- 1 Department of Pediatrics, Baylor College of Medicine, Houston, TX, USA
- 2 Department of Anesthesiology, Baylor College of Medicine, Houston, TX, USA
- 3 Department of Physiology and Biophysics, Baylor College of Medicine, Houston, TX, USA
Transient receptor potential (TRP) C1 and C3 (TRPC1 and TRPC3) are expressed in vascular smooth muscle cells and are thought to be involved in vascular contractility. In the present study, we determined the effect of systemic hypertension on TRPC1/TRPC3 channel expression and vascular contractility in rat carotid artery (CA). CA were studied from male spontaneously hypertensive rats (SHR), Wistar-Kyoto (WKY), and Long Evans (LE) rats. TRPC1/3 expression was determined by RT-PCR and Western blot. TRP channel function was evaluated by whole-cell patch clamp, using UTP (60 μM) to stimulate TRPC1/3 channels. Contractions of endothelium-denuded CA segments to UTP (1–300 μM) and phenylephrine (Phe; 0.1 nM–10 μM) were measured in an isometric tension bath. TRPC1 and TRPC3 mRNA was present in CA of both WKY and SHR. Western blot demonstrated 3.1 ± 1.2 times greater TRPC3 expression and 0.5 ± 0.2 times TRPC1 in SHR versus WKY CA. Isolated CA showed potentiated contraction to UTP in the SHR versus WKY. Activation of voltage-dependent Ca2+ channels (VDCC) in UTP-mediated constriction only occurred in SHR CA. Contraction to Phe was unaltered between WKY and SHR CA and involved equal significant VDCC activation in both groups. Patch clamp demonstrated that the UTP-stimulated current (Iutp) was greater in SHR compared to the normotensive WKY and LE rats with peak Iutp (at −110 mV) of −63 ± 24 pA compared to −25 ± 4 pA, respectively. We demonstrate that UTP-mediated but not Phe-mediated constrictions are potentiated in the CA during hypertension. Expression of TRPC1 is decreased whereas TRPC3 is increased in SHR CA. Interestingly, VDCC activation only contributes to UTP-mediated contraction of SHR CAs whereas it contributes substantially and equally in Phe-mediated contraction. We speculate that the alteration of TRPC channel expression in hypertension leads to greater smooth muscle depolarization, VDCC activation, and vascular contractility in the UTP (but not Phe) signaling pathway.
Introduction
Hypertension is associated with profound alteration in smooth muscle cell calcium homeostasis and proliferation (Sugiyama et al., 1990; Bendhack et al., 1992; Touyz and Schiffrin, 1997). Smooth muscle cell contractility and proliferation is largely dependant on increased intracellular calcium concentration which is brought about by voltage-dependent Ca2+ channels (VDCC) and non-voltage gated calcium entry pathways (Nelson et al., 1990; McDonald et al., 1994; Kuriyama et al., 1998; Hofmann et al., 1999; Large, 2002; Inoue et al., 2004; Taylor and Moneer, 2004). The bulk of calcium influx is generally thought to be mediated by VDCC. However, canonical transient receptor potential (TRPC) channels have also been shown to be important signal transducers for agonist mediated vascular contractility. The TRPC channels comprise a seven member family (TRPC1–7) of non-selective cation channels with varying Na+ and Ca2+ permeability (Clapham et al., 2005; Ramsey et al., 2006). They are activated by a variety of vasoconstrictors such as ATP, UTP, endothelin, and Angiotensin II (Hurst et al., 1998; Reading et al., 2005; Peppiatt-Wildman et al., 2007; Alvarez et al., 2008; Abramowitz and Birnbaumer, 2009). In addition to a potential direct contribution to calcium influx, the TRPC channels are thought to promote calcium entry by providing the depolarizing stimulus (Na+ and Ca2+ current) for VDCC activation and subsequent smooth muscle contraction (Welsh et al., 2002; Gudermann et al., 2004).
TRPC3 expression has recently been found to be upregulated in monocytes from spontaneously hypertensive rats (SHR; Liu et al., 2005a) and patients with essential hypertension (Liu et al., 2006). This upregulation was associated with increased calcium influx into these cells. More recently, Liu et al. (2009) showed increased TRPC3 channel expression and function in intact aorta and cultured aortic smooth muscle cells from SHR using Angiotensin II mediated calcium influx and aortic contraction.
TRPC1 is associated with VSMC proliferation after vascular injury (Kumar et al., 2006) and its expression is upregulated in hypoxia induced pulmonary hypertension (Lin et al., 2004) and in cardiac hypertrophy (Ohba et al., 2007). However, the role of this channel in vascular contractility is still not resolved, with some studies indicating a role for TRPC1 in smooth muscle contraction and others not (Kunichika et al., 2004; Bergdahl et al., 2005; Dietrich et al., 2007; Wolfle et al., 2010). TRPC1 is known to form heterotetramers with several TRPC channel members, including TRPC3 (Liu et al., 2005b; Zagranichnaya et al., 2005), thus making the study of TRPC1 expression in hypertension a logical parallel.
Given the emerging pattern of upregulation of TRPC1 and TRPC3 expression in other arteries in systemic and pulmonary hypertension, we sought to determine if these channels were similarly upregulated in carotid artery (CA) of hypertensive rats. Additionally, we sought to determine the effect of hypertension on TRPC channel function at the cellular level and vascular contractility at the whole artery level.
Materials and Methods
Male SHR and Wistar-Kyoto (WKY) rats with an average age of 13 ± 3 weeks from Charles River Laboratories were used for the study. SHR weighed 276 ± 36 g and WKY 281 ± 36 g. Blood pressure was measured using tail-cuff plethysmography. Average systolic blood pressure for the SHR was 157 ± 18 mmHg while for WKY was 102 ± 12 mmHg. In some experiments age-matched Long Evans (LE) rats were used as additional normotensive controls. Experiments were carried out in accordance with the National Institutes of Health guidelines for the care and use of laboratory animals and were approved by the Animal Protocol Review Committee at Baylor College of Medicine. The rats were anesthetized with Isoflurane followed by exsanguination prior to harvesting the carotid arteries.
RNA Extraction and RT-PCR Analysis
RNA was extracted from liquid nitrogen frozen carotid arteries using Trizol Reagent with the PureLink Micro Kit (Invitrogen, Carlsbad, CA, USA). Arteries were ground with a pestle and subjected to a Tissue Tearor for 45 s prior to addition of TRIzol. The rest of the protocol was then carried out according to the instructions for the PureLink kit. RNA was quantified using a UV Photospectrometer at the 260- and 280-nm wavelengths to assess purity and concentration. RNA was then treated with DNAse I (Invitrogen, Carlsbad, CA, USA) and then reverse transcribed to cDNA using the Super Script III First Strand Synthesis System (Invitrogen, Carlsbad, CA, USA). RNase H was added and incubated for 20 min at 37°C to degrade any remaining RNA. Control samples (RT-) were prepared by substitution of dH2O for reverse transcriptase to control for genomic contamination. PCR amplification was performed using Platinum Taq DNA polymerase (Invitrogen, Carlsbad, CA, USA) according to the following protocol: 94°C activation (2 min) followed by 97°C dissociation (15 s), 56°C anneal (30 s), and 72°C elongation (30 s) for 33 cycles. Primer pairs were as follows: TRPC1 forward: 5′-gtgcttgcggcttgagat-3′, TRPC1 reverse: 5′-tgccatagctggggaac-3′; TRPC3 forward: 5′-ctggccaacatagagaaggagt-3′, TRPC3 reverse: 5′-caccgattccagatctccat-3 (5)′. PCR products were analyzed by electrophoresis and digitally imaged for analysis. The predicted size of the PCR amplicons was 114 and 141 bp for TRPC1 and TRPC3, respectively.
Western Blot Analysis
Two and a half millimeter sections of CA were harvested, cleaned of connective tissue and blood, and frozen in liquid nitrogen. The segments were pulverized in a matching bullet tube and pestle, homogenized in RIPA buffer (Sigma, St. Louis, MO, USA) containing 50 mM Tris.HCl, pH 8.0, with 150 mM sodium chloride, 1.0% Non-idet P-40, 0.5% sodium deoxycholate, and 0.1% sodium dodecyl sulfate with protease inhibitor cocktail (Roche, Indianapolis, IN, USA) and incubated on ice for 15 min. After centrifugation (15 min at 15,000 g), a portion of the supernatant was used for total protein quantification by DC Protein Assay (Bio-Rad, Hercules, CA, USA). Equal amounts of sample protein were mixed with LDS sample buffer and sample reducing agent (Invitrogen, Carlsbad, CA, USA), and heated at 70°C for 10 min. Total protein samples (Chen et al., 2010; micrograms) were loaded into each lane of the gel (10% NuPAgE Bis–Tris gel). The gel was run at room temperature at 150 V for 90 min and then transferred to a nitrocellulose membrane by iBlot Dry Blotting System (Invitrogen, Carlsbad, CA, USA), according to the manufacturer’s instructions. The membrane was blocked in PBS supplemented with 5% non-fat dry milk and 0.2% Tween 20 (block solution) for 3 h, followed by incubation with anti-TRPC3 antibody (1:800) or anti-TRPC1 antibody (1:400; all from Sigma, St. Louis, MO, USA). The membrane was washed in PBS with 0.2% Tween 20 (PBS-T, 2 × 5 min then 3 × 10 min) and then incubated in a goat anti-rabbit secondary antibody conjugated to horseradish peroxidase (1:10,000 diluted in block solution, Thermo Fisher, Rockford, IL, USA) for 1 h. The lower portion of the membrane was probed separately with anti-GAPDH antibody (1:200,000) at 4°C overnight as a loading control. The GAPDH antibody used goat anti-mouse HRP (1:50,000 diluted in block solution) as the secondary antibody. The membrane was washed with PBS-T (as above) and then layered with Pierce SuperSignal West Femto Maximum Sensitivity Substrate (4 min), followed by exposure to film. Densitometric analysis was performed with Image J (NIH).
Isolation of Rat CA SMC
Right and left CA were harvested, cleaned of connective tissue and blood, and cut into two segments which were incubated for 10 min in cold (4°C) digestion buffer, containing 140 mM NaCl, 5 mM KCl, 2 mM MgCl2, 10 mM glucose, 10 mM HEPES, and 1 mg/ml Bovine Serum Albumin (Sigma, A9647), with pH adjusted to 7.4 with NaOH. Arteries were then digested with 1.86 mg/ml papain (Sigma, P4762), 1 mg/ml 1,4-dithioerythritol (Sigma, D8255) and 1 mg/ml BSA in digestion buffer for 30 min at 37°C. The tissue was next washed with digestion buffer twice and further digested with 1.5 mg/ml collagenase H (Sigma, C8051), 1 mg/ml hyaluronidase (Sigma, H3506), and 1 mg/ml BSA in digestion buffer containing 50 μM CaCl2 for 10–20 min at 37°C. The tissue was washed several times with digestion buffer and triturated with a fire-polished Pasteur pipette. The cells were placed on ice and used within 8 h.
Patch Clamp Electrophysiology
The whole-cell patch clamp configuration was used for measurements of whole-cell currents in freshly isolated SMC using a MultiClamp 700B amplifier (Axon Instruments) and pCLAMP 10 software (Axon Instruments, Union City, CA, USA), as reported previously (Chen et al., 2009). Patch electrodes were pulled from borosilicate glass (1.65 outer diameter, 1.28 inner diameter; Warner Instruments, Hamden, CT, USA) and polished to a pipette resistance of 2 MΩ. The pipette buffer contained (in mM) 156 CsCl, 1 MgCl2, 0.91 CaCl2, 2 EGTA, 7.85 CsOH, and 10 HEPES; pH was adjusted to 7.2 with CsOH. The calculated free Ca2+ concentration was 131 nM. The bath buffer contained (mM) 156 NaCl, 1.8 CaCl2, 10 glucose, and 10 HEPES; pH was adjusted to 7.4 with NaOH. The isolated cells were placed in a recording chamber on the stage of an inverted microscope and continually superfused with bath buffer. Whole-cell currents were recorded in the voltage-clamp mode over the voltage range of −110 to +80 mV (sweep rate of 0.131 mV/ms; −50 mV holding potential). Whole-cell ionic currents were measured in the absence or presence of 60 μM UTP. Lanthanum chloride (100 μM) was added 5 min after UTP stimulation. Data were digitized and filtered at 1 kHz. All recordings were performed at room temperature (20–22°C).
Isometric Tension Recordings in Isolated Carotid Arteries
Isolated CAs from SHR and WKY rats were placed in ice-cold physiological solution containing the following (in mM): 137 NaCl, 5.6 KCl, 1 MgCl2, 10 glucose, 2.5 CaCl2, and 10 HEPES (pH 7.4). Each artery was subsequently cut into four ring segments that were 2.5 mm in length. The rings were denuded of the endothelium by placing curved forceps in the lumen and gently rolling the artery along a length of submerged paper towel. Arteries were then mounted in an eight channel artery myograph (ChuelTech, Houston, TX, USA) containing warm (37°C), gassed (75%N2, 20%O2, 5%CO2) physiological saline solution containing (in mM) 119 NaCl, 4.7 KCl, 25 NaHCO3, 1.2 KH2PO4, 1 MgSO4, 2.5 CaCl2, and 11.1 glucose (pH 7.4). In experiments using Ca2+-free physiological saline solution, CaCl2 was omitted and 0.1 mM EGTA was added. Each ring was stretched to a resting tension of 1.0 g based on preliminary length-tension studies. Rings were then subjected to four 40 mM KCl exposures, followed by one 10−5 M carbachol exposure to confirm the absence of endothelium. Denuded vessels that demonstrated partial relaxation to carbachol were excluded from further study. Concentration-response curves (CRC) were performed to UTP (1 × 10−6 to 3 × 10−4 M) or phenylephrine (1 × 10−10 to 1 × 10−5 M) in half-log steps in the presence and absence of verapamil (1 × 10−5 M). Single concentration responses to UTP (100 μM) were also performed in some protocols. Data was digitized and analyzed with Powerlab/8SP with Chart version 4.24.
Statistical Methods
Data are presented as mean ± SEM. CRC were evaluated by two way RM-ANOVA with post hoc Holm–Sidak test for individual comparisons. Repeated single concentration UTP responses were compared by paired t-test within the same group and by t-test between groups. Densitometry data was first normalized to the respective GAPDH signal and then the SHR value divided by the WKY value. The resulting values were then evaluated between groups by Mann–Whitney Rank Sum test (TRPC3) or t-test (TRPC1) based on the outcome of the normality test. Patch clamp data was compared by Mann–Whitney Rank Sum test.
Results
Determination of TRPC1/3 Expression in WKY and SHR CA
Spontaneously hypertensive rats and WKY CA both express mRNA for TRPC1 and TRPC3 (Figure 1A). PCR was run with and without reverse transcriptase to control for genomic DNA contamination. Protein expression for TRPC1 and TRPC3 was detected by Western blot in SHR and WKY CA (Figure 1B). Expression of TRPC3 appeared clearly increased in SHR arteries while TRPC1 appeared slightly decreased. Densitometric analysis of the blots (Figure 1C) revealed that TRPC3 was increased by 3.1 ± 1.2 times (P = 0.002; n = 6 each) in SHR compared to WKY, whereas TRPC1 expression was slightly decreased to 0.5 ± 0.2 times WKY expression (P = 0.014; n = 7 each). We also examined TRPC3 expression in cerebral arteries in SHR and WKY (Figure A1 in Appendix). TRPC3 expression was similarly upregulated in both the middle cerebral and basilar arteries.
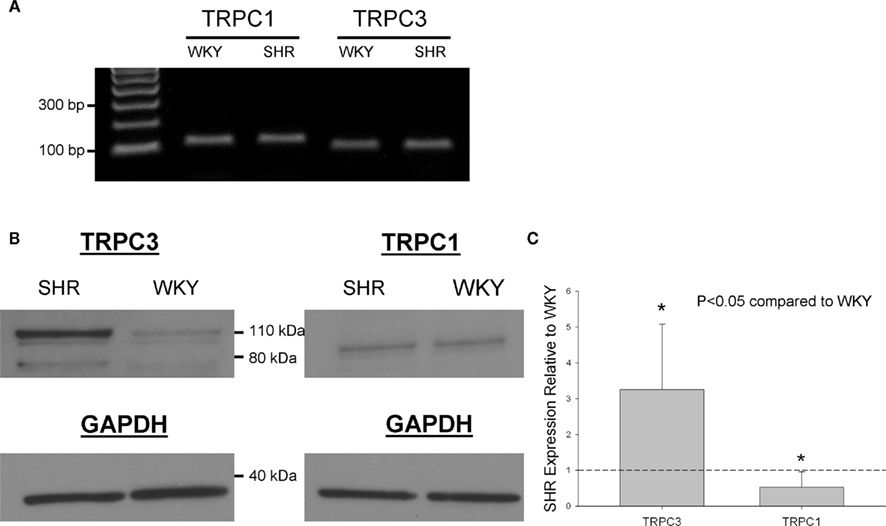
Figure 1. Expression of TRPC1 and TRPC3 mRNA and protein in WKY and SHR carotid artery (CA). (A) RT-PCR results comparing expression of TRPC1 and TRPC3 in rat CA of WKY and SHR. A 100-bp ladder is shown to the left. (B) Representative Western blots demonstrating TRPC1 and TRPC3 expression (upper panels) in rat CA of WKY and SHR. The lower portion of the membrane was probed with GAPDH (lower panels) for normalization. Mass standards for 110, 80, and 40 kDa are indicated. (C) Densitometric analysis of the band intensity of SHR compared to WKY. Data are presented as mean ± SEM for TRPC3 (n = 6 each) and TRPC1 (n = 7 each); * = P < 0.05 compared to WKY.
Evaluation of Vascular Contractility and TRP Channel Function in SHR and WKY Carotid Arteries
Isometric tension bath studies
UTP has been shown to promote vasoconstriction of pressurized cerebral arteries through activation of TRPC3 channels (Reading et al., 2005). Given the upregulation of TRPC3 in the SHR arteries we examined the effect of UTP stimulation on tension development.
Carotid arteries were denuded of the endothelium in order to focus on the effect of UTP on smooth muscle contraction. Removal of the endothelium was functionally confirmed by the absence of relaxation to 10 μM carbachol in KCl-contracted arteries. UTP (1–300 μM) CRC were obtained in the presence and absence of verapamil (10 μM) to determine the contribution of VDCC in the UTP-mediated vasocontraction. Absence of vasocontraction to KCl in the presence of verapamil confirmed the effectiveness of the VDCC blocker (Figure A2 in Appendix). We compared UTP CRC between SHR and WKY with and without verapamil (Figure 2A). In the absence of verapamil, UTP CRC were significantly potentiated in SHR compared to WKY (P < 0.05, n = 7–12). These data demonstrated a notable increase in vascular contractility to UTP in the hypertensive group. In the presence of verapamil, CA contractile response to UTP was significantly decreased in SHR (P < 0.05, n = 12–13) to a level comparable to WKY. Verapamil did not affect the contractile response to UTP in the WKY group (P = NS, n = 6–7). These data demonstrate that VDCC contribute to UTP-mediated vasocontraction in the SHR CA but not those of WKY.
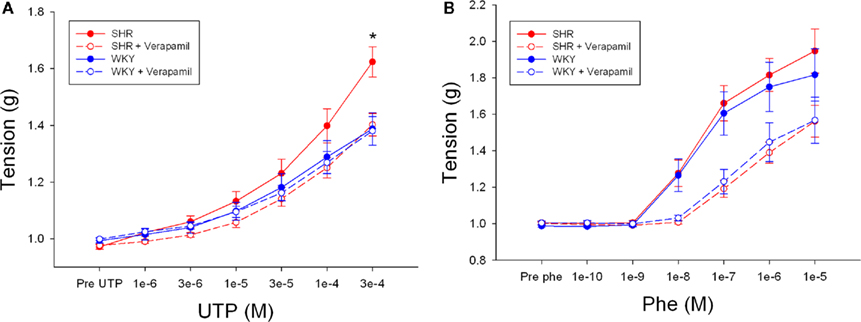
Figure 2. Concentration-response curves (CRC) to UTP (A) and Phe (B) for WKY (n = 5–7) and SHR endothelium-denuded CA (n = 11–13). Responses are presented for control (solid lines) and verapamil (dashed lines) for WKY and SHR. Data are presented as mean ± SEM; * = P < 0.05 by RM-ANOVA between WKY and SHR groups.
In order to determine if the greater contractility was specific to the UTP signaling pathway, we also performed CRC to phenylephrine (Phe). Constrictions to Phe (1 × 10−10 to 1 × 10−5 M) in endothelium-denuded arteries were similarly compared in the absence and presence of verapamil (Figure 2B). Addition of verapamil significantly attenuated the Phe response in both SHR and WKY groups. In contrast to UTP, however, addition of verapamil attenuated contractile response to Phe in both WKY and SHR and did not differ between SHR and WKY (P = NS, n = 5–11). Similar to the contractile response to UTP in the presence of verapamil, there was no difference in Phe-induced contraction between SHR and WKY in the presence of verapamil. Together, these data demonstrate a similar dependence on VDCC activation between SHR and WKY CA in the Phe signaling pathway.
We next examined the dependence of extracellular Ca2+ in the UTP-mediated constriction. In this protocol, we administered UTP twice with a washout in between. In the Ca2+-free group, we replaced the Ca2+-containing buffer with Ca2+-free buffer containing 0.1 mM EGTA just prior to the second UTP administration. In Ca2+-free conditions, UTP produced a small transient constriction (Figure 3). There was no difference in response to UTP in absence of extracellular Ca2+ between SHR (red) and WKY (black) groups. These data demonstrate that the constriction to UTP is substantially dependent on calcium influx for both groups.
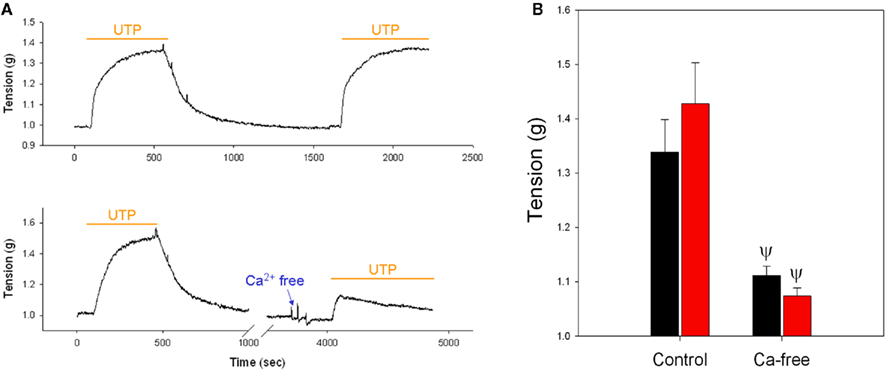
Figure 3. Summary of UTP-mediated constrictions (100 μM) between WKY and SHR. (A) Representative tension recordings in which Ca2+ was present throughout (upper recording) or removed between UTP administrations (lower recording). (B) Summary of first UTP constriction and second UTP constriction in Ca2+-free media for WKY and SHR CA (n = 6–8). Ψ = P < 0.05 compared to control response with paired t-test.
Patch Clamp Electrophysiology
TRP channel function was evaluated in freshly isolated CASMC by whole-cell patch clamp method. Whole-cell currents were recorded in the voltage-clamp mode over the voltage range of –110 to +80 mV (Figure 4). UTP activated non-selective cation currents (IUTP), which we previously demonstrated to be inhibited by 100 μM lanthanum chloride (non-selective TRPC channel blocker) and by intracellular application of TRPC3 and TRPC1 antibodies (Chen et al., 2009). IUTP was primarily inward and reversed around 0 mV. These currents were significantly greater in SHR compared to WKY and LE rats. The mean peak inward IUTP in the SHR CASMC at −110 mV was −63 ± 24 pA (n = 7) compared to −25 ± 4 pA in the pooled WKY and LE CASMC (P = 0.011, Mann–Whitney Rank Sum test). The LE (n = 15) and WKY (n = 5) responses were similar (−23 versus −32 pA) and thus pooled for comparison with the SHR. Median peak inward IUTP for each group was −23, −18.5, and −48 pA for LE, WKY, and SHR respectively.
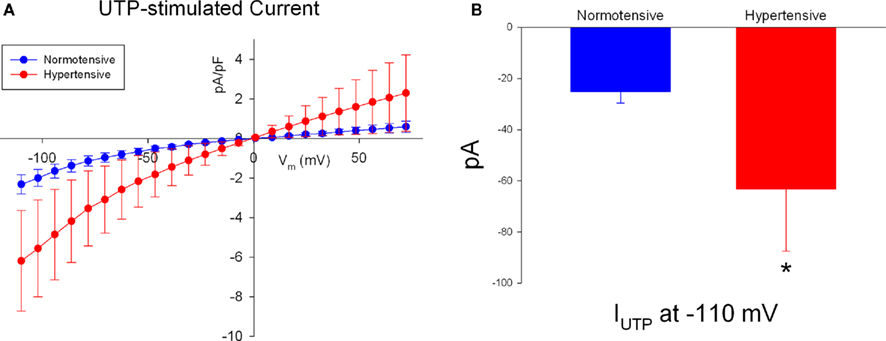
Figure 4. (A) Summary current-voltage (I–V) relationships demonstrating UTP-stimulated current for freshly isolated smooth muscle cells from normotensive WKY/LE and hypertensive SHR. Currents were normalized to cell capacitance. (B) Summary of UTP-stimulated current at −110 mV for WKY/LE (n = 20 cells) and SHR (n = 7 cells). Data are presented as mean ± SEM; * = P < 0.05 compared to WKY/LE.
Discussion
We present the following novel findings regarding the expression and function of TRPC1 and TRPC3 in CA of hypertensive rats: (1) TRPC3 protein expression is increased and TRPC1 protein is decreased in SHR CA; (2) SHR CA demonstrates greater contractility to UTP but not Phe; (3) VDCC contribute to contraction of SHR CA but not WKY CA; (4) UTP stimulates a TRPC-like current that is significantly greater in SHR CASMC. These findings are discussed in greater detail below.
Expression of TRPC1/3 in Hypertension
We examined the expression of TRPC1 and TRPC3 in rat CA in hypertensive SHR and normotensive WKY rats. TRPC3 protein expression was found to be significantly upregulated in SHR CA compared to WKY. These findings are consistent with what Liu et al. (2009) recently showed in SHR aorta and what Chen et al. (2010) found in SHR mesenteric artery. Together, these data suggest widespread TRPC3 upregulation in multiple vascular beds during hypertension.
TRPC1 has previously been demonstrated to assemble with TRPC3 endogenously and in expression systems (Lintschinger et al., 2000; Liu et al., 2005b; Zagranichnaya et al., 2005) and most recently endogenously in rat CA (Chen et al., 2009). Interestingly, TRPC1 protein in CA did not demonstrate a parallel increase in expression. Instead, TRPC1 expression was actually slightly decreased in SHR compared to WKY. This is in contrast to the study by Chen et al. (2010) that showed increased expression of both TRPC1 and TRPC3 in the mesenteric arterioles of the SHR.
TRPC Channel Function and Vascular Contractility in Hypertension
The major role of TRPC channels in smooth muscle contraction is often ascribed to activation of VDCCs through cell depolarization (Gudermann et al., 2004). In addition, L-type Ca2+ channels have been shown to be upregulated in mesenteric, skeletal muscle, and renal arteries in SHR (Pratt et al., 2002; Pesic et al., 2004). Given the apparent role of VDCCs in the mechanism of TRPC channel activation and the reported upregulation of VDCCs in other arteries in hypertension, we sought to determine the relative role of VDCCs in the CA of SHR and WKY as well. The role of VDCCs in the UTP and Phe-stimulated contraction was evaluated with verapamil using a concentration that completely inhibited KCl-mediated contractions. CRCs to UTP demonstrated that SHR (but not WKY) CAs were attenuated by verapamil. The residual verapamil-insensitive contraction was similar between SHR and WKY CAs.
There are two major points to make based on our findings with verapamil. First, VDCCs only contribute significantly to UTP-mediated contraction in CA of hypertensive rats whereas they contribute equally in Phe-mediated contraction. In CA of normotensive rats, the contraction to UTP is essentially independent of VDCC activation, though it is still heavily dependent on Ca2+ influx. This finding is consistent with the idea that a significant portion of Ca2+ influx, particularly in larger arteries, can be independent of VDCC activation (Albert et al., 2007). From our study, we know that VDCC are present and capable of mediating significant contraction since Phe and KCl-mediated contraction was significantly or completely blocked by verapamil. We also know that the UTP-mediated constriction requires Ca2+-influx, since the acute removal of Ca2+ resulted in substantial inhibition. Together, these data demonstrate that UTP stimulates Ca2+ influx that is independent of VDCC activation in WKY CA but becomes partially VDCC-dependent in SHR CA. In contrast to the UTP mechanism, the Phe signaling pathway appears to be similar between WKY and SHR and equally dependent on VDCC activation.
The second major point addresses the difference in verapamil sensitivity between SHR and WKY CA. The contribution of VDCCs is significantly increased in the SHR but not WKY CA. Increased expression and function of L-type Ca2+ channels has been reported for other SHR arteries (Pratt et al., 2002; Pesic et al., 2004). We have not measured expression of this VDCC in the CA, but our data argue against the potentiated constrictions being simply explained by increased expression of VDCC. Addition of Phe produced significant VDCC-dependent constriction in both SHR and WKY CA that was similar in magnitude. If the greater constriction to UTP were primarily due to upregulated VDCC in SHR arteries, one would expect the constrictions to Phe to be potentiated as well. Instead, our data favors a model in which coupling of the UTP receptor(s) with VDCC activation is greater in SHR CA. Increased expression of TRPC3 channels linked to UTP receptor activation would theoretically increase the magnitude of SMC depolarization and subsequently the level of VDCC activation. In support of this possibility, the SHR demonstrated greater UTP-stimulated whole-cell current in freshly isolated CASMCs. This current reversed at the same point (∼0 mV) as in the WKY cells and exhibited similar rectification properties. These results indicate that hypertension indeed results in increased function of TRPC-like channels capable of producing a depolarizing current. While we previously demonstrated a role for both TRPC1 and TRPC3 channels in the UTP-stimulated current in normotensive CASMCs (Chen et al., 2009), further studies will be needed to confirm the molecular identity of the channel(s) responsible for the increased current in SHR CASMCs.
While TRPC1 channels have been shown to be mediators of store operated Ca2+ entry in smooth muscle cells (Xu and Beech, 2001) and possibly contribute to vasoconstriction (Wolfle et al., 2010), these channels have also been described in a mechanism of smooth muscle relaxation. In this latter model, TRPC1 channels form a functional unit with BKCa channels in which Ca2+ influx through TRPC1 promotes smooth muscle hyperpolarization through activation of associated BKCa channels (Kwan et al., 2009). If this model is valid for intact CA, then a decrease in TRPC1 expression would be expected to reduce BKCa channel activation during agonist stimulation. This reduced BKCa channel activation would permit greater smooth muscle depolarization and VDCC activation, ultimately leading to greater contraction. While this scenario is consistent with our findings of greater contractility to UTP and a greater involvement of VDCC in SHR CA, considerably more studies would be required to demonstrate any altered role of BKCa channels in SHR as well as a functional link to TRPC1 in this artery.
In summary, we report that UTP-mediated constrictions are potentiated in the CA during hypertension. Examination of expression levels of TRPC1 and TRPC3 demonstrated that TRPC1 is decreased whereas TRPC3 is increased in SHR CA. In addition, we demonstrate that VDCC activation plays a role in UTP-mediated contraction of hypertensive but not normotensive CAs. We propose that increased TRPC3 channel expression in hypertension leads to greater Ca2+ and Na+ influx, which provides Ca2+ directly (through TRPC3 channel) and indirectly (through VDCC activation) for smooth muscle contraction. The decreased expression of TRPC1 was contrary to what we expected. However, given the reported link between TRPC1 and BKCa channel activation, a possible explanation is that decreased TRPC1 expression leads to reduced BKCa channel activation in SHR smooth muscle. When taken as a whole, it is clear from these and other recent studies that expression of multiple TRPC channel members is altered in hypertension with significant functional consequence in the vasculature.
Conflict of Interest Statement
The authors declare that the research was conducted in the absence of any commercial or financial relationships that could be construed as a potential conflict of interest.
Acknowledgments
These studies were supported by NIH R01 HL088435 to Sean P. Marrelli. We thank Dr. Jie Chen for performing the patch clamp measurements.
References
Abramowitz, J., and Birnbaumer, L. (2009). Physiology, and pathophysiology of canonical transient receptor potential channels. FASEB J. 23, 297–328.
Albert, A. P., Saleh, S. N., Peppiatt-Wildman, C. M., and Large, W. A. (2007). Multiple activation mechanisms of store-operated TRPC channels in smooth muscle cells. J. Physiol. 583, 25–36.
Alvarez, J., Coulombe, A., Cazorla, O., Ugur, M., Rauzier, J. M., Magyar, J., Mathieu, E. L., Boulay, G., Souto, R., Bideaux, P., Salazar, G., Rassendren, F., Lacampagne, A., Fauconnier, J., and Vassort, G. (2008). ATP/UTP activate cation-permeable channels with TRPC3/7 properties in rat cardiomyocytes. Am. J. Physiol. Heart Circ. Physiol. 295, H21–H28.
Bendhack, L. M., Sharma, R. V., and Bhalla, R. C. (1992). Altered signal transduction in vascular smooth muscle cells of spontaneously hypertensive rats. Hypertension 19, II142–II148.
Bergdahl, A., Gomez, M. F., Wihlborg, A. K., Erlinge, D., Eyjolfson, A., Xu, S. Z., Beech, D. J., Dreja, K., and Hellstrand, P. (2005). Plasticity of TRPC expression in arterial smooth muscle: correlation with store-operated Ca2+ entry. Am. J. Physiol. Cell Physiol. 288, C872–C880.
Chen, J., Crossland, R. F., Noorani, M. M., and Marrelli, S. P. (2009). Inhibition of TRPC1/TRPC3 by PKG contributes to NO-mediated vasorelaxation. Am. J. Physiol. Heart Circ. Physiol. 297, H417–H424.
Chen, X., Yang, D., Ma, S., He, H., Luo, Z., Feng, X., Cao, T., Ma, L., Yan, Z., Liu, D., Tepel, M., and Zhu, Z. (2010). Increased rhythmicity in hypertensive arterial smooth muscle is linked to transient receptor potential canonical channels. J. Cell. Mol. Med. 14, 2483–2494.
Clapham, D. E., Julius, D., Montell, C., and Schultz, G. (2005). International Union of Pharmacology. XLIX. Nomenclature, and structure-function relationships of transient receptor potential channels. Pharmacol. Rev. 57, 427–450.
Dietrich, A., Kalwa, H., Storch, U., Schnitzler, M., Salanova, B., Pinkenburg, O., Dubrovska, G., Essin, K., Gollasch, M., Birnbaumer, L., and Gudermann, T. (2007).Pressure-induced, and store-operated cation influx in vascular smooth muscle cells is independent of TRPC1. Pflugers Arch. 455, 465–477.
Gudermann, T., Schnitzler, M., and Dietrich, A. (2004). Receptor-operated cation entry–more than esoteric terminology? Sci. STKE 2004, e35.
Hofmann, F., Lacinova, L., and Klugbauer, N. (1999).Voltage-dependent calcium channels: from structure to function. Rev. Physiol. Biochem. Pharmacol. 139, 33–87.
Hurst, R. S., Zhu, X., Boulay, G., Birnbaumer, L., and Stefani, E. (1998). Ionic currents underlying HTRP3 mediated agonist-dependent Ca2+ influx in stably transfected HEK293 cells. FEBS Lett. 422, 333–338.
Inoue, R., Morita, H., and Ito, Y. (2004). Newly emerging Ca2+ entry channel molecules that regulate the vascular tone. Expert Opin. Ther. Targets 8, 321–334.
Kumar, B., Dreja, K., Shah, S. S., Cheong, A., Xu, S. Z., Sukumar, P., Naylor, J., Forte, A., Cipollaro, M., McHugh, D., Kingston, P. A., Heagerty, A. M., Munsch, C. M., Bergdahl, A., Hultgardh-Nilsson, A., Gomez, M. F., Porter, K. E., Hellstrand, P., and Beech, D. J. (2006). Upregulated TRPC1 channel in vascular injury in vivo, and its role in human neointimal hyperplasia. Circ. Res. 98, 557–563.
Kunichika, N., Yu, Y., Remillard, C. V., Platoshyn, O., Zhang, S., and Yuan, J. X. (2004). Overexpression of TRPC1 enhances pulmonary vasoconstriction induced by capacitative Ca2+ entry. Am. J. Physiol. Lung Cell. Mol. Physiol. 287, L962–L969.
Kuriyama, H., Kitamura, K., Itoh, T., and Inoue, R. (1998). Physiological features of visceral smooth muscle cells, with special reference to receptors, and ion channels. Physiol. Rev. 78, 811–920.
Kwan, H. Y., Shen, B., Ma, X., Kwok, Y. C., Huang, Y., Man, Y. B., Yu, S., and Yao, X. (2009). TRPC1 associates with BK(Ca) channel to form a signal complex in vascular smooth muscle cells. Circ. Res. 104, 670–678.
Large, W. A. (2002). Receptor-operated Ca2(+)-permeable nonselective cation channels in vascular smooth muscle: a physiologic perspective. J. Cardiovasc. Electrophysiol. 13, 493–501.
Lin, M. J., Leung, G. P., Zhang, W. M., Yang, X. R., Yip, K. P., Tse, C. M., and Sham, J. S. (2004). Chronic hypoxia-induced upregulation of store-operated, and receptor-operated Ca2+ channels in pulmonary arterial smooth muscle cells: a novel mechanism of hypoxic pulmonary hypertension. Circ. Res. 95, 496–505.
Lintschinger, B., Balzer-Geldsetzer, M., Baskaran, T., Graier, W. F., Romanin, C., Zhu, M. X., and Groschner, K. (2000). Coassembly of Trp1, and Trp3 proteins generates diacylglycerol-, and Ca2+-sensitive cation channels. J. Biol. Chem. 275, 27799–27805.
Liu, D., Scholze, A., Zhu, Z., Kreutz, R., Wehland-von-Trebra, M., Zidek, W., and Tepel, M. (2005a). Increased transient receptor potential channel TRPC3 expression in spontaneously hypertensive rats. Am. J. Hypertens. 18, 1503–1507.
Liu, X., Bandyopadhyay, B. C., Singh, B. B., Groschner, K., and Ambudkar, I. S. (2005b). Molecular analysis of a store-operated, and 2-acetyl-sn-glycerol-sensitive non-selective cation channel. Heteromeric assembly of TRPC1-TRPC3. J. Biol. Chem. 280, 21600–21606.
Liu, D., Scholze, A., Zhu, Z., Krueger, K., Thilo, F., Burkert, A., Streffer, K., Holz, S., Harteneck, C., Zidek, W., and Tepel, M. (2006). Transient receptor potential channels in essential hypertension. J. Hypertens. 24, 1105–1114.
Liu, D., Yang, D., He, H., Chen, X., Cao, T., Feng, X., Ma, L., Luo, Z., Wang, L., Yan, Z., Zhu, Z., and Tepel, M. (2009). Increased transient receptor potential canonical type 3 channels in vasculature from hypertensive rats. Hypertension 53, 70–76.
McDonald, T. F., Pelzer, S., Trautwein, W., and Pelzer, D. J. (1994). Regulation, and modulation of calcium channels in cardiac, skeletal, and smooth muscle cells. Physiol. Rev. 74, 365–507.
Nelson, M. T., Patlak, J. B., Worley, J. F., and Standen, N. B. (1990). Calcium channels, potassium channels, and voltage dependence of arterial smooth muscle tone. Am. J. Physiol. 259, C3–C18.
Ohba, T., Watanabe, H., Murakami, M., Takahashi, Y., Iino, K., Kuromitsu, S., Mori, Y., Ono, K., Iijima, T., and Ito, H. (2007). Upregulation of TRPC1 in the development of cardiac hypertrophy. J. Mol. Cell Cardiol. 42, 498–507.
Peppiatt-Wildman, C. M., Albert, A. P., Saleh, S. N., and Large, W. A. (2007). Endothelin-1 activates a Ca2+-permeable cation channel with TRPC3, and TRPC7 properties in rabbit coronary artery myocytes. J. Physiol. 580, 755–764.
Pesic, A., Madden, J. A., Pesic, M., and Rusch, N. J. (2004). High blood pressure upregulates arterial L-type Ca2+ channels: is membrane depolarization the signal? Circ. Res. 94, e97–e104.
Pratt, P. F., Bonnet, S., Ludwig, L. M., Bonnet, P., and Rusch, N. J. (2002). Upregulation of L-type Ca2+ channels in mesenteric, and skeletal arteries of SHR. Hypertension 40, 214–219.
Ramsey, I. S., Delling, M., and Clapham, D. E. (2006). An introduction to TRP channels. Annu. Rev. Physiol. 68, 619–647.
Reading, S. A., Earley, S., Waldron, B. J., Welsh, D. G., and Brayden, J. E. (2005). TRPC3 mediates pyrimidine receptor-induced depolarization of cerebral arteries. Am. J. Physiol. Heart Circ. Physiol. 288, H2055–H2061.
Sugiyama, T., Yoshizumi, M., Takaku, F., and Yazaki, Y. (1990). Abnormal calcium handling in vascular smooth muscle cells of spontaneously hypertensive rats. J. Hypertens. 8, 369–375.
Taylor, C. W., and Moneer, Z. (2004). Regulation of capacitative, and non-capacitative Ca2+ entry in A7r5 vascular smooth muscle cells. Biol. Res. 37, 641–645.
Touyz, R. M., and Schiffrin, E. L. (1997). Role of calcium influx, and intracellular calcium stores in angiotensin II-mediated calcium hyper-responsiveness in smooth muscle from spontaneously hypertensive rats. J. Hypertens. 15, 1431–1439.
Welsh, D. G., Morielli, A. D., Nelson, M. T., and Brayden, J. E. (2002). Transient receptor potential channels regulate myogenic tone of resistance arteries. Circ. Res. 90, 248–250.
Wolfle, S. E., Navarro-Gonzalez, M. F., Grayson, T. H., Stricker, C., and Hill, C. E. (2010). Involvement of nonselective cation channels in the depolarisation initiating vasomotion. Clin. Exp. Pharmacol. Physiol. 37, 536–543.
Keywords: calcium, carotid artery, hypertension, transient receptor potential channel, vascular smooth muscle
Citation: Noorani MM, Noel RC and Marrelli SP (2011) Upregulated TRPC3 and downregulated TRPC1 channel expression during hypertension is associated with increased vascular contractility in rat. Front. Physio. 2:42. doi: 10.3389/fphys.2011.00042
Received: 13 May 2011;
Accepted: 11 July 2011;
Published online: 22 July 2011.
Edited by:
John D. Imig, Medical College of Wisconsin, USAReviewed by:
Kathryn M. Gauthier, Medical College of Wisconsin, USAXueying Zhao, Morehouse School of Medicine, USA
Ahmed A. Elmarakby, Georgia Health Science University, USA
Copyright: © 2011 Noorani, Noel and Marrelli. This is an open-access article subject to a non-exclusive license between the authors and Frontiers Media SA, which permits use, distribution and reproduction in other forums, provided the original authors and source are credited and other Frontiers conditions are complied with.
*Correspondence: Sean P. Marrelli, Baylor College of Medicine, Room 434-D, Houston, TX 77030, USA. e-mail:bWFycmVsbGlAYmNtLmVkdQ==