 8.8% of voxels associated with pharyngeal residue, and 0.1% of voxels associated with impaired laryngeal elevation (Wilmskoetter et al., 2019).
Corona radiata
The corona radiata is a collection of both ascending and descending white matter tracts that spread toward the cortex and connect with the internal capsule. Three studies found that damage to the corona radiata was associated with some swallowing deficits. Galovic et al. reported two interesting findings regarding this region in their 2017 study examining the associations between lesion locations and impaired oral intake at ~1 and 4 weeks post stroke. First, they found that at ~1-week post-stroke, the statistical map of voxels associated with impaired oral intake included lesions in the superior corona radiata at a greater extent than any other area (65% of lesioned voxels). Secondly, at the same time point, the percent of damage in this area was negatively correlated with the degree of oral intake and the majority of patients with lesions in more >50% of the corona radiata had impaired oral intake (Galovic et al., 2017). In the 2016 study by the same research group, the corona radiata was identified in 12% of lesioned voxels associated with decreased oral intake at 48 h post-stroke (Galovic et al., 2016), further implicating this tract in swallowing control. The Wilmskoetter et al. study (2019) also reported that lesions including the right superior corona radiata were associated to a small extent with impaired laryngeal elevation (voxel overlap of 0.2%), impaired laryngeal vestibular closure (voxel overlap of 0.1%), and pharyngeal residue (voxel overlap of 1.2%). Lesions including the right posterior corona radiata were associated to a slightly larger extent with pharyngeal residue scores (voxel overlap of 8.8%) (Wilmskoetter et al., 2019). Finally, as reported previously, Lee et al. (2020) found that bilateral internal capsule/corona radiata/basal ganglia lesions (all grouped together) were prognosticated persistent dysphagia (Lee et al., 2020).
Corpus callosum
The corpus callosum (CC) was identified in two papers as important in swallowing control. In children with CP and left hemisphere lesions affecting primarily the sensorimotor cortex area (n = 13), increased clinical signs of dysphagia were correlated with reduced structural integrity of the corpus callosum quantified by FA decrease (r = −0.667, p = 0.013), fiber count decrease (r = −0.829, p < 0.001), and radial diffusivity increase (r = 0.594, p = 0.032) (Mouräo et al., 2017). In particular, reduced fiber count in the middle (r = −0.762, p = 0.002) and posterior (r = −0.739, p = 0.004) corpus callosum was associated with increased (worse) total score on the Dysphagia Disorder Survey for this group of children. A similar pattern was not observed for the group of children with right hemisphere lesions (n = 7), however the vast majority of these children had subcortical or peri-ventricular white matter (PVWM) lesions affecting intra-hemispheric connections. The authors concluded that CC integrity and inter-hemispheric communication might be more critical for swallowing control when the sensorimotor cortex is impacted, and not as critical when subcortical intra-hemispheric connections are disrupted (Mouräo et al., 2017). In the study by Li et al. (2014) including stroke patients with and without dysphagia and healthy controls, mean FA for the corpus callosum was significantly decreased in stroke patients with dysphagia when compared to the healthy controls, but when these stroke patients were compared to the group of patients without dysphagia, this difference was not significant.
External capsule
The external capsule, a series of association tracts between the putamen and claustrum, was reported in three studies. In the 2016 Galovic et al. study, 10% of lesioned voxels associated with impaired oral intake at the acute stroke phase (<48 h after imaging) overlapped the external capsule (Galovic et al., 2016). In the 2017 study by the same group, 8% of lesioned voxels associated with impaired oral intake at 1-week post-stroke overlapped this white matter area (Galovic et al., 2017). Finally, Wilmskoetter et al. (2019) reported that damage to the right external capsule was associated to some extent with impaired laryngeal elevation (voxel overlap of 8.2%) and impaired laryngeal vestibule closure (voxel overlap of 5.5%) in their sample of 68 patients post stroke (Wilmskoetter et al., 2019).
Three Additional Themes
In addition to insights on specific white matter tracts, there were several studies that generally investigated white matter and its role in swallowing control, without specifying tract locations. Three themes emerged from this literature and were reinforced by some previously discussed studies. These were topics on lesion severity, hemispheric involvement, and time post-stroke.
Lesion Severity
Although lesion severity was not consistently reported in all studies included in this synthesis, six studies indicated that severity of the white matter lesion impacts components of swallowing. In a retrospective study of 63 mild stroke patients (NIHSS ≤ 5), severity of white matter lesions (measured using the Fazekas scale) was correlated with prolonged oral transit time (r = 0.384, p = 0.003) and increased penetration occurrences (r = 0.322, p = 0.015), even after controlling for variables such as age, sex, initial stroke severity, lesion laterality, and lesion location (Moon et al., 2017). In addition, a larger retrospective study including 322 stroke patients found that a higher NIHSS score (indicating higher stroke severity) and more severe white matter hyperintensities identified in MRI scans were both identified as risk factors for suspected dysphagia as measured with the Gugging Swallow Screen. However, when the analysis was restricted to patients with supratentorial damage, white matter hyperintensities did not remain significant risk factors (Fandler et al., 2017). Further, severity of damage to one specific white matter tract, the CBT, predicted prognosis for dysphagia recovery in two studies by Jang et al. (2020a,b).
In another study, by Kumar et al. (2012), the aim was to examine the influence of age, NIHSS score, time post stroke, and lesion characteristics in predicting placement of a percutaneous endoscopic gastrostomy (PEG) tube in 77 patients with severe dysphagia resulting from an acute-subacute hemispheric lesion. Baseline NIHSS score and bilateral hemispheric involvement were the most significant predictors of PEG tube placement in this cohort (Kumar et al., 2012). Further, as reported earlier, in the study by Galovic et al. (2017) the amount of damage in the superior corona radiata was negatively correlated with the degree of oral intake, further implicating that white matter lesion load or severity plays a role in the development of swallowing difficulties (Galovic et al., 2017).
Finally, in a prospective study of 49 healthy adults (43 to 79 years of age), VFSS evaluations and a brain MRI scan were performed in order to examine the effect of subtle changes to white matter, or “unidentified bright objects,” on temporal/durational aspects of swallowing. Results showed that total swallow duration (p < 0.009) and oral transit duration (p < 0.047) differed significantly by MRI score, i.e., by number of “unidentified bright objects” in white matter (Levine et al., 1992). This was the only study included in this review that indicated that even in healthy individuals, small changes/aberrations to white matter might be influential for swallowing control.
Hemispheric Involvement
Three studies found a potential effect of lateralization of a white matter lesion to dysphagia diagnosis and/or severity. Cola et al. (2010) investigated 20 acute stroke patients, ten with left subcortical damage and ten with right subcortical damage. They found a significant statistical interaction between hemisphere and lesion location (chi square = 9.85, p = 0.002) and concluded that lesions to the left PVWM may be more disruptive to swallowing that right PVWM lesions. On the other hand, the study by Wilmskoetter et al. (2019) found that the majority of gray and white matter areas implicated in swallowing dysfunction in their post-stroke sample were in the right hemisphere. However, they also observed that two of four pharyngeal components of the MBSIMP©TM were associated with some lesions to the left hemisphere, thus concluding that although both hemispheres play a role in swallowing control, the control of the right hemisphere appears to be more prominent (Wilmskoetter et al., 2019). Similarly, the study by Mouräo et al. (2017) reported that children with CP and right hemisphere lesions (the majority of which were in the PVWM area) presented with more severe clinical dysphagia compared to children with CP with left hemisphere lesions (Mouräo et al., 2017). However, the majority of children in the left hemisphere group did not have PVWM lesions and there was a relatively small sample of children in each subgroup (13 left hemisphere, 7 right hemisphere), limiting the interpretation of this finding. Regardless of the individual contributions of left and right hemispheres, there is evidence that bilateral lesions, particularly to the pyramidal tract, tend to be more disruptive than unilateral lesions (Kumar et al., 2012; Ko et al., 2019; Jang et al., 2020a,b).
Time Post-stroke
Additionally, two studies demonstrated that white matter damage may have particular clinical relevance in the acute post-stroke phase. Galovic et al. (2017) reported that the map of lesioned areas associated with impaired oral intake at 1-week post-stroke affected white matter structures in 89% (of the voxels), whereas the respective map for patients with persistent dysphagia at 4 weeks post stroke covered mostly gray matter areas, and only 24% white matter (Galovic et al., 2017). Similarly, the same research team previously (2013) reported that patients with damage to PVWM had higher aspiration risk at 48 h post-stroke compared to patients without PVWM damage (OR = 4.8, p < 0.001) (Galovic et al., 2013). Both studies indicate that disruptions in white matter areas early post-stroke likely disrupt the communication between gray matter areas that are critical in swallowing, but also that recovery of these connections can occur quickly and can be essential in helping restore swallowing function.
Gaps in the Investigation of White Matter and Swallowing
The last research question (Research Question 4) sought to identify specific gaps in the literature in order to help guide future research in this area. Through our qualitative synthesis three major gaps were identified. These gaps were: (1) limited representation of populations, (2) imaging and swallowing methodology, and (3) research design and statistical rigor.
Limited Representation of Populations
Two clinical populations were represented in the available literature: patients post stroke (20 studies) and children with cerebral palsy (1 study), along with one study examining variability within healthy adults (Levine et al., 1992). Representation across age, race, and ethnicity was significantly limited (Table 2). Only one study included pediatric patients; all other studies included wide adult age ranges, often without accounting for effects of age in their statistical analysis. Finally, only two studies reported race, and one reported ethnicity.
Imaging and Swallowing Methodology
Studies included in this review greatly varied in both imaging and swallowing methodology used, and critically few used current gold standard methodology for both measures. Specifically, most identified articles measured white matter lesions and rarely used methods to measure white matter integrity, such as tractography, fractional anisotropy, radial diffusivity, mean diffusivity, and fibers count. There were also inconsistences in the reporting of imaging parameters (such as signal strength or scanner type), which limits interpretation of findings. However, clinical scales that were reported across multiple studies, such as the Fazekas scale, improved comparability between those studies. In regard to swallowing evaluation and analysis, relatively few papers used gold-standard swallowing measurement methods (VFSS or FEES) in conjunction with validated analysis tools (Table 4). Even when VFSS or FEES methodologies were utilized, the measures used to analyze the data were often limited or not validated (Table 4).
Research Design and Statistical Rigor
Finally, issues with research design or statistical rigor were observed across many studies (see Tables 1, 4). Several retrospective studies included inconsistent assessment methods or lacked carefully controlled research protocols. Further, few studies provided evidence of adequate power or reported blinding outcome assessors, and less than half of the studies thoroughly controlled for the potential impact of confounding variables in their statistical analysis. One key area that should also be highlighted in future studies is incorporation of confounding factors in research design and analysis.
These identified gaps in representation, methodology, and study design are important and need to be carefully considered in future research.
Discussion
The majority of swallowing neurophysiology work has focused on the contributions of CNS gray matter in the control of human swallowing, and much less attention has been given to the white matter tracts that form connections between gray matter areas. These tracts hold promise for patients with dysphagia because, in addition to being communication highways, they are also known to be primary drivers of recovery after injury or disease and are highly capable of adaptation with rehabilitation and re-learning (Trivedi et al., 2008; Schulz et al., 2014; Kato and Izumiyama, 2017, p. 201; Barghi et al., 2018).
In this systematic review, we sought to identify and systematically evaluate the literature describing the role of white matter in the neural control of swallowing in order to answer four primary questions: what patient populations have been studied in this literature; what methodologies have been used to assess white matter integrity and swallowing; what specific white matter tracts are implicated in swallowing control; and what are the main gaps in the literature that need to be addressed in future research.
To summarize, we identified 22 articles that fit our inclusion criteria. All studies were observational, i.e., there was no intervention assessed, and almost half followed a retrospective cohort design, with the other half being either case control studies or variations of prospective cohort designs. Using a modified NIH quality assessment protocol (Study Quality Assessment Tools | NHLBI, NIH, 2019) (Table 1), five studies were rated as having “Good” quality, 15 studies were of “Fair” quality; and two studies were rated as “Poor” and were excluded from the qualitative synthesis used to answer question 3 of this review.
Regarding the first research question (populations), stroke was by far the most represented diagnosis in the identified literature. Only two of the 22 reviewed papers examined different populations; one study examined children with CP, and one focused on healthy older adults. The majority of studies included acute adult stroke patients, with almost exclusively first strokes with no prior infarcts or comorbidities, and a mix of types of strokes (see Table 4). This proportionally high representation of one diagnosis in the literature is likely due to relevance and convenience. Patients post stroke often exhibit white matter damage with subsequent deficits, and also have readily available neuroimaging scans that can be studied retrospectively. Since neuroimaging is costly, it is unsurprising that research on this topic has started with a population who has existing scans and related damage. However, white matter damage has been documented in other populations that are also at high risk for developing swallowing difficulties, including people with traumatic brain injury (Herrera et al., 2016), dementia (Love and Miners, 2015), chronic drug abuse (Narayana et al., 2014), multiple sclerosis (Tassorelli et al., 2008), and gestational hypoxia (Baud et al., 2004; Kaur and Ling, 2009), as well as in typical aging (Metzler-Baddeley et al., 2019). In addition to a gap in representation of clinical populations, few of the reviewed studies included healthy control participants or focused on white matter integrity measures in healthy participants, leaving an additional gap in our understanding of white matter connections in normal swallowing.
In regard to the second question (methodologies to assess white matter and swallowing), we observed an interesting dichotomy. The majority of studies that measured characteristics of the white matter structures themselves (e.g., FA, tract volume, mean diffusivity, etc.), evaluated swallowing via clinical methods or screenings, instead of using instrumental tools. On the other hand, most of the studies that examined swallowing physiology in some depth or with validated tools tended to utilize the more crude (or lesion-based) white matter imaging techniques (e.g., lesion volume calculations, lesion severity scales, etc.).
Imaging advancements in the use of DWI/DTI have allowed for a significant increase in our understanding of brain connections and their role in recovery and rehabilitation in related fields (Trivedi et al., 2008; Schlaug et al., 2009; Huber et al., 2018). For this reason, we expected to find these methodologies used in swallowing neurophysiology literature as well. However, we observed that very few of the reviewed studies utilized these sequences to investigate white matter integrity in their samples, despite the fact that many research groups had access to the relevant DWI scans. Instead, they relied on lesion-based methods, which are useful when examining patients with stroke or another pathology with specific lesions, but do not quantifiably measure white matter itself. Since lesions often affect multiple brain areas at once this approach can result in unspecified conclusions. Alternatively, techniques that measure and describe characteristics of white matter, such as FA, radial diffusivity, mean diffusivity, or fibers count, reveal changes specific to the white matter structures. These techniques are sensitive enough to detect differences even within healthy populations (Madden et al., 2008; Ziegler et al., 2010; Bennett et al., 2011; Schulz et al., 2014). The few studies in this review that used these techniques indicated that these metrics could be valid predictors for swallowing outcomes post stroke (Jang et al., 2020a,b), and they helped identify more specific white matter tracts of interest for swallowing control (Mouräo et al., 2017; Wilmskoetter et al., 2019).
To further delineate elements critical to advancing these efforts, we also reviewed these studies' swallowing methodology. Fifteen of the identified studies used gold-standard measures that allow in-depth evaluation of the oropharyngeal swallowing phases (VFSS or FEES). Further, several of these studies used timing/temporal measures to quantify imaging analysis, and one study used the MBSIMP©TM to standardize clinical interpretation. This allowed for more in-depth discussion of subcomponents of swallowing in relation to nervous system damage. For the remaining studies that did not use instrumental methods, it is difficult to adequately characterize the underlying mechanisms of dysphagia in their participants. This was, in some cases, partially ameliorated with the use of validated and standardized clinical assessments. Our understanding of swallowing physiology in relation to white matter integrity could be improved through detailed kinematic or morphometric analysis methods (Molfenter and Steele, 2014; Pearson et al., 2016), which help assess aspects of swallowing physiology more objectively.
Our findings on question 2 highlight the importance of combining high quality neuroimaging and swallowing physiology expertise to comprehensively investigate the neural control of swallowing. The need for strong interdisciplinary collaborations that will enable the combination of recent advanced imaging methods with in-depth swallowing evaluation and analysis techniques is apparent.
Given the methodological issues described above, for question 3 (i.e., white matter tracts implicated in swallowing control), we completed a synthesis of the findings of 20/22 studies that had a quality rating of at least Fair. Since the methods and results of the studies reviewed were heterogeneous, we cannot definitively describe the specific role of white matter in the neural control of swallowing. However, through our synthesis, the following white matter tracts or structures were frequently reported (Figure 2): the pyramidal tract (as a whole) and some of its subparts like the corticospinal and corticobulbar tracts, the internal capsule, the superior longitudinal fasciculus, the corona radiata, the corpus callosum, and the external capsule.
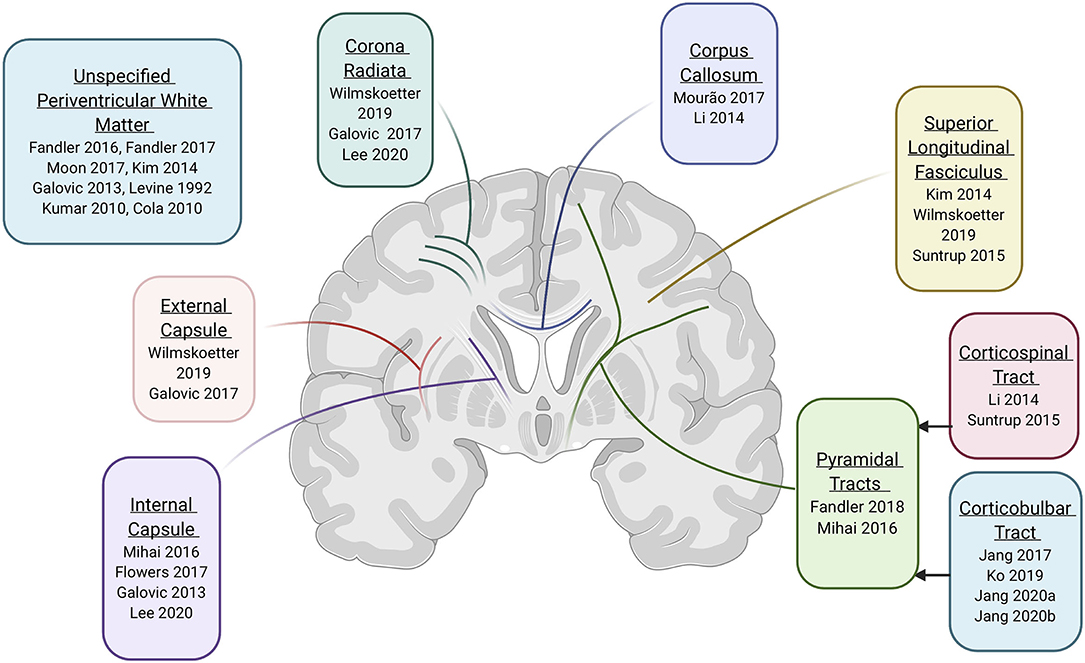
Figure 2. Evidence for specific white matter tracts involved in neural control of swallowing (created with Biorender.com).
Fibers in three of these tracts/structures are categorized as projection fibers. Projection fibers were implicated in four of five studies that received a “Good” quality rating. Projection fibers connect the cortex with deep nuclei, lower parts of the brain (i.e., the brainstem), and the spinal cord. The role of the cortex and brainstem are extensively documented in animal and human studies of the neural control of swallowing (e.g., Jean, 1984; Robbins et al., 1993; Hamdy et al., 1997; Malandraki et al., 2011), so it logically follows that disruptions in the connections between these areas could affect swallowing. Specifically, dysphagia was frequently associated with bilateral damage to the pyramidal tracts, internal capsule, or corona radiata. The pyramidal tract originates in the bilateral supplementary motor area and dorsal premotor area (Wang et al., 2019) and ends at the brainstem (corticobulbar tract) or spinal cord (corticospinal tract) (Lohia and McKenzie, 2020b), and was the most clearly implicated projection tract. This is unsurprising since the supplementary motor area (SMA) has been repeatedly implicated in volitional swallowing, particularly in the preparatory phase (Huckabee et al., 2003; Satow et al., 2004; Hamdy, 2006; Malandraki et al., 2009), and communication between the SMA and subcortical areas (e.g., the basal ganglia and brainstem) is needed for swallow initiation (Hamdy et al., 1996). A subpart of the pyramidal tract, the corticobulbar tract, was reported in five reviewed papers, with two papers finding that severity of CBT injury appears to have prognostic value for predicting swallowing recovery post-stroke (Jang et al., 2020a,b).
Fibers in the superior longitudinal fasciculus and external capsule are categorized as association fibers and connect brain regions within the same hemisphere. In two of the papers rated as “Good,” damage to these fibers was also associated with swallowing deficits. The superior longitudinal fasciculus carries input from parietal sensorimotor centers to frontal motor areas influential for swallowing coordination and initiation (Schmahmann et al., 2008). The external capsule connects the pre-frontal cortex and the supplementary motor area with the basal ganglia, and it has been hypothesized to be the key for the engagement of the basal ganglia in swallowing motor control (Schmahmann et al., 2008). Damage to these tracts was associated with impaired oral intake post-stroke and was associated (to a small extent) with deficits in specific pharyngeal subcomponents of swallowing in one study (Wilmskoetter et al., 2019).
Finally, one commissural tract, the corpus callosum, was identified in two studies. It is established that swallowing involves bilateral cerebral control (Hamdy et al., 1999; Malandraki et al., 2009), and the main pathway connecting the hemispheres is the corpus callosum. Although direct evidence is scarce, it has been theorized that the corpus callosum may be involved in communications between swallowing areas in the right and left hemispheres (Mouräo et al., 2017). In the Mouräo et al.' study (2017), which included a relatively small sample of children with CP (n = 20), it was concluded that disruptions in the corpus callosum were more influential for swallowing control when lesions affected cortical MCA areas, thus suggesting some influential disruptions in interhemispheric connections. Given that the quality of the studies implicating the corpus callosum was “Fair,” further research is needed to elucidate the role of inter-hemispheric connectivity for swallowing control.
In addition to insight on specific white matter tracts/structures of interest, there were three common themes regarding severity, hemispheric contribution, and time post-stroke that emerged from this literature. First, studies provided evidence that severity of white matter lesions was predictive of dysphagia severity and/or recovery (Jang et al., 2020a,b). Further, even among healthy individuals, changes to white matter were associated with changes in swallowing control (i.e., total swallow duration and oral transit duration) (Levine et al., 1992). This finding is consistent with prior literature, supporting that severity of impairment from stroke (NIHSS score) moderately predicts clinically relevant dysphagia (Jeyaseelan et al., 2015), and lesion severity also predicts post-stroke dysphagia (Otto et al., 2016; Cabib et al., 2017; Rofes et al., 2018) and warrants further investigation.
The second theme involved the role of each hemisphere's white matter in swallowing control. White matter lesions of both hemispheres were reported to correlate with swallowing deficits, but more evidence pointed to the potential influence of the right hemisphere's white matter areas (Mouräo et al., 2017; Wilmskoetter et al., 2019). This finding is not surprising, as several prior neuroimaging studies have also shown that gray matter areas of the right hemisphere play a more prominent role in the pharyngeal phase of swallowing compared to areas in the left hemisphere (Robbins et al., 1993; Daniels et al., 1996, p. 199; Hamdy et al., 1996; Malandraki et al., 2010; Wilmskoetter et al., 2018). In addition, there was consistent evidence that bilateral white matter damage is particularly disruptive to the neural control of swallowing (Kumar et al., 2012; Ko et al., 2019; Jang et al., 2020a,b), also paralleling related literature on bilateral gray matter damage and dysphagia (Ickenstein et al., 2003).
Finally, two studies (Galovic et al., 2013, 2017) indicated that disruptions in white matter connections are particularly disruptive to swallowing in the early post-stroke phase (i.e., within a week post stroke), but have quick recovery potential and can help restore swallowing function. The critical role of white matter in swallowing recovery was further highlighted in two additional studies that showed swallowing recovery upon white matter tracts adaptations post stroke (Mihai et al., 2016; Jang et al., 2017). These studies prompt questions surrounding the critical role that white matter plasticity may play in swallowing recovery. They also identify an area in need of rigorous exploration and with high potential impact for swallowing recovery and rehabilitation.
Limitations
A systematic review is always limited by the available evidence. We identified only twenty-two studies that met our inclusion criteria, even though our inclusion criteria were rather broad. Another limitation is that meta-analysis was not possible due to heterogeneous study designs, and all synthesis of findings was qualitative. Finally, in order to synthesize data, we used a quality assessment, but we had to modify the most relevant quality assessment available because not all components applied to this type of observational research, which we acknowledge introduces some bias.
Conclusion
This systematic review highlighted the critical role of white matter in the neural control of swallowing, which is an area that has been significantly understudied. Findings indicated that white matter damage can be directly tied to swallowing deficits, and several white matter tracts (such as the pyramidal tracts, internal capsule, superior longitudinal fasciculus, corona radiata, corpus callosum, and external capsule) were implicated across studies. Despite these findings, several methodological limitations were also identified in most reviewed studies and need to be addressed in the future. It is our hope that this systematic review will serve as a starting point for future research that will build a more thorough understanding of the role of white matter in the neural control of normal swallowing, and, more critically, will inspire future work on delineating its role in dysphagia recovery and rehabilitation.
Data Availability Statement
The raw data supporting the conclusions of this article will be made available by the authors, without undue reservation.
Author Contributions
GM conceptualized the study, study design, and is the guarantor of the study. GM, AA, and RH designed the study and wrote the article. BM designed and performed the search, with input from all other authors. HC provided input on the methods. AA and RA screened, read, and assessed all studies. GM resolved disagreements and trained AA and RA in qualitative review. All authors read and revised manuscript drafts, and approved the final manuscript version.
Funding
This study was partially supported by the National Institute on Deafness and Other Communication Disorders Early Investigator R21 Grant (Grant 1R21DC015867-01A1, PI: Malandraki). Publication of this article was funded in part by the Purdue University Libraries Open Access Publishing Fund.
Conflict of Interest
The authors declare that the research was conducted in the absence of any commercial or financial relationships that could be construed as a potential conflict of interest.
Acknowledgments
Figure 2 was created with Biorender.com.
Supplementary Material
The Supplementary Material for this article can be found online at: https://www.frontiersin.org/articles/10.3389/fnhum.2021.628424/full#supplementary-material
Abbreviations
CNS, central nervous system; NGT, nasogastric tube; DWI, diffusion weighted imaging; FA, fractional anisotropy; TV, tract volume; VFSS, videofluoroscopic swallow study; PAS, penetration aspiration scale; FOIS, functional oral impact scale; CBT, corticobulbar tract; CD, cannot determine; GUSS, Gugging Swallow Screen; LA, leukoaraiosis (hyperintensity around ventricles); ASHA, NOMS American Speech Language Hearing Association National Outcome Measurement System; OR, odds ratio; WM, white matter; MCA, middle cerebral artery; NIHSS, national institute of health stroke scale; VLSM, voxel based lesion symptom mapping; ROI, regions of interest; MBSIMP©TM, Modified Barium Swallow Impairment Profile; CNS, Canadian Neurological Scale; MRI, magnetic resonance imaging; SLP, speech language pathologist; NR, Not Rated; FEES, Fiberoptic Endoscopic Evaluation of Swallowing; GMFCS, gross motor function classification scale; MACS, manual ability classification scale; RD, radial diffusivity; MD, mean diffusivity; FC, fibers count; DDS, Dysphagia Disorder Survey; DMSS, dysphagia management staging scale; fMRI, functional magnetic resonance imaging; BODS-2, Bogenhausen Dysphagia Score Part 2; FEDSS, fiberoptic endoscopic dysphagia severity scale; SMA, supplementary motor area; PVWM, periventricular white matter.
References
Alexander, A. L., Lee, J. E., Lazar, M., and Field, A. S. (2007). Diffusion tensor imaging of the brain. Neurotherapeutics 4, 316–329. doi: 10.1016/j.nurt.2007.05.011
Amri, M., Car, A., and Jean, A. (1984). Medullary control of the pontine swallowing neurones in sheep. Exp. Brain Res. 55, 105–110. doi: 10.1007/BF00240503
Awad, I. A., Johnson, P. C., Spetzler, R. F., and Hodak, J. A. (1986). Incidental subcortical lesions identified on magnetic resonance imaging in the elderly. II. Postmortem pathological correlations. Stroke 17, 1090–1097. doi: 10.1161/01.STR.17.6.1090
Barghi, A., Allendorfer, J. B., Taub, E., Womble, B., Hicks, J. M., Uswatte, G., et al. (2018). Phase II randomized controlled trial of constraint-induced movement therapy in multiple sclerosis. Part 2: effect on white matter integrity. Neurorehabil. Neural Repair. 32, 233–241. doi: 10.1177/1545968317753073
Bartolome, G. (2006). “Kapitel 10 - Grundlagen der funktionellen Dysphagietherapie (FDT),” in Schluckstörungen (Dritte Ausgabe), eds. G. Bartolome, H. Schröter-Morasch, D. Buchholz, H. Feussner, C. Hannig, S. Neumann, et al. (Munich: Urban and Fischer), 245–370.
Bates, E., Wilson, S. M., Saygin, A. P., Dick, F., Sereno, M. I., Knight, R. T., et al. (2003). Voxel-based lesion–symptom mapping. Nat. Neurosci. 6, 448–450. doi: 10.1038/nn1050
Baud, O., Daire, J.-L., Dalmaz, Y., Fontaine, R. H., Krueger, R. C., Sebag, G., et al. (2004). Gestational hypoxia induces white matter damage in neonatal rats: a new model of periventricular leukomalacia. Brain Pathol. 14, 1–10. doi: 10.1111/j.1750-3639.2004.tb00492.x
Bennett, I. J., Madden, D. J., Vaidya, C. J., Howard, J. H., and Howard, D. V. (2011). White matter integrity correlates of implicit sequence learning in healthy aging. Neurobiol. Aging 32, 2317.e1-2317.e12. doi: 10.1016/j.neurobiolaging.2010.03.017
Bhattacharyya, N. (2014). The prevalence of dysphagia among adults in the United States. Otolaryngol. Head Neck Surg. 151, 765–769. doi: 10.1177/0194599814549156
Bosma, J. F. (1957). Deglutition: pharyngeal stage. Physiol. Rev. 37, 561–561. doi: 10.1152/physrev.1957.37.4.561-s
Breier, J. I., Hasan, K. M., Zhang, W., Men, D., and Papanicolaou, A. C. (2008). Language dysfunction after stroke and damage to white matter tracts evaluated using diffusion tensor imaging. Am. J. Neuroradiol. 29, 483–487. doi: 10.3174/ajnr.A0846
Buklina, S. B. (2005). The corpus callosum, interhemisphere interactions, and the function of the right hemisphere of the brain. Neurosci. Behav. Physiol. 35, 473–480. doi: 10.1007/s11055-005-0082-5
Cabib, C., Ortega, O., Vilardell, N., Mundet, L., Clavé, P., and Rofes, L. (2017). Chronic post-stroke oropharyngeal dysphagia is associated with impaired cortical activation to pharyngeal sensory inputs. Eur. J. Neurol. 24, 1355–1362. doi: 10.1111/ene.13392
Car, A., and Roman, C. (1969). Deglutitions and oesophageal reflex contractions induced by electrical stimulation of the medulla oblongata. Exp. Brain Res. 11, 75–92.
Chanraud, S., Zahr, N., Sullivan, E. V., and Pfefferbaum, A. (2010). MR diffusion tensor imaging: a window into white matter integrity of the working brain. Neuropsychol. Rev. 20, 209–225. doi: 10.1007/s11065-010-9129-7
Clark, K. A., Nuechterlein, K. H., Asarnow, R. F., Hamilton, L. S., Phillips, O. R., Hageman, N. S., et al. (2011). Mean diffusivity and fractional anisotropy as indicators of disease and genetic liability to schizophrenia. J. Psychiatr. Res. 45, 980–988. doi: 10.1016/j.jpsychires.2011.01.006
Cola, M. G., Daniels, S. K., Corey, D. M., Lemen, L. C., Romero, M., and Foundas, A. L. (2010). Relevance of subcortical stroke in dysphagia. Stroke 41, 482–486. doi: 10.1161/STROKEAHA.109.566133
Crary, M. A., Mann, G. D. C., and Groher, M. E. (2005). Initial psychometric assessment of a functional oral intake scale for dysphagia in stroke patients. Arch. Phys. Med. Rehabil. 86, 1516–1520. doi: 10.1016/j.apmr.2004.11.049
Daniels, S. K., Ballo, L. A., Mahoney, M.-C., and Foundas, A. L. (2000). Clinical predictors of dysphagia and aspiration risk: outcome measures in acute stroke patients. Arch. Phys. Med. Rehabil. 81, 1030–1033. doi: 10.1053/apmr.2000.6301
Daniels, S. K., Brailey, K., and Foundas, A. L. (1999). Lingual discoordination and dysphagia following acute stroke: analyses of lesion localization. Dysphagia 14, 85–92. doi: 10.1007/PL00009592
Daniels, S. K., Foundas, A. L., Iglesia, G. C., and Sullivan, M. A. (1996). Lesion site in unilateral stroke patients with dysphagia. J. Stroke Cerebrovasc. Dis. 6, 30–34. doi: 10.1016/S1052-3057(96)80023-1
De Erausquin, G. A., and Alba-Ferrara, L. (2013). What does anisotropy measure? Insights from increased and decreased anisotropy in selective fiber tracts in schizophrenia. Front. Integr. Neurosci. 7:9. doi: 10.3389/fnint.2013.00009
de Figueiredo, E. H. M. S. G., Borgonovi, A. F. N. G., and Doring, T. M. (2011). Basic concepts of MR imaging, diffusion mr imaging, and diffusion tensor imaging. Magn. Reson. Imaging Clin. N. Am. 19, 1–22. doi: 10.1016/j.mric.2010.10.005
de Lama Lazzara, G., Lazarus, C., and Logemann, J. A. (1986). Impact of thermal stimulation on the triggering of the swallowing reflex. Dysphagia 1, 73–77. doi: 10.1007/BF02407117
Doty, R. W. (1951). Influence of stimulus pattern on reflex deglutition. Am. J. Physiol. 166, 142–158. doi: 10.1152/ajplegacy.1951.166.1.142
Doty, R. W. (1968). Neural organization of deglutition. Alimentary Canal 1861. Available online at: https://ci.nii.ac.jp/naid/10011018245/ (accessed July 31, 2020).
Emos, M. C., and Agarwal, S. (2020). “Neuroanatomy, Internal Capsule,” in StatPearls (Treasure Island, FL: StatPearls Publishing). Available online at: http://www.ncbi.nlm.nih.gov/books/NBK542181/ (accessed July 29, 2020).
Fandler, S., Gattringer, T., Eppinger, S., Doppelhofer, K., Pinter, D., Niederkorn, K., et al. (2017). Frequency and predictors of dysphagia in patients with recent small subcortical infarcts. Stroke 48, 213–215. doi: 10.1161/STROKEAHA.116.015625
Fandler, S., Gattringer, T., Pinter, D., Pirpamer, L., Borsodi, F., Eppinger, S., et al. (2018). Dysphagia in supratentorial recent small subcortical infarcts results from bilateral pyramidal tract damage. Int. J. Stroke 13, 815–819. doi: 10.1177/1747493018778141
Fazekas, F., Chawluk, J. B., Alavi, A., Hurtig, H. I., and Zimmerman, R. A. (1987). MR signal abnormalities at 1.5 T in Alzheimer's dementia and normal aging. AJR Am. J. Roentgenol. 149, 351–356. doi: 10.2214/ajr.149.2.351
Flowers, H. L., AlHarbi, M. A., Mikulis, D., Silver, F. L., Rochon, E., Streiner, D., et al. (2017). MRI-based neuroanatomical predictors of dysphagia, dysarthria, and aphasia in patients with first acute ischemic stroke. Cerebrovasc. Dis. Extra 7, 21–34. doi: 10.1159/000457810
Frank, L. R. (2001). Anisotropy in high angular resolution diffusion-weighted MRI. Magn. Reson. Med. 45, 935–939. doi: 10.1002/mrm.1125
Galovic, M., Leisi, N., Müller, M., Weber, J., Abela, E., Kägi, G., et al. (2013). Lesion location predicts transient and extended risk of aspiration after supratentorial ischemic stroke. Stroke 44, 2760–2767. doi: 10.1161/STROKEAHA.113.001690
Galovic, M., Leisi, N., Müller, M., Weber, J., Tettenborn, B., Brugger, F., et al. (2016). Neuroanatomical correlates of tube dependency and impaired oral intake after hemispheric stroke. Eur. J. Neurol. 23, 926–934. doi: 10.1111/ene.12964
Galovic, M., Leisi, N., Pastore-Wapp, M., Zbinden, M., Vos, S. B., Mueller, M., et al. (2017). Diverging lesion and connectivity patterns influence early and late swallowing recovery after hemispheric stroke. Hum. Brain Mapp. 38, 2165–2176. doi: 10.1002/hbm.23511
Gordon, C., Hewer, R. L., and Wade, D. T. (1987). Dysphagia in acute stroke. Br. Med. J. (Clin. Res. Ed) 295, 411–414. doi: 10.1136/bmj.295.6595.411
Hamdy, S., Aziz, Q., Rothwell, J. C., Hobson, A., Barlow, J., and Thompson, D. G. (1997). Cranial nerve modulation of human cortical swallowing motor pathways. Am. J. Physiol. Gastro. Liver Physiol. 272, G802–G808. doi: 10.1152/ajpgi.1997.272.4.G802
Hamdy, S., Aziz, Q., Rothwell, J. C., Singh, K. D., Barlow, J., Hughes, D. G., et al. (1996). The cortical topography of human swallowing musculature in health and disease. Nat. Med. 2, 1217–1224. doi: 10.1038/nm1196-1217
Hamdy, S., Mikulis, D. J., Crawley, A., Xue, S., Lau, H., Henry, S., et al. (1999). Cortical activation during human volitional swallowing: an event-related fMRI study. Am. J. Physiol. Gastro. Liver Physiol. 277, G219–G225. doi: 10.1152/ajpgi.1999.277.1.G219
Herrera, J. J., Bockhorst, K., Kondraganti, S., Stertz, L., Quevedo, J., and Narayana, P. A. (2016). Acute white matter tract damage after frontal mild traumatic brain injury. J. Neurotrauma 34, 291–299. doi: 10.1089/neu.2016.4407
Huber, E., Donnelly, P. M., Rokem, A., and Yeatman, J. D. (2018). Rapid and widespread white matter plasticity during an intensive reading intervention. Nat. Commun. 9:2260. doi: 10.1038/s41467-018-04627-5
Huckabee, M.-L., Deecke, L., Cannito, M. P., Gould, H. J., and Mayr, W. (2003). Cortical control mechanisms in volitional swallowing: the bereitschaftspotential. Brain Topogr. 16, 3–17. doi: 10.1023/A:1025671914949
Huisman, T. A. G. M. (2010). Diffusion-weighted and diffusion tensor imaging of the brain, made easy. Cancer Imaging 10, S163–S171. doi: 10.1102/1470-7330.2010.9023
Ickenstein, G. W., Kelly, P. J., Furie, K. L., Ambrosi, D., Rallis, N., Goldstein, R., et al. (2003). Predictors of feeding gastrostomy tube removal in stroke patients with dysphagia. J. Stroke Cerebrovasc. Dis. 12, 169–174. doi: 10.1016/S1052-3057(03)00077-6
Jang, S., Kim, J., Seo, Y., and Kwak, S. (2017). Recovery of an injured corticobulbar tract in a patient with stroke: a case report. Medicine 96:e7636. doi: 10.1097/MD.0000000000007636
Jang, S. H., Kwak, S. Y., Chang, C. H., Jung, Y. J., Kim, J., Kim, S. H., et al. (2020a). Prognostic prediction of dysphagia by analyzing the corticobulbar tract in the early stage of intracerebral hemorrhage. Dysphagia 35, 985–992. doi: 10.1007/s00455-020-10093-3
Jang, S. H., Lee, J., and Kim, M. S. (2020b). Dysphagia prognosis prediction via corticobulbar tract assessment in lateral medullary infarction: a diffusion tensor tractography study. Dysphagia. doi: 10.1007/s00455-020-10182-3. [Epub ahead of print].
Jean, A. (1984). Brainstem organization of the swallowing network. BBE 25, 109–116. doi: 10.1159/000118856
Jean, A., Car, A., and Roman, C. (1975). Comparison of activity in pontine versus medullary neurones during swallowing. Exp. Brain Res. 22, 211–220. doi: 10.1007/BF00237690
Jeyaseelan, R. D., Vargo, M. M., and Chae, J. (2015). National Institutes of Health Stroke Scale (NIHSS) as an early predictor of poststroke dysphagia. PMR 7, 593–598. doi: 10.1016/j.pmrj.2014.12.007
Kato, H., and Izumiyama, M. (2017). Damage to the pyramidal tract leads to post-stroke reorganization of brain motor network. J. Neurol. Sci. 381, 598–599. doi: 10.1016/j.jns.2017.08.1687
Kaur, C., and Ling, E. A. (2009). Periventricular white matter damage in the hypoxic neonatal brain: Role of microglial cells. Progress Neurobiol. 87, 264–280. doi: 10.1016/j.pneurobio.2009.01.003
Kessler, J. P., and Jean, A. (1985). Identification of the medullary swallowing regions in the rat. Exp. Brain Res. 57, 256–263. doi: 10.1007/BF00236530
Kim, S. Y., Kim, T. U., Hyun, J. K., and Lee, S. J. (2014). Differences in videofluoroscopic swallowing study (VFSS) findings according to the vascular territory involved in stroke. Dysphagia 29, 444–449. doi: 10.1007/s00455-014-9525-x
Kingsley, P. B., and Monahan, W. G. (2004). Selection of the optimum b factor for diffusion-weighted magnetic resonance imaging assessment of ischemic stroke. Magn. Res. Med. 51, 996–1001. doi: 10.1002/mrm.20059
Ko, E. J., Choi, K. H., and Kwon, S. U. (2019). The relationship between leukoaraiosis involving contralateral corticobulbar tract and dysphagia in patients with acute unilateral corona radiata infarction with corticobulbar tract involvement. Dysphagia 34, 654–664. doi: 10.1007/s00455-018-9963-y
Kou, Z., and Iraji, A. (2014). Imaging brain plasticity after trauma. Neural Regen. Res. 9, 693–700. doi: 10.4103/1673-5374.131568
Kumar, S., Langmore, S., Goddeau, R. P., Alhazzani, A., Selim, M., Caplan, L. R., et al. (2012). Predictors of percutaneous endoscopic gastrostomy tube placement in patients with severe dysphagia from an acute-subacute hemispheric infarction. J. Stroke Cerebrovasc. Dis. 21, 114–120. doi: 10.1016/j.jstrokecerebrovasdis.2010.05.010
Langmore, S. E., Terpenning, M. S., Schork, A., Chen, Y., Murray, J. T., Lopatin, D., et al. (1998). Predictors of aspiration pneumonia: how important is dysphagia? Dysphagia 13, 69–81. doi: 10.1007/PL00009559
Lee, W. H., Lim, M. H., Seo, H. G., Seong, M. Y., Oh, B.-M., and Kim, S. (2020). Development of a novel prognostic model to predict 6-month swallowing recovery after ischemic stroke. Stroke 51, 440–448. doi: 10.1161/STROKEAHA.119.027439
Leiva-Salinas, C., and Wintermark, M. (2010). Imaging of ischemic stroke. Neuroimaging Clin. N. Am. 20, 455–468. doi: 10.1016/j.nic.2010.07.002
Leow, L. P., Huckabee, M.-L., Anderson, T., and Beckert, L. (2010). The impact of dysphagia on quality of life in ageing and Parkinson?s disease as measured by the swallowing quality of Life (SWAL-QOL) questionnaire. Dysphagia 25, 216–220. doi: 10.1007/s00455-009-9245-9
Levine, R., Robbins, J. A., and Maser, A. (1992). Periventricular white matter changes and oropharyngeal swallowing in normal individuals. Dysphagia 7, 142–147. doi: 10.1007/BF02493446
Li, S., Ma, Z., Tu, S., Zhou, M., Chen, S., Guo, Z., et al. (2014). Altered resting-state functional and white matter tract connectivity in stroke patients with dysphagia. Neurorehabil. Neural Repair. 28, 260–272. doi: 10.1177/1545968313508227
Logemann, J. A., Shanahan, T., Rademaker, A. W., Kahrilas, P. J., Lazar, R., and Halper, A. (1993). Oropharyngeal swallowing after stroke in the left basal ganglion/internal capsule. Dysphagia 8, 230–234. doi: 10.1007/BF01354543
Lohia, A., and McKenzie, J. (2020a). “Neuroanatomy, Pyramidal Tract Lesions,” in StatPearls (Treasure Island, FL: StatPearls Publishing). Available online at: http://www.ncbi.nlm.nih.gov/books/NBK540976/ (accessed July 31, 2020).
Lohia, A., and McKenzie, J. (2020b). “Neuroanatomy, Pyramidal Tract Lesions,” in StatPearls (Treasure Island, FL: StatPearls Publishing). Available at: http://www.ncbi.nlm.nih.gov/books/NBK540976/ (accessed September 7, 2020).
Love, S., and Miners, J. S. (2015). White matter hypoperfusion and damage in dementia: post-mortem assessment. Brain Pathol. 25, 99–107. doi: 10.1111/bpa.12223
Madden, D. J., Spaniol, J., Costello, M. C., Bucur, B., White, L. E., Cabeza, R., et al. (2008). Cerebral white matter integrity mediates adult age differences in cognitive performance. J. Cogn. Neurosci. 21, 289–302. doi: 10.1162/jocn.2009.21047
Malandraki, G. A., Johnson, S., and Robbins, J. (2011). Functional MRI of swallowing: from neurophysiology to neuroplasticity. Head Neck 33, S14–S20. doi: 10.1002/hed.21903
Malandraki, G. A., Sutton, B. P., Perlman, A. L., and Karampinos, D. C. (2010). Age-related differences in laterality of cortical activations in swallowing. Dysphagia 25, 238–249. doi: 10.1007/s00455-009-9250-z
Malandraki, G. A., Sutton, B. P., Perlman, A. L., Karampinos, D. C., and Conway, C. (2009). Neural activation of swallowing and swallowing-related tasks in healthy young adults: an attempt to separate the components of deglutition. Hum. Brain Mapp. 30, 3209–3226. doi: 10.1002/hbm.20743
Mann, C. J. (2003). Observational research methods. Research design II: cohort, cross sectional, and case-control studies. Emerg. Med. J. 20, 54–60. doi: 10.1136/emj.20.1.54
Martin, R. E., Goodyear, B. G., Gati, J. S., and Menon, R. S. (2001). Cerebral cortical representation of automatic and volitional swallowing in humans. J. Neurophysiol. 85, 938–950. doi: 10.1152/jn.2001.85.2.938
Martin, R. E., Kemppainen, P., Masuda, Y., Yao, D., Murray, G. M., and Sessle, B. J. (1999). Features of cortically evoked swallowing in the awake primate (Macaca fascicularis). J. Neurophysiol. 82, 1529–1541. doi: 10.1152/jn.1999.82.3.1529
Martin, R. E., MacIntosh, B. J., Smith, R. C., Barr, A. M., Stevens, T. K., Gati, J. S., et al. (2004). Cerebral areas processing swallowing and tongue movement are overlapping but distinct: a functional magnetic resonance imaging study. J. Neurophysiol. 92, 2428–2443. doi: 10.1152/jn.01144.2003
Martin, R. E., and Sessle, B. J. (1993). The role of the cerebral cortex in swallowing. Dysphagia 8, 195–202. doi: 10.1007/BF01354538
Martin-Harris, B., Brodsky, M. B., Michel, Y., Castell, D. O., Schleicher, M., Sandidge, J., et al. (2008). MBS measurement tool for swallow impairment-MBSImp: establishing a standard. Dysphagia 4, 392–405. doi: 10.1007/s00455-008-9185-9
Meadows, J. C. (1973). Dysphagia in unilateral cerebral lesions. J. Neurol. Neurosurg. Psychiatry 36, 853–860. doi: 10.1136/jnnp.36.5.853
Metzler-Baddeley, C., Mole, J. P., Sims, R., Fasano, F., Evans, J., Jones, D. K., et al. (2019). Fornix white matter glia damage causes hippocampal gray matter damage during age-dependent limbic decline. Sci. Rep. 9:1060. doi: 10.1038/s41598-018-37658-5
Mihai, P. G., Otto, M., Domin, M., Platz, T., Hamdy, S., and Lotze, M. (2016). Brain imaging correlates of recovered swallowing after dysphagic stroke: a fMRI and DWI study. Neuroimage Clin. 12, 1013–1021. doi: 10.1016/j.nicl.2016.05.006
Miller, F. R., and Sherrington, C. S. (1915). Some observations on the bucco-pharyngeal stage of reflex deglutition in the cat. Quarterly J. Exp. Physiol. 9, 147–186. doi: 10.1113/expphysiol.1915.sp000201
Moher, D., Liberati, A., Tetzlaff, J., Altman, D. G., and Group, T. P. (2009). Preferred reporting items for systematic reviews and meta-analyses: the PRISMA statement. PLoS Med 6:e1000097. doi: 10.1371/journal.pmed.1000097
Molfenter, S. M., and Steele, C. M. (2014). Kinematic and temporal factors associated with penetration–aspiration in swallowing liquids. Dysphagia 29, 269–276. doi: 10.1007/s00455-013-9506-5
Moon, H. I., Nam, J.-S., Leem, M. J., and Kim, K. H. (2017). Periventricular white matter lesions as a prognostic factor of swallowing function in older patients with mild stroke. Dysphagia 32, 480–486. doi: 10.1007/s00455-017-9788-0
Mori, S., and Zhang, J. (2006). Principles of diffusion tensor imaging and its applications to basic neuroscience research. Neuron 51, 527–539. doi: 10.1016/j.neuron.2006.08.012
Mouräo, L. F., Friel, K. M., Sheppard, J. J., Kuo, H.-C., Luchesi, K. F., Gordon, A. M., et al. (2017). The role of the corpus callosum in pediatric dysphagia: preliminary findings from a diffusion tensor imaging study in children with unilateral spastic cerebral palsy. Dysphagia 32, 703–713. doi: 10.1007/s00455-017-9816-0
Namasivayam, A. M., and Steele, C. M. (2015). Malnutrition and dysphagia in long-term care: a systematic review. J. Nutr. Gerontol. Geriatr. 34, 1–21. doi: 10.1080/21551197.2014.1002656
Narayana, P. A., Herrera, J. J., Bockhorst, K. H., Esparza-Coss, E., Xia, Y., Steinberg, J. L., et al. (2014). Chronic cocaine administration causes extensive white matter damage in brain: diffusion tensor imaging and immunohistochemistry studies. Psychiatry Res. Neuroimaging 221, 220–230. doi: 10.1016/j.pscychresns.2014.01.005
National Heart Lung Blood Institute. (2019). Study quality assessment tools. Available online at: https://www.nhlbi.nih.gov/health-topics/study-quality-assessment-tools
Otto, D. M., Ribeiro, M. de C., Barea, L. M., Mancopes, R., Almeida, S. T. de, Otto, D. M., et al. (2016). Association between neurological injury and the severity of oropharyngeal dysphagia after stroke. CoDAS 28, 724–729. doi: 10.1590/2317-1782/20162015139
Ouzzani, M., Hammady, H., Fedorowicz, Z., and Elmagarmid, A. (2016). Rayyan—a web and mobile app for systematic reviews. Syst. Rev. 5:210. doi: 10.1186/s13643-016-0384-4
Pearson, W. G., Taylor, B. K., Blair, J., and Martin-Harris, B. (2016). Computational analysis of swallowing mechanics underlying impaired epiglottic inversion. Laryngoscope 126, 1854–1858. doi: 10.1002/lary.25788
Penfield, W. (1955). The twenty-ninth maudsley lecture: the role of the temporal cortex in certain psychical phenomena. J. Mental Sci. 101, 451–465. doi: 10.1192/bjp.101.424.451
Penfield, W., and Welch, K. (1949). Instability of response to stimulation of the sensorimotor cortex of man. J. Physiol. 109, 358–365. doi: 10.1113/jphysiol.1949.sp004399
Puig, J., Pedraza, S., Blasco, G., Daunis-i-Estadella, J., Prados, F., Remollo, S., et al. (2011). Acute damage to the posterior limb of the internal capsule on diffusion tensor tractography as an early imaging predictor of motor outcome after stroke. Am. J. Neuroradiol. 32, 857–863. doi: 10.3174/ajnr.A2400
Reber, E., Gomes, F., Dähn, I. A., Vasiloglou, M. F., and Stanga, Z. (2019). Management of dehydration in patients suffering swallowing difficulties. J. Clin. Med. 8:1923. doi: 10.3390/jcm8111923
Robbins, J., Levine, R. L., Maser, A., Rosenbek, J. C., and Kempster, G. B. (1993). Swallowing after unilateral stroke of the cerebral cortex. Arch. Phys. Med. Rehabil. 74, 1295–1300. doi: 10.1016/0003-9993(93)90082-L
Rofes, L., Muriana, D., Palomeras, E., Vilardell, N., Palomera, E., Alvarez-Berdugo, D., et al. (2018). Prevalence, risk factors and complications of oropharyngeal dysphagia in stroke patients: A cohort study. Neurogastroenterol Motil. 30:e13338. doi: 10.1111/nmo.13338
Rosenbek, J. C., Robbins, J. A., Roecker, E. B., Coyle, J. L., and Wood, J. L. (1996). A penetration-aspiration scale. Dysphagia 11, 93–98. doi: 10.1007/BF00417897
Rovira, A., Griv,é, E., Rovira, A., and Alvarez-Sabin, J. (2005). Distribution territories and causative mechanisms of ischemic stroke. Eur. Radiol. 15, 416–426. doi: 10.1007/s00330-004-2633-5
Sampaio-Baptista, C., and Johansen-Berg, H. (2017). White matter plasticity in the adult brain. Neuron 96, 1239–1251. doi: 10.1016/j.neuron.2017.11.026
Satow, T., Ikeda, A., Yamamoto, J., Begum, T., Thuy, D. H. D., Matsuhashi, M., et al. (2004). Role of primary sensorimotor cortex and supplementary motor area in volitional swallowing: a movement-related cortical potential study. Am. J. Physiol. Gastro. Liver Physiol. 287, G459–G470. doi: 10.1152/ajpgi.00323.2003
Schlaug, G., Marchina, S., and Norton, A. (2009). Evidence for plasticity in white matter tracts of chronic aphasic patients undergoing intense intonation-based speech therapy. Ann. N. Y. Acad. Sci. 1169, 385–394. doi: 10.1111/j.1749-6632.2009.04587.x
Schmahmann, J. D., Pandya, D. N., Wang, R., Dai, G., D'Arceuil, H. E., de Crespigny, A. J., et al. (2007). Association fibre pathways of the brain: parallel observations from diffusion spectrum imaging and autoradiography. Brain 130, 630–653. doi: 10.1093/brain/awl359
Schmahmann, J. D., Smith, E. E., Eichler, F. S., and Filley, C. M. (2008). Cerebral white matter. Ann. N. Y. Acad. Sci. 1142, 266–309. doi: 10.1196/annals.1444.017
Schulz, R., Zimerman, M., Timmermann, J. E., Wessel, M. J., Gerloff, C., and Hummel, F. C. (2014). White matter integrity of motor connections related to training gains in healthy aging. Neurobiol. Aging 35, 1404–1411. doi: 10.1016/j.neurobiolaging.2013.11.024
Sheppard, J. J., Hochman, R., and Baer, C. (2014). The dysphagia disorder survey: validation of an assessment for swallowing and feeding function in developmental disability. Res. Dev. Disabil. 35, 929–942. doi: 10.1016/j.ridd.2014.02.017
Sims, J. R., Gharai, L. R., Schaefer, P. W., Vangel, M., Rosenthal, E. S., Lev, M. H., et al. (2009). ABC/2 for rapid clinical estimate of infarct, perfusion, and mismatch volumes. Neurology 72, 2104–2110. doi: 10.1212/WNL.0b013e3181aa5329
Sporns, O., Tononi, G., and Kötter, R. (2005). The human connectome: a structural description of the human brain. PLoS Comput. Biol 1, 0245–0251. doi: 10.1371/journal.pcbi.0010042
Study Quality Assessment Tools | NHLBI, NIH. (2019). Available online at: https://www.nhlbi.nih.gov/health-topics/study-quality-assessment-tools (accessed July 31, 2020).
Sumi, T. (1972). Reticular ascending activation of frontal cortical neurons in rabbits, with special reference to the regulation of deglutition. Brain Res. 46, 43–54. doi: 10.1016/0006-8993(72)90004-2
Suntrup, S., Kemmling, A., Warnecke, T., Hamacher, C., Oelenberg, S., Niederstadt, T., et al. (2015). The impact of lesion location on dysphagia incidence, pattern and complications in acute stroke. Part 1: dysphagia incidence, severity and aspiration. Eur. J. Neurol. 22, 832–838. doi: 10.1111/ene.12670
Suzuki, M., Asada, Y., Ito, J., Hayashi, K., Inoue, H., and Kitano, H. (2003). Activation of cerebellum and basal ganglia on volitional swallowing detected by functional magnetic resonance imaging. Dysphagia 18, 71–77. doi: 10.1007/s00455-002-0088-x
Tassorelli, C., Bergamaschi, R., Buscone, S., Bartolo, M., Furnari, A., Crivelli, P., et al. (2008). Dysphagia in multiple sclerosis: from pathogenesis to diagnosis. Neurol. Sci. 29, 360–363. doi: 10.1007/s10072-008-1044-9
Toogood, J. A., Barr, A. M., Stevens, T. K., Gati, J. S., Menon, R. S., and Martin, R. E. (2005). Discrete functional contributions of cerebral cortical foci in voluntary swallowing: a functional magnetic resonance imaging (fMRI) “Go, No-Go” study. Exp. Brain Res. 161, 81–90. doi: 10.1007/s00221-004-2048-1
Trivedi, R., Gupta, R. K., Shah, V., Tripathi, M., Rathore, R. K. S., Kumar, M., et al. (2008). Treatment-induced plasticity in cerebral palsy: a diffusion tensor imaging study. Pediatr. Neurol. 39, 341–349. doi: 10.1016/j.pediatrneurol.2008.07.012
Vos, S. B., Aksoy, M., Han, Z., Holdsworth, S. J., Maclaren, J., Viergever, M. A., et al. (2016). Trade-off between angular and spatial resolutions in in vivo fiber tractography. Neuroimage 129, 117–132. doi: 10.1016/j.neuroimage.2016.01.011
Wahlund, L. O., Barkhof, F., Fazekas, F., Bronge, L., Augustin, M., Sjögren, M., et al. (2001). A new rating scale for age-related white matter changes applicable to MRI and CT. Stroke 32, 1318–1322. doi: 10.1161/01.STR.32.6.1318
Wan, P., Chen, X., Zhu, L., Xu, S., Huang, L., Li, X., et al. (2016). Dysphagia post subcortical and supratentorial stroke. J. Stroke Cerebrovasc. Dis. 25, 74–82. doi: 10.1016/j.jstrokecerebrovasdis.2015.08.037
Wang, Z.-M., Shan, Y., Zhang, M., Wei, P.-H., Li, Q.-G., Yin, Y.-Y., et al. (2019). Projections of brodmann area 6 to the pyramidal tract in humans: quantifications using high angular resolution data. Front. Neural Circ. 13:62. doi: 10.3389/fncir.2019.00062
Wardlaw, J. M., Valdés Hernández, M. C., and Muñoz-Maniega, S. (2015). What are white matter hyperintensities made of? J. Am. Heart Assoc. 4:e001140. doi: 10.1161/JAHA.114.001140
Wilmskoetter, J., Bonilha, L., Martin-Harris, B., Elm, J. J., Horn, J., and Bonilha, H. S. (2019). Mapping acute lesion locations to physiological swallow impairments after stroke. Neuroimage Clin. 22:101685. doi: 10.1016/j.nicl.2019.101685
Wilmskoetter, J., Martin-Harris, B., Pearson, W. G., Bonilha, L., Elm, J. J., Horn, J., et al. (2018). Differences in swallow physiology in patients with left and right hemispheric strokes. Physiol. Behav. 194, 144–152. doi: 10.1016/j.physbeh.2018.05.010
Keywords: white matter, swallowing, dysphagia, deglutition, neurophysiology, diffusion weighted imaging, diffusion tensor MRI
Citation: Alvar A, Hahn Arkenberg R, McGowan B, Cheng H and Malandraki GA (2021) The Role of White Matter in the Neural Control of Swallowing: A Systematic Review. Front. Hum. Neurosci. 15:628424. doi: 10.3389/fnhum.2021.628424
Received: 17 November 2020; Accepted: 26 March 2021;
Published: 28 June 2021.
Edited by:
Teresa Lever, University of Missouri, United StatesReviewed by:
Pere Clavé, Hospital de Mataro, SpainEmilia Michou, University of Patras, Greece
Antonio Schindler, University of Milan, Italy
Copyright © 2021 Alvar, Hahn Arkenberg, McGowan, Cheng and Malandraki. This is an open-access article distributed under the terms of the Creative Commons Attribution License (CC BY). The use, distribution or reproduction in other forums is permitted, provided the original author(s) and the copyright owner(s) are credited and that the original publication in this journal is cited, in accordance with accepted academic practice. No use, distribution or reproduction is permitted which does not comply with these terms.
*Correspondence: Georgia A. Malandraki, bWFsYW5kcmFraUBwdXJkdWUuZWR1
†These authors have contributed equally to this work