- 1 Department of Biology, University of Akron, Akron, OH, USA
- 2 Program in Integrated Bioscience, University of Akron, Akron, OH, USA
All organisms face tradeoffs with regard to how limited energy resources should be invested. When is it most favorable to grow, to reproduce, how much lipid should be allocated to storage in preparation for a period of limited resources (e.g., winter), instead of being used for growth or maturation? These are a few of the high consequence fitness “decisions” that represent the balance between energy acquisition and allocation. Indeed, for animals to make favorable decisions about when to grow, eat, or reproduce, they must integrate signals among the systems responsible for energy acquisition, storage, and demand. We make the argument that leptin signaling is a likely candidate for an integrating system. Great progress has been made understanding the leptin system in mammals, however our understanding in fishes has been hampered by difficulty in cloning fish orthologs of mammalian proteins and (we assert), underutilization of the comparative approach.
Leptin as an Integrating Signal in Mammals
Although many peptides are involved in the interplay among appetite, metabolic rate, and energy stores, leptin is arguably the best understood candidate for a central regulating system. Leptin was first cloned in ob/ob mice in 1994 (Zhang et al., 1994). These mice do not express leptin as a result of a homozygous mutation of the ob gene. They are chronically obese, hyperphagic, hypothermic, and do not sexually mature; injections of leptin rescues all of these phenotypes. Leptin has demonstrated effects in regulating appetite, fat metabolism, reproduction, body temperature, growth, bone remodeling, immune function, stress, inflammatory responsiveness, hematopoiesis, and angiogenesis (Pelleymounter et al., 1995; Barash et al., 1996; Bennett et al., 1996; Grunfeld et al., 1996; Fruhbeck et al., 1997; Sierra-Honigmann et al., 1998; Steppan et al., 2000; Dagogo-Jack et al., 2005; Lafrance et al., 2010; and reviewed in Swoap, 2008; Friedman, 2009). Its pleiotropic effects and its potential for treating obesity have resulted in >28,000 published studies to date (ISI).
In mammals, leptin is synthesized by adipose tissue and secreted into the blood. As adipose stores grow, leptin expression and serum concentrations increase in proportion to total fat stores (Maffei et al., 1995; Considine et al., 1996), although different types of adipose tissue differ in their signaling amplitude (Cnop et al., 2002). Leptin binds to leptin receptors in the hypothalamus, which signal through JAK–STAT pathways to alter transcription and affect phenotype (Tartaglia, 1997). When adipose stores are depleted, circulating leptin titer drops and the effect on phenotype is reversed (e.g., high leptin attenuates appetite and low leptin stimulates appetite; Zhang et al., 1994). Ahima and Flier (2000) proposed that leptin evolved as a starvation signal; low lipid reserves lead to low leptin titers, which communicates to the central nervous system that energy is unavailable for processes that are not life sustaining (e.g., low body fat human females can become infertile, which can be successfully treated with recombinant leptin; LaMarca and Volpe, 2004). The earlier an organism can sense the starvation event, the more energy it can save by ramping down leptin-stimulated functions, and the better its chances for surviving the event. In concordance with its ability to affect such a diversity of processes, leptin interacts with a diversity of hormones and signaling peptides (Figure 1). It appears that chronic stimulation of leptin secretion is mediated by molecules such as glucose, insulin, sex steroids, and glucocorticoids (Larsson and Ahren, 1996; Elbers et al., 1997; Newcomer et al., 1998; Moreno-Aliaga et al., 2001), suggesting that these hormones may be involved in directly regulating serum leptin concentrations (Figure 1; Laferrere et al., 2002; Lee and Fried, 2009).
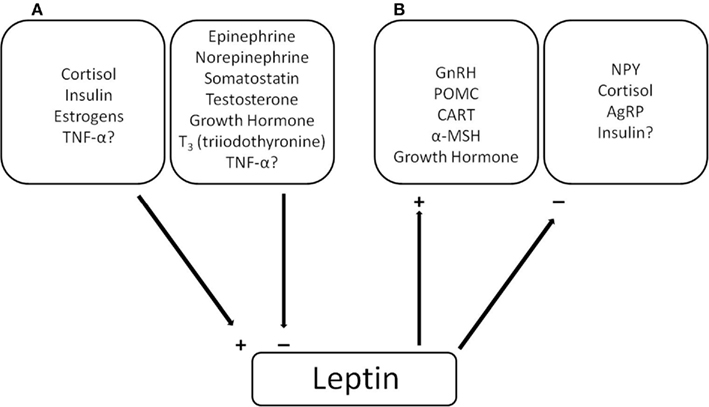
Figure 1. (A) The effects of various peptides/hormones on leptin expression/secretion in mammals. (B) The effect of leptin on the expression/secretion of various peptide/hormones. The + and − indicate stimulatory and inhibitory effects respectively. Findings are reviewed in Rayner and Trayhurn (2001) and Reidy and Weber (2000).
Comparative Studies in Mammals
In focusing on the role of leptin in obesity, considerably less research effort is directed at determining leptin’s role in natural, non-model, and non-pathological systems, and how its function is either conserved or not among diverse taxa over evolutionary time. The few exceptions are exemplary for the insight they give into the evolution of energy sensing systems, and into leptin signaling itself. Mammals that hibernate or go through daily torpor typically experience temporary leptin resistance (Kronfeld-Schor et al., 2000; Clarke et al., 2001; Rousseau et al., 2003). This is interpreted as adaptive, for if the appetite-suppressing functions of leptin were operating during pre-hibernation fattening, the mammal would not be able to accumulate sufficient fat stores to sustain them through hibernation. In the same theme of adaptation to low-resource environments, Yang et al. (2008) argued that pikas (small, Tibetan plateau lagomorphs) had undergone adaptive evolution in the pika leptin gene. Pika leptin is more divergent than closely related leptins, and pikas express high titers of leptin, which the authors argue is essential to adaptation to both hypoxia and low temperature. The authors asserted that these animals manipulated leptin resistance in adapting to their environment. Leptin resistance is something of great interest to a pharmaceutical industry interested in reducing leptin resistance in obese humans. Thus a comparative approach to studying leptin function can lead to insight not readily available when studying model species exclusively.
What Aspects of Leptin Function are Conserved between Fish and Mammals?
Although cloning leptin in multiple mammalian species progressed rapidly, identifying an orthologous leptin gene among ectotherms did not occur until 11 years after leptin was first identified in mice. Our group first suggested that fish express leptin by documenting a leptin-immunoreactive protein (Johnson et al., 2000), and Kurokawa first cloned leptin from Fugu via gene synteny. After Kurokawa’s seminal discovery, it was obvious why leptin was so difficult to find. Fugu leptin’s primary structure is only 13% identical to human leptin (Kurokawa et al., 2005; Figures 2 and 3). However, threading algorithms that use the carbon backbone of the human-leptin crystal structure (Zhang et al., 1997) predict that the tertiary structures of fish leptins are very similar to mammalian leptins (Kurokawa et al., 2005; Huising et al., 2006; Gorissen et al., 2009; Figure 4). One difference in fish leptin biology is its lack of expression in adipose tissue. Fish leptin mRNA is expressed primarily in liver (Takifugu, Kurokawa et al., 2005; Oryzias, Kurokawa and Murashita, 2009; Oncorhynchus, Murashita et al., 2008; Danio, Gorissen et al., 2009; Cyprinus, Huising et al., 2006), with only a single report of weak, transient leptin expression in fish adipose tissue (Pfundt et al., 2009). For most fish species, liver is the highest leptin-expressing tissue, although gonad may be highest in zebrafish (Gorissen et al., 2009).
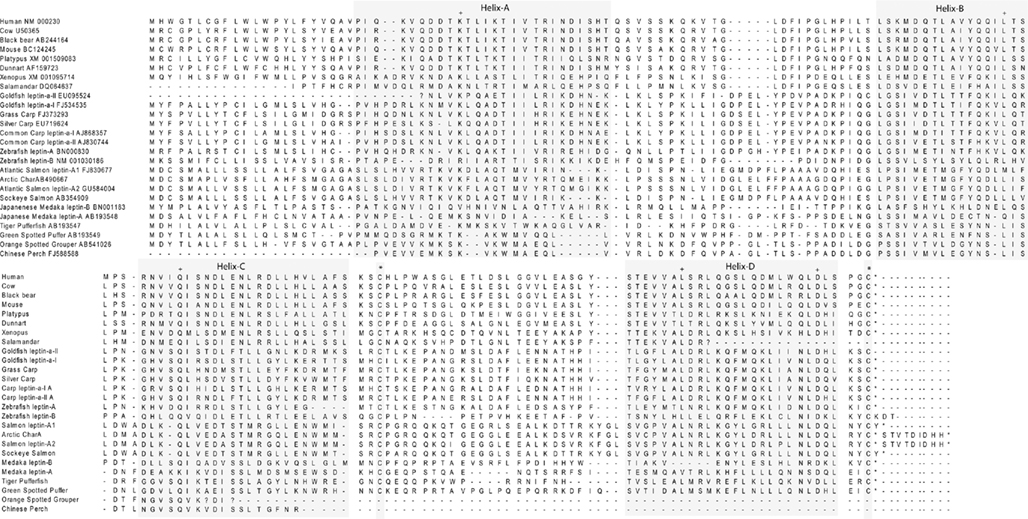
Figure 2. Multiple sequence alignment of all fish leptin sequences entered in public databases, excluding duplicate sequences. Accession numbers are listed alongside taxa in the first row of sequence. Initial alignment of amino acid sequences was performed in ClustalW with additional manual alignment informed by prior work of Gorissen et al. (2009) to identify four alpha-helices (A–D). Cysteine residues reported to form leptin’s disulfide bridge are shaded and indicated by an asterisk. Other residues with greater than 90% conservation are indicated by a plus sign.
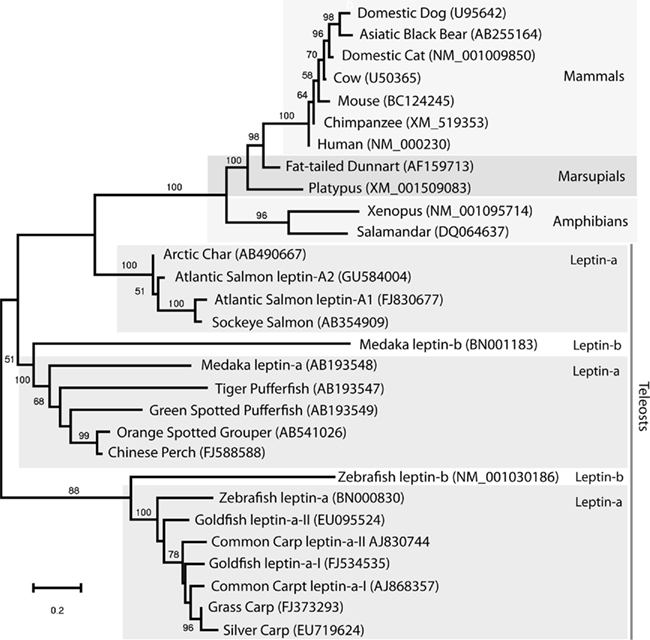
Figure 3. Evolutionary history of leptin inferred using the Maximum Likelihood method based on the JTT matrix-based model (log likelihood −5574.1124) conducted in MEGA4 (Tamura et al., 2007). The initial unrooted tree for the heuristic search was constructed using maximum parsimony. Representative vertebrate leptins including all available fish leptin amino acid sequences (excluding duplicate sequences and the 5′ to Helix-A) were used for analysis. Numbers at nodes represent support based on 500 bootstrap replications. Lack of bootstrap value indicates less then 50% support at that node. GenBank accession numbers are in parentheses. Leptin paralog names follow those of Gorissen et al. (2009).
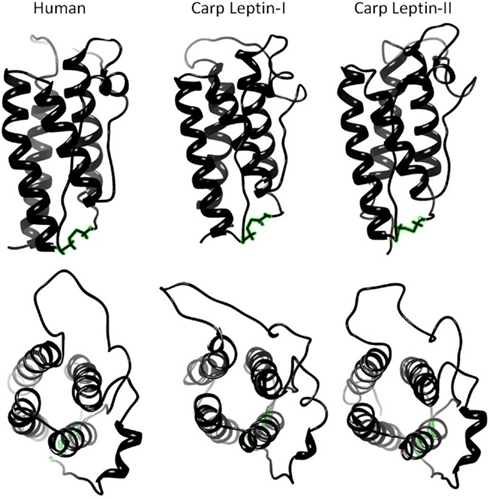
Figure 4. Threading models of human and carp leptins showing characteristic four-helix bundle and disulfide bond. Models were predicted using LOMETS (Wu and Zhang, 2007) threading server and energy minimized (with disulfide bonds) using YASARA (www.yasara.org) with AMBER03 force field (Duan et al., 2003).
Since the first fish leptin gene was described, fish leptin have been cloned from several species (Figure 2), and the molecular tools necessary to understand leptin function in fishes are now at hand. Soon after, amphibian leptins were cloned and characterized (Boswell et al., 2006; Crespi and Denver, 2006), and there appears to be a genuine leptin sequence in the green anole genome (Boorse and Libbon, 2010). A bird leptin was first reported in 1998 (Taouis et al., 1998) for chicken but several investigators have been unable to replicate their results (Friedman-Einat et al., 1999; Amills et al., 2003) and no leptin gene has been identified in sequenced bird genomes, although the leptin receptor for chicken and other birds has been identified without debate (Horev et al., 2000). One group went so far as to call for a ban on publishing studies claiming to have measured leptin in birds (Sharp et al., 2008). Unlike land animals which apparently have only a single copy of the leptin, duplicate leptin genes have been identified for Atlantic Char, Japanese medaka, common carp, zebrafish, and goldfish (Rønnestad et al., 2010). Phylogeny reconstruction (Figure 3) supports a hypothesis (Gorissen et al., 2009) of ancient duplication of leptin (leptin-a and leptin-b) after the split from land vertebrates followed by at least one additional later genome duplication resulting in duplicate leptin-a sequences. Gorissen et al. (2009) argue that mammalian leptins cluster with leptin-b, based upon sequence alignment, gene structure, and genome structure (synteny with mammalian leptins). However, we argue that assigning the fish ortholog to mammalian leptins is not possible at this time. Figure 3 illustrates that although some sequences within fish leptins form well-supported clades (e.g., goldfishes and carps, salmonids), in general there is a polytomy among fish leptins, with “a” and “b” isoforms intermixed within clades, and no clearly identifiable fish ortholog to mammalian leptins. Another recent review addresses the relationship among fish leptins and also concludes that there is a polytomy among non-mammal leptins (Vissers et al., 2010). Additional sampling of fish genomes will be required to sort out competing phylogenetic hypotheses regarding the evolution of leptin genes in fish. Further, other characters (e.g., gene structure for all fish species, physiological data on the behavior of leptin-a vs. b) mapped onto the tree may resolve which fish leptin gave rise to mammalian leptins. If the isoforms differ in their physiological function (a focus of our current investigations), identifying the fish ortholog will be an important clue as to the origin of leptin function in mammals. Although other vertebrates may be better candidates for studying this aspect of leptin’s evolutionary history, no leptin sequences are published for reptiles or birds, and few exist for amphibians. Once several clones are available for taxa between fish and mammals it will likely be possible to resolve if the mammalian isoform has a single origin among lower vertebrates or multiple origins (followed by secondary loss). Until then the fish sequences are the best data available for this important question.
Although identifiably distinct in primary structure, it is possible that all fish leptin isoforms are similar in function, given that tertiary structure is generally conserved (Figure 4). We assert that understanding the evolution of leptin function will pay great dividends in understanding mammalian leptins, and that fishes are the logical organisms to study, and thus the potential exists for conserved function among vertebrates. Because the diversity of non-mammal leptins is (currently) greatest among fishes, this review will focus on leptin’s role in fishes.
Reproduction
One of leptin’s most established roles in mammals (and most effective use as a treatment) appears to be promoting reproduction. Early studies in female ob/ob mice, which are normally infertile, showed that recombinant leptin can stimulate ovulation, ultimately facilitating pregnancy and parturition (Chehab et al., 1996). Physiologically, leptin stimulates the hypothalamic–pituitary–gonadal axis by promoting the release of gonadotropin releasing hormone (GnRH) from the hypothalamus, and luteinizing hormone (LH) and follicle-stimulating hormone (FSH) from the pituitary leading to a cascade of hormonal responses that promote reproduction (Yu et al., 1997; Watanobe, 2002). Leptin also causes ovarian and uterine mass to increase in female mice, and in males leptin injections increase seminal vesicle and testis mass (Barash et al., 1996). Conversely, women who are deficient in leptin either from genetic abnormalities, eating disorders, or hyper-athleticism show irregular or absence of menstruation (Friedman, 2009). Leptin is also implicated to be one of the permissive factors in the onset of puberty (Fernandez-Fernandez et al., 2006); children with higher body fat enter puberty sooner and have higher serum leptin concentrations (Aksglaede et al., 2009).
In fishes, leptin also appears to stimulate the reproductive axis. Leptin receptor mRNA is expressed in the hypothalamic lateral tuberal nucleus (the fish homolog of the arcuate nucleus in mammals), and also expressed at high levels in zebrafish gonad (Liu et al., 2010). Administration of mammalian leptin led to increases in LH and somatolactin in sea bass (Peyon et al., 2001, 2003). Additionally, in vitro studies using human leptin caused increased release of FSH and LH from the pituitary of female trout (Weil et al., 2003). Recently, a temporal study of Arctic charr (Salvelinus alpinus) noted that higher leptin expression was apparent during the months when body lipids were declining in accordance with the onset of sexual maturity (Frøiland et al., 2010). However, to date there have been no studies in fishes using species-specific leptin to test its effects on the reproductive axis. This is likely to be addressed soon, given the availability of recombinant leptin for many fish species.
Food Intake and Fasting
Perhaps the most extensively studied aspect of leptin in mammals is its ability to modulate food intake and consequently contribute to body weight regulation. As with many leptin deficiency-related pathologies, administration of recombinant leptin has corrective effects and reduces food intake and body mass in ob/ob mice (Weigle et al., 1995, reviewed in Ahima and Flier, 2000; Smith and Ferguson, 2008; and Arora and Arora, 2008). This work established that leptin’s anorexigenic (appetite inhibiting) effects originate in the arcuate nucleus of the hypothalamus. Here leptin suppresses the orexigenic (appetite-stimulating) peptides, agouti-related peptide (AgRP), and neuropeptide Y (NPY), while stimulating the anorexigenic peptides, α-melanocyte stimulating hormone (α-MSH) and cocaine and amphetamine-related transcript (CART). Stimulated neurons in this region may also project into the lateral hypothalamus to decrease the expression of the potent orexigen, melanin-concentrating hormone (MCH). Additionally, leptin’s role in satiety is supplemented by the actions of the gut hormone cholecystokinin (CCK), and complemented by ghrelin, which both respond to the presence of nutrients and/or gut distension (Bado et al., 1998). Fasting causes rapid decreases in serum leptin before fat mass decreases and so reverses the processes detailed above to conserve energy and stimulate appetite (Boden et al., 1996; Ahima, 2000; Swoap et al., 2006; Zhan et al., 2009).
A lipostatic model for food intake regulation has been proposed for fishes, and like mammals, suggests that they can sense the amount of lipid stores present and modulate feeding behavior accordingly (Johansen et al., 2002). Indeed, attributes such as growth and maturity are strongly related to lipid stores (Fauconneau et al., 1995; Jonsson and Jonsson, 2005), however less is known about the regulatory effects of lipids on food intake. In fishes, leptin’s role as a satiety signal is not dogma as it is in mammals, for studies show either limited or no correlation between leptin concentration and food intake. In common carp, 6 weeks of feeding to satiation or fasting did not alter leptin mRNA expression in hepatocytes (Huising et al., 2006). This was true even though the fasted fish lost ∼30% of their initial body mass after 6 weeks. Similarly, overfed zebrafish had no significant change in leptin mRNA expression, despite significant changes in body mass index (Oka et al., 2010). Injections of mammalian leptin in coho salmon (Oncorhynchus kisutch) or green sunfish (Lepomis cyanellus) did not affect food intake and/or body weight (Baker et al., 2000; Londraville and Duvall, 2002). In goldfish (Carassius auratus), intraperitoneal injections of human leptin for 10 days caused a reduction in food intake and relative body weight at doses of 1 μg leptin/g body weight (de Pedro et al., 2006). No changes were observed in the hypothalamic content of the orexigenic peptide, NPY. Both the hyperphysiological dose and heterologous leptins used in these studies make them difficult to interpret. Human and fish leptins show very poor sequence conservation (∼25%) and near pharmacological doses of leptin were needed to elicit an anorexigenic response. Consequently, the results may not accurately reflect how native leptin functions in this species. Studies using species-specific leptin found that injections reduced food intake and NPY expression significantly in rainbow trout (Oncorhynchus mykiss) and grass carp (Ctenopharyngodon idellus) although the effect was only transient, lasting less than 1 day (Murashita et al., 2008; Li et al., 2010). As with mammals, appetite in these animals also appears to be regulated by several of the peptide hormones mentioned previously for mammals; for which many have been identified in non-mammalian vertebrates (see Gorissen et al., 2006; Vissers et al., 2010 for reviews).
Fat Metabolism
One of the more direct roles of leptin in energy balance is the stimulation of lipolytic pathways. In mammals, leptin promotes lipolysis and inhibits insulin-stimulated lipogenesis (Cohen et al., 2002, and reviewed in Reidy and Weber, 2000). This effect can also be observed indirectly via an increase in plasma non-esterified fatty acids (NEFA) after leptin injection (Ajuwon et al., 2003). Moreover, in promoting lipid-based metabolism, leptin modulates the transition from carbohydrate to lipid oxidation (Hwa et al., 1997). For example, leptin inhibits the expression of acetyl CoA carboxylase in adipocytes; an essential enzyme for the conversion of carbohydrates to triacylglycerols (Bai et al., 1996). There is also evidence for a negative feedback loop, where uptake of fatty acids appears to attenuate leptin secretion. Fatty acid uptake inhibits leptin release from adipocytes in vitro (Shintani et al., 2000; Cammisotto et al., 2003), and mice with impaired fatty acid uptake have higher serum leptin concentrations (Hajri et al., 2007). Importantly, leptin’s lipolytic effects can occur independently of endocrine signaling to the hypothalamus. Fruhbeck et al. (1997) used cultured adipocytes and showed that leptin increased lipolytic rate, with no neural or adrenergic influence. This suggests that leptin may participate in autocrine or paracrine signaling which is further supported by the observation that both leptin and leptin receptor may be expressed in adipocytes, brain, kidney, liver, muscle, pancreas, placenta, and adrenals (Lollmann et al., 1997; Rayner and Trayhurn, 2001).
Leptin’s role in the fat metabolism of fishes remains to be characterized. In humans and many other mammals, lipids are stored primarily in adipose tissue and significant deposition in liver is considered pathological (steatosis, McCullough, 2006). In most fishes triacylglycerols (TAG) are stored in muscle and liver in addition to adipose tissue dispersed viscerally, cephalically, and subcutaneously (Henderson and Tocher, 1987; Fauconneau et al., 1991). A few studies are consistent with leptin’s role in promotion of lipolysis while decreasing lipogenesis. Ten days of human-leptin injections decreased hepatic lipid content in goldfish (de Pedro et al., 2006) and mouse-leptin injections increased fatty acid-binding protein and carnitine palmitoyl transferase in green sunfish (Londraville and Duvall, 2002). In grass carp, leptin acutely reduced liver expression of stearoyl CoA desaturase-1, an enzyme critical for the biosynthesis of monounsaturated fatty acids (Li et al., 2010). Interestingly, most of leptin’s effects on fat metabolism-related gene expression in grass carp were acute, including the increase in leptin expression that occurred on the first day of injections. The only effect that persisted through 13 days of injections was reduced hepatic lipoprotein lipase expression (LPL), a capillary enzyme that hydrolyzes TAGs of circulating lipoproteins (Li et al., 2010). Increased expression of LPL is also seen in the white adipose tissue of fasted mammals (Botion, 2001). Further, there is indirect evidence that leptin contributes to NEFA release into circulation. Fasted animals commonly have higher NEFAs (Dole, 1956; Bertile et al., 2003; Huising et al., 2006) and a recent study found that fasted trout had higher plasma leptin concentrations than fed individuals, although serum NEFAs were not measured (Kling et al., 2009). Finally, the anorexigen melanin stimulating hormone (MSH), which is stimulated by leptin in mammals, has strong lipolytic effects in trout (Yada et al., 2000). Although the relationship between leptin and MSH has not been investigated in fishes, it is a candidate hormone through which leptin can influence fat metabolism. Together, these data lead to the hypothesis that leptin contributes to the starvation-induced transition from carbohydrate to fat-based metabolism by stimulating mobilization of lipid stores.
Stress
The link between leptin and stress hormones is not well understood even in mammals, although the roles of both systems in influencing energy metabolism suggest a relationship. The best characterized stress hormones are the catecholamines (epinephrine and norepinephrine) of the sympathetic nervous system (SNS) and the terminal glucocorticoids of the hypothalamic–pituitary–adrenal (HPA) axis (e.g., cortisol and corticosterone). Cortisol stimulates leptin expression and secretion in humans (Dagogo-Jack et al., 1997; Newcomer et al., 1998; O’Conner et al., 2007) and may have secretagogue effects that exceed insulin (Askari et al., 2000). Additionally, inhibiting cortisol biosynthesis reduces serum leptin concentrations in humans (Dagogo-Jack et al., 2005), and leptin can inhibit cortisol secretion suggesting a feedback between these systems (Bornstein et al., 1997; Pralong et al., 1998). Leptin’s stimulation by cortisol has been suggested to act as a counter-regulatory response to prevent overeating and weight gain from the orexigenic actions of cortisol (Dagogo-Jack et al., 1997). It is possible that cortisol signals leptin to mobilize fatty acids as compensation for energetically demanding stress. Epinephrine and norepinephrine, released via the SNS, both have inhibitory effects on leptin expression and secretion (Kosaki et al., 1996; Mantzoros et al., 1996; Carulli et al., 1999). Leptin increases sympathetic outflow either directly by stimulating the synthesis and release of catecholamines (Takekoshi et al., 1999) or by stimulating regions in the brain that project to sympathetic preganglionic neurons (Dunbar et al., 1997; Elmquist et al., 1997), suggesting feedback between leptin and the SNS.
All of the above named stress hormones are connected to leptin through its effects on fat metabolism. Catecholamines, glucocorticoids, and leptin can all independently promote lipolysis, typically by activating lipolytic enzymes such as hormone sensitive lipase (HSL) and lipoprotein lipase (LPL; Kurpad et al., 1994; Samra et al., 1996a, b; Fruhbeck et al., 1997). Although this phenomenon has not been directly studied in fishes, there is indirect support for a relationship between stress hormones and leptin. Fasting increases cortisol in mammals (Chang et al., 2002; El-Migdadi et al., 2002). In fish, fasting can increase cortisol (Kelley et al., 2001; Barcellos et al., 2010), have no effect (Sumpter et al., 1991; Pottinger et al., 2003) or even result in decreased plasma cortisol (Small, 2005). Not surprisingly, the duration of the fast influences cortisol’s response. Peterson and Small (2004) found that cortisol peaked after 30 days in channel catfish (Ictalurus punctatus) but was not different from fed controls before or after that peak. As previously mentioned, both leptin and cortisol stimulate lipolysis and may contribute to the consistently observed increase in NEFAs during prolonged fasting (Dole, 1956; Pottinger et al., 2003; Huising et al., 2006). This relationship would have particular relevance for temperate-zone fishes, where overwinter fasting typically involves a switch to lipid-based ATP production, and cortisol may initiate this shift by stimulating leptin.
In addition to fasting, fishes commonly experience oxygen stress in their environment. Hypoxia can trigger a variety of responses, both at the behavioral and metabolic levels. Interestingly, leptin signaling responds to hypoxia exposure. In mammals, long-term hypoxia increases serum leptin and leptin receptor expression (Ducsay et al., 2006). In fishes, hypoxia triggers a marked upregulation of leptin expression (Chu et al., 2010) and leptin receptor expression (Wong et al., 2007). The physiological consequences of this response are unknown, but we offer the following speculation. Fibrinogen-α and hypoxia-inducible factor-1 (HIF-1) are upregulated in response to hypoxia, and both proteins directly or indirectly stimulate leptin and/or vascular endothelial growth factor (VEGF) expression, ultimately stimulating angiogenesis (Shiose et al., 2004; Marques et al., 2008). This suggests that leptin stimulation via fibrinogen-α/HIF-1 results in enhanced vascularization, which would be adaptive during hypoxia exposure.
Life History
Fish have dramatically lower metabolic rates than comparably sized endotherms and often continue to grow long after they reach sexual maturity; this suggests that regulation of energy balance is likely different than in mammals that are close to maximal size soon after becoming reproductive. Many species of fishes also differ from mammals in that they are polyploid. Carp are tetraploid and thus have a more diverse genome with which to respond to life history “decisions” (Figueroa et al., 2005; Huising et al., 2006). With increased gene copy number comes the potential for multiple isoforms of a protein, and diversity of function for that protein family. This adds another level of regulation and complexity, and represents another possible point of digression from the mammalian paradigm of energy balance.
Fishes face tradeoffs with respect to life history strategies just as all organisms do. These tradeoffs are inevitable given that resources such as food and mates are finite, and choosing to invest in one resource often means foregoing another. In fish aquaculture, it is well established that fishes which grow faster mature earlier; once matured they grow slower than their immature congeners (Felip et al., 2006, 2008). Immature Atlantic salmon possess much higher lipid densities and consequently mature earlier than brown trout (Jonsson and Jonsson, 2005). At maturity, however, salmon have lower lipid densities and two-fold greater gonadal energy content suggesting that the lipid energy was allocated to reproductive potential. Induction of triploidy (which causes sterility) results in fishes that grow faster, assumedly resulting from energy saved from reproduction (reviewed in Maxime, 2008). However, as noted by others (O’Flynn et al., 1997), triploidy often produces highly variable results with respect to growth performance and other measures of health due to the unpredictable phenotypes that result. While the mechanisms that control the timing and extent of processes such as growth and development are numerous (involving the stimulation and regulation of many physiological systems) and largely uncharacterized in fishes, it is reasonable to suspect that fishes and mammals might share the same basic signaling pathways.
Teleost Model of Leptin and Allostasis
Leptin has been described as an anti-obesity hormone, lipostat, satiety signal, starvation signal, and signal of energy sufficiency. These descriptions are in many respects all accurate, but tend to be situation dependent. We assert that leptin’s involvement in energy balance warrants a descriptor that recognizes its contribution to allostasis. The concept of allostasis is gaining appreciation in the field of stress physiology. It is a more inclusive variation of “homeostasis” and is based on the reasoning that animals must alter physiological processes in order to endure various challenges, so-called “stability through change” (McEwen, 2000). This process is highlighted by the adaptive changes that occur during stress responses, namely the activation of systems like the SNS and HPA in order to mobilize energy and survive whatever challenge initiated this metabolic cascade. We favor the viewpoint that leptin is an allostatic hormone, closely tied to the stress response and allostasis of vertebrates. Leptin acting centrally mediates different sets of responses than leptin acting peripherally. Central leptin acts through the hypothalamus where it stimulates energy consuming processes while inhibiting further energy intake; peripheral leptin can also interact with the hypothalamus but primarily binds to peripheral tissue receptors where it stimulates the liberation of lipid stores, in effect providing the energy for the systems it is stimulating. Catabolism of fat stores may also function to fuel the energy demands of stress.
As detailed above, cortisol stimulates but catecholamines inhibit leptin release. In a crude sense, catecholamines can be regarded as the quick response stress hormones, causing glucose to pour into the bloodstream to supply the “flight-or-fight” response. For such a response, it may be maladaptive to stimulate lipolysis through leptin; it is simply too slow and recruits an inappropriate energy substrate for rapid energy generation. However, sustained infusion of catecholamines does stimulate lipolysis in adipose tissue (Galton and Bray, 1967), which may be related to the gluconeogenic action of catecholamines in liver (Chu et al., 2003) and/or recruitment of leptin for longer-term stressors.
Cortisol release is more gradual and sustained than catecholamines (Chen et al., 2002; Barcellos et al., 2011). More enduring stressors that stimulate cortisol release would stimulate leptin release and lipolytic pathways. Fasting provides an example of long-term stress where our view of leptin as an allostatic hormone is more parsimonious than traditional functions assigned to leptin. During fasting, fishes commonly have elevated cortisol levels. Short-term fasting causes a rapid decrease in plasma leptin in mammals (Zhan et al., 2009), this may be a consequence of their costly metabolism (i.e., 24 h of fasting imparts more energetic detriment to endotherms than ectotherms). Moreover, a drop in circulating leptin does not preclude the maintenance or even elevation of local (autocrine/paracrine) signaling. Long-term fasting, such as in hibernating marmots (Marmota flaviventris), elevates serum leptin levels for several months (Florant et al., 2004). Food-restricted pregnant rats have elevated leptin levels compared to ad libitum fed rats (Jelks et al., 2010). Leptin concentrations are highest in lizards emerging from overwintering in Spring, and lowest as they add to fat bodies in the Fall in preparation for hibernation (Spanovich et al., 2006). Finally, overwintering arctic charr express steadily increasing serum leptin despite decreasing liver lipids and no food in their gut (Frøiland et al., 2010). Combined, these studies suggest that leptin’s role is to regulate liberation or storage of lipids, rather than as a barometer of total lipids stores (Figure 5). In many situations, high lipid stores will coincide with high lipid turnover, and so a general association of total lipid stores with leptin titer can be demonstrated (Maffei et al., 1995). It is also possible that the association between total fat stores and leptin in pathological obesity (e.g., obese humans, ob/ob mice) is not representative of the relationship in wild-type vertebrates. We assert that a comparative approach to studying leptin is needed to understand its function in all vertebrates (including humans). Now that several fish leptin clones are available, we predict that the non-mammal focused studies will influence models of leptin’s evolution, and its function in mammals.
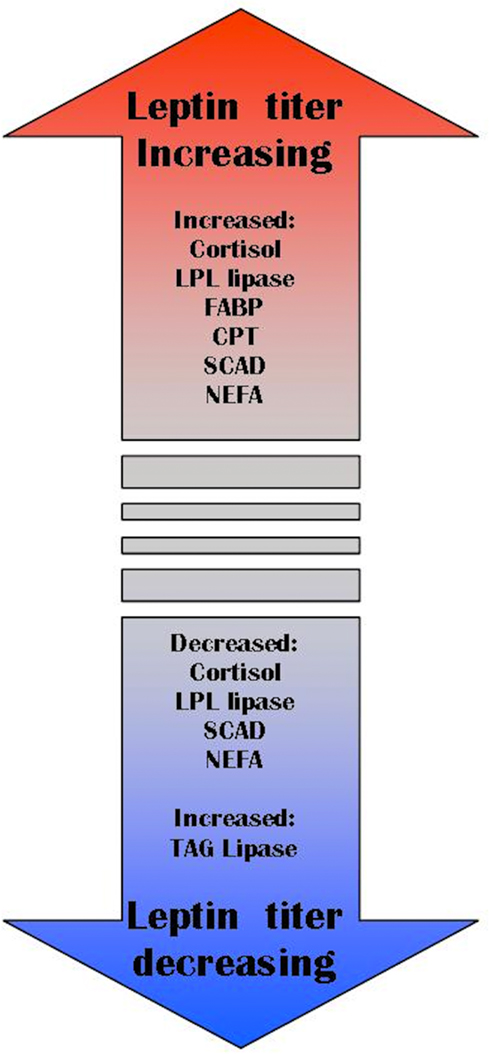
Figure 5. Allostasis model of leptin function. LPL lipase (lipoprotein lipase), FABP (fatty acid-binding protein), CPT (carnitine palmitoyl transferase), SCAD (stearoyl CoA desaturase-1), NEFA (non-esterified fatty acids), TAG lipase (triacylglycerol lipase).
Conflict of Interest Statement
The authors declare that the research was conducted in the absence of any commercial or financial relationships that could be construed as a potential conflict of interest.
References
Ahima, R. S. (2000). Leptin and the neuroendocrinology of fasting. Front. Horm. Res. 26:42–56. doi: 10.1159/000061014
Ajuwon, K. M., Kuske, J. L., Anderson, D. B., Hancock, D. L., Houseknecht, K. L., Adeola, O., and Spurlock, M. E. (2003). Chronic leptin administration increases serum NEFA in the pig and differentially regulates PPAR expression in adipose tissue. J. Nutr. Biochem. 14, 576–583.
Aksglaede, L., Juul, A., Olsen, L. W., and Sorensen, T. I. A. (2009). Age at puberty and the emerging obesity epidemic. PLoS ONE 4, e8450. doi: 10.1371/journal.pone.0008450
Amills, M., Jimenez, N., Villalba, D., Tor, M., Molina, E., Cubilo, D. Marcos, C., Francesch, A., and Sanchez, A. Estany, J. (2003). Identification of three single nucleotide polymorphisms in the chicken insulin-like growth factor 1 and 2 genes and their associations with growth and feeding traits. Poult. Sci. 82, 1485–1493.
Arora, A., and Arora, S. (2008). Leptin and its metabolic interactions – an update. Diabetes Obes. Metab. 10, 973–993.
Askari, H., Liu, J., and Dagogo-Jack, S. (2000). Hormonal regulation of human leptin in vivo: effects of hydrocortisone and insulin. Int. J. Obes. 24, 1254–1259.
Bado, A., Levasseur, S., Kermorgant, S., Laigneau, J. P., Bortoluzzi, M. N., Moizo, L., Lehy, T., Guerre-Millo, M., Le Marchand-Brustel, Y., and Lewin, M. J. (1998). The stomach is a source of leptin. Nature 394, 790–793.
Bai, Y. L., Zhang, S. Y., Kim, KS, Lee, J. K., and Kim, K. H. (1996). Obese gene expression alters the ability of 30A5 preadipocytes to respond to lipogenic hormones. J. Biol. Chem. 271, 13939–13942.
Baker, D. M., Larsen, D. A., Swanson, P., and Dickhoff, W. W. (2000). Long-term peripheral treatment of coho salmon (Oncorhynchus kisutch) with human leptin has no clear physiologic effect. Gen. Comp. Endocrinol. 118, 134–138.
Barash, I. A., Cheung, C. C., Weigle, D. S., Ren, H. P., Kabigting, E. B., Kuijper, J. L., Clifton, D. K., and Steiner, R. A. (1996). Leptin is a metabolic signal to the reproductive system. Endocrinology 137, 3144–3147.
Barcellos, L. J., Volpato, G. L., Barreto, R. E., Coldebella, I., and Ferreira, D. (2011). Chemical communication of handling stress in fish. Physiol. Behav. 103, 372–375.
Barcellos, L. J. G., Marqueze, A., Trapp, M., Quevedo, R. M., and Ferreira, D. (2010). The effects of fasting on cortisol, blood glucose, and liver and muscle glycogen in adult jundia Rhamdia quelen. Aquaculture 300, 231–236.
Bennett, B. D., Solar, G. P., Yuan, J. Q., Mathias, J., Thomas, G. R., and Matthews, W. (1996). A role for leptin and its cognate receptor in hematopoiesis. Curr. Biol. 6, 1170–1180.
Bertile, F., Oudart, H., Criscuolo, F., Maho, Y. L., and Racolt, T. (2003). Hypothalamic gene expression in long-terms fasted rats: relationship with body fat. Biochem. Biophys. Res. Commun. 303, 1106–1113.
Boden, G., Chen, X., Mozzoli, M., and Ryan, I. (1996). Effect of fasting on serum leptin in normal human subjects. J. Clin. Endocrinol. Metab. 81, 3419–3423.
Boorse, G. C., and Libbon, J. V. (2010). Genomic characterization of two leptin genes and a leptin receptor gene in the green anole, Anolis carolinensis. Integr. Comp. Biol. 50, E207–E207.
Bornstein, S. R., Uhlmann, K., Haidan, A., Ehrhart-Bornstein, M., and Scherbaum, W. A. (1997). Evidence for a novel peripheral action of leptin as a metabolic signal to the adrenal gland – leptin inhibits cortisol release directly. Diabetes 46, 1235–1238.
Boswell, T., Dunn, I. C., Wilson, P. W., Joseph, N., Burt, D. W., and Sharp, P. J. (2006). Identification of a non-mammalian leptin-like gene: characterization and expression in the tiger salamander (Ambystoma tigrinum). Gen. Comp. Endocrinol. 146, 157–166.
Botion, L. M. (2001). The influence of fasting/refeeding on the lipoprotein lipase activity of adipose tissue and muscle. Braz. J. Med. Biol. Res. 34, 1411–1414.
Cammisotto, P. G., Gelinas, Y., Deshaies, Y., and Bukowiecki, L. J. (2003). Regulation of leptin secretion from white adipocytes by free fatty acids. Am. J. Physiol. Endocrinol. Metab. 285, E521–E526.
Carulli, L., Ferrari, S., Bertolini, M., Tagliafico, R., and Delrio, G. (1999). Regulation of ob gene expression: evidence for epinephrine-induced suppression in human obesity. J. Clin. Endocrinol. Metab. 84, 3309–3312.
Chang, L. L., Kau, M. M., Wun, W. S. A., Ho, L. T., and Wang, P. S. (2002). Effects of fasting on corticosterone production by zona fasciculata-reticularis cells in ovariectomized rats. J. Investig. Med. 50, 86–94.
Chehab, F., Lim, M., and Lu, R. (1996). Correction of the sterility defect in homozygous obese female mice by treatment with the human recombinant leptin. Nat. Genet. 12, 318–320.
Chen, W. H., Sun, L. T., Tsai, C. L., Song, Y. L., and Chang, C. F. (2002). Cold stress induced modulation of catecholamines, cortisol, immunoglobulin M, and leukocyte phagocytosis in tilapia. Gen. Comp. Endocrinol. 126, 90–100.
Chu, C. A., Galassetti, P., Igawa, K., Sindelar, D. K., Neal, D. W., Burish, M., and Cherrington, A. D. (2003). Interaction of free fatty acids and epinephrine in regulating hepatic glucose production in conscious dogs. Am. J. Physiol. Endocrinol. Metab. 284, E291–E301.
Chu, D. L. H., Li, V. W. T., and Yu, R. M. K. (2010). Leptin: clue to poor appetite in oxygen-starved fish. Mol. Cell. Endocrinol. 319, 143–146.
Clarke, I. J., Tilbrook, A. J., Turner, A. I., Doughton, B. W., and Goding, J. W. (2001). Sex, fat and the tilt of the earth: effects of sex and season on the feeding response to centrally administered leptin in sheep. Endocrinology 142, 2725–2728.
Cnop, M., Landchild, M. J., Vidal, J., Havel, P. J., Knowles, N. G., Carr, D. R., Wang, F., Hull, R. L., Boyko, E. J., Retzlaff, B. M., Walden, C. E., Knopp, R. H., and Kahn, S. E. (2002). The concurrent accumulation of intra-abdominal and subcutaneous fat explains the association between insulin resistance and plasma leptin concentrations: distinct metabolic effects of two fat compartments. Diabetes 51, 1005–1015.
Cohen, P., Miyazaki, M., Socci, P., Hagge-Greenburg, A., Liedtke, W., Soukas, A. A., Sharma, R., Hudgins, L. C., Ntambi, J. M., and Friedman, J. M. (2002). Role for stearoyl-CoA desaturase-1 in leptin-mediated weight loss. Science 297, 240–243.
Considine, R. V., Sinha, K., Heiman, M. L., Kriauciunas, A., Stephens, T. W., Nyce, M. R., Ohannesian, J. P., Marco, C. C., McKee, L. J., Bauer, T. L., and Caro, J. F. (1996). Serum immunoreactive-leptin concentrations in normal-weight and obese humans. N. Engl. J. Med. 334, 292–295.
Crespi, E. J., and Denver, R. J. (2006). Leptin (ob gene) of the South African clawed frog. Proc. Natl. Acad. Sci. U.S.A. 103, 10092–10097.
Dagogo-Jack, S., Selke, G., Melson, A. K., and Newcomer, J. W. (1997). Robust leptin secretory responses to dexamethasone in obese subjects. J. Clin. Endocrinol. Metab. 82, 3230–3233.
Dagogo-Jack, S., Tykodi, G., and Umamaheswaran, I. (2005). Inhibition of cortisol biosynthesis decreases circulating leptin levels in obese humans. J. Clin. Endocrinol. Metab. 90, 5333–5335.
de Pedro, N., Martinez-Alvarez, R., and Delgado, M. J. (2006). Acute and chronic leptin reduces food intake and body weight in goldfish (Carassius auratus). J. Endocrinol. 188, 513–520.
Dole, V. P. (1956). Relation between non-esterified fatty acids in plasma and the metabolism of glucose. J. Clin. Invest. 35, 150–154.
Duan, Y., Wu, C., Chowdhury, S., Lee, M. C., Xiong, G., Zhang, W., Yang, R., Cieplak, P., Luo, R., Lee, T., Caldwell, J., Wang, J., and Kollman, P. (2003). A point-charge force field for molecular mechanics simulations of proteins based on condensed-phase quantum mechanical calculations. J. Comput. Chem. 24, 1999–2012.
Ducsay, C. A., Hyatt, K., Mlynarczyk, M., Kaushal, K. M., and Myers, D. A. (2006). Long-term hypoxia increases leptin receptors and plasma leptin concentrations in the late-gestation ovine fetus. Am. J. Physiol. Regul. Integr. Comp. Physiol. 291, R1406–R1413.
Dunbar, J. C., Hy, Y., and Lu, H. (1997). Intracerebroventricular leptin increases lumbar and renal sympathetic nerve activity and blood pressure in normal rats. Diabetes 46, 2040–2043.
El-Migdadi, F., Ei-Akawi, Z., Abudheese, R., and Bashir, N. (2002). Plasma levels of adrenocorticotropic hormone and cortisol in people living in an environment below sea level (Jordan Valley) during fasting in the month of Ramadan. Horm. Res. 58, 279–282.
Elbers, J. M. H., Asscheman, H., Seidell, J. C., Frolich, M., Meinders, A. E., and Gooren, L. J. G. (1997). Reversal of the sex difference in serum leptin levels upon cross-sex hormone administration in transsexuals. J. Clin. Endocrinol. Metab. 82, 3267–3270.
Elmquist, J. K., Ahima, R. S, Maratos-Flier, E., Flier, J. S., and Saper, C. B. (1997). Leptin activates neurons in ventrobasal hypothalamus and brainstem. Endocrinology 138, 838–842.
Fauconneau, B., Alami-Durante, H., Laroche, M., Marcel, J., and Vallot, D. (1995). Growth and meat quality relations in carp. Aquaculture 129, 265–297.
Fauconneau, B., Corraze, G., Lebail, P. Y., and Vernier, J. M. (1991). Lipid storage in fish: cellular, metabolic and hormonal control. Inra. Prod. Anim. 3, 369–381.
Felip, A., Zanuy, S., and Carrillo, M. (2006). Comparative analysis of growth performance and sperm motility between precocious and con-precocious males in the European sea bass (Dicentrarchus labrax, L.). Aquaculture 256, 570–578.
Felip, A., Zanuy, S., Muriach, B., Cerda-Reverter, J. M., and Carrillo, M. (2008). Reduction of sexual maturation in male Dicentrarchus labrax by continuous light both before and during gametogenesis. Aquaculture 275, 347–355.
Fernandez-Fernandez, R., Martini, A. C., Navarro, V. M., Castellano, J. M., Dieguez, C., Aguilar, E., Pinilla, L., and Tena-Sempere, M. (2006). Novel signals for the integration of energy balance and reproduction. Mol. Cell. Endocrinol. 254–255, 127–132.
Figueroa, J., San Martin, R., Flores, C., Grothusen, H., and Kausel, G. (2005). Seasonal modulation of growth hormone mRNA and protein levels in carp pituitary: evidence for two expressed genes. J. Comp. Physiol. B 175, 185–192.
Florant, G. L., Porst, H., Peiffer, A., Hudachek, S. F., Pittman, C., Summers, S. A., Rajala, M. W., and Scherer, P. E. (2004). Fat-cell mass, serum leptin, and adiponectin changes during weight gain and loss in yellow-bellied marmots (Marmota flaviventris). J. Comp. Physiol. B 174, 633–639.
Friedman-Einat, M., Boswell, T., Horev, G., Girishvarma, G., Dunn, I. C. Talbot, R. T., and Sharp, P. J. (1999). The chicken leptin gene: has it been cloned? Gen. Comp. Endocrinol. 115, 354–363.
Frøiland, E., Murashita, K., Jorgensen, E. H., and Kurokawa, T. (2010). Leptin and ghrelin in anadromous Arctic charr: cloning and change in expressions during a seasonal feeding cycle. Gen. Comp. Endocrinol. 165, 136–143.
Fruhbeck, G., Aguado, M., and Martinez, J. A. (1997). In vitro lipolytic effect of leptin on mouse adipocytes: evidence for a possible autocrine/paracrine role of leptin. Biochem. Biophys. Res. Commun. 240, 590–594.
Galton, D. L., and Bray, G. A. (1967). Studies on lipolysis in human adipose tissue. J. Clin. Invest. 46, 621–629.
Gorissen, M., Bernier, N. J., Nabuurs, S. B., Flik, G., and Huising, M. O. (2009). Two divergent leptin paralogues in zebrafish (Danio rerio) that originate early in teleostean evolution. J. Endocrinol. 201, 329–339.
Gorissen, M., Flik, G., and Huising, M. (2006). Peptides and proteins regulating food intake: a comparative view. Anim. Biol. 56, 447–473.
Grunfeld, C., Zhao, C., Fuller, J., Pollock, A., Moser, A., Friedman, J. M., and Feingold, K. R. (1996). Endotoxin and cytokines induce expression of leptin, the ob gene product, in hamsters. J. Clin. Invest. 97, 2152–2157.
Hajri, T., Hall, A. M., Jensen, D. R., Pietka, T. A., Drover, V. A., Tao, H., Eckel, R., and Abumrad, N. A. (2007). CD36-facilitated fatty acid uptake inhibits leptin production and signaling in adipose tissue. Diabetes 56, 1872–1880.
Henderson, R. J., and Tocher, D. R. (1987). The lipid composition and biochemistry of freshwater fish. Prog. Lipid Res. 26, 281–347.
Horev, G., Einat, P., Aharoni, T., Eshdat, Y., and Friedman-Einat, M. (2000). Molecular cloning and properties of the chicken leptin-receptor (CLEPR) gene. Mol. Cell. Endocrinol. 162, 95–106.
Huising, M., Geven, E., Kruiswijk, C., Nabuurs, S., Stolte, E., Spanings, T., Verburg-van Kemenade, L., and Flik, G. (2006). Increased leptin expression in common carp (Cyprinus carpio) after feeding but not after fasting or feeding to satiation. Endocrinology 147, 5786–5797.
Hwa, J. J., Fawzi, A. B., Graziano, M. P., Ghibaudi, L., Williams, P., Van Heek, M., Rudinski, M., Sybertz, E., and Strader, C. D. (1997). Leptin increases energy expenditure and selectively promotes fat metabolism in ob/ob mice. Am. J. Physiol. 272, R1204–R1209.
Jelks, A., Belkacemi, L., Han, G., Chong, W. L., Ross, M. G., and Desai, M. (2010). Paradoxical increase in maternal plasma leptin levels in food-restricted gestation: contribution by placental and adipose tissue. Reprod. Sci. 16, 665–675.
Johansen, S. J. S., Ekli, M., and Jobling, M. (2002). Is there lipostatic regulation of feed intake in Atlantic salmon Salmo salar L.? Aquac. Res. 33, 515–524.
Johnson, R. M., Johnson, T. M., and Londraville, R. L. (2000). Evidence for leptin expression in fishes. J. Exp. Biol. 286, 718–724.
Jonsson, B., and Jonsson, N. (2005). Lipid energy reserves influence life-history decision of Atlantic salmon (Salmo salar) and brown trout (S. trutta) in fresh water. Ecol. Freshw. Fish 14, 296–301.
Kelley, K. M., Haigwood, J. T., Perez, M., and Galima, M. M. (2001). Serum insulin-like growth factor binding proteins (IGFBPSs) as markers for anabolic/catabolic condition in fishes. Comp. Biochem. Physiol. B Biochem. Mol. Biol. 129, 229–236.
Kling, P., Ronnestad, I., Stefansson, S. O., Murashita, K., Kurokawa, T., and Bjornsson, B. T. (2009). A homologous salmonid leptin radioimmunoassay indicates elevated plasma leptin levels during fasting of rainbow trout. Gen. Comp. Endocrinol. 162, 307–312.
Kosaki, A., Yamada, K., and Kuzuya, H. (1996). Reduced expression of the leptin gene (ob) by catecholamine through a G(s) protein-coupled pathway in 3T3-L1 adipocytes. Diabetes 45, 1744–1749.
Kronfeld-Schor, N., Richardson, C., Silvia, B. A., Kunz, T. H., and Widmaier, E. P. (2000). Dissociation of leptin secretion and adiposity during prehibernatory fattening in little brown bats. Am. J. Physiol. Regul. Integr. Comp. Physiol. 279, R1277–R1281.
Kurokawa, T., and Murashita, K. (2009). Genomic characterization of multiple leptin genes and a leptin receptor gene in the Japanese medaka, Oryzias latipes. Gen. Comp. Endocrinol. 161, 229–237.
Kurokawa, T., Uji, S., and Suzuki, T. (2005). Identification of cDNA coding for a homologue to mammalian leptin from pufferfish, Takifugu rubripes. Peptides 26, 45–750.
Kurpad, A. V., Khan, K., Calder, G., Coppack, S. W., Frayn, K. N., Macdonald, I. A., and Elia, M. (1994). Effect of noradrenaline on glycerol turnover and lipolysis in the whole body and subcutaneous adipose tissue in humans in vivo. Clin. Sci. 86, 177–184.
Laferrere, B., Caixas, A., Fried, S. K., Bashore, C., Kim, J., and Pi-Sunger, F. X. (2002). A pulse of insulin and dexamethasone stimulates serum leptin in fasting human subjects. Eur. J. Endocrinol. 146, 839–845.
Lafrance, V., Wataru Inoue, W., Kana, B., and Luhesh, G. N. (2010). Leptin modulates cell morphology and cytokine release in microglia. Brain Behav. Immun. 24, 358–365.
LaMarca, A., and Volpe, A. (2004). Recombinant human leptin in women with hypothalamic amenorrhea. N. Engl. J. Med. 351, 2343; author reply 2343.
Larsson, H., and Ahren, B. (1996). Short-term dexamethasone treatment increases plasma leptin independently of changes in insulin sensitivity in healthy women. J. Clin. Endocrinol. Metab. 81, 4428–4432.
Lee, M. J., and Fried, S. K. (2009). Integration of hormonal and nutrient signals that regulate leptin synthesis and secretion. Am. J. Physiol. Endocrinol. Metab. 296, E1230–E1238.
Li, G. G., Liang, X. F., Guangzhao, Q. X., Yu, Y., and Lai, K. (2010). Gene structure, recombinant expression and functional characterization of grass carp leptin. Gen. Comp. Endocrinol. 166, 117–127.
Liu, Q., Chen, Y., Copeland, D., Ball, H., Duff, R. J., Rockich, B., and Londraville, R. L. (2010). Expression of leptin receptor gene in developing and adult zebrafish. Gen. Comp. Endocrinol. 166, 346–355.
Lollmann, B., Gruninger, S., Stricker-Krongrad, A., and Chiesi, M. (1997). Detection and quantification of the leptin receptor splice variants of Ob-Ra, b, and e in different mouse tissues. Biochem. Biophys. Res. Commun. 238, 648–652.
Londraville, R. L., and Duvall, C. S. (2002). Murine leptin injections increase intracellular fatty acid-binding protein in green sunfish (Lepomis cyanellus). Gen. Comp. Endocrinol. 129, 56–62.
Maffei, M., Halaas, J., Ravussin, E., Pratley, R. E., Lee, G. H., Zhang, Y., Fei, H., Kim, S., Lallone, R., Ranganathan, S., Kern, P. A., and Friedman, J. M. (1995). Leptin levels in human and rodent: measurement of plasma leptin and ob RNA in obese and weight-reduced subjects. Nat. Med. 1, 1155–1161.
Mantzoros, C. S., Qu, D. Q., Frederich, R. C., Susulic, V. S., Lowell, B. B., Maratos-Flier, E., and Flier, J. S. (1996). Activation of beta(3) adrenergic receptors suppresses leptin expression and mediates a leptin-independent inhibition of food intake in mice. Diabetes 45, 909–914.
Marques, I. J., Leito, J. T. D., Spaink, H. P., Testerink, J., Jaspers, R. T., Witte, F., van den Berg, S., and Bagowski, C. P. (2008). Transcriptome analysis of the response to chronic constant hypoxia in zebrafish hearts. J. Comp. Physiol. B 178, 77–92.
Maxime, V. (2008). The physiology of the triploid fish: current knowledge and comparisons with diploid fish. Fish Fish. (Oxf.) 9, 67–78.
McCullough, A.J. (2006). Pathophysiology of nonalcoholic steatohepatitis. J. Clin. Gastroenterol. 40, S17–S29.
McEwen, B. S. (2000). The neurobiology of stress: from serendipity to clinical relevance. Brain Res. 886, 172–189.
Moreno-Aliaga, M. J., Stanhope, K. L., and Havel, P. J. (2001). Transcriptional regulation of the leptin promoter by insulin-stimulated glucose metabolism in 3t3-11 adipocytes. Biochem. Biophys. Res. Commun. 283, 544–548.
Murashita, K., Uji, S., Yamamoto, T., Ronnestad, I., and Kurokawa, T. (2008). Production of recombinant leptin and its effects on food intake in rainbow trout (Oncorhynchus mykiss). Comp. Biochem. Physiol. B Biochem. Mol. Biol. 150, 377–384.
Newcomer, J. W., Selke, G., Melson, A. K., Gross, J., Vogler, G. P., and Dagogo-Jack, S. (1998). Dose-dependent cortisol-induced increases in plasma leptin concentration in healthy humans. Arch. Gen. Psychiatry 55, 995–1000.
O’Conner, D. M., Blache, D., Hoggard, N., Brookes, E., Wooding, F. B., Fowden, A. L., and Forhead, A. J. (2007). Developmental control of plasma leptin and adipose leptin messenger ribonucleic acid in the ovine fetus during late gestation: role of glucocorticoids and thyroid hormones. Endocrinology 148, 3750–3757.
O’Flynn, F. M., McGeachy, S. A., Friars, G. W., Benfey, T. J., and Bailey, J. K. (1997). Comparisons of cultured triploid and diploid Atlantic salmon (Salmo salar L.). ICES J. Mar. Sci. 54, 1160–1165.
Oka, T., Nishimura, Y., Zang, L., Shimada, Y., Wang, Z., Umemoto, N., Kuroyanagi, J., Nishimura, N., and Tanaka, T. (2010). Diet-induced obesity in zebrafish shares common pathophysiological pathways with mammalian obesity. BMC Physiol. 10, 21. doi: 10.1186/1472-6793-10-21
Pelleymounter, M. A., Cullen, M. J., Baker, M. B., Hecht, R., Winters, D., Boone, T., and Collins, F. (1995). Effects of the obese gene product on body weight regulation in ob/ob mice. Science 269, 540–543.
Peterson, B. C., and Small, B. C. (2004). Effects of fasting on circulating IGF-binding proteins, glucose, and cortisol in channel catfish (Ictalurus punctatus). Domest. Anim. Endocrinol. 26, 231–240.
Peyon, P., de Celis, S. V. R., Gomez-Requeni, P., Zanuy, S., Perez-Sanchez, J., and Carrillo, M. (2003). In vitro effect of leptin on somatolactin release in the European sea bass (Dicentrarchus labrax): dependence on the reproductive status and interaction with NPY and GnRH. Gen. Comp. Endocrinol. 132, 284–292.
Peyon, P., Zanuy, S., and Carillo, M. (2001). Actions of leptin on in vitro luteinizing hormone release in the European sea bass (Dicentrarchus labrax). Biol. Reprod. 65, 1573–1578.
Pfundt, B., Sauerwein, H., and Mielenz, M. (2009). Leptin mRNA and protein immunoreactivity in adipose tissue and liver of rainbow trout (Oncorhynchus mykiss) and immunohistochemical localization in liver. Anat. Histol. Embryol. 38, 406–410.
Pottinger, T. G., Rand-Weaver, M., and Sumpter, J. P. (2003). Overwinter fasting and re-feeding in rainbow trout: plasma growth hormone and cortisol levels in relation to energy mobilization. Comp. Biochem. Physiol. B Biochem. Mol. Biol. 136, 403–417.
Pralong, F. P., Roduit, R., Waeber, G., Castillo, E., Mosimann, F., Thorens, B., and Gaillard, R. C. (1998). Leptin inhibits directly glucocorticoid secretion by normal human and rat adrenal gland. Endocrinology 139, 4264–4268.
Rayner, D. V., and Trayhurn, P. (2001). Regulation of leptin production: sympathetic nervous system interactions. J. Mol. Med. 79, 8–20.
Reidy, S. P., and Weber, J. M. (2000). Leptin: an essential regulator of lipid metabolism. Comp. Biochem. Physiol. A Mol. Integr. Physiol. 125, 285–297.
Rønnestad, I., Nilsen, T. O., Murashita, K., Angotzi, A. R., Moen, G. G., Stefansson, S. O., Kling, P., Bjornsson, B. T., and Kurokawa, T. (2010). Leptin and leptin receptor genes in Atlantic salmon: cloning, phylogeny, tissue distribution and expression correlated to long-term feeding status. Gen. Comp. Endocrinol. 168, 55–70.
Rousseau, K., Atcha, Z., and Loudon, A. S. (2003). Leptin and seasonal mammals. J. Neuroendocrinol. 15, 409–414.
Samra, J. S., Clark, M. L., Humphreys, S. M., Macdonald, I. A., Matthews, D. R., and Frayn, K. N. (1996a). Effect of rise in cortisol concentration on regulation of lipolysis in subcutaneous adipose tissue. Am. J. Physiol. 271, E996–E1002.
Samra, J. S., Simpson, E. J., Clark, M. L., and Frayn, K. N. (1996b). Effects of epinephrine infusion on adipose tissue: interactions between blood flow and lipid metabolism. Am. J. Physiol. 271, E834–E839.
Sharp, P. J., Dunn, I. C, Waddington, D., and Boswell, T. (2008). Chicken leptin. Gen. Comp. Endocrinol. 158, 2–4.
Shintani, M., Nishimura, H., Yonemitsu, S., Masuzaki, H., Ogawa, Y., Hosoda, K., Inoue, G., Yoshimasa, T., and Nakao, K. (2000). Downregulation of leptin by free fatty acids in rat adipocytes: effects of triacsin C, palmitate, and 2-bromopalmitate. Metab. Clin. Exp. 49, 326–330.
Shiose, S., Hata, Y., Noda, Y., Sassa, Y., Takeda, A., Yoshikawa, H., Fujisawa, K., Kubota, T., and Ishibashi, T. (2004). Fibrinogen stimulates in vitro angiogenesis by choroidal endothelial cells via autocrine VEGF. Graefes Arch. Clin. Exp. Ophthalmol. 242, 777–783.
Sierra-Honigmann, M. R., Nath, A. K., Murakami, C., García-Cardeña, G., Papapetropoulos, A., Sessa, W. C., Madge, L. A., Schechner, J. S., Schwabb, M. B., Polverini, P. J., and Flores-Riveros, J. R. (1998). Biological action of leptin as an angiogenic factor. Science 281, 1683–1686.
Small, B. C. (2005). Effect of fasting on nychthemeral concentrations of plasma growth hormone (GH), insulin-like growth factor 1 (IGF-1), and cortisol in channel catfish (Ictalurus punctatus). Comp. Biochem. Physiol. B Biochem. Mol. Biol. 142, 217–223.
Smith, P., and Ferguson, A. V. (2008). Neurophysiology of hunger and satiety. Dev. Disabil. Res. Rev. 14, 96–104.
Spanovich, S., Niewiarowski, P. H., and Londraville, R. L. (2006). Seasonal effects on circulating leptin in the lizard Sceloporus undulatus from two populations. Comp. Biochem. Physiol. B Biochem. Mol. Biol. 143, 507–513.
Steppan, C. M., Crawford, T. D., Chidsey-Frink, K. L., Ke, H., and Swick, A. G. (2000). Leptin is a potent stimulator of bone growth in ob/ob mice. Regul. Pept. 92, 73–78.
Sumpter, J. P., Le Bail, P. Y., Pickering, A. D., Pottinger, T. G., and Carragher, J. F. (1991). The effect of starvation on growth and plasma growth hormone concentrations of rainbow trout, Oncorhynchus mykiss. Gen. Comp. Endocrinol. 83, 94–102.
Swoap, S. J. (2008). The pharmacology and molecular mechanisms underlying temperature regulation and torpor. Biochem. Pharmacol. 76, 817–824.
Swoap, S. J., Gutilla, M. J., Liles, L. C. Smith, R. O., and Weinshenker, D. (2006). The full expression of fasting-induced torpor requires beta 3-adrenergic receptor signaling. J. Neurosci. 26, 241–245.
Takekoshi, K., Motooka, M., Isobe, K., Nomura, F., Manmoku, T., Ishii, K., and Nakai, T. (1999). Leptin directly stimulates catecholamine secretion and synthesis in cultured porcine adrenal medullary chromaffin cells. Biochem. Biophys. Res. Commun. 261, 426–431.
Tamura, K., Dudley, J., Nei, M., and Kumar, S. (2007). MEGA4: molecular evolutionary genetics analysis (MEGA) software version 4.0. Mol. Biol. Evol. 24, 1596–1599.
Taouis, M., Chen, J. W., Daviaud, C., Dupont, J., Derouet, M., and Simon, J. (1998). Cloning the chicken leptin gene. Gene 208, 239–242.
Vissers, M., Gorissen, M., Flik, G., and Huising, M. O. (2010). “Leptin in teleost fish,” in Leptin in Non-Mammalian Vertebrates, ed. M. Paolucci (Transworld Research Network), Kerala, 1–24.
Watanobe, H. (2002). Leptin directly acts within the hypothalamus to stimulate gonadotropin-releasing hormone secretion in vivo in rats. J. Physiol. 545, 255–268.
Weigle, D. S., Bukowski, T. R., Foster, D. C., Holderman, S., Kramer, J. M., Lasser, G., Lofton-Day, C. E., Prunkard, D. E., Raymond, C., and Kuijpert, J. L. (1995). Recombinant ob protein reduces feeding and body weight in the ob/ob mouse. J. Clin. Invest. 96, 2065–2070.
Weil, C., Le Bail, P. Y., Sabin, N., and Le Gac, F. (2003). In vitro action of leptin on FSH and LH production in rainbow trout (Onchorynchus mykiss) at different stages of the sexual cycle. Gen. Comp. Endocrinol. 130, 2–12.
Wong, M. M. L., Yu, R. M. K., Ng, P. K. S., Law, S. H. W., Tsang, A. K. C., and Kong, R. Y. C. (2007). Characterization of a hypoxia-responsive leptin receptor (omLepRL) cDNA from the marine medaka (Oryzias melastigma). Mar. Pollut. Bull. 54, 797–803.
Wu, S., and Zhang, Y. (2007). LOMETS: a local meta-threading-server for protein structure prediction. Nucleic Acids Res. 35, 3375–3382.
Yada, T., Azuma, T., Takahashi, A., Suzuki, Y., and Hirose, S. (2000). Effects of desacetyl-alpha-MSH on lipid mobilization in the rainbow trout, Oncorhynchus mykiss. Zool. Sci. 17, 1123–1127.
Yang, J., Wang, Z. L., Zhao, X. Q., de Wang, P., de Qi, L., Xu, B. H., Ren, Y. H., and Tian, H. F. (2008). Natural selection and adaptive evolution of leptin in the ochotona family driven by the cold environmental stress. PLoS ONE 3, e1472. doi: 10.1371/journal.pone.0001472
Yu, W., Kimura, M., Walczewska, A., Karanth, S., and McCann, S. (1997). Role of leptin in hypothalamic-pituitary function. Proc. Natl. Acad. Sci. U.S.A. 94, 1023–1028.
Zhan, X. M., Li, Y. L., and Wang, D. H. (2009). Effects of fasting and refeeding on body mass, thermogenesis, and serum leptin in Brandt’s voles (Lasiopodomys brandtii). J. Therm. Biol. 34, 237–243.
Zhang, F., Basinski, M. B., Beals, J. M., Briggs, S. L., Churgay, L. M., Clawson, D. K., DiMarchi, R. D., Furman, T. C., Hale, J. E., Hsiung, H. M., Schoner, B. E., Smith, D. P., Zhang, X. Y., Wery, J. P., and Schevitz, R. W. (1997). Crystal structure of the obese protein leptin-E100. Nature 387, 206–209.
Keywords: lipid metabolism, endothermy, life history strategy
Citation: Copeland DL, Duff RJ, Liu Q, Prokop J and Londraville RL (2011) Leptin in teleost fishes: an argument for comparative study. Front. Physio. 2:26. doi: 10.3389/fphys.2011.00026
Received: 04 April 2011;
Accepted: 27 May 2011;
Published online: 14 June 2011.
Edited by:
Andrew Gracey, University of Southern California, USAReviewed by:
Michael Berenbrink, University of Liverpool, UKMikko Nikinmaa, University of Turku, Finland
Copyright: © 2011 Copeland, Duff, Liu, Prokop and Londraville. This is an open-access article subject to a non-exclusive license between the authors and Frontiers Media SA, which permits use, distribution and reproduction in other forums, provided the original authors and source are credited and other Frontiers conditions are complied with.
*Correspondence: Richard Lyle Londraville, Department of Biology, University of Akron, C310 ASEC, Akron, OH 44325-3908, USA. e-mail:bG9uZHJhdmlsbGVAdWFrcm9uLmVkdQ==