- 1Department of Biomedical Sciences, University of Teramo, Teramo, Italy
- 2European Center for Brain Research/Santa Lucia Foundation, Rome, Italy
Two main molecular targets of Δ9-tetrahydrocannabinol (Δ9-THC), the psychoactive principle of Cannabis sativa, are type-1 (CB1) and type-2 (CB2) cannabinoid receptors (Howlett et al., 2010). In the past few years many endogenous agonists of CB receptors have been characterized, and are collectively called “endocannabinoids” (Maccarrone et al., 2010). They are mainly amides and esters of long-chain polyunsaturated fatty acids isolated from brain and peripheral tissues and, although structurally different from plant cannabinoids, share critical pharmacophores with Δ9-THC (Pertwee, 2010). Two arachidonate derivatives, N-arachidonoylethanolamine (anandamide, AEA) and 2-arachidonoylglycerol (2-AG), were shown to mimic Δ9-THC by functionally activating CB receptors, and these are the endocannabinoids whose biological activity has been best characterized to date (Di Marzo, 2009; Maccarrone et al., 2010).
CB1 receptor is the most abundant G protein-coupled receptor (GPCR) in the brain (Howlett et al., 2010). Together with its endogenous agonists (AEA, 2-AG, and other congeners), CB1 belongs to an ancient neurosignaling system that plays important control functions within the central nervous system (Katona and Freund, 2008). Alterations in this so-called “endocannabinoid system” have been extensively investigated in a wide range of neurodegenerative and neuroinflammatory disorders, spanning from Alzheimer’s disease, Parkinson’s disease and Huntington’s disease, to amyotrophic lateral sclerosis and multiple sclerosis (Bisogno and Di Marzo, 2010). For this reason, research on the therapeutic potential of drugs modulating the endocannabinoid system is very intense (Di Marzo, 2009). More recently, it has become evident the involvement of membrane lipids, especially cholesterol and glycosphingolipids, in regulating the function of GPCRs like β2-adrenergic and serotonin1A receptors, as well as of several other membrane-associated proteins like caveolins (Pontier et al., 2008; Prinetti et al., 2009; Paila et al., 2010; Shrivastava et al., 2010). Also a role for membrane cholesterol in the functional regulation of CB1 has been well-documented (for an updated review see Dainese et al., 2010). Acute cholesterol depletion by methyl-β-cyclodextrin has been shown to double CB1-dependent signaling via adenylyl cyclase and mitogen-activated protein kinases in neuronal cells (Bari et al., 2005a,b). Conversely, it has been reported that in the same cells CB1-dependent binding and signaling was significantly reduced by cholesterol enrichment (Bari et al., 2005a,b, 2006). Notably, the CB2 receptor that is structurally and functionally related to CB1 is completely insensitive to the modulation of membrane cholesterol content (Bari et al., 2006), and does not reside in cholesterol-rich microdomains like lipid rafts (Bari et al., 2006; Rimmerman et al., 2008). As yet, the molecular basis for the different response of these two receptor subtypes to cholesterol remains unclear, although its impact on the therapeutic exploitation of CB1-dependent endocannabinoid signaling versus that dependent on CB2 could be immense.
Here, I would like to comment that subtle, yet specific, differences might underpin the differential sensitivity of CB1 and CB2 to membrane cholesterol, possibly explaining the apparent redundancy of having two largely overlapping receptor subtypes that are activated by similar compounds (endocannabinoids) and trigger similar transduction pathways: (i) inhibition of adenylyl cyclase, (ii) regulation of ionic currents (e.g., inhibition of voltage-gated L, N, and P/Q-type Ca2+ channels, and activation of K+ channels), and (iii) activation of focal adhesion kinase, mitogen-activated protein kinase, and cytosolic phospholipase A2 (Di Marzo, 2009; Maccarrone et al., 2010).
In general, cholesterol may act on the conformation of a membrane receptor by indirectly altering the physico-chemical properties of the bilayer, or by directly interacting with the receptor itself. Although a unique conserved structural determinant for protein interaction with cholesterol has not yet been identified, a well-known motif is the cholesterol interaction/recognition amino acid sequence consensus [L/V-X(1–5)-Y-X(1–5)-R/K], named CRAC (Epand, 2006). This motif has been demonstrated in caveolin-1, peripheral-type benzodiazepine receptor (Li and Papadopoulos, 1998; Jamin et al., 2005), and in other proteins targeted to lipid rafts (Xie et al., 2010). Interestingly, by sequence alignment of human CB1 and CB2 we have recently identified the presence of CRAC in the last 11 amino acids of the transmembrane helix 7 of both CB1 and CB2 (Oddi et al., 2011). In particular, we found that in the highly conserved CRAC region (82% amino acid identity), CB1 differs from CB2 for one residue only: lysine 402 of CB1 (Figure 1) corresponds to glycine 304 in CB2 (Oddi et al., 2011). We also found that the CB1(K402G) mutant where the CRAC sequence of CB1 was converted into that of CB2 had a reduced propensity to reside in cholesterol-rich membrane regions, and lost its sensitivity to membrane cholesterol enrichment (Oddi et al., 2011). Therefore, one residue in complex proteins like GPCRs can be enough to direct their interaction with membrane lipids, thus affecting signal transduction thereof.
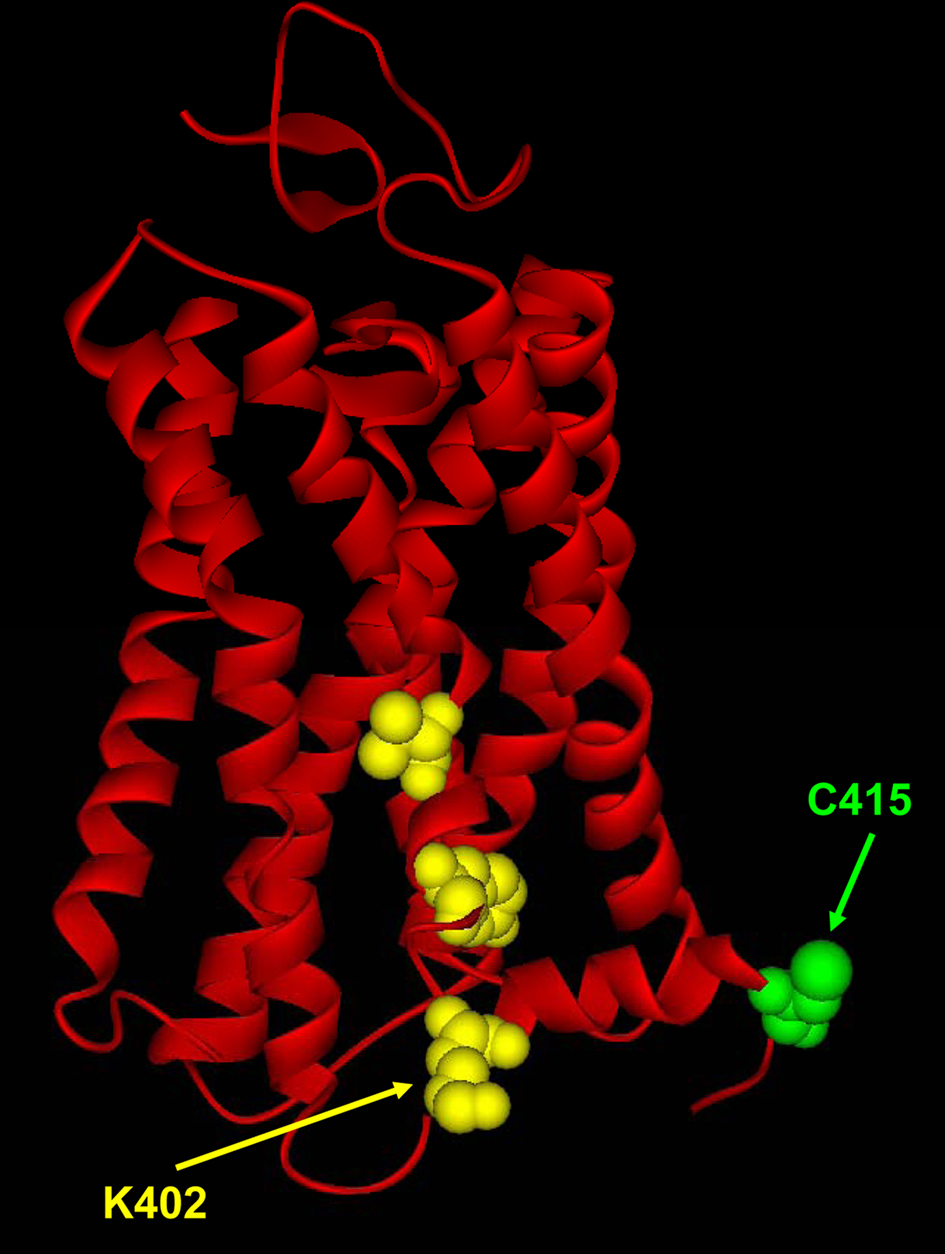
Figure 1. Three-dimensional model of CB1, based on sequence alignment with visual rhodopsin in the inactivated state (PDB code: 1F88). The model was obtained using the protein structure homology-modeling server SWISS-MODEL, integrated in the Deep-View program (Dainese et al., 2010). The three residues (V392, Y397, K402) that form the CRAC sequence are represented as yellow spheres, sized to the Van der Waals radii; these residues belong to the transmembrane helix 7 of CB1. Recently, we have generated a mutant where a lysine residue (K402) was substituted by glycine, thus converting the CRAC sequence of CB1 into that of CB2 (Oddi et al., 2011). Additionally, the C-terminal component of CB1, i.e., the intracellular juxtamembrane α-helix 8, contains a cysteine residue (C415, in green) that could be constitutively palmitoylated. See text for details. The model was kindly provided by Dr. Enrico Dainese (University of Teramo, Italy).
Different non-mutually exclusive mechanisms could be proposed to explain the differential sensitivity of CB1 and CB2 to membrane cholesterol: (i) compartmentalization in cholesterol-rich microdomains; (ii) caveolar endocytosis; (iii) cholesterol-dependent receptor dimerization; (iv) hydrophobic mismatch; (v) modulation of the rate of endocannabinoid movement within the membrane (Dainese et al., 2010). Additionally, it is possible that the different effect of membrane cholesterol on CB1 and CB2 is due to subtle differences in the domain(s) that interact(s) with the surrounding (non-annular) lipids, by analogy with other GPCRs (Paila et al., 2010). Additionally, other lipid-interacting residues might direct the interaction of CB1 with the surrounding membrane lipids, e.g., cysteine 415 in its C-terminal (Figure 1), that could be the target of palmitoylation (Dainese et al., 2010). The latter reversible post-translational modification can be used by cells to regulate CB1 targeting to cholesterol-rich subdomains of the membrane, thus influencing its interaction with coupled G proteins.
I believe that the comparison between CB1 and CB2 might represent an interesting paradigm that goes well-beyond endocannabinoid signaling. In fact, the modulation of CB1 by cholesterol might disclose a novel ligand–receptor interaction, where a third player comes into the game: membrane lipids. As a consequence, the membrane environment might play a role in receptor-dependent signaling, with a potential impact on several neurotransmission pathways, as well as several neurodegenerative/neuroinflammatory diseases where CB1 is known to play a role. More in general, it should be recalled that CB1-dependent signaling impacts fundamental processes as different as immune response, energy homeostasis, reproduction, and skin differentiation (Di Marzo, 2009; Maccarrone et al., 2010), thus it can be anticipated that cholesterol-dependent regulation of CB1 can have a physiological relevance well-beyond the central nervous system.
In conclusion, membrane environment seems to be critical for the regulation of signal transduction pathways triggered by G protein-coupled receptors like CB1. Despite the three-dimensional complexity of these proteins, we learn from the comparison of CB1 with CB2 that just one amino acid residue can direct receptor functioning, calling for attention on the plasma membrane as a key-player in ligand recognition on the cell surface.
Acknowledgments
Financial support from Ministero dell’Istruzione, dell’Università e della Ricerca (PRIN 2008 grant), and from Fondazione TERCAS (grant 2009-2012) is gratefully acknowledged.
References
Bari, M., Paradisi, A., Pasquariello, N., and Maccarrone, M. (2005a). Cholesterol-dependent modulation of type 1 cannabinoid receptors in nerve cells. J. Neurosci. Res. 81, 275–283.
Bari, M., Battista, N., Fezza, F., Finazzi-Agrò, A., and Maccarrone, M. (2005b). Lipid rafts control signaling of type-1 cannabinoid receptors in neuronal cells. Implications for anandamide-induced apoptosis. J. Biol. Chem. 280, 12212–12220.
Bari, M., Spagnuolo, P., Fezza, F., Oddi, S., Pasquariello, N., Finazzi-Agrò, A., and Maccarrone, M. (2006). Effect of lipid rafts on Cb2 receptor signaling and 2-arachidonoyl-glycerol metabolism in human immune cells. J. Immunol. 177, 4971–4980.
Bisogno, T., and Di Marzo, V. (2010). Cannabinoid receptors and endocannabinoids: role in neuroinflammatory and neurodegenerative disorders. CNS Neurol. Disord. Drug Targets (e-published on July 16).
Dainese, E., Oddi, S., and Maccarrone, M. (2010). Interaction of endocannabinoid receptors with biological membranes. Curr. Med. Chem. 17, 1487–1499.
Di Marzo, V. (2009). The endocannabinoid system: its general strategy of action, tools for its pharmacological manipulation and potential therapeutic exploitation. Pharmacol. Res. 60, 77–84.
Epand, R. M. (2006). Cholesterol and the interaction of proteins with membrane domains. Prog. Lipid Res. 45, 279–294.
Howlett, A. C., Blume, L. C., and Dalton, G. D. (2010). CB1 cannabinoid receptors and their associated proteins. Curr. Med. Chem. 17, 1382–1393.
Jamin, N., Neumann, J. M., Ostuni, M. A., Vu, T. K., Yao, Z. X., Murail, S., Robert, J. C., Giatzakis, C., Papadopoulos, V., and Lacapere, J. J. (2005). Characterization of the cholesterol recognition amino acid consensus sequence of the peripheral-type benzodiazepine receptor. Mol. Endocrinol. 19, 588–594.
Katona, I., and Freund, T. F. (2008). Endocannabinoid signaling as a synaptic circuit breaker in neurological disease. Nat. Med. 14, 923–930.
Li, H., and Papadopoulos, V. (1998). Peripheral-type benzodiazepine receptor function in cholesterol transport. Identification of a putative cholesterol recognition/interaction amino acid sequence and consensus pattern. Endocrinology 139, 4991–4997.
Maccarrone, M., Gasperi, V., Catani, M. V., Diep, T. A., Dainese, E., Hansen, H. S., and Avigliano, L. (2010). The endocannabinoid system and its relevance for nutrition. Annu. Rev. Nutr. 30, 423–440.
Oddi, S., Dainese, E., Fezza, F., Lanuti, M., Barcaroli, D., De Laurenzi, V., Centonze, D., and Maccarrone, M. (2011). Functional characterization of putative cholesterol binding sequence (CRAC) in human type-1 cannabinoid receptor. J. Neurochem. (in press).
Paila, Y. D., Ganguly, S., and Chattopadhyay, A. (2010). Metabolic depletion of sphingolipids impairs ligand binding and signaling of human serotonin1A receptors. Biochemistry 49, 2389–2397.
Pertwee, R. G. (2010). Receptors and channels targeted by synthetic cannabinoid receptor agonists and antagonists. Curr. Med. Chem. 17, 1360–1381.
Pontier, S. M., Percherancier, Y., Galandrin, S., Breit, A., Gales, C., and Bouvier, M. (2008). Cholesterol-dependent separation of the beta2-adrenergic receptor from its partners determines signaling efficacy: insight into nanoscale organization of signal transduction. J. Biol. Chem. 283, 24659–24672.
Prinetti, A., Loberto, N., Chigorno, V., and Sonnino, S. (2009). Glycosphingolipid behaviour in complex membranes. Biochim. Biophys. Acta 1788, 184–193.
Rimmerman, N., Hughes, H. V., Bradshaw, H. B., Pazos, M. X., Mackie, K., Prieto, A. L., and Walker, J. M. (2008). Compartmentalization of endocannabinoids into lipid rafts in a dorsal root ganglion cell line. Br. J. Pharmacol. 153, 380–389.
Shrivastava, S., Pucadyil, T. J., Paila, Y. D., Ganguly, S., and Chattopadhyay, A. (2010). Chronic cholesterol depletion using statin impairs the function and dynamics of human serotonin1A receptors. Biochemistry 49, 5426–5435.
Citation: Maccarrone M (2010) Membrane environment and endocannabinoid signaling. Front. Physio. 1:140. doi: 10.3389/fphys.2010.00140
Received: 06 October 2010;
Accepted: 06 October 2010;
Published online: 29 October 2010.
Copyright: © 2010 Maccarrone. This is an open-access article subject to an exclusive license agreement between the authors and the frontiers research foundation, which permits unrestricted use, distribution, and reproduction in any medium, provided the original authors and source are credited.
*Correspondence: mmaccarrone@unite.it