Effect of music intervention on subjective scores, heart rate variability, and prefrontal hemodynamics in patients with chronic pain
- 1Institute of Rehabilitation Engineering and Technology, University of Shanghai for Science and Technology, Shanghai, China
- 2Department of Rehabilitation Medicine, Changhai Hospital, Naval Medical University, Shanghai, China
Introduction: Music interventions have been proposed in recent years as a treatment for chronic pain. However, the mechanisms by which music relieves pain are unclear, and the effects of music intervention on physiological indicators in patients with chronic pain remain to be explored. This study aimed to explore whether a music intervention would have effects on subjective pain ratings, heart rate variability, and functional connectivity of the cerebral cortex in patients with chronic pain.
Methods: A randomized controlled study was conducted on 37 pain patients aged 18–65 years, with the control group receiving usual care, and the intervention group receiving music intervention (8–150 Hz, 50–70 dB) for 30 min before bedtime for 7 days on top of usual care. Pain visual analog scale and heart rate variability were used as subjective and objective physiological indices before and after the music intervention, respectively. Changes in oxyhemoglobin and deoxyhemoglobin concentrations in the cerebral cortex were measured by functional near-infrared spectroscopy, and whole-brain correlation analysis was used to quantify the connectivity of prefrontal brain regions associated with the pain response.
Results: Results showed that patients with chronic pain in the intervention group had significantly lower visual assessment scale scores, as well as significantly lower overall voluntary mobility during pain episodes, resulting in relatively higher vagal innervation compared to the control group. In addition, connections between the bilateral dorsolateral prefrontal cortex (BA9, BA46) and frontal areas (BA10) were significantly higher in the intervention group.
Discussion: This study demonstrates the effectiveness of the combined application of music interventions with usual care in reducing pain levels in patients with chronic pain and provides insight into the pathological mechanisms of music interventions for analgesia, providing direction for new baseline indicators for quantitative clinical assessment of pain. The study was registered in the Chinese Clinical Trial Registry (No. ChiCTR2100052993).
Clinical trial registration: [https://www.chictr.org.cn/showproj.aspx?proj=136268], identifier [ChiCTR2100052993].
Introduction
Episodes of chronic pain are generally considered particularly distressing, and chronic pain without respite can even cause depression and anxiety that interfere with the patient’s normal life. Over the past 20 years, opioids have been the primary treatment choice for chronic pain, but with poor long-term outcomes and the potential for opioid dependence (Manhapra, 2022). The high prevalence, burden, and refractoriness of chronic pain and the opioid dependence crisis have drawn the attention of scholars to complementary or alternative therapies (EBioMedicine, 2016).
Among various non-pharmacological interventions, music interventions are widely used for chronic pain relief due to their advantages such as inexpensive, non-discriminatory, and non-adverse effects (Sihvonen et al., 2017). A growing body of evidence supports the use of music interventions for pain management (Martin-Saavedra et al., 2018; Howlin and Rooney, 2021; Hsu et al., 2022). Hsu et al. (2022) implemented a music intervention in 66 elderly patients with osteoarthritis, and the visual analog scales (VAS) scores were significantly lower in the intervention group than in the control group. Some researchers have found that music intervention may reduce pain levels in patients with chronic pain, and have significant positive effects on physical comfort and relaxation (Howlin and Rooney, 2021; Ting et al., 2022).
The clinical use of music interventions in pain medicine is promising. Psychology argues that music interventions can improve motivation, elevate mood, and relieve pain by intentionally distracting people from unpleasant sensations and reducing the perception of pain or anxiety (Kristjánsdóttir and Kristjánsdóttir, 2011). On the other hand, neural mechanism theories suggest that the melody and rhythm of music influence autonomic nervous system regulation and pain perception by influencing the limbic system and hypothalamus to reduce the secretion of catecholamines (Loewy, 2022). Musical melodies of 8–13 Hz stimulate alpha brain waves to relax consciousness (Han et al., 2022). Also, music interventions promote high endorphin secretion and lower blood pressure, heart rate, respiratory rate, oxygen consumption, and plasma lactate levels, thus contributing to pain relief (Wang et al., 2020c). A recent study (Zhou et al., 2022) revealed the mechanism by which music induces analgesia through corticothalamic circuits through experiments on mice. The mechanisms by which music interventions are effective for chronic pain are still being explored.
Exploring the effectiveness of chronic pain interventions through changes in objective physiological indicators has gradually become a hot topic in pain assessment (Ling et al., 2014; Jess et al., 2016; Boissoneault et al., 2019). In clinical practice, patients with chronic pain often exhibit signs of the autonomic nervous system, such as fatigue, sleep disturbances, and decreased appetite, which affect pain VAS (Wang et al., 2020a) and the level of heart rate variability (HRV) (Forte et al., 2022). It was found that during fibromyalgia episodes, the low-frequency (sympathetic) power spectrum increases, and the high-frequency (vagus) decreases, with a corresponding increase in pain VAS (Reneau, 2020). Functional neuroimaging studies have shown pain-related activation in several cortical areas during chronic pain, including the prefrontal, cingulate, insula, and primary and secondary somatosensory cortices (Morton et al., 2016). The Prefrontal is primarily responsible for the perception of and response to pain (Wang et al., 2020b). Changes in prefrontal cortex blood flow concentration are associated with chronic pain episodes captured by functional near-infrared spectroscopy (fNIRS) (Öztürk et al., 2021). Furthermore, it was observed that the functional connectivity in the prefrontal cortex decreases during pain episodes, while network connectivity increases after the local intervention, which was associated with individual pain relief (Kim et al., 2020). Functional connectivity between the medial prefrontal cortex and frontopolar cortex has also been shown to be improved in patients with trigeminal neuralgia (Tsai et al., 2018). However, few studies have explored the effectiveness of chronic pain interventions through a combination of VAS, heart rate variability, and brain network functional connectivity.
To investigate the effectiveness of music intervention for chronic pain, we compared the changes in VAS, heart rate (HR), and HRV of patients before and after music intervention. We are also interested in the impact of music intervention on the functional connectivity of the prefrontal cortex when assessing pain in patients suffering from chronic pain. We expect the music intervention to promote better functional connectivity between the dorsolateral prefrontal cortex and frontopolar area during pain assessment in patients with chronic pain, while decreasing pain scores and autonomous activity in pain patients.
Methods
The study was registered before patient recruitment and was conducted by the Department of Pain, Huadong Hospital Affiliated to Fudan University, Shanghai, China, from December 15, 2021, to February 28, 2022. This study has completed ethical approval (2021K104-F221) and Chinese clinical trial registration (ChiCTR2100052993). After receiving verbal and written study information, an approved consent form was signed by the patient in case of study participation.
Participants
Eligible participants ranged in age from 18 to 65 years with chronic pain. Additionally, cognitive impairments, antipsychotic or antidepressant drugs in the past three months, insensitivity to the audio used in this experiment, being in psychotherapy, and other serious illnesses were identified as exclusion criteria.
Sample size estimation was based on the effect and standard variation found in the systematic review (Hsu et al., 2022), and was conducted with G*power 3.1 (Faul et al., 2009) setting α = 0.05 and β = 0.20. It was found that 17 participants were needed in each group. We included 19 patients in each group considering attrition.
Participants were randomly assigned to the following two groups in a 1:1 allocation ratio. An independent statistician randomized blocks of random sizes, hiding the task from clinicians and patients.
Procedures
Experimental paradigm
A pretest-posttest design was used, with one music intervention group and one control group. A repeated-measures design was designed, in which each patient’s pain VAS and electrocardiogram (ECG) recordings were measured before and after the intervention. The measurements required participants to refrain from alcohol for 24 h and caffeinated beverages and nicotine for 4 h prior to this examination, as these substances have known effects on nociception and heart rate variability.
In addition, we immediately monitored the cerebral hemodynamics of the patients through fNIRS after the intervention, and the patients in the intervention group always wore headphones to listen to music.
Music intervention
Music with a frequency of 8–13 Hz can stimulate alpha brainwaves to aid mental relaxation (Huang et al., 2016). Low-frequency somatic vibration music with frequencies of 16–150 Hz can promote sleep and stabilize mood (Kühlmann et al., 2018). Considering that 60–85 dB is the optimal sound threshold range for the human ear (White, 2000), the researchers suggested that music interventions at a volume of 55–70 dB (Standley, 2002), 10 dB higher than ambient noise, would be appropriate.
The participants in the control group underwent 7 days of usual care (appropriate exercise directed by a clinician), while participants in the intervention group listened to specific music (8–150 Hz, 50–70 dB) for 30 min each day before bedtime for 7 days on top of their usual care.
Measurements
Pain visual analog scale
The Pain VAS is a pain intensity assessment tool that changes from a score of 1 to 10 to indicate pain levels ranging from pain-free to extreme pain.
Electrocardiogram acquisition
Participants were asked to sit upright in a chair and keep their bodies stable for 5 min of ECG signal acquisition, and to maintain natural respiration to avoid motion artifacts. In this study, the Power-Lab/16sp system (Castle Hill, AD Instruments, Australia) was used to acquire and amplify ECG signals at a sampling rate of 1 kHz. The ECG signals were then filtered through a 45 Hz low-pass filter and a 1 Hz high-pass filter. The RR interval sequence of the ECG signals was calculated, and the time-domain parameters [Heart rate (HR), SDNN, RMSSD, and pNN50], frequency domain parameters (normalized low-frequency power HFn, normalized high-frequency power LFn, and the ratio of low-frequency power to high-frequency power LF/HF), and Poincaré trace parameters (SD1 and SD2) were calculated to assess the individual autonomous function.
Functional near-infrared spectroscopy measurement
Manual palpation, a common method of clinical pain phenotype identification, was used as a pain stimulus measure in the fNIRS measurement of cortical functional connectivity in the study. The purpose of this manipulation is to simulate the patient’s state when he or she is in the most pain. The clinician induced referred pain with the thumb and maintained a pressure of 3–4 kg/cm2 for 30 s (Tong et al., 2022), with 1 min interval each time, for a total of 3 times to reduce measurement error. During fNIRS monitoring, patients were instructed to sit in a relaxed position with their eyes closed throughout the procedure. The measurements began with a 1-min baseline calibration period, followed by three cycles of pain stimulation, as shown in Figure 1.
We used the Brite24 Continuous Wave system fNIRS (Artinis, Netherlands) with 18 optodes (10 light sources, 8 detectors, 10 Hz sampling rate). Two different wavelengths (752 nm and 841 nm) of NIR light were used to detect changes in oxyhemoglobin (HbO) and deoxyhemoglobin (HbR) concentrations. 18 optodes were positioned to cover the frontal cortex (30 mm distance between probes), making a total of 18 channels as shown in Figure 2A. The center of the row of the middle probe of probes was positioned approximately at FPz according to the international 10/20 system. The topographical distribution of the fNIRS channels was visualized on a standard human cortical surface using the NIRS_SPM software (Figure 2B). The channel was registered with the corresponding cortical region in the MNI space underneath and transferred to the corresponding cortical projection point on the ICBM-152 brain model (Lancaster et al., 2007). Thus, the regions of interest (ROIs) were CH5, CH8, CH9, CH10, CH18 (BA10), CH6, CH12, CH17 (BA9), CH7, CH11 (BA46), CH3, CH15 (BA6).
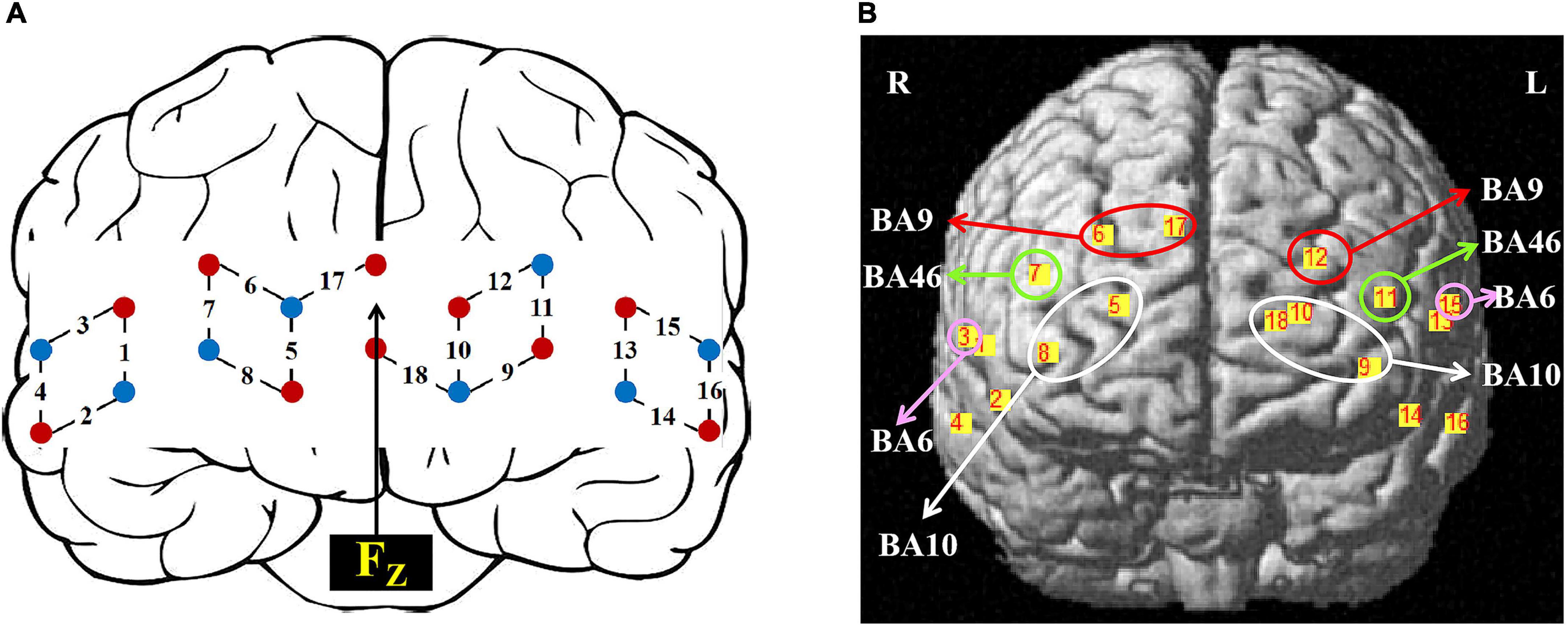
Figure 2. Setup of fNIRS probes and channels. (A) Schematic illustration of the fNIRS probe layout. (B) The topographical distribution of the fNIRS recording channels.
The fNIRS indicators include HbO, HbR, and total oxygenated concentrations, where increased HbO concentration and decreased HbR concentration are often used as indicators of activation of cortical production. In the present study, HbO and HbR were used as analytical indicators. HbO and HbR data were pre-processed in the Matlab-based NIRS_KIT toolbox, comprising a polynomial regression model for detrending, robust regression-based inverse time distribution repair for motion correction (to remove spike artifacts and baseline drift), and an infinite pulse response filter to eliminate mechanical and physiological noise. To minimize interferences such as scalp blood flow, we also used the short-distance reference channel noise regression from the toolkit. The HbO and HbR data were then analyzed using generalized linear models (GLM) to obtain statistically significant and concentration-dependent scaling factors β, reflecting the extent to which each channel is activated.
Statistics
In this study, data from VAS, HR, and HRV before and after the music intervention were statistically analyzed using the SPSS26 software. The values were expressed as mean ± standard deviation. The Shapiro-Wilk test was used to test whether the experimentally obtained data satisfied a normal distribution. Qualitative data, such as gender, were analyzed using the χ2 test. Quantitative data that satisfied normal distribution, such as VAS, HR, and the indicators in HRV, were analyzed using a two-way ANOVA.
In addition, the Shapiro-Wilk test was used to check the normality of the fNIRS data. The comparison of β values in each ROI was performed with an independent samples t-test. For the connectivity analysis, we averaged the individual HbO and HbR concentration changes in the subjects’ ROIs. After multiple comparisons, we obtained four sets of 8 × 8 matrices as well as four brain connectivity maps. The test statistic and its corresponding P were given, with differences considered statistically significant at P < 0.05.
Results
We recruited 38 people to participate in this study, and one person was eliminated due to insufficient fNIRS data quality (intervention: n = 19; control: n = 18) (Figure 3). The differences in general information between the two groups were not statistically significant (P < 0.05), as shown in Table 1.
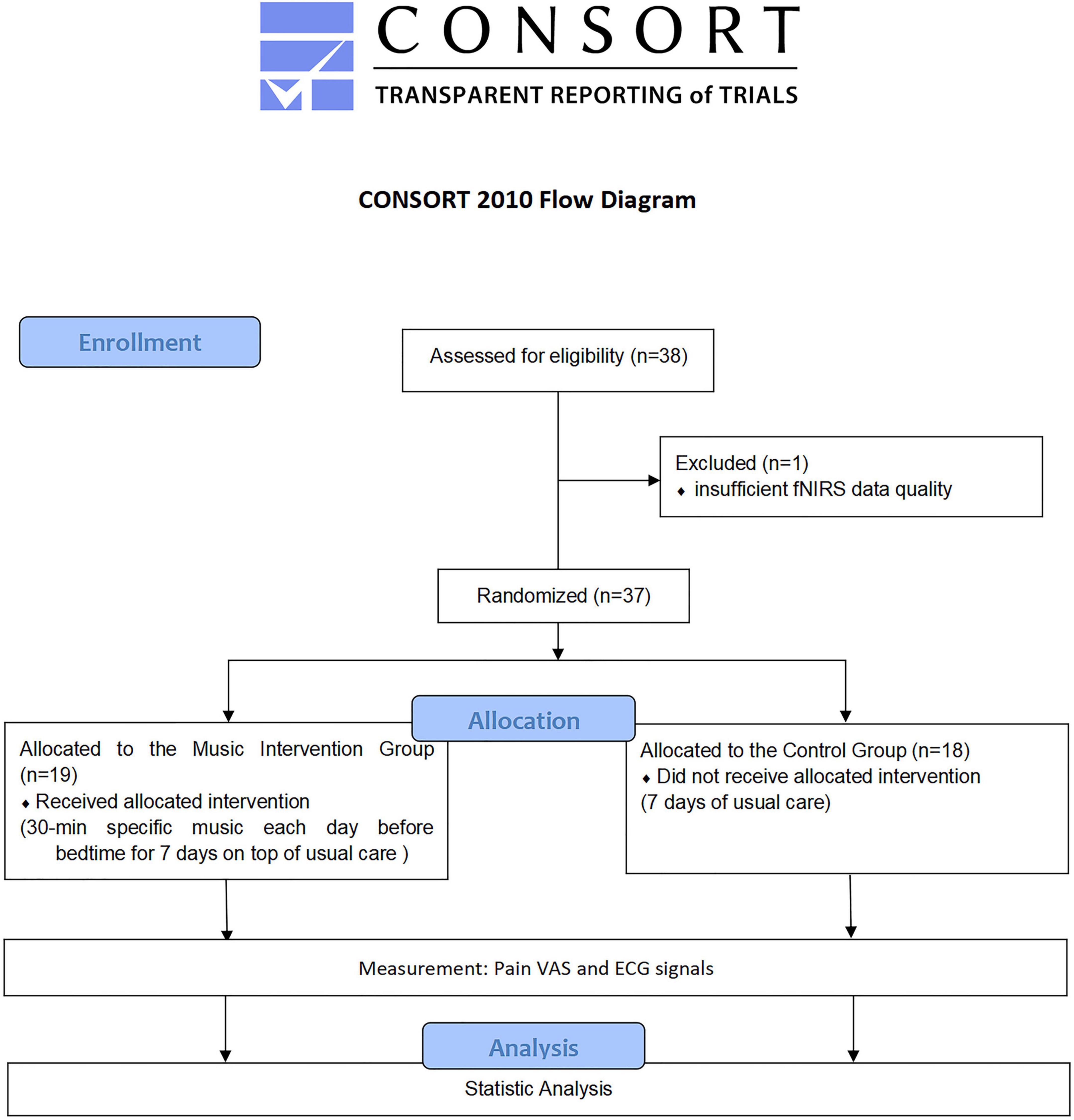
Figure 3. Flow chart (Boutron et al., 2017).
Comparison of visual analog scale scores
As shown in Table 2, the time-by-group interaction effects were more significant (P < 0.01). Further simple effects test analysis yielded that in the intervention group, the VAS values were significantly higher in the pre-test than in the post-test (F = 31.50, P < 0.001, η2 = 0.71). In the control group, the VAS values of the pre-test and post-test were not significant (F = 1.00, P = 0.34, η2 = 0.07).
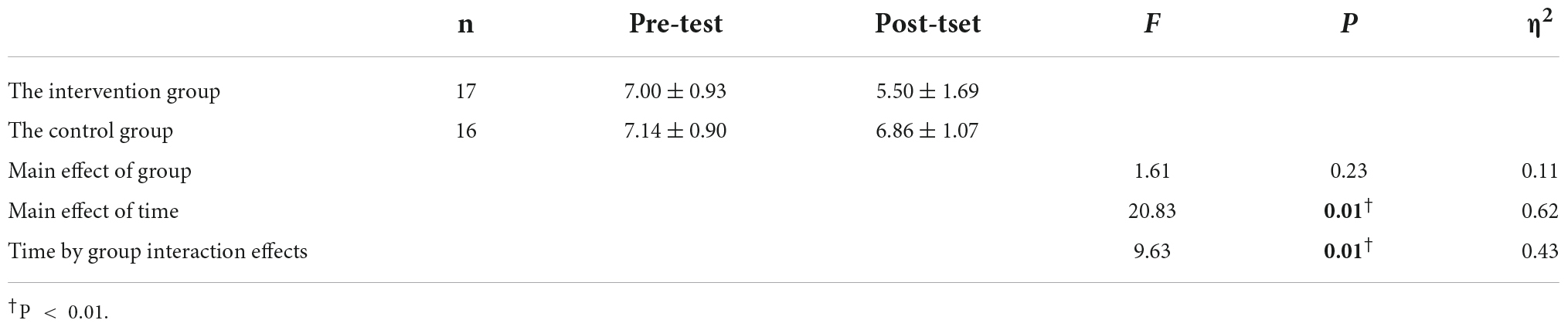
Table 2. Results of the analysis of the VAS of the two groups of patients in the pre-test and post-test.
Comparison of heart rate
The HR of the participants was derived based on ECG signals. As shown in Table 3, the time-by-group interaction effects were significant (P < 0.05). After simple effects tests, the HR was significantly higher in the pre-test than in the post-test in the intervention group (F = 22.39, P < 0.001, η2 = 0.67). In the control group, HR was not significant in the pre-test versus post-test (F = 1.14, P = 0.31, η2 = 0.09).
Comparison of heart rate variability
Table 4 represents the results of the two-way ANOVA performed on HRV indicators. Due to significant time-by-group interaction effects (P < 0.05), further simple effects test analysis yielded that in the control group, the time-domain parameters (SDNN, RMSSD, pNN50), part of the frequency domain indicators (LFn, LF/HF) and the nonlinear indicator SD2 was significantly lower in the pre-test than in the post-test (F = 20.27, P < 0.05, η2 = 0.65; F = 20.27, P < 0.05, η2 = 0.65; F = 6.48, P < 0.05, η2 = 0.37; F = 11.97, P < 0.05, η2 = 0.52; F = 10.33, P < 0.05, η2 = 0.48; F = 18.39, P < 0.01, η2 = 0.63), and the frequency domain index HFn of the pre-test was significantly higher than that of the post-test (F = 13.63, P < 0.05, η2 = 0.55). In the intervention group, the nonlinear indicator SD1 was significantly lower in the pre-test than in the post-test (F = 21.69, P < 0.001, η2 = 0.66). The results demonstrate the effectiveness of the music intervention in relieving pain and reducing voluntary activity.
Comparison of prefrontal connectivity based on changes in oxyhemoglobin/deoxyhemoglobin concentration
The activation intensity was calculated separately based on the activation level of the channels in the task, with higher β values indicating stronger activation. Table 5 shows the comparison of the activation intensity of each ROI for all subjects in the intervention and control groups, and the activation intensity in the intervention group was significantly lower than that in the control group.
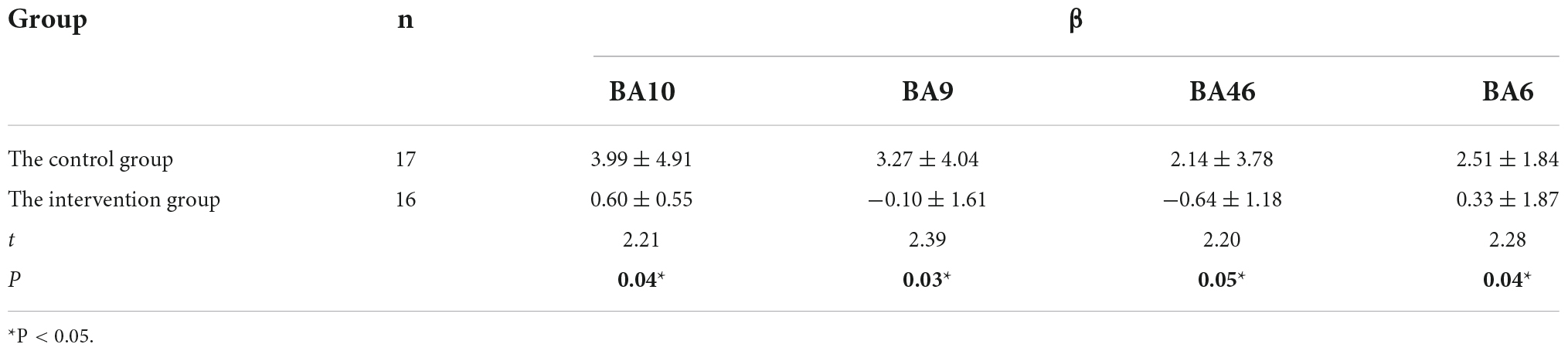
Table 5. Comparison of the activation intensity of ROI brain regions between two groups of patients.
To better analyze the correlation between the ROIs and brain connectivity analysis, we divided the region of interest into two blocks and continued the Pearson analysis based on the experimentally measured HbO and HbR concentrations, and the results are shown in Figure 4 and Tables 6–9.
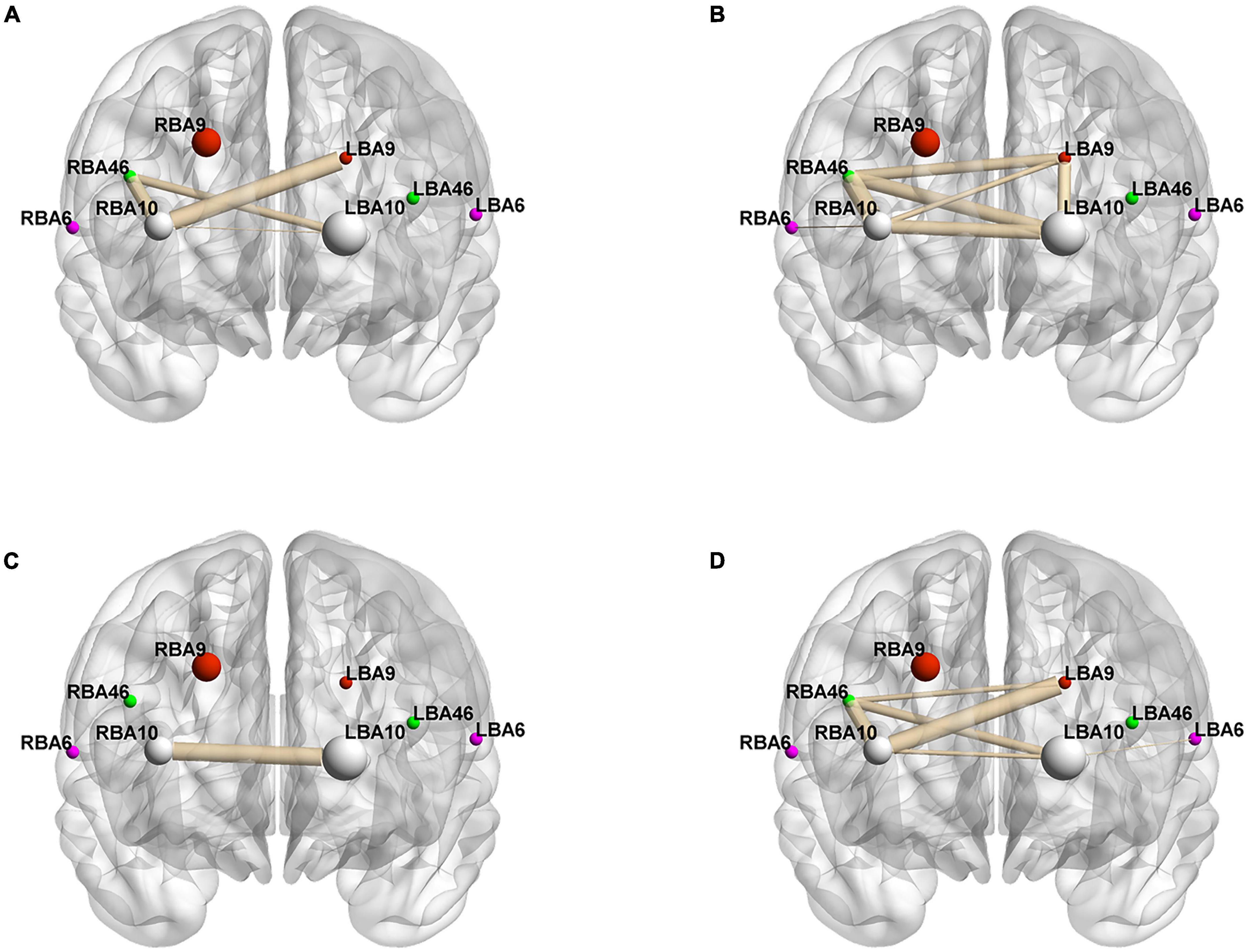
Figure 4. Whole-brain connectivity of ROIs in the prefrontal lobe. (A,B) Portray whole-brain connectivity based on changes in HbO concentration in the control and intervention groups, respectively, and (C,D) portray whole-brain connectivity based on changes in HbR concentration in the control and intervention groups, respectively. Where the round spheres represent each of the 8 ROIs, the beige line segments indicate the correlation between the ROIs, and the thickness indicates the degree of correlation. To make the images clearer, only significant correlations are shown in the figure (P < 0.05).
Figures 4A,B, and Tables 6–9 present the connectivity of prefrontal ROIs as a function of HbO concentration change. Figure 4A and Table 6 indicate significant correlations in RBA10-LBA9, RBA10-RBA46, and RBA46-LBA10 during pain in the control group (P < 0.05). Compared to the control group, the intervention group had significantly increased complexity of brain region connectivity, with significant correlations in LBA9-RBA46, LBA9-RBA10, and LBA9-LBA10 (P < 0.05) and elevated correlations in RBA10-RBA46, RBA10-LBA10 and RBA46-LBA10 (P < 0.01), as shown in Figure 4B and shown in Table 7.
Figures 4C,D, and Tables 8, 9 display prefrontal ROI connectivity according to HbR concentration change. As shown in Figure 4C and Table 8, there was a significant LBA10-RBA10 correlation in the control group during pain (P < 0.05). In the intervention group, significant correlations (P < 0.05) were observed in RBA10-RBA46, RBA10-LBA10, RBA46-LBA9, RBA46-LBA10, and highly significant correlations (P < 0.01) in RBA10-LBA9, as shown in Figure 4D and Table 9.
Discussion
Previous studies have shown that music intervention induces rather strong vagal modulation by activating the mesencephalic and hippocampal systems of the central nervous system, promoting the secretion of endogenous opioids in the body, reducing patients’ subjective nociception, and stabilizing patients’ vital signs such as blood pressure and HR (Guerrier et al., 2021). Furthermore, music-induced analgesia is associated with the modulation of neural down-regulation of pain in vivo, where the prefrontal cortex is involved in extremely complex higher cognitive processes related to the perception and processing of pain and music (Koelsch et al., 2022). In this study, we compared changes in pain VAS scores, HRV, and cerebrocortical hemodynamics in patients with chronic pain following a music intervention.
In this study, VAS was used to assess patients’ subjective pain perception. As shown in Table 2, the VAS scores were lower in the intervention group, indicating that the music intervention was able to significantly reduce the patients’ subjective pain levels, providing some evidence of the positive effect of music intervention on chronic pain. This is related to the contribution of the limbic dopamine system of the brain to the affective component of pain perception (Sihvonen et al., 2022).
The HR is regulated by several reflex pathways in the brainstem and autonomic cardiac nodes, while the latter is influenced by forebrain cortical structures such as the hypothalamus, amygdala, insular cortex, and orbitofrontal cortex (Esler, 2016). Studies have shown that music can induce affective and emotional regulation through structural activity in the anteriorbrain, thereby reducing HR (Taruffi, 2021). As shown in Table 3, the HR in the intervention group was significantly lower than in the control group, indicating a significant sedative effect of the music intervention on HR. This is consistent with previous studies confirming the effectiveness of music intervention in reducing patients’ pain levels.
Heart rate variability (HRV) analysis is currently a better non-invasive tool to investigate autonomic function, reflecting the regulation of heart rhythm by the central and autonomic nervous systems. Moreover, HRV allows an objective evaluation of sympathetic and vagal nerve activity (Winbo et al., 2020). In the results of the time-domain analysis, SDNN mainly reflects the change in overall HRV activity, which is the slowly changing component of HRV. RMSSD and pNN50 primarily reflect vagal nerve activity, which reflects the rapidly evolving component of HRV. As shown in Table 4, the time-domain indices SDNN, RMSSD, and pNN50 were significantly higher in the control group during the pain period in the post-test than in the pre-test, indicating that pain stimulation led to an increase in overall autonomic and vagal activity. In addition, the post-test of time-domain parameters was lower in the intervention group than the pre-test, demonstrating that the music intervention was able to suppress the overall autonomic activity triggered by the pain stimulus, while the vagus nerve played a dominant regulatory role in the music intervention.
On the results of frequency-domain analysis, HFn reflects the functioning level of the vagus nerve, and LFn shows the regulation of the sympathetic nerve with the change in total efficiency. LF/HF reflects the balance of sympathetic and vagus nerves, with an increase, indicating active sympathetic nerves, and a decrease indicating that sympathetic and vagus nerves are imbalanced. In this study, both LFn and LF/HF were significantly increased and HFn was significantly decreased in the control group compared to the pre-test. The findings suggested that overall autonomic activity increases in response to painful stimuli, with the sympathetic nerves acting as the primary regulator. However, the music intervention inhibited the tendency, and allowed the vagus nerve to occupy an active state.
The HRV nonlinear analysis allows quantitative reflection of the structure and complexity of the RR time interval. SD1 indicates the activity of the vagus nerve. SD1 was significantly higher in the pre-test than in the post-test in the intervention group, reflecting a greater effect of the music intervention on vagal activity. SD2 reflects the change in the overall autonomic function. SD2 in the control group was significantly lower in the pre-test than in the post-test, reflecting the inhibitory effect of the music intervention on overall autonomic activity.
Previous studies have found that pain not only activates an area of the brain, but induces synchronized or coordinated areas of activity across multiple brain regions, most of which involve unilateral or bilateral BA6, BA9, BA10, and BA46 (Pascalis et al., 2015; Shi et al., 2022). It was found that the music intervention breaks the original brain connectivity network and weakens or even inactivates the connections between relevant areas of the brain, slowing the perception of pain. The prefrontal ROIs connections in the control group were lower and mostly transcerebral connections as shown in Figure 4 and Tables 5–8, consistent with the finding that pain activates the bilateral brain regions (Song et al., 2016). Brain connectivity based on HbO and HbR levels increased significantly, indicating that music intervention increased the functional coupling of pain perception and processing in the cerebral cortex (Lunde et al., 2019). Significantly enhanced connectivity of the bilateral dorsolateral prefrontal cortex and frontopolar regions represented by BA9, BA46, and BA10 would further improve the upward processing of perceptual information in patients. With the music intervention, we have seen increased connectivity in the bilateral dorsolateral prefrontal cortex, which is involved in downward maintenance and regulation of pain detection, perception, and inhibition (Lunde et al., 2019). We also found that music intervention can positively modulate the functional connections between the frontopolar area and the dorsolateral prefrontal cortex, favoring the pituitary secretion of enkephalins (Crane, 2021), thus improving functional connections in neural pathways related to pain perception and treatment, pain-reliever in patients.
Previous studies on the analgesic effects of music interventions are usually unidimensional, and there is a lack of objective evidence to verify the multidimensional effects of music interventions on patients with pain, and thus the effectiveness of music interventions to relieve pain is debatable. This study investigated synchronous changes in subjective pain scores, heart rate variability, and prefrontal cerebral hemodynamics in patients with chronic pain during music intervention. We found that the music intervention used in this experiment significantly reduced patients’ pain VAS scores, relieved HR, and significantly suppressed overall autonomic activity by innervating vagal nerve activity. This confirms the view of previous studies on the effect of music interventions on pain relief (Lee, 2016). Furthermore, we combined the collected cerebral hemodynamic signals with validated data related to functional brain activity, and the music intervention reduced HbO concentrations in brain regions associated with pain processing and increased functional coupling of pain perception and processing in the cerebral cortex. Additionally, we found that music intervention not only resulted in a significant increase in connectivity between the bilateral dorsolateral prefrontal cortex and frontopolar areas, but also positively modulated functional connectivity between frontopolar areas and the dorsolateral prefrontal cortex. By the changes in pain perception, heart rate variability, and prefrontal cerebral hemodynamic parameters in the subjects after the music intervention, we further demonstrated the effectiveness of appropriate music intervention in helping to distract and relieve anxiety and thus pain to some extent. These findings in heart rate variability and functional networks provide a physiological basis for music interventions for analgesia, contribute to understanding the pathogenesis of pain, and provide new directions for quantitative clinical assessment of pain.
However, it is difficult to fully explore the effects of various musical factors (frequency or volume) on pain patients with a single intervention approach. In the follow-up study, we will add different types of music intervention groups and design a double-blind randomized controlled trial to recruit a larger sample size of patients with chronic pain as a clinical reference. Further, we will determine the optimal music for analgesic intervention based on musical characteristics, and explore the mechanism of music intervention in improving the response of pain in brain regions with multimodal brain imaging techniques such as EEG and fMRI.
Data availability statement
The raw data supporting the conclusions of this article will be made available by the authors, without undue reservation.
Ethics statement
The studies involving human participants were reviewed and approved by the Medical Ethics Committee of Huadong Hospital Affiliated to Fudan University. The patients/participants provided their written informed consent to participate in this study. Written informed consent was obtained from the individual(s) for the publication of any potentially identifiable images or data included in this article.
Author contributions
JD and PS designed and performed the experiments. JD analyzed the data and wrote the manuscript. PS, HY, and FF reviewed the manuscript. All authors approved the final manuscript.
Funding
This work was supported by the National Key R&D Program of China (2019YFC1711800).
Acknowledgments
We would like to thank Huadong Hospital Affiliated to Fudan University for helping in conducting this study. We would also like to thank the patients for participating in this study. In addition, we would like to especially thank our team members Jiayue Zhang and Yuhui Zhou for their hard work in this experiment.
Conflict of interest
The authors declare that the research was conducted in the absence of any commercial or financial relationships that could be construed as a potential conflict of interest.
Publisher’s note
All claims expressed in this article are solely those of the authors and do not necessarily represent those of their affiliated organizations, or those of the publisher, the editors and the reviewers. Any product that may be evaluated in this article, or claim that may be made by its manufacturer, is not guaranteed or endorsed by the publisher.
Abbreviations
VAS, visual analog scale; HRV, heart rate variability; fNIRS, functional near-infrared spectroscopy; HbO, oxyhemoglobin; HbR, deoxyhemoglobin; ECG, electrocardiogram; HR, heart rate.
References
Boissoneault, J., Letzen, J., Robinson, M., and Staud, R. (2019). Cerebral blood flow and heart rate variability predict fatigue severity in patients with chronic fatigue syndrome. Brain Imaging Behav. 13, 789–797. doi: 10.1007/s11682-018-9897-x
Boutron, I., Altman, D. G., Moher, D., Schulz, K. F., and Ravaud, P. (2017). CONSORT Statement for randomized trials of nonpharmacologic treatments: A 2017 update and a CONSORT Extension for nonpharmacologic trial abstracts. Ann. Intern. Med. 167, 40–47. doi: 10.7326/M17-0046
Crane, J. (2021). Rhythmic interventions, musical returns: The health benefits of music as an embodied, everyday practice. Health Commun. 36, 920–923. doi: 10.1080/10410236.2020.1723053
EBioMedicine (2016). Chronic pain: The need and hope for opioid alternatives. EBioMedicine 5:1. doi: 10.1016/j.ebiom.2016.03.011
Esler, M. (2016). The sympathetic regulation of the heart. Eur. Heart J. 37, 2808–2809. doi: 10.1093/eurheartj/ehw365
Faul, F., Erdfelder, E., Buchner, A., and Lang, A.-G. (2009). Statistical power analyses using G*Power 3.1: Tests for correlation and regression analyses. Behav. Res. Methods 41, 1149–1160. doi: 10.3758/BRM.41.4.1149
Forte, G., Troisi, G., Pazzaglia, M., Pascalis, V. D., and Casagrande, M. (2022). Heart rate variability and pain: A systematic review. Brain Sci. 12:153. doi: 10.3390/brainsci12020153
Guerrier, G., Bernabei, F., Lehmann, M., Pellegrini, M., Giannaccare, G., and Rothschild, P.-R. (2021). Efficacy of preoperative music intervention on pain and anxiety in patients undergoing cataract surgery. Front. Pharmacol. 12:748296. doi: 10.3389/fphar.2021.748296
Han, J., Chen, C., Zheng, S., Zhou, T., Hu, S., Yan, X., et al. (2022). Functional connectivity increases in response to high-definition transcranial direct current stimulation in patients with chronic disorder of consciousness. Brain Sci. 12:1095. doi: 10.3390/brainsci12081095
Howlin, C., and Rooney, B. (2021). Cognitive agency in music interventions: Increased perceived control of music predicts increased pain tolerance. Eur. J. Pain 25, 1712–1722. doi: 10.1002/ejp.1780
Hsu, H.-F., Chen, K.-M., and Belcastro, F. (2022). The effect of music interventions on chronic pain experienced by older adults: A systematic review. J. Nurs. Scholarsh. 54, 64–71. doi: 10.1111/jnu.12712
Huang, R., Wang, J., Wu, D., Long, H., Yang, X., Liu, H., et al. (2016). The effects of customised brainwave music on orofacial pain induced by orthodontic tooth movement. Oral. Dis. 22, 766–774. doi: 10.1111/odi.12542
Jess, G., Pogatzki-Zahn, E. M., Zahn, P. K., and Meyer-Frieem, C. H. (2016). Monitoring heart rate variability to assess experimentally induced pain using the analgesia nociception index: A randomised volunteer study. Eur. J. Anaesthesiol. 33, 118–125. doi: 10.1097/EJA.0000000000000304
Kim, S.-H., Lee, M.-W., Kang, M.-J., Lee, S. G., Lee, J.-G., and Mun, C.-W. (2020). Comparison analysis between the medication efficacy of the milnacipran and functional connectivity of neural networks in fibromyalgia patients. Brain Sci. 10:295. doi: 10.3390/brainsci10050295
Koelsch, S., Andrews-Hanna, J. R., and Skouras, S. (2022). Tormenting thoughts: The posterior cingulate sulcus of the default mode network regulates valence of thoughts and activity in the brain’s pain network during music listening. Hum. Brain Mapp. 43, 773–786. doi: 10.1002/hbm.25686
Kristjánsdóttir, Ó, and Kristjánsdóttir, G. (2011). Randomized clinical trial of musical distraction with and without headphones for adolescents’ immunization pain. Scand. J. Car. Sci. 25, 19–26. doi: 10.1111/j.1471-6712.2010.00784.x
Kühlmann, A. Y. R., de Rooij, A., Kroese, L. F., van Dijk, M., Hunink, M. G. M., and Jeekel, J. (2018). Meta-analysis evaluating music interventions for anxiety and pain in surgery. Br. J. Surg. 105, 773–783. doi: 10.1002/bjs.10853
Lancaster, J. L., Tordesillas-Gutiérrez, D., Martinez, M., Salinas, F., Evans, A., Zilles, K., et al. (2007). Bias between MNI and talairach coordinates analyzed using the ICBM-152 brain template. Hum. Brain Mapp. 28, 1194–1205. doi: 10.1002/hbm.20345
Lee, J. H. (2016). The Effects of music on pain: A meta-analysis. J. Music Ther. 53, 430–477. doi: 10.1093/jmt/thw012
Ling, P., Siyuan, Y., Wei, W., Quan, G., and Bo, G. (2014). Assessment of postoperative pain intensity by using photoplethysmography. J. Anesth. 28, 846–853. doi: 10.1007/s00540-014-1837-3
Loewy, J. (2022). Underlying music mechanisms influencing the neurology of pain: An integrative model. Brain Sci. 12:1317. doi: 10.3390/brainsci12101317
Lunde, S. J., Vuust, P., Garza-Villarreal, E. A., and Vase, L. (2019). Music-induced analgesia: How does music relieve pain? Pain 160, 989–993. doi: 10.1097/j.pain.0000000000001452
Manhapra, A. (2022). Complex persistent opioid dependence—an opioid-induced chronic pain syndrome. Curr. Treat. Opt. Oncol. 23, 921–935. doi: 10.1007/s11864-022-00985-x
Martin-Saavedra, J. S., Vergara-Mendez, L. D., and Talero-Gutiérrez, C. (2018). Music is an effective intervention for the management of pain: An umbrella review. Complement. Ther. Clin. Pract. 32, 103–114. doi: 10.1016/j.ctcp.2018.06.003
Morton, D., Jones, A., and Sandhu, J. (2016). Brain imaging of pain: State of the art. J.Pain Res. 9, 613–624. doi: 10.2147/JPR.S60433
Öztürk, Ö, Algun, Z. C., Bombacı, H., and Erdoğan, S. B. (2021). Changes in prefrontal cortex activation with exercise in knee osteoarthritis patients with chronic pain: An fNIRS study. J. Clin. Neurosci. 90, 144–151. doi: 10.1016/j.jocn.2021.05.055
Pascalis, V. D., Varriale, V., and Cacace, I. (2015). Pain modulation in waking and hypnosis in women: Event-related potentials and sources of cortical activity. PLoS One 10:e0128474. doi: 10.1371/journal.pone.0128474
Reneau, M. (2020). Heart rate variability biofeedback to treat fibromyalgia: An integrative literature review. Pain Manag. Nurs. 21, 225–232. doi: 10.1016/j.pmn.2019.08.001
Shi, P., Liu, J., Du, J., Yu, H., and Fang, F. (2022). Pain modulation induced by electronic wrist-ankle acupuncture: A functional near-infrared spectroscopy study. Pain Pract. 22, 182–190. doi: 10.1111/papr.13076
Sihvonen, A. J., Pitkäniemi, A., Särkämö, T., and Soinila, S. (2022). Isn’t there room for music in chronic pain management? J. Pain 23, 1143–1150. doi: 10.1016/j.jpain.2022.01.003
Sihvonen, A. J., Särkämö, T., Leo, V., Tervaniemi, M., Altenmüller, E., and Soinila, S. (2017). Music-based interventions in neurological rehabilitation. Lancet Neurol. 16, 648–660. doi: 10.1016/S1474-4422(17)30168-0
Song, Z., Zhang, M., and Huang, P. (2016). Aberrant emotion networks in early major depressive disorder patients: An eigenvector centrality mapping study. Transl. Psychiatry 6, e819–e819. doi: 10.1038/tp.2016.81
Standley, J. M. (2002). A meta-analysis of the efficacy of music therapy for premature infants. J. Pediatr. Nurs. 17, 107–113. doi: 10.1053/jpdn.2002.124128
Taruffi, L. (2021). Mind-wandering during personal music listening in everyday life: Music-evoked emotions predict thought valence. Int. J. Env. Res. Public Health 18:12321. doi: 10.3390/ijerph182312321
Ting, B., Tsai, C.-L., Hsu, W.-T., Shen, M.-L., Tseng, P.-T., Chen, D. T.-L., et al. (2022). Music intervention for pain control in the pediatric population: A systematic review and meta-analysis. J. Clin. Med. 11:991. doi: 10.3390/jcm11040991
Tong, H., Maloney, T. C., Payne, M. F., King, C. D., Ting, T. V., Kashikar-Zuck, S., et al. (2022). Processing of pain by the developing brain: Evidence of differences between adolescent and adult females. Pain 163, 1777–1789. doi: 10.1097/j.pain.0000000000002571
Tsai, Y.-H., Yuan, R., Patel, D., Chandrasekaran, S., Weng, H.-H., Yang, J.-T., et al. (2018). Altered structure and functional connection in patients with classical trigeminal neuralgia. Hum. Brain Mapp. 39, 609–621. doi: 10.1002/hbm.23696
Wang, B.-K., Liu, T.-H., Xie, F., and Liu, Y.-Q. (2020a). Pain vision system for evaluating chronic pain: A comparison with VAS Scoring. Pain Res. Manag. 2020:e6312581. doi: 10.1155/2020/6312581
Wang, C., Bao, C., Gao, J., Gu, Y., and Dong, X.-W. (2020b). Pain modulates neural responses to reward in the medial prefrontal cortex. Hum. Brain Mapp. 41, 1372–1381. doi: 10.1002/hbm.24882
Wang, Y., Wei, J., Guan, X., Zhang, Y., Zhang, Y., Zhang, N., et al. (2020c). Music intervention in pain relief of cardiovascular patients in cardiac procedures: A systematic review and meta-analysis. Pain Med. 21, 3055–3065. doi: 10.1093/pm/pnaa148
White, J. M. (2000). State of the science of music interventions. Crit. Care Nurs. Clin. North Am. 12, 219–225. doi: 10.1016/S0899-5885(18)30114-X
Winbo, A., Ashton, J. L., and Montgomery, J. M. (2020). Neuroscience in the heart: Recent advances in neurocardiac communication and its role in cardiac arrhythmias. Int. J. Biochem. Cell Biol. 122:105737. doi: 10.1016/j.biocel.2020.105737
Keywords: chronic pain, functional near-infrared spectroscopy, heart rate variability, music intervention, prefrontal hemodynamics
Citation: Du J, Shi P, Fang F and Yu H (2022) Effect of music intervention on subjective scores, heart rate variability, and prefrontal hemodynamics in patients with chronic pain. Front. Hum. Neurosci. 16:1057290. doi: 10.3389/fnhum.2022.1057290
Received: 29 September 2022; Accepted: 02 November 2022;
Published: 17 November 2022.
Edited by:
Michele Davide Mignogna, University of Naples Federico II, ItalyCopyright © 2022 Du, Shi, Fang and Yu. This is an open-access article distributed under the terms of the Creative Commons Attribution License (CC BY). The use, distribution or reproduction in other forums is permitted, provided the original author(s) and the copyright owner(s) are credited and that the original publication in this journal is cited, in accordance with accepted academic practice. No use, distribution or reproduction is permitted which does not comply with these terms.
*Correspondence: Ping Shi, rehabishi@163.com; Fanfu Fang, fangfanfu@126.com