- 1Department of Nuclear Medicine, University of KwaZulu-Natal, Durban, South Africa
- 2Department of Nuclear Medicine, University of Pretoria, Pretoria, South Africa
Introduction: Breast cancer is a complex disease and constitutes the leading cause of cancer in women globally. Conventional treatment modalities include surgery, chemotherapy, radiation therapy, and hormonal therapy; all of these have their limitations and often result in significant side effects or toxicity. Targeted radionuclide therapy based on a theranostic approach has been successfully applied in several malignancies, such as prostate cancer, thyroid cancer, and neuro-endocrine tumours. Several studies have also highlighted the potential of theranostic applications in breast cancer.
Aim: This review aims to provide an overview of the most promising current and future theranostic approaches in breast cancer.
Discussion: The discussion includes pre-clinical as well as clinical data on some of the most successful targets used to date. Examples of potential theranostic approaches include those targeting the Human epidermal growth factor receptor 2 (HER2) expression, angiogenesis, aspects of the tumour microenvironment, Gastrin-releasing peptide receptor (GRPR), Prostate-specific membrane antigen (PSMA) and Chemokine receptor 4 (CXCR-4) expression. Several challenges to widespread clinical implementation remain, which include regulatory approval, access to the various radiopharmaceuticals and imaging technology, cost-effectiveness, and the absence of robust clinical data.
Conclusion: Theranostic approaches have the potential to greatly improve diagnosis, treatment, and outcomes for patients with breast cancer. More research is needed to fully explore the potential of such approaches and to identify the best potential targets, considering feasibility, costs, efficacy, side effects and outcomes.
1. Introduction
The importance and impact of breast cancer is well known, as it is the most common malignancy to affect women globally (1). This necessitates further exploration of novel, targeted approaches, especially where conventional therapies such as surgery, radiation- and chemotherapy fails. Targeted radionuclide therapies are emerging as a promising theranostic strategy that follows identification of sufficient target expression via imaging as an essential first step (2). The aim of this review is to provide an overview of the current and potential targets for translation into theranostic approaches.
Obtaining a clear map of the immunohistopathology and receptor expression, is crucial to selecting and individualising available therapies appropriately. Knowledge of the status of biomarker expression of Estrogen receptors, Progesterone receptors, HER-2 and Ki-67 is integral for accurate subtype classification, direct management, and the best and most appropriate line of therapy (3). The heterogeneity of breast cancer (both intra-and inter-tumoural) however, is well known and unfortunately the treatment plan tends to be largely based on the primary tumour characteristics (4, 5). Considering the impracticality and unacceptability of obtaining multiple biopsies from various disease involvement sites, the advantage of obtaining a whole-body non-invasive investigation with PET/CT first to guide therapy is apparent. Once the therapeutic target is clear, the appropriate isotope can be selected to deliver targeted ionising radiation to cancer cells, whilst sparing healthy cells in what is known as a two-step theranostic approach (6, 7). Figure 1 highlights some of the most important targets in theranostic approaches, which includes Estrogen- and Progesterone receptors, HER-2, GRPR, FAP, PSMA, SSTR, CXCR-4, hypoxia, DNA targets and integrins.
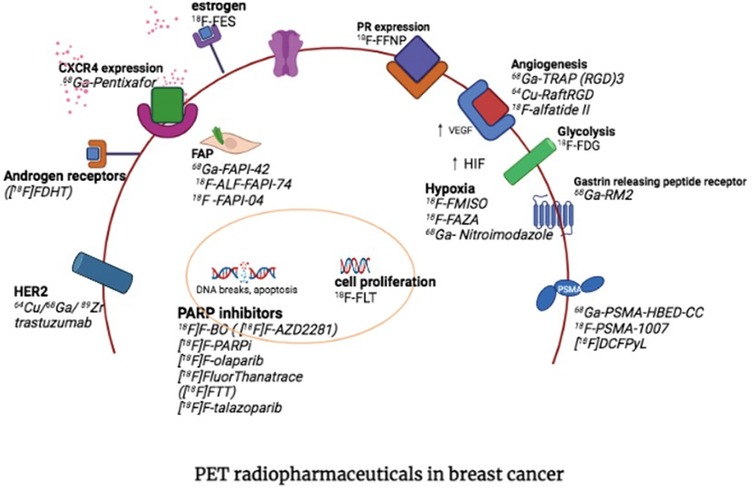
Figure 1. PET targets for theranostic approaches in breast cancer (with permission from Hadebe et al) (7).
The aim of this review is to summarize some of the most interesting and important pre-clinical and clinical approaches with regards to theranostics in breast cancer and to briefly discuss some of the practical and clinical considerations.
2. Human epidermal growth factor receptor 2 (HER2)
2.1. Background and rationale
Expression of this receptor occurs in around 20% of patients with breast cancer; it is currently determined by means of biopsy and immunohistochemistry and is considered a negative prognostic factor (8). It is located on the outer cell surface where it is involved in the regulation of cell growth and differentiation. Tumours with HER2 expression tend to be more aggressive and are associated with a relatively high mortality (8, 9).
Trastuzomab (Herceptin™) is a recombinant G1 immunoglobulin monoclonal antibody that targets and blocks the extracellular domain of the HER2 receptor. It is an FDA-approved humanized monoclonal antibody that targets HER2/neu cancer cells, and which causes an inhibitory effect on cell growth (10, 11). It follows then that trastuzomab would make for an attractive pharmaceutical to label with both imaging and therapeutic isotopes.
Non-representative tissue biopsies may unfortunately result in false negatives on immunohistochemistry in up to 20% of cases (12). Considering the potential impact this has on patient management, there is a strong case for PET/CT imaging in determination of HER2 expression.
2.2. Pre-clinical evidence
In the pre-clinical setting, studies have made use of the following radiopharmaceuticals, namely 64Cu-trastuzomab, 211At-trastuzomab, 111In-DOTA-trastuzomab and 177Lu/90Y-trastuzomab, amongst others. These studies (performed on mouse models) have shown mostly promising results with a significant increase in median survival demonstrated (13, 14).
2.3. Clinical evidence
A group from Chandigarh, India demonstrated the feasibility of in-house labelling of trastuzomab with Lu-177 via the DOTA chelator. They reported 12 h of stability, and image confirmation of the targeted primary and metastatic lesions was obtained at 5 days post-injection. More recently, the same group shared their findings in 6 patients with HER2 positive metastatic breast cancer compared to four patients with HER2 negative breast cancer (15).
SPECT/CT imaging confirmed uptake in the primary and metastatic lesions in contrast to the HER2 neg group that did not demonstrate any abnormal tracer accumulation. 177Lu-trastuzumab administration was well tolerated in nine patients and one patient experienced mild fever. Fever is a known side effect that is associated with trastuzumab administration, and the patient was managed with simple antipyretics. No unexpected toxicities were observed with 177Lu-trastuzumab administration and biochemistry, and cardiac function was not affected. Intense liver accumulation is a limiting factor as expected, and further studies are needed. Feasibility of this form of targeted therapy in metastatic HER2 breast cancer was demonstrated (15).
One of the first studies to be published on the use of trastuzomab imaging in patients with breast cancer was by Dijkers and colleagues and involved the use of 89Zr-trastuzomab in 14 patients. Normal biodistribution of Zr-89-trastuzumab was reported in the liver, kidneys, spleen, and brain apart from localization in metastatic lesions (16).
Other investigators included Gebhart et al. and Bhusari et al. (15, 17). The latter proceeded to substitute 89Zr with Lu-177 in a group of 10 patients with breast cancer to demonstrate feasibility and the possibility of SPECT/CT imaging for target confirmation and dosimetry. They established an optimal imaging time point of 4–5 days post-injection (allowing for sufficient background clearance) and confirmed feasibility of this imaging and therapeutic approach. HER2 positive breast lesions demonstrated sufficient uptake with 10 mCi of 177Lu-trastuzumab whilst no uptake was noted on HER2 negative lesions. The authors highlighted the possibility of a therapeutic approach with 177Lu-trastuzumab in patients with HER2 positive lesions that are refractory to standard forms of therapy (15).
2.4. Treatment considerations
As a complete antibody, trastuzomab is a large protein with a high molecular weight, a long half-life, relatively poor tumour infiltration and inadequate renal filtration. This may lead to limited internalisation and tumour effects, off-target binding with haemato- and hepatotoxicity and inhomogeneous tumour distribution. Most of these problems could, however, be eliminated by making use of an antibody fragment with resultant increased target to background ratio, rapid elimination, and reduced toxicity. The issue of tumour resistance to trastuzomab remains however, and the intense liver accumulation remains problematic (18, 19).
Trastuzomab-directed radionuclide therapy appears feasible and allows for labelling to multiple radioisotopes with promising pre-clinical- and clinical results. The inability to visualise liver lesions due to intense physiological uptake is a limitation.
3. Fibroblast activation protein inhibitors (FAPI)
3.1. Background and rationale
Cancer-associated fibroblasts (CAF) fulfil an integral role within the tumour micro-environment, where they support and facilitate malignant growth and metastatic spread. These fibroblasts express a protein, FAP-alpha, which provides an attractive target for both imaging and therapeutic translation as it is expressed in the stromal tissues of many malignancies, whilst remaining largely absent from normal tissues. Considering that the stroma can account for up to 90% of the total tumor mass, this may increase tumor cell accessibility for pharmacologic, immunologic, or cell-based therapies. Simultaneous irradiation of CAFs and surrounding tumor cells may also be achieved through the crossfire effect. Additionally, since FAP-expressing CAFs are known to be immunosuppressive, a combination with immunotherapy may produce a synergistic therapeutic effect (20, 21).
Fibroblast Activation Protein is a type II integral membrane glycoprotein from the serine protease family that plays a role in fibrogenesis and in the remodelling of the extracellular matrix. FAP-inhibitors (derived from quinoline peptidomimetics) are used to target these receptors and several forms of FAPI are available, such as FAPI-04, FAPI-02, FAPI-46, FAPI-2286. Major advantages offered by this target include its superior target to background ratios, the lack of any required patient preparation and its potential for therapeutic translation (22–24).
Fibroblast Activation Protein as a theranostic target has enjoyed significant attention in recent times with a 2023 review article including 35 publications on targeted radioligand therapy in both the pre-clinical and clinical settings and stating that over 100 patients have been treated with different FAP targeted radionuclide therapies (including 177Lu-FAPI-04, 90Y-FAPI-46, 177Lu-FAP-2286, 177Lu-DOTA.SA.FAPI and 177Lu-DOTAGA.(SA.FAPi)2. These studies have reported objective responses in difficult to treat end-stage cancer patients with manageable adverse events (25).
3.2. Clinical evidence
Ballal and colleagues shared their experience in a 2020 publication on the compassionate use of 177Lu-DOTA-FAPi in a 31-year-old female with breast cancer and a metastatic brain lesion. The primary breast tumour was characterised as ER-, PR- and HER 2+, and she had disease progression on conventional therapy. They administered 3.2 GBq of 177Lu-DOTA-FAPi with target lesion confirmation on dosimetry images, which resulted in symptomatic relief.
A later study with SA.FAPI reported on a total of ten patients, which included four with breast cancer (26).
Baum and colleagues also reported on the use of 5.8 =/− 2 GBq of 177Lu-FAPI-2286 in eleven patients with a variety of malignancies that included four patients with breast cancer. Treatment in this group was well tolerated with acceptable dosimetry and toxicity in addition to resulting in significant pain relief. Post-therapy images revealed significant uptake of 177Lu-FAP-2286 and long retention of the radiopharmaceutical in all patients (27). In contrast, FAPI-02 and FAPI-04 demonstrated earlier washout with a corresponding shorter tumour retention time (28).
Lindner et al. used 2.9 GBq of 90Y-FAPI-04 to treat a patient with metastatic breast cancer, which resulted in significant pain reduction without significant side effects. The authors postulated that higher treatment doses could potentially be used to increase the tumoricidal effect. Bremsstrahlung images confirmed the intended target lesion delivery up to one day post-injection (28).
Assadi et al. treated 21 patients (including 5 breast cancer patients) with advanced stage malignancies with 1.85–4.44 GBq of 177Lu-FAPi-46 per cycle. Feasibility and an acceptable side effect profile was demonstrated in this compassionate use setting (29).
The LUMIERE trial is a phase I/II study to evaluate the safety, dosimetry, pharmacokinetics, and preliminary anti-tumor activity of 177Lu-FAP-2286 in patients with advanced or metastatic solid tumours (30).
A dosimetry study by Kuyumcu et al. following administration of 177Lu-FAPI-04 to four patients (which included one patient with breast cancer) also highlighted the short tumour retention time of FAPI-04 whilst confirming acceptable dosimetry (31).
These initial experiences highlight the safety and feasibility of using radiopharmaceuticals such as 90Y-FAPI-04 and 177Lu-DOTA.SA.FAPI in settings where patients are refractory to conventional forms of therapy.
3.3. Treatment considerations
Several FAP-based tracers have been reported with most data referring to FAPI-04, FAPI-46, FAP-2286, and DATAGA.(SA.FAPI)2, and promising clinical data reported in patients with breast cancer.
Studies have reported acceptable side effect and toxicity profiles, with the most important reported adverse events related to bone marrow toxicity (anaemia, leukopenia, and reduced number of platelets), similar to the now known side effect profiles of 177Lu-PSMA and 177Lu- DOTATATE. Based on the inconsistent data from the various small and non-standardised studies, it is not yet possible to conclusively determine which FAP inhibitor has the best efficacy and toxicity profile. The reported absorbed dose to the tumor are comparable to those of 177Lu-DOTATATE and 177Lu-PSMA-617 and range from 0.62 ± 0.55 Gy/GBq, 2.81 ± 1.25 Gy/Gbq, 3.0 ± 2.7 Gy/GBq, and 6.70 (IQR: 3.40–49) Gy/GBq, for 177Lu-FAPI-04, 90Y-FAPI-46, 177Lu-FAP-2286, and 177Lu-DOTAGA.(SA.FAPi)2, respectively (32, 33).
Most malignancies express FAP in the tumour micro-environment, while some cancers express FAP on their cellular membrane (e.g., sarcoma, certain ovarian, and pancreatic cancers). Crucial to the success of FAP-based targeted radionuclide therapy is the issue of tumour retention time, which should be long enough to achieve a tumoricidal effect, without increasing radiation to non-target lesions and organs. To date the most promising results have been reported with 177Lu-FAP-2286, 177Lu-DOTAGA.(SA. FAPi)2, 177Lu-PNT6555, and the albumin-binding tracers (e.g., 177Lu-EB-FAP) which demonstrates uptake up to 168-h post injection. Attempts to increase tumour retention time have focused on labelling FAPi to radio-isotopes with shorter half-lives (such as Y-90, 188Re, 153Sm, 213Bi, or 212Pb) in an attempt to better match the peptide half-life to that of the isotope. Other strategies to improve on dose delivery to the tumour include development of FAPI-derivatives with better pharmacokinetics and substituting b-emitters with alpha emitters (34).
A publication by Mori summarizes the most important challenges to be addressed with FAPi-based radioligand therapy as follows: (1) increasing tumor retention time to achieve sufficient cumulative activity in the tumor to facilitate biologically effective radiation doses, (2) selection of the most appropriate radionuclide to match the physiological and biological half-life of the tracer, (3) heterogeneity, and (4) minimizing radiation toxicity (35).
4. Prostate-specific membrane antigen (PSMA)
4.1. Background and rationale
Prostate-specific membrane antigen (PSMA) is a type 2 transmembrane protein that is encoded by the FOLH1 (folate hydrolase 1) gene and is overexpressed not only in prostate cancer cells but also in the neo-vasculature of several solid tumours such as breast cancer. Preclinical data suggest that PSMA may be involved in cancer-related angiogenesis through degradation of the extracellular matrix and by participating in integrin signal transduction. PSMA expression has been closely linked both to enhanced angiogenesis and tumour aggressiveness. Interestingly, estrogen and progesterone-negative breast tumours tend to exhibit a higher PSMA expression than receptor-positive phenotypes. In the setting of triple negative breast cancer, this becomes especially useful as therapeutic receptor targets are absent and expression has been demonstrated to increase in hypoxic conditions (36, 37).
4.2. Preclinical evidence
A 2023 in vitro study publication by Heesch and colleagues analysed PSMA and its isoform expression in Triple Negative Breast Cancer cells, breast cancer stem cells, and tumor-associated endothelial cells. They found PSMA expression in 91% of the investigated Triple Negative Breast Cancer cell lines and highlighted the possible role of therapeutic strategies in this clinical setting. The researchers demonstrated that TNBC cells strongly induce PSMA in tumor-associated vasculature, which makes it a promising candidate to target tumor angiogenesis. Interestingly, they also found that hypoxia strongly increased PSMA expression in breast cancer cells, which could be approached by administering PSMA therapy in a hypo-fractionated way, with the first fraction targeting PSMA-expressing endothelial cells and, in this way, decreasing the oxygen supply (38).
Morgenroth et al. used a mouse model of triple negative breast cancer to evaluate the potential of prostate-specific membrane antigen (PSMA) as a target for radio-ligand therapy. The researchers used 177Lu-PSMA-617 and their results demonstrated that it strongly impaired the vitality and angiogenic potential of human umbilical vein endothelial cells. Target lesion localisation was confirmed and the authors concluded that 177Lu-PSMA-617 could potentially be used in a theranostic approach (39).
In tumours other than prostate cancer, PSMA expression happens mainly in the endothelial cells of tumor-associated neo-vasculature, with no endothelial expression under physiological conditions (40).
4.3. Clinical evidence
In a 2013 study by Wernicke et al, the researchers set out to evaluate PSMA expression in tumour-associated vasculature in 106 patients with invasive breast cancer ranging from stage I–IV. Representative tumour tissue from each participant was stained for PSMA expression together with CD31 for confirmation of vasculature presence. The level of PSMA expression detected was scored from 0 to 2 in the following way: 0 = no detectable expression up to 2 = presence of PSMA expression in >50% of micro vessels. PSMA expression was present in 74% of primary breast cancer tumours and in all (n = 14) of those with brain metastases. Furthermore, its expression was absent in 98% of normal breast tissue and associated vasculature. Their results further demonstrated that patients with the highest PSMA expression (score of 2) demonstrated higher median tumour size, higher Ki-67, and poorer overall survival. Patients in whom Estrogen receptors were negative were more likely to have a PSMA score of 2 (41).
In a study consisting of 19 patients with breast cancer, Sathekge et al. demonstrated significant PSMA uptake in 84% of the 81 malignant and metastatic lesions with 68Ga-PSMA-HBED-CC PET/CT. Metastatic lesions demonstrated higher SUV mean values compared to the primary tumours and no specific association with the presence or absence of progesterone receptors could be demonstrated. The authors highlighted the potential role in anti-angiogenic therapeutic strategies (42).
In one of the biggest studies to date, Tolkach and colleagues evaluated PSMA expression in the tumour endothelium (via immunohistochemistry) of 315 breast cancer patients with invasive non-special type carcinoma (NST) and lobular carcinoma. This group demonstrated PSMA expression in 60% of their study population, with higher expression noted in higher grade tumours and those with HER-2 expression and the highest expression in those with triple negative breast cancers (43).
A 2021 review article by Uijen et al, summarizes the use of PSMA-based radioligand therapy in solid tumours other than prostate cancer, which included breast cancer. The authors summarised the PSMA expression (based on SUVmax values) as low to moderate in breast cancer and did not highlight breast cancer as an ideal candidate for treatment with PSMA-based radioligand therapy (40).
In one of the few published cases of PSMA-based targeted radionuclide therapy in breast cancer, Tolkach et al. shares their experience in treating a young woman with an aggressive triple negative breast cancer that was unresponsive to several conventional lines of therapy. Two cycles of Lu-177-PSMA-RLT at a dose of 7.5 GBq was administered based on sufficient PSMA expression on 68Ga-PSMA PET/CT. Post-injection SPECT imaging confirmed delivery of the radiopharmaceutical to the targeted lesions and no adverse effects were reported. Treatment was discontinued after the second cycle of therapy based on disease progression (43).
4.4. Treatment considerations
Considering the beta particle tissue range (+/−2 mm) of radioligand therapy with 177Lu-PSMA, it could potentially still reach nearby tumour cells in well-perfused malignancies, even if PSMA is exclusively over-expressed in the endothelial cells of neo-vasculature, with resultant abscopal effect. Other concerns with 177Lu-PSMA therapy that targets PSMA expression only on the neo-vasculature relate to the expected short tumour retention time and reduced efficacy.
5. Somatostatin receptors (SSTRs)
Somatostatin receptor expression occurs in breast cancer whenever there is neuro-endocrine differentiation. This demonstrates overexpression of mostly SSTR2A and SSTR5 on immunohistochemistry and is associated with a worse prognosis (44). Kumar et al. used RT-PCR and immunohistochemistry to investigate the SSTR subtype expression in breast cancer and reported the following levels of expression: SSTR1 (84%), SSTR2 (79%), SSTR3 (89%), SSTR4 (68%), and SSTR5 (68%). Estrogen receptor (ER) levels were seen to correlate with SSTR 1, 2, and 4, while a correlation with progesterone receptor (PR) levels was seen only with SSTR2 (45).
A study by Zou et al. on 160 specimens of ductal breast carcinoma reported SSTR expression as follows: SSTR1 (90%), SSTR4 (71.3%), SSTR5 (44.4%), SSTR3 (41.9%), and SSTR2 (34.4%). They found that SSTR expression was inversely correlated to tumor differentiation, and there was no association between receptor expression and hormone receptors (ER/PR). These variations highlight the need for further studies to elaborate on the expression of SSTR and ER/PR hormone receptors (46).
5.1. Preclinical studies
Dalm et al. targeted SSTR2 receptors in a mouse model of breast cancer with both an SSTR agonist (177Lu-DOTATATE) and an antagonist (177Lu-DOTA-JR11). Results demonstrated a five times higher tumour accumulation of 177Lu-DOTA-JR11 compared to the agonist target and this uptake translated to better tumour control and survival rates in mice (47).
5.2. Clinical studies
Savelli and colleagues evaluated the use of 90Y-DOTATOC in a patient with metastatic breast cancer with neuroendocrine differentiation and liver metastases. They administered 2.57 GBq of 90Y-DOTATOC over a course of three cycles and used bremsstrahlung to confirm the intended target with SPECT/CT at 4 days post-administration. They reported a significant CT and biochemistry response in the absence of any adverse effects (48).
In a case study published by Liu et al., the authors administered 6 cycles of 177Lu-DOTATOC in a patient with metastatic invasive ductal breast carcinoma and primary large-cell neuroendocrine breast cancer. Intense tracer accumulation was confirmed in the target lesions, which led to a partial treatment response in the absence of any significant side effects or toxicity (49).
The current level of evidence is unfortunately mostly limited to pre-clinical studies and isolated clinical case reports.
6. Chemokine receptor 4 (CXCR-4)
The CXCR4 chemokine (in association with CXCL12) is actively involved in processes that support growth and metastatic spread, such as gene expression, cell proliferation and migration, tumorigenesis, and angiogenesis. Increased CXCR4 is strongly associated with a shorter overall survival and a shorter progression-free survival. Patients who are eligible for CXCR4-directed radioligand therapy may be treated with β-emitters such as 177Lu/90Y-PentixaTher, and this approach has been used successfully mainly in the clinical settings of hematological malignancies (50).
CXCR4-directed therapies have the potential to provide therapeutic options to breast cancer patients without sufficient expression of hormonal receptors or those who become treatment resistant. The feasibility of such an approach has been demonstrated in pre-clinical studies with successful inhibition of cancer progression and metastases.
An example of a selective CXCR4 antagonist is balixafortide, which has been shown to enhance the cytotoxic effect of chemotherapy in breast cancer. Pernas et al. demonstrated the safety and tolerability of balixafortide in combination with eribulin in 56 heavily pre-treated patients with relapsed HER2-negative metastatic breast cancer (51). These findings were subsequently confirmed in the phase 3 randomized multicentre FORTRESS trial with objective responses documented in 35% of patients (52).
Injected 177Lu-Pentixfor binds to plasma proteins with high stability, leaving less than 5% available to bind to CXCR4 receptors on white blood cells and platelets. Radiopharmaceutical accumulation takes place in CXCR4-expressing malignant and metastatic lesions (with high tumour retention) as well as in the liver, kidneys, and bone marrow. Tracer accumulation in the bone marrow has a half-life of several days, which makes this the critical organ for acute toxicity. Bone marrow doses range from 0.14 to 2.3 (median value, 0.5) Gy/GBq 177Lu, which makes it suitable for myeloablative therapy; even in this setting, Lu-177 may be best substituted with the shorter-lived Y-90. Reports on renal dosimetry (as the dose-limiting organ) also vary greatly, ranging from 0.14 to 2.3 (median value, 0.5) Gy/GBq 177Lu (53).
Considering the promising results that have been obtained with CXCR4-directed radioligand therapy in hematological malignancies, the same approach could potentially be applied to solid tumor patients with sufficient CXCR4 expression on PET. However, optimal bone marrow support (stem cell transplant) would likely be required.
7. Gastrin-releasing peptide receptor (GRPR)
This transmembrane G-protein coupled receptor can be conjugated to bombesin and provides several attractive agonist and antagonist possibilities for both imaging and therapeutic possibilities. Gastrin-releasing peptide receptor (GRPR) is overexpressed in over 75% of breast cancers and can be efficiently targeted with 177Lu-Bombesin to deliver therapeutic radiation doses to malignant cells. Bombesin is an analog of the mammalian gastrin-releasing peptide (GRP) and has demonstrated high sensitivity to detect the GRP receptor in breast cancer (54).
The bombesin agonist AMBA, linked to DOTA, as 177Lu-AMBA was first reported to demonstrate high therapeutic potential in several prostate cancer models with different levels of GRPR expression. Other therapeutic labelling options include Terbium and Scandium's therapeutic isotopes as well as alpha emitters such as Ac-225 and Bi-213.
Initial studies with radiotracers labelled to GRPR agonists highlighted some unfavourable characteristics related to low metabolic stability, unfavourable abdominal accumulation, and adverse effects, which motivated a change toward GRPR antagonists. These safer analogues demonstrate improvements in metabolic stability and background clearance.68Ga-RM2 is an example of such a GRPR antagonist that is one of the more frequently used imaging possibilities (55).
In a 2016 pre-clinical feasibility study, Aranda-Lara and colleagues demonstrated high tumour uptake of 177Lu-Bombesin in a mouse-model. More recent preclinical studies in mice models have demonstrated the increased efficacy of the mTOR inhibitor rapamycin when used in combination with 177Lu-RM2 (56, 57).
7.1. Clinical studies
A first in human study evaluated the use of 177Lu-RM2 in the setting of metastatic castration-resistant prostate cancer. Tumour absorbed doses were found to be therapeutically relevant, whereas rapid clearance from normal GRPR-rich organs, such as the pancreas, was confirmed. (The latter is considered to be the dose-limiting organ due to its high radioligand uptake). Despite the encouraging dosimetry results, certain derivatives demonstrate poor metabolic stability, which limits theranostic use (56). Lu-177-AMTG was identified as the therapeutic candidate with the most potential, but further studies are needed. 68Ga/177Lu-NeoBOMB1 provides another promising theranostic approach which has been evaluated in prostate cancer patients with good detection of primary tumours and liver and bone metastases (58).
Combination therapies with mTOR-inhibitors, PRRT, immunotherapy and hormone therapy in breast cancer may provide another promising route toward higher therapeutic responses.
8. Poly-ADP ribose polymerase (PARP)
Poly-ADP ribose Polymerase-1 (PARP1) is a nuclear DNA repair enzyme which functions as an early sensor of single strand DNA breaks. Breast Cancer proteins (BRCA1/2mut) may result in faulty double strand DNA repair mechanisms, leading to PARP1-mediated processes. Rapidly proliferating cancer cells are under higher replicative stress, which leads to genomic instability and overexpression of PARP1. PARP-1 inhibition presents a target for theranostic approaches in breast (and ovarian) cancer (59).
Imaging of PARP1 expression may identify patients who are likely to respond to PARP inhibitor treatment; it may provide prognostic information and it has the potential to provide personalised and targeted treatment options. Examples of FDA-approved PARP inhibitors (PARPis) include olaparib, rucaparib, niraparib and talazoparib (60, 61).
8.1. Preclinical studies
Sankaranarayanan et al, investigated a theranostic approach in a triple negative breast cancer xenografted mouse model by radiolabelling the PARPi-derivative Olaparib to the Auger emitters 123/125I. SPECT/CT images were acquired at 4 h or 24 h post injection of 123I-PARPi-01, followed by a therapeutic approach with 125I-PARPi-01 administered in 4 doses of 10 MBq/dose injected 10 days apart. A significant delay in tumour growth and doubling time was demonstrated on weekly CT scans, in the absence of significant radiotoxicity to the thyroid and liver. A significantly higher apoptosis ratio was demonstrated in the 125I-PARPi-01-treated tumour tissues (62).
This presents a potential targeted radionuclide therapy approach for patients with triple negative breast cancer, although improvements to the pharmacokinetics are necessary prior to its potential clinical application.
9. Integrin imaging with arginine-glysine-aspartic acid (RGD)
Angiogenesis with integrin expression is an essential component of malignant growth and metastatic spread. Integrin αvβ3 is a receptor for extra-cellular matrix proteins with the exposed arginine(R)-glycine(G)-aspartic acid(D) tripeptide sequence, and as such it provides an interesting molecular target for both diagnostic and therapeutic approaches in solid tumours (63).
Apart from detecting areas of neo-angiogenesis, RGD uptake values on a baseline PET study can be used to predict how likely a tumour is to respond to antiangiogenic therapy (64, 65). Furthermore, it can be used to monitor the occurrence of adverse events in normal organs.
In diagnostic PET/CT studies, researchers have generally demonstrated lower values of RGD accumulation when compared to FDG, with superior brain lesion detection of RGD but inferior detection of liver involvement. RGD uptake varies significantly depending on hormonal receptor expression in breast cancer. 18F-Alfatide II has for example demonstrated higher uptake than 18F-FDG in HER2 (–) and ER(+) breast cancer lesions. 68Ga-BBN-RGD represents another example of a RGD-based PET/CT tracer (66–68).
Both 18F-Alfatide II and 68Ga-BBN-RGD offer good diagnostic performance in the detection of breast cancer, which is comparable to 18F-FDG but not necessarily superior. RGD-based tracers may however have superior detection in breast cancer that expresses estrogen receptors in the absence of HER2 (67, 68).
In a theranostic approach, 68Ga may be replaced with 177Lu in order to target lesions with integrin overexpression, as has been successfully demonstrated in pre-clinical studies with 177Lu-EB-RGD in the setting of lung cancer. Clinical translation in the setting of breast cancer may potentially enable a highly personalised approach using 68Ga-RGD PET/CT to non-invasively image and quantify integrin αvβ3 expression and accordingly select the most suitable patients who are likely to benefit from therapy (69).
10. Radiopharmaceutical choice
Mapping and identification of the primary tumour characteristics is crucial in directing selection of the most appropriate imaging and therapeutic targets. Therapeutic isotope possibilities include both alpha- and beta emitters, such as I-131, Lu-177, Y-90, Ac-225, Ra-223 and Tb-161 amongst others, and these will likely be selected based on a combination of factors that include cost, availability, logistics, tumour size and distribution patterns.
The beta-emitter Lu-177 is currently the most widely used therapeutic radioisotope after 131I following its excellent track record in the settings of prostate cancer and neuroendocrine tumours. Other advantages include the relatively long half-life (to allow transport and other logistics), favourable nuclear decay characteristics, chemistry that allows for versatile labelling options, high radiochemical yield and stability with DOTA-derivatives and the post-treatment imaging possibility for target confirmation and dosimetry.
Alpha emitters provide the advantages of a high linear transfer energy and high tumoricidal- and bystander effects that are independent of oxygen supply and cell cycle timings. Disadvantages include access, high costs and reliable supply in addition to problematic dosimetry assessments. 225Actinium have been tested since 2016 with encouraging results in prostate cancers amongst others.
Therapeutic isotopes should be selected based on the range of radiation required in tissue with appropriate matching of the half-life to that of the linked peptide, whilst considering the safety profile in the context of the patient's existing organ function and co-morbidities.
11. Conclusions
Despite advances in the treatment of breast cancer, there is still a significant portion of the breast cancer population who are not ideally suited to, or who ultimately fail, currently available therapies and are subsequently left with few or no options. It is this group that may potentially benefit most from targeted radioligand therapies chosen to suit the individual patient's particular tumour characteristics and receptor expression. Notwithstanding increasing reports on the therapeutic use of various radiolabeled targets in breast cancer, evidence remains limited to small, non-standardized observational studies and to isolated case reports. Theranostic approaches have the potential to greatly improve diagnosis, treatment, and outcomes for patients with breast cancer. This review highlights the need for further research to explore the potential of such approaches and to identify the best potential targets, whilst considering access, feasibility, costs, efficacy, side effects and outcomes.
Author contributions
All three authors contributed to the conceptualisation of the submission. MV wrote the article with input and suggestions from BH. Finalisation and review was by MS. All authors contributed to the article and approved the submitted version.
Conflict of interest
The authors declare that the research was conducted in the absence of any commercial or financial relationships that could be construed as a potential conflict of interest.
The author MS declared that they were an editorial board member of Frontiers, at the time of submission. This had no impact on the peer review process and the final decision.
Publisher's note
All claims expressed in this article are solely those of the authors and do not necessarily represent those of their affiliated organizations, or those of the publisher, the editors and the reviewers. Any product that may be evaluated in this article, or claim that may be made by its manufacturer, is not guaranteed or endorsed by the publisher.
References
1. Siegel RL, Miller KD, Fuchs HE, Jemal A. Cancer statistics, 2022. CA Cancer J Clin. (2022) 72(1):7–33. doi: 10.3322/caac.21708
2. Balma M, Liberini V, Buschiazzo A, Racca M, Rizzo A, Nicolotti DG, et al. The role of theragnostics in breast cancer: a systematic review of the last 12 years. Curr Med Imaging. (2023) 19(8):817–31. doi: 10.2174/1573405619666230216114748
3. Gradishar WJ, Moran MS, Abraham J, Aft R, Agnese D, Allison KH, et al. Breast cancer, version 3.2022, NCCN clinical practice guidelines in oncology. J Natl Compr Cancer Network. (2022) 20(6):691–722. doi: 10.6004/jnccn.2022.0030
4. Zardavas D, Irrthum A, Swanton C, Piccart M. Clinical management of breast cancer heterogeneity. Nat Rev Clin Oncol. (2015) 12(7):381–94. doi: 10.1038/nrclinonc.2015.73
5. Liang Y, Zhang H, Song X, Yang Q. Metastatic heterogeneity of breast cancer: molecular mechanism and potential therapeutic targets. In: Skvortsova I, editor. Seminars in cancer biology. Vol. 60. Academic Press (2020). p. 14–27.
6. Redfern J. Theranostics: cancer imaging and therapy using injectable radionuclide-labeled ligands. Pharm Pharm Int J. (2020) 8:325–31. doi: 10.15406/ppij.2020.08.00313
7. Hadebe B, Harry L, Ebrahim T, Pillay V, Vorster M. The role of PET/CT in breast cancer. Diagnostics. (2023) 13(4):597. doi: 10.3390/diagnostics13040597
8. Kreutzfeldt J, Rozeboom B, Dey N, De P. The trastuzumab era: current and upcoming targeted HER2 + breast cancer therapies. Am J Cancer Res. (2020) 10(4):1045. PMID: 32368385.32368385
9. Velikyan I, Schweighöfer P, Feldwisch J, Seemann J, Frejd FY, Lindman H, et al. Diagnostic HER2-binding radiopharmaceutical, [68Ga] ga-ABY-025, for routine clinical use in breast cancer patients. Am J Nucl Med Mol Imaging. (2019) 9(1):12. PMID: 30911434.30911434
10. Wolff AC, Hammond ME, Hicks DG, Dowsett M, McShane LM, Allison KH, et al. Recommendations for human epidermal growth factor receptor 2 testing in breast cancer: American society of clinical oncology/college of American pathologists clinical practice guideline update. Arch Pathol Lab Med. (2014) 138(2):241–56. doi: 10.5858/arpa.2013-0953-SA
11. Hudis CA. Trastuzumab—mechanism of action and use in clinical practice. N Engl J Med. (2007) 357(1):39–51. doi: 10.1056/NEJMra043186
12. Wolff AC, Hammond ME, Allison KH, Harvey BE, McShane LM, Dowsett M. HER2 testing in breast cancer: american society of clinical oncology/college of American pathologists clinical practice guideline focused update summary. J Oncol Pract. (2018) 14(7):437–41. doi: 10.1200/JOP.18.00206
13. Boskovitz A, McLendon RE, Okamura T, Sampson JH, Bigner DD, Zalutsky MR. Treatment of HER2-positive breast carcinomatous meningitis with intrathecal administration of α-particle-emitting 211At-labeled trastuzumab. Nucl Med Biol. (2009) 36(6):659–69. doi: 10.1016/j.nucmedbio.2009.04.003
14. Guryev EL, Volodina NO, Shilyagina NY, Gudkov SV, Balalaeva IV, Volovetskiy AB, et al. Radioactive (90Y) upconversion nanoparticles conjugated with recombinant targeted toxin for synergistic nanotheranostics of cancer. Proc Natl Acad Sci USA. (2018) 115(39):9690–5. doi: 10.1073/pnas.1809258115
15. Bhusari P, Vatsa R, Singh G, Parmar M, Bal A, Dhawan DK, et al. Development of lu-177-trastuzumab for radioimmunotherapy of HER2 expressing breast cancer and its feasibility assessment in breast cancer patients. Int J Cancer. (2017) 140(4):938–47. doi: 10.1002/ijc.30500
16. Dijkers EC, Oude Munnink TH, Kosterink JG, Brouwers AH, Jager PL, De Jong JR, et al. Biodistribution of 89Zr-trastuzumab and PET imaging of HER2-positive lesions in patients with metastatic breast cancer. Clin Pharmacol Ther. (2010) 87(5):586–92. doi: 10.1038/clpt.2010.12
17. Gebhart G, Lamberts LE, Wimana Z, Garcia C, Emonts P, Ameye L, et al. Molecular imaging as a tool to investigate heterogeneity of advanced HER2-positive breast cancer and to predict patient outcome under trastuzumab emtansine (T-DM1): the ZEPHIR trial. Ann Oncol. (2016) 27(4):619–24. doi: 10.1093/annonc/mdv577
18. Lobo ED, Hansen RJ, Balthasar JP. Antibody pharmacokinetics and pharmacodynamics. J Pharm Sci. (2004) 93(11):2645–68. doi: 10.1002/jps.20178
19. Altunay B, Morgenroth A, Beheshti M, Vogg A, Wong NC, Ting HH, et al. HER2-directed antibodies, affibodies and nanobodies as drug-delivery vehicles in breast cancer with a specific focus on radioimmunotherapy and radioimmunoimaging. Eur J Nucl Med Mol Imaging. (2021) 48:1371–89. doi: 10.1007/s00259-020-05094-1
20. Galli F, Aguilera JV, Palermo B, Markovic SN, Nisticò P, Signore A. Relevance of immune cell and tumor microenvironment imaging in the new era of immunotherapy. J Exp Clin Cancer Res. (2020) 39(1):1–21. doi: 10.1186/s13046-020-01586-y
21. Janco JM T, Lamichhane P, Karyampudi L, Knutson KL. Tumor-infiltrating dendritic cells in cancer pathogenesis. J Immunol. (2015) 194(7):2985–91. doi: 10.4049/jimmunol.1403134
22. Gonzalez H, Hagerling C, Werb Z. Roles of the immune system in cancer: from tumor initiation to metastatic progression. Genes Dev. (2018) 32(19–20):1267–84. doi: 10.1101/gad.314617.118
23. Sliker BH, Campbell PM. Fibroblasts influence the efficacy, resistance, and future use of vaccines and immunotherapy in cancer treatment. Vaccines (Basel). (2021) 9(6):634. doi: 10.3390/vaccines9060634
24. Sollini M, Kirienko M, Gelardi F, Fiz F, Gozzi N, Chiti A. State-of-the-art of FAPI-PET imaging: a systematic review and meta-analysis. Eur J Nucl Med Mol Imaging. (2021) 48(13):4396–414. doi: 10.1007/s00259-021-05475-0
25. Privé BM, Boussihmad MA, Timmermans B, van Gemert WA, Peters SM, Derks YH, et al. Fibroblast activation protein-targeted radionuclide therapy: background, opportunities, and challenges of first (pre) clinical studies. Eur J Nucl Med Mol Imaging. (2023) 50:1–3. doi: 10.1007/s00259-023-06144-0
26. Ballal S, Yadav MP, Kramer V, Moon ES, Roesch F, Tripathi M, et al. A theranostic approach of [68Ga] ga-DOTA.SA.FAPi PET/CT-guided [177Lu] lu-DOTA.SA.FAPi radionuclide therapy in an end-stage breast cancer patient: new frontier in targeted radionuclide therapy. Eur J Nucl Med Mol Imaging. (2021) 48:942–4. doi: 10.1007/s00259-020-04990-w
27. Baum RP, Schuchardt C, Singh A, Chantadisai M, Robiller FC, Zhang J, et al. Feasibility, biodistribution, and preliminary dosimetry in peptide-targeted radionuclide therapy of diverse adenocarcinomas using 177Lu-FAP-2286: first-in-humans results. J Nucl Med. (2022) 63(3):415–23. doi: 10.2967/jnumed.120.259192
28. Lindner T, Loktev A, Altmann A, Giesel F, Kratochwil C, Debus J, et al. Development of quinoline-based theranostic ligands for the targeting of fibroblast activation protein. J Nucl Med. (2018) 59(9):1415–22. doi: 10.2967/jnumed.118.210443
29. Assadi M, Rekabpour SJ, Jafari E, Divband G, Nikkholgh B, Amini H, et al. Feasibility and therapeutic potential of 177Lu–fibroblast activation protein inhibitor–46 for patients with relapsed or refractory cancers: a preliminary study. Clin Nucl Med. (2021) 46(11):e523–30. doi: 10.1097/RLU.0000000000003810
30. McConathy J, Dhawan M, Goenka AH, Lim EA, Menda Y, Chasen B, et al. Abstract CT251: LuMIERE: a phase 1/2 study investigating safety, pharmacokinetics, dosimetry, and preliminary antitumor activity of 177Lu-FAP-2286 in patients with advanced or metastatic solid tumors. Cancer Res. (2022) 82(12 Suppl):CT251. doi: 10.1158/1538-7445.AM2022-CT251
31. Kuyumcu S, Kovan B, Sanli Y, Buyukkaya F, Simsek DH, Özkan ZG, et al. Safety of fibroblast activation protein–targeted radionuclide therapy by a low-dose dosimetric approach using 177Lu-FAPI04. Clin Nucl Med. (2021) 46(8):641–6. doi: 10.1097/RLU.0000000000003667
32. Del Prete M, Buteau FA, Arsenault F, Saighi N, Bouchard LO, Beaulieu A, et al. Personalized 177 Lu-octreotate peptide receptor radionuclide therapy of neuroendocrine tumours: initial results from the P-PRRT trial. Eur J Nucl Med Mol Imaging. (2019) 46:728–42. doi: 10.1007/s00259-018-4209-7
33. Violet J, Jackson P, Ferdinandus J, Sandhu S, Akhurst T, Iravani A, et al. Dosimetry of 177Lu-PSMA-617 in metastatic castration-resistant prostate cancer: correlations between pretherapeutic imaging and whole-body tumor dosimetry with treatment outcomes. J Nucl Med. (2019) 60(4):517–23. doi: 10.2967/jnumed.118.219352
34. Ballal S, Yadav MP, Moon ES, Kramer VS, Roesch F, Kumari S, et al. First-in-human results on the biodistribution, pharmacokinetics, and dosimetry of [177Lu] Lu-DOTA.SA.FAPi and [177Lu] Lu-DOTAGA.(SA.FAPi) 2. Pharmaceuticals. (2021) 14(12):1212. doi: 10.3390/ph14121212
35. Mori Y, Kratochwil C, Haberkorn U, Giesel FL. Fibroblast activation protein inhibitor theranostics: early clinical translation. PET Clin. (2023) 18(3):419–28. doi: 10.1016/j.cpet.2023.02.007
36. Kratochwil C, Fendler WP, Eiber M, Hofman MS, Emmett L, Calais J, et al. Joint EANM/SNMMI procedure guideline for the use of 177Lu-labeled PSMA-targeted radioligand-therapy (177Lu-PSMA-RLT). Eur J Nucl Med Mol Imaging. (2023) 50:1–6. doi: 10.1007/s00259-023-06255-8
37. Spatz S, Tolkach Y, Jung K, Stephan C, Busch J, Ralla B, et al. Comprehensive evaluation of prostate specific membrane antigen expression in the vasculature of renal tumors: implications for imaging studies and prognostic role. J Urol. (2018) 199(2):370–7. doi: 10.1016/j.juro.2017.08.079
38. Heesch A, Ortmanns L, Maurer J, Stickeler E, Sahnoun SE, Mottaghy FM, et al. The potential of PSMA as a vascular target in TNBC. Cells. (2023) 12(4):551. doi: 10.3390/cells12040551
39. Morgenroth A, Tinkir E, Vogg AT, Sankaranarayanan RA, Baazaoui F, Mottaghy FM. Targeting of prostate-specific membrane antigen for radio-ligand therapy of triple-negative breast cancer. Breast Cancer Res. (2019) 21:1. doi: 10.1186/s13058-019-1205-1
40. Uijen MJM, Derks YHW, Merkx RIJ, Schilham MGM, Roosen J, Privé BM, et al. PSMA radioligand therapy for solid tumors other than prostate cancer: background, opportunities, challenges, and first clinical reports. Eur J Nucl Med Mol Imaging. (2021) 48:4350–68. doi: 10.1007/s00259-021-05433-w
41. Wernicke AG, Varma S, Greenwood EA, Christos PJ, Chao KC, Liu H, et al. Prostate-specific membrane antigen expression in tumor-associated vasculature of breast cancers. APMIS. (2014) 122(6):482–9. doi: 10.1111/apm.12195
42. Sathekge M, Lengana T, Modiselle M, Vorster M, Zeevaart J, Maes A, et al. 68Ga-PSMA-HBED-CC PET imaging in breast carcinoma patients. Eur J Nucl Med Mol Imaging. (2017) 44:689–94. doi: 10.1007/s00259-016-3563-6
43. Tolkach Y, Gevensleben H, Bundschuh R, Koyun A, Huber D, Kehrer C, et al. Prostate-specific membrane antigen in breast cancer: a comprehensive evaluation of expression and a case report of radionuclide therapy. Breast Cancer Res Treat. (2018) 169:447–55. doi: 10.1007/s10549-018-4717-y
44. Tsang JY, Gary MT. Breast cancer with neuroendocrine differentiation: an update based on the latest WHO classification. Mod Pathol. (2021) 34(6):1062–73. doi: 10.1038/s41379-021-00736-7
45. Kumar U, Grigorakis SI, Watt HL, Sasi R, Snell L, Watson P, et al. Somatostatin receptors in primary human breast cancer: quantitative analysis of mRNA for subtypes 1–5 and correlation with receptor protein expression and tumor pathology. Breast Cancer Res Treat. (2005) 92:175–86. doi: 10.1007/s10549-005-2414-0
46. Zou YI, Tan H, Zhao Y, Zhou Y, Cao LI. Expression and selective activation of somatostatin receptor subtypes induces cell cycle arrest in cancer cells. Oncol Lett. (2019) 17(2):1723–31. doi: 10.3892/ol.2018.9773
47. Dalm SU, Nonnekens J, Doeswijk GN, de Blois E, van Gent DC, Konijnenberg MW, et al. Comparison of the therapeutic response to treatment with a 177Lu-labeled somatostatin receptor agonist and antagonist in preclinical models. J Nucl Med. (2016) 57(2):260–5. doi: 10.2967/jnumed.115.167007
48. Savelli G, Zaniboni A, Bertagna F, Bosio G, Nisa L, Rodella C, et al. Peptide receptor radionuclide therapy (PRRT) in a patient affected by metastatic breast cancer with neuroendocrine differentiation. Breast Care. (2012) 7(5):408–10. doi: 10.1159/000343612
49. Liu Q, Zhang J, Kulkarni HR, Baum RP. 177Lu-DOTATOC peptide receptor radionuclide therapy in a patient with neuroendocrine breast carcinoma and breast invasive ductal carcinoma. Clin Nucl Med. (2020) 45(5):e232–5. doi: 10.1097/RLU.0000000000003005
50. Chatterjee S, Azad BB, Nimmagadda S. The intricate role of CXCR4 in cancer. Adv Cancer Res. (2014) 124:31–82. doi: 10.1016/B978-0-12-411638-2.00002-1
51. Pernas S, Martin M, Kaufman PA, Gil-Martin M, Pardo PG, Lopez-Tarruella S, et al. Balixafortide plus eribulin in HER2-negative metastatic breast cancer: a phase 1, single-arm, dose-escalation trial. Lancet Oncol. (2018) 19(6):812–24. doi: 10.1016/S1470-2045(18)30147-5
52. Kaufman P, Martin M, Mayer I, Vahdat L, Pernas S, Schmid P, et al. Abstract PD13-01: balixafortide (a CXCR4 antagonist)+ eribulin versus eribulin alone in patients with HER2 negative, locally recurrent or metastatic breast cancer: an international, randomized, phase 3 trial (FORTRESS). Cancer Res. (2022) 82(4 Suppl):PD13-01. doi: 10.1158/1538-7445.SABCS21-PD13-01
53. Buck AK, Serfling SE, Lindner T, Hänscheid H, Schirbel A, Hahner S, et al. CXCR4-targeted theranostics in oncology. Eur J Nucl Med Mol Imaging. (2022) 49(12):4133–44. doi: 10.1007/s00259-022-05849-y
54. Beer M, Montani M, Gerhardt J, Wild PJ, Hany TF, Hermanns T, et al. Profiling gastrin-releasing peptide receptor in prostate tissues: clinical implications and molecular correlates. Prostate. (2012) 72(3):318–25. doi: 10.1002/pros.21434
55. Stoykow C, Erbes T, Maecke HR, Bulla S, Bartholomä M, Mayer S, et al. Gastrin-releasing peptide receptor imaging in breast cancer using the receptor antagonist 68Ga-RM2 and PET. Theranostics. (2016) 6(10):1641. doi: 10.7150/thno.14958
56. Aranda-Lara L, Ferro-Flores G, Azorín-Vega E, de María Ramírez F, Jiménez-Mancilla N, Ocampo-García B, et al. Synthesis and evaluation of Lys1 (α, γ-folate) Lys3 (177Lu-DOTA)-bombesin (1-14) as a potential theranostic radiopharmaceutical for breast cancer. Appl Radiat Isot. (2016) 107:214–9. doi: 10.1016/j.apradiso.2015.10.030
57. Kurth J, Krause BJ, Schwarzenböck SM, Bergner C, Hakenberg OW, Heuschkel M. First-in-human dosimetry of gastrin-releasing peptide receptor antagonist [177Lu]Lu-RM2: a radiopharmaceutical for the treatment of metastatic castration-resistant prostate cancer. Eur J Nucl Med Mol Imaging. (2020) 47(1):123–35. doi: 10.1007/s00259-019-04504-3
58. Mansi R, Nock BA, Dalm SU, Busstra MB, van Weerden WM, Maina T. Radiolabeled bombesin analogs. Cancers (Basel). (2021) 13(22):5766. doi: 10.3390/cancers13225766
59. Jowanaridhi B, Sriwiang W. Radiolabeling efficiency and stability study on Lutetium-177 labeled bombesin peptide. J Phys Conf Ser. (2019) 1380(1):012020. doi: 10.1088/1742-6596/1380/1/012020
60. Knight JC, Koustoulidou S, Cornelissen B. Imaging the DNA damage response with PET and SPECT. Eur J Nucl Med Mol Imaging. (2017) 44:1065–78. doi: 10.1007/s00259-016-3604-1
61. Kossatz S, Weber W, Beheshti M, Morgenroth A, Mottaghy FM. Advancements in PARP1 targeted nuclear imaging and theranostic probes. J Clin Med. (2020) 9(7):2130. doi: 10.3390/jcm9072130
62. Ambur Sankaranarayanan R, Florea A, Allekotte S, Vogg AT, Maurer J, Schäfer L, et al. PARP Targeted auger emitter therapy with [125I] PARPi-01 for triple-negative breast cancer. EJNMMI Res. (2022) 12(1):1–2. doi: 10.1186/s13550-021-00873-9
63. Auguste P, Lemiere S, Larrieu-Lahargue F, Bikfalvi A. Molecular mechanisms of tumor vascularization. Crit Rev Oncol Hematol. (2005) 54(1):53–61. doi: 10.1016/j.critrevonc.2004.11.006
64. Fang PE, Ming CH. The advances of anti-angiogenesis and normalization of tumor vasculature. Zhongguo Fei Ai Za Zhi. (2009) 12(7):799–804. doi: 10.3779/j.issn.1009-3419.2009.07.011
65. Ellis LM, Liu W, Ahmad SA, Fan F, Do Jung Y, Shaheen RM, et al. Overview of angiogenesis: biologic implications for antiangiogenic therapy. InSeminars in Oncology. (2001) 28:94–104. doi: 10.1016/S0093-7754(01)90287-8
66. Cui J, Yue JB. Current status and advances in arginine-glycine-aspartic acid peptide-based molecular imaging to evaluate the effects of anti-angiogenic therapies. Precision Radiation Oncology. (2019) 3(1):29–34. doi: 10.1002/pro6.60
67. Kumar S, Rayamajhi S, Shukla J, Vatsa R, Mittal B. Performance of ga-68 DOTA RGD PET/CT for detecting lymph nodal and distant metastasis in breast cancer: a comparative study with F-18 FDG PET/CT. J Nucl Med. (2015) 56(Suppl 3):568.
68. Wu J, Wang S, Zhang X, Teng Z, Wang J, Yung BC, et al. 18F-alfatide II PET/CT for identification of breast cancer: a preliminary clinical study. J Nucl Med. (2018) 59(12):1809–16. doi: 10.2967/jnumed.118.208637
Keywords: theranostics, breast cancer, RLT/radioligand therapy, targeted radionuclide therapy, FAPI, CXCR4
Citation: Vorster M, Hadebe BP and Sathekge MM (2023) Theranostics in breast cancer. Front. Nucl. Med. 3:1236565. doi: 10.3389/fnume.2023.1236565
Received: 7 June 2023; Accepted: 21 July 2023;
Published: 4 August 2023.
Edited by:
Egesta Lopci, University of Milan, ItalyReviewed by:
Lurdes Gano, Centro de Ciências e Tecnologias Nucleares (C2TN), PortugalJianguo Lin, Jiangsu Institute of Nuclear Medicine, China
© 2023 Vorster, Hadebe and Sathekge. This is an open-access article distributed under the terms of the Creative Commons Attribution License (CC BY). The use, distribution or reproduction in other forums is permitted, provided the original author(s) and the copyright owner(s) are credited and that the original publication in this journal is cited, in accordance with accepted academic practice. No use, distribution or reproduction is permitted which does not comply with these terms.
*Correspondence: M. Vorster, Vm9yc3Rlck0xQHVrem4uYWMuemE=; bWFyaXphdm9yc3RlckBnbWFpbC5jb20=
†ORCID:
M. Vorster
orcid.org/0000-0001-5643-1553
M. M. Sathekge
orcid.org/0000-0002-2806-0625