- Institute of Biology, University of Southern Denmark, Odense, Denmark
Water balance in teleost fish is maintained with contributions from the major osmoregulatory organs: intestine, gills, and kidney. Overall water fluxes have been studied in all of these organs but not until recently has it become possible to approach the mechanisms of water transport at the molecular level. This mini-review addresses the role of the kidney in osmoregulation with special emphasis on euryhaline teleosts. After a short review of current knowledge of renal functional morphology and regulation, we turn the focus to recent molecular investigations of the role of aquaporins in water and solute transport in the teleost kidney. We conclude that there is much to be achieved in understanding water transport and its regulation in the teleost kidney and that effort should be put into systematic mapping of aquaporins to their tubular as well as cellular localization.
Introduction
Multiple aquaporins have been functionally characterized and mapped along the nephron in the mammalian kidney, where they play a pivotal role in the maintenance of water homeostasis (Nielsen et al., 2002). Teleost kidneys are unable to produce hyperosmotic urine due to the lack of a loop of Henle, yet the role of aquaporins is suspected to be related to conservation of water in seawater (SW) and possibly excretion of water in freshwater (FW). In 2010 Cerdà and Finn summarized that 11 orthologs have been reported in whole kidney tissue (incl. vasculature and supporting tissues) of various teleosts: aqp-1aa, -1ab, -3a, -3b, -7, -8aa, -8ab, -9a, -10a, 10b, and -12. We have recently localized yet a paralog of aqp8 – termed aqp8b in the kidney of Atlantic salmon (Salmo salar) and rainbow trout (Oncorhynchus mykiss; Figures 1E,F). Only a few aquaporins have been localized in specific tubule cells, and their dynamics and functional role are far from known (Table 1).
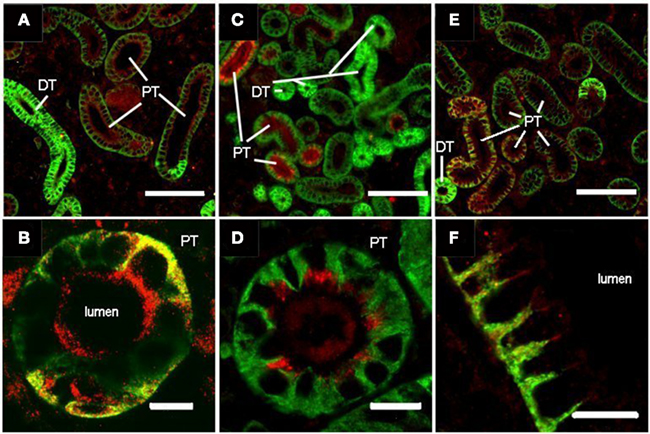
Figure 1. Localization of three aquaporins (Aqp) isoforms in the FW rainbow trout kidney: Aqp1aa (A,B), Aqp1ab (C,D), and Aqp8b (E,F). Sections were prepared as described in Madsen et al. (in review). Sections were double stained with polyclonal rabbit antibodies against S. salar Aqps (red) and monoclonal mouse α-5 against the α-subunit of Na+, K+-ATPase (green; The Developmental Studies Hybridoma Bank developed under auspices of the NICHD and maintained by The University of Iowa, Department of Biological Sciences, Iowa City, IA, USA) and visualized using a cocktail of secondary goat antibodies (Alexa Fluor® 568 anti-rabbit and Oregon Green® 488 anti-mouse. Yellow color appears where the two antibodies co-localize. Letters show different tubule segments distinguished by the cellular localization and abundance of Na+, K+-ATPase (basolateral, less abundant: proximal tubules, PT; entire cell, more abundant: distal tubules, DT). Aqp1aa stains the brush border and some basolateral membrane of PT, Aqp1ab is present subapically and in brush border of PT and Aqp8b is present in both apical and basolateral positions in some proximal tubules. Size bars are 100 and 10 μm in upper and lower panels, respectively.
Functional Morphology of the Kidney in Euryhaline Teleosts
Freshwater-fish encounter osmotic water load from the environment and the primary function of the filtrating kidney is to produce large amounts of hypotonic urine. Salts are inevitably lost in this process as well as across gills and skin, which is compensated for by branchial and dietary NaCl uptake. SW fish are threatened by dehydration and compensate by ingesting and absorbing SW followed by excretion of excess salts in the gill. The kidney switches to water saving with excretion of divalent ions as the main function. Thus euryhaline fish are able to make major adjustments in renal function as the salinity changes (Beyenbach, 2004).
The mesonephric teleost kidney has a poor 3-dimensional organization: It lacks a well defined cortex, medulla, and a loop of Henle which is characteristic of the metanephric kidney of mammals. Vasculature, glomeruli, and tubular segments are interwoven and interspersed with hematopoietic tissue especially in the anterior part (Anderson and Loewen, 1975; Resende et al., 2010). The number and size of glomeruli as well as the differentiation of functional segments of teleost nephrons are reduced compared to the mammalian type, and vary according to evolutionary origin and habitat. In euryhaline teleosts, glomeruli with closed Bowman’s capsules precede proximal tubule segments I and II followed by distal and collecting tubules and collecting ducts which drain into the mesonephric duct. Paired mesonephric ducts then merge distally forming the urinary bladder, which further drains through the urogenital papilla (see Hickman and Trump, 1969).
Glomerular filtration (GFR) varies between species and is absent in a few aglomerular marine species (Beyenbach, 2004). GFR is typically 7–10× higher in FW than in SW, reflecting the contrasting demands in the two habitats (Brown et al., 1978; Elger et al., 1987; McDonald, 2007). This active adjustment of GFR according to hydrational status is markedly different from mammals and is achieved through vascular adjustments serving to change perfusion pressure and recruitment of individual nephrons (McDonald, 2007). In rainbow trout (Oncorhynchus mykiss) <50% of the nephrons are filtering in FW fish (Brown et al., 1980). This number decreases to about 5% in SW-acclimated fish (McDonald, 2007) but the average single nephron GFR (SNGFR) is almost three times higher in SW- than in FW-acclimated trout (Brown et al., 1978). This illustrates that fish kidneys may undergo a major switch from being filtratory in FW to being predominantly secretory in SW.
The function of the proximal segment(s) of the kidney tubule is somewhat controversial. There is, however, consensus that one primary function is secretion of Mg2+ especially in marine and SW-acclimated species. Mg2+ secretion involves apical exocytosis of vesicles enriched with Mg2+ (Renfro, 1999). Accompanying fluid secretion may occur in both filtering and non-filtering nephrons but would be expected to occur particularly in SW fish in order to facilitate Mg2+-secretion in tubules with low GFR. In FW-kidneys it could play an additional role in excretion of excess water (Beyenbach, 2004). With regard to NaCl and water transport, available data are more diverging. In some species, there is evidence that the proximal segments of both FW and SW fish are responsible for absorption of Na+ and Cl− as wells as glucose and other important osmolytes (Nishimura and Imai, 1982; Dantzler, 2003; Beyenbach, 2004). The mechanism for this builds on basolateral Na+, K+-ATPase (Katoh et al., 2008; Teranishi and Kaneko, 2010). The apical Na+-entry pathway into proximal cells is yet unclear but may involve Na+/H+-exchange (Braun and Dantzler, 1997; Ivanis et al., 2008) and Na+-glucose cotransporters (SGLT1). The role of SGLT1 has generally not been investigated much in teleost kidneys but its mRNA expression is indeed very high in mixed renal tissue of rainbow trout (Madsen, unpublished). In little skate (Leucoraja erinacea) SGLT1 is present in proximal as well as distal tubules (Althoff et al., 2007). Like in mammals, the absorptive-type Na+, K+, 2Cl− cotransporter (NKCC2) is absent in fish in this segment (Katoh et al., 2008). In accordance with the mammalian model it was earlier believed that some trans- and para-cellular water absorption occurred in the proximal segments driven by a suspected locally established osmotic gradient (Hickman and Trump, 1969). This would make good sense in a SW fish but not in FW fish, where body water is excessive. This model is, however, contradicted by more recent demonstrations of basolateral secretory-type NKCC1 at least in killifish (Fundulus heteroclitus) proximal segments (Katoh et al., 2008), suggesting a pathway for NaCl secretion which may occur in conjunction with Mg2+ secretion and thus set up a reverse osmotic gradient facilitating water secretion in this segment (Beyenbach, 2004). Secreted NaCl would be expected to be reabsorbed in the distal and collecting segments. In SW fish, branchial NaCl secretion would easily compensate for this recycling of salt in the kidney. It is further possible that processes operate differently in PI and PII segments and in different teleosts.
Distal tubules and collecting ducts together make up a variable percentage of whole nephrons in different fish (Katoh et al., 2008) and the primary activity here is reabsorption of NaCl in both FW and SW. This is favored by extensive expression of basolateral Na+, K+-ATPase, apical NKCC2 (Katoh et al., 2008) and NaCl cotransporter (NCC; Kato et al., 2011), and basolateral kidney-specific Cl-channels (Miyazaki et al., 2002). Accordingly the distal segment is absent in the majority of truly marine species (Hickman and Trump, 1969). In FW fish the distal segments are proposed to have low water permeability in order to minimize water reabsorption and promote the formation of hypotonic urine (McDonald, 2007). Upon acclimation to SW, the fractional reabsorption of water increases along the nephron by increasing tubular water permeability as seen in mammalian collecting tubules. Reabsorption of NaCl may promote the osmotic removal of water – thereby creating isotonic urine primarily consisting of MgSO4 and other unwanted osmolytes (Beyenbach, 2004).
Surprisingly little information is available about the molecular mechanisms of tubular water movement and how it is controlled in fish. Among the most obvious candidates for renal control are arginine vasotocin and angiotensin which have vascular effects that decrease SNGFR and number of filtering nephrons and thereby exert a profound antidiuretic effect (Amer and Brown, 1995; Brown et al., 2000). Prolactin, cortisol, and growth hormone are also suspected to play important roles in modulation of renal water and ion homeostasis (Manzon, 2002; Martinez et al., 2005; Breves et al., 2011), however, there is very little concrete evidence.
Aquaporins in the Teleost Kidney
Aquaporin 1
Aquaporin 1 exists as two paralogs in teleosts: aqp1aa and aqp1ab (Cerdà and Finn, 2010). The first report of kidney aquaporins was made by Martinez et al. (2005), who found two classic aquaporins (aqp1 and aqp1dup – now aqp1ab) and one aquaglyceroporin (aqpe – now aqp10b, Tingaud-Sequeira et al., 2010) in FW- and SW-acclimated European eels (Anguilla anguilla). The mRNA expression of these declined during SW-acclimation – and also partly during the pre-migratory metamorphosis from the yellow to silver form. A somewhat similar expression pattern was reported in black porgy (Acanthopagrus schlegeli; An et al., 2008), where aqp1 expression varied according to salinity in the order: 10% SW > FW > SW. This response is opposite of what would be expected, if these aquaporins are involved in tubular water absorption, and accordingly the authors concluded that aqp1 may be involved in water secretion. In contrast, the expression of aqp1 mRNA in the kidney of sea bass (Dicentrarchus labrax) was four to five times higher in SW- than in FW-acclimated fish (Giffard-Mena et al., 2007). In Atlantic salmon, Tipsmark et al. (2010) reported increasing mRNA levels of aqp1aa during SW-acclimation (and smoltification), but this was accompanied by a concomitant decrease in the mRNA of aqp1ab, suggesting that these paralogs play differential roles in water homeostasis in the Atlantic salmon kidney. The bass and salmon studies agree well, since the sea-bass aqp1 ortholog is more similar to the aa- than the ab-ortholog of Atlantic salmon aqp1. In stenohaline zebrafish kidney only one aqp1 paralog is found (aqp1aa), which adds further complexity to the physiological roles of aqp1 (Tingaud-Sequeira et al., 2010). At the moment it is unclear to what degree the diverging results obtained for aqp1 can be explained by species differences or the varying experimental designs.
There is very little knowledge of the localization of Aqp1 protein isoforms along the teleost nephron. Martinez et al. (2005) found positive immunoreaction in the eel kidney using a homologous antibody. Staining was generally present in the endothelium in yellow eels; but in both FW and SW silver eels a “subset” of renal tubules (presumably proximal) exhibited strong staining in the brush border zone of epithelial cells. No attempts were made to further determine the segmental origin of these tubules. Aqp1aa also appeared in apical localization of renal tubules of SW-acclimated gilthead sea bream (Sparus aurata, Cerdà and Finn, 2010). Initial studies of rainbow trout show a clear segmentation of Aqp1 protein isoforms in renal tubules. Irrespective of salinity, Aqp1aa is present in the apical and basolateral membrane of proximal tubules, judged from the intensity and pattern of Na+, K+-ATPase localization (Figures 1A,B). The Na+, K+-ATPase is present basolaterally in all tubule segments but is more abundant in the highly folded membranes of distal and collecting tubules than in proximal segments of the nephron (Katoh et al., 2008) allowing differentiation between tubule segments. Aqp1ab is found in the apical brush border of proximal and distal tubules in SW-acclimated rainbow trout (not shown), while being predominantly withdrawn to a subapical position in proximal tubules in FW-acclimated rainbow trout (Figures 1C,D). This suggests that trafficking may contribute to the regulation of this isoform as has been reported for fish oocytes (Fabra et al., 2005, 2006; Chaube et al., 2011) and also discussed for intestinal Aqp1ab elsewhere in this Special Topic (Madsen et al., in review).
Only one study has reported hormonal effects on kidney aqp1 mRNA. Martinez et al. (2005) found that expression of aqp1aa and aqp1ab in yellow eels was suppressed by cortisol, whereas the hormone had no effect in silver eels, where the mRNAs were already at lower levels. This confirms the conception of cortisol being a SW-adapting hormone in eel.
Aquaporin 2
In strong contrast to mammals, this isoform has not been detected in teleosts (Cerdà and Finn, 2010). However, one study has documented aquaporin 2 in the kidney of African lungfish (Protopterus annectens; Konno et al., 2010), where mRNA and protein levels increased during aestivation. Moreover, Aqp2 was localized to the apical membrane of late distal tubule cells, where the vasopressin/vasotocin-V2 type receptor was present basolaterally. This suggests that water deficiency in the fish world may have promoted convergent evolution of a mechanism for renal water conservation similar to that responsible for urine concentration in mammals (Nielsen et al., 2002).
Aquaporin 3
The aquaglyceroporin aqp3 exists as duplicate paralogs in zebrafish: aqp3a and aqp3b (Cerdà and Finn, 2010) but only aqp3a mRNA is present in the kidney of zebrafish. Aqp3b mRNA was first reported absent from kidneys of SW-acclimated eel using Northern blotting (Cutler and Cramb, 2002). Later, the same group used RT-PCR to detect low levels of aqp3b mRNA in renal tissues which increased upon SW-acclimation (Cutler et al., 2007). This agrees with tilapia (Oreochromis mossambicus), where aqp3a mRNA is present in higher levels in SW- than FW-kidney (n = 1; Watanabe et al., 2005), and with Atlantic salmon where renal aqp3a mRNA increased >10-fold within 24 h after FW- to SW-transfer and stayed elevated for 2 weeks (Tipsmark et al., 2010). The same trend was seen in sea bass (Giffard-Mena et al., 2007), though not confirmed in a subsequent study (Giffard-Mena et al., 2008). In silver sea bream (Sparus sarba) the Aqp3a protein was detected by immunoblotting using homologous antibodies but no change was seen upon acclimation to a range of salinities (Deane and Woo, 2006). Finally, Kim et al. (2010) found no expression of aqp3b in kidney of Japanese eel (A. japonica). From this comparison, it seems that the aqp3b isoform is either absent or present in rather low levels in fish kidneys. In those species where aqp3a is expressed, the abundance is higher and mostly increases in response to hyperosmotic conditions. Thus Aqp3a may have a role in water conservation. A supplemental role in glycerol and urea transport is another putative function of Aqp3 (MacIver et al., 2009).
There is only one preliminary report of renal Aqp3 localization. Using a homologous antibody Cutler et al. (2007) detected Aqp3b protein in the apical domain of eel renal tubule cells (Cutler et al., 2007). This contrasts the basolateral location in distal segments of the mammalian kidney, where it serves a role in cellular water exit (Nielsen et al., 2002).
Aquaporin 8
In mammals, the role of renal AQP8 is unclear, since it is predominantly located in intracellular vesicles in proximal tubule and collecting duct cells (Elkjær et al., 2001). In addition to water, this isoform may also transport ammonium and thus be involved in acid–base control (Liu et al., 2006). In fish, there is very little information regarding the expression and function of this isoform – mostly related to intestinal function (Cutler et al., 2009; Kim et al., 2010; Madsen et al., in review). In zebrafish kidney, two paralogs of aqp8 are expressed: aqp8aa and aqp8ab (Tingaud-Sequeira et al., 2010), whereas no expression of these paralogs was detected in Atlantic salmon (aqp-8aa and -8ab: Tipsmark et al., 2010) or Japanese eel (aqp8aa: Kim et al., 2010). However, we have recently discovered a third paralog of aqp8 (aqp8b) in salmon, which is abundantly expressed in both intestinal and renal tissue. This paralog is also found in other teleost species but surprisingly not in the intestine or kidney of zebrafish (Cerdà and Finn, 2010; Tingaud-Sequeira et al., 2010). Preliminary results using homologous antibodies show a predominant staining of the basolateral membrane of some renal tubule cells in FW-acclimated rainbow trout (Figures 1E,F). We suspect that these tubules are proximal based on the moderate basolateral staining for Na+, K+-ATPase. This is the first demonstration of a basolateral Aqp8 in fish kidney tubules, which may serve together with the Aqp1aa isoform as important exit pathways for reabsorbed water.
Aquaporin 10
Aquaporin 10, another aquaglyceroporin, is not expressed in the kidneys of mammals but is located in the apical membrane of the small intestine, where it is suspected to participate in transport of water and small solutes (Hatakeyama et al., 2001; Mobasheri et al., 2004). In zebrafish kidney, aqp10 is expressed as two paralogs: aqp10a and aqp10b (Cerdà and Finn, 2010). Orthologs of aqp10b were also identified in kidneys of SW-acclimated gilthead sea bream (sbaqp: Santos et al., 2004), European eel (aqpe: Martinez et al., 2005), and Atlantic salmon (Tipsmark et al., 2010). In sea bream, aqp10b mRNA was demonstrated by in situ hybridization in tubule cells of undefined origin. Functional assays using Xenopus oocytes have further shown that this aquaglyceroporin is capable of water, glycerol and urea uptake (Santos et al., 2004; MacIver et al., 2009). In the eel study, SW-acclimation was associated with lower levels of aqp10b mRNA in yellow eels, whereas silver eels showed no response. In salmon, the effect of salinity was opposite; aqp10b expression was increased fivefold at 8 days after transfer to SW. Even though species differences seem to exist, all available evidence points to a salinity dependent expression and thus a role of Aqp10b in fluid handling in the teleost kidney. The endocrine regulation of this isoform remains unknown.
Additional Aquaporins
Aquaglyceroporins 7 and 9 and one unorthodox aquaporin 12 have been reported in the zebrafish kidney (Tingaud-Sequeira et al., 2010) but no information is available on their functional characteristics, tubular localization, nor their expression dynamics. In mammals, AQP7 is present in brush border membranes of proximal tubules, where it is suspected a role in glycerol metabolism (Noda et al., 2010). AQP9 and -12 have not been located in the kidney of mammals (Nielsen et al., 2002).
Conclusion and Perspectives for Future Research
Euryhaline teleosts have a capacity to acclimate to salinities ranging from strongly hypotonic to strongly hypertonic. In doing so their kidney function is dramatically altered. Despite this fact, surprisingly few studies have addressed the role and dynamics of aquaporins in fish kidneys. These have so far only probed for transcriptional regulation of few aquaporins and found interesting though conflicting results. Most data have demonstrated apical aquaporin expression in renal tubules, and future studies are encouraged to systematically investigate the specific protein isoforms, their dynamics and cellular expression as well as their hormonal and environmental regulation. This review has tried to show that there is still much to learn about water homeostasis in fish.
Conflict of Interest Statement
The authors declare that the research was conducted in the absence of any commercial or financial relationships that could be construed as a potential conflict of interest.
Acknowledgments
Steffen S. Madsen was supported by a grant from the Danish National Research Foundation (FNU, 09-070689).
References
Althoff, T., Hentschel, H., Luig, J., Schütz, H., Kasch, M., and Kinne, R. K. H. (2007). Na+-D-glucose cotransporter in the kidney of Leucoraja erinacea: molecular identification and intrarenal distribution. Am. J. Physiol. Regul. Integr. Comp. Physiol. 292, R2391–R2399.
Amer, S., and Brown, J. A. (1995). Glomerular actions of arginine vasotocin in the in-situ perfused trout kidney. Am. J. Physiol. Regul. Integr. Comp. Physiol. 269, R775–R780.
An, K. W., Kim, N. N., and Choi, C. Y. (2008). Cloning and expression of aquaporin 1 and arginine vasotocin receptor mRNA from the black porgy, Acanthopagrus schlegeli: effect of freshwater acclimation. Fish Physiol. Biochem. 34, 185–194.
Anderson, B. G., and Loewen, R. D. (1975). Renal morphology of freshwater trout. Am. J. Anat. 143, 93–114.
Aoki, M., Kaneko, T., Katoh, F., Hasegawa, S., Tsutsui, N., and Aida, K. (2003). Intestinal water absorption through aquaporin 1 expressed in the apical membrane of mucosal epithelial cells in seawater-adapted Japanese eel. J. Exp. Biol. 206, 3495–3505.
Braun, E. J., and Dantzler, W. H. (1997). “Vertebrate renal system,” in Handbook of Physiology, Vol. 1, Section 13. Comparative Physiology, ed. W. H. Dantzler (New York: Oxford University Press), 481–576.
Breves, J. P., Seale, A. P., Helms, R. E., Tipsmark, C. K., Hirano, T., and Grau, E. G. (2011). Dynamic gene expression of GH/PRL-family hormone receptors in gill and kidney during freshwater-acclimation of Mozambique tilapia. Comp. Biochem. Physiol. 158A, 194–200.
Brown, J. A., Jackson, B. A., Oliver, J. A., and Henderson, I. W. (1978). Single nephron filtration rates (SNGFR) in the trout, Salmo gairdneri. Pflügers Arch. 377, 101–108.
Brown, J. A., Oliver, J. A., Henderson, I. W., and Jackson, B. A. (1980). Angiotensin and single nephron glomerular function in the trout, Salmo gairdneri. Am. J. Physiol. Regul. Integr. Comp. Physiol. 239, R509–R514.
Brown, J. A, Paley, R. K., Amer, S., and Stephen, J. (2000). Evidence for an intrarenal renin-angiotensin system in the rainbow trout, Oncorhynchus mykiss. Am. J. Physiol. Regul. Integr. Comp. Physiol. 278, R1685–R1691.
Cerdà, J., and Finn, R. N. (2010). Piscine aquaporins: an overview of recent advances. J. Exp. Zool. 313A, 1–28.
Chaube, R., Chauvigné, F., Tinguad-Sequeira, A., Joy, K. P., Acharjee, A., Singh, V., and Cerdà, J. (2011). Molecular and functional characterization of catfish (Heteropneustes fossilis) aquaporin-1b: changes in expression during ovarian development and hormone-induced follicular maturation. Gen. Comp. Endocrinol. 170, 162–171.
Cutler, C. P., and Cramb, G. (2002). Branchial expression of an aquaporin 3 (AQP-3) homologue is downregulated in the European eel (Anguilla anguilla) following seawater acclimation. J. Exp. Biol. 205, 2643–2651.
Cutler, C. P., Martinez, A. S., and Cramb, G. (2007). The role of aquaporin 3 in teleost fish. Comp. Biochem. Physiol. 148A, 82–91.
Cutler, C. P., Philips, C., Hazon, N., and Cramb, G. (2009). Aquaporin 8 (AQP8) intestinal mRNA expression increases in response to salinity acclimation in yellow and silver European eels (Anguilla anguilla). Comp. Biochem. Physiol. 153A, :S78.
Dantzler, W. H. (2003). Regulation of renal proximal and distal tubule transport: sodium, chloride and organic anions. Comp. Biochem. Physiol. 136A, 453–478.
Deane, E. E., and Woo, N. Y. S. (2006). Tissue distribution, effects of salinity acclimation and ontogeny of aquaporin 3 in the marine teleost, silver sea bream (Sparus sarba). Mar. Biotechnol. 8, 663–671.
Elger, E., Elger, B., Hentschel, H., and Stolte, H. (1987). Adaptation of renal function to hypotonic medium in the winter flounder (Pseudopleuronectes americanus). J. Comp. Physiol. B 157B, 21–30.
Elkjær, M. L., Nejsum, L. N., Gresz, V., Kwon, T. H., Jensen, U. F., Frøkiær, J., and Nielsen, S. (2001). Immunolocalization of aquaporin-8 in rat kidney, gastrointestinal tract, testis and airways. Am. J. Physiol. Renal Physiol. 281, F1047–F1057.
Fabra, M., Raldúa, D., Bozzo, M. G., Deen, P. M. T., Lubzens, E., and Cerdà, J. (2006). Yolk proteolysis and aquaporin-1o play essential roles to regulate fish oocyte hydration during meiosis resumption. Dev. Biol. 295, 250–262.
Fabra, M., Raldúa, D., Power, D. M., Deen, P. M. T., and Cerdà, J. (2005). Marine fish egg hydration is aquaporin-mediated. Science 307, 545.
Giffard-Mena, I., Boulo, V., Aujoulat, F., Fowden, H., Castille, R., Charmantier, G., and Cramb, G. (2007). Aquaporin molecular characterization in the sea-bass (Dicentrarchus labrax): the effect of salinity on AQP1 and AQP3 expression. Comp. Biochem. Physiol. 148A, 430–444.
Giffard-Mena, I., Lorin-Nebel, C., Charmantier, G., Castille, R., and Boulo, V. (2008). Adaptation of the sea-bass (Dicentrarchus labrax) to fresh water: role of aquaporin and Na+/K+-ATPases. Comp. Biochem. Physiol. 150A, 332–338.
Hatakeyama, S., Yoshida, Y., Tani, T., Koyama, Y., Nihei, K., Ohshiro, K., Kamiie, J., Yaoita, E., Suda, T., Hatakeyama, K., and Yamamoto, T. (2001). Cloning of a new aquaporin (AQP10) abundantly expressed in duodenum and jejunum. Biochem. Biophys. Res. Comm. 287, 814–819.
Hickman, C. P. J., and Trump, B. F. (1969). “The kidney,” in Fish Physiology, Vol. I, eds W. S. Hoar, and D. J. Randall (New York: Academic Press), 91–239.
Ivanis, G., Braun, M., and Perry, S. F. (2008). Renal expression and localization of SLC9A3 sodium/hydrogen exchanger and its possible role in acid-base regulation in freshwater rainbow trout (Oncorhynchus mykiss). Am. J. Physiol. Regul. Integr. Comp. Physiol. 295, R971–R978.
Kato, A., Muro, T., Kimura, Y., Li, S., Islam, Z., Ogoshi, M., Doi, H., and Hirose, S. (2011). Differential expression of Na+-Cl- cotransporter and Na+-K+-Cl- cotransporter 2 in the distal nephrons of euryhaline and seawater pufferfishes. Am. J. Physiol. Regul. Integr. Comp. Physiol. 300, R284–R297.
Katoh, F., Cozzi, R. R. F., Marshall, W. S., and Goss, G. G. (2008). Distinct Na+/K+/2Cl- cotransporter localization in kidneys and gills of two euryhaline species, rainbow trout and killifish. Cell Tissue Res. 334, 265–281.
Kim, Y. K., Watanabe, S., Kaneko, T., Do Huh, M., and Park, S. I. (2010). Expression of aquaporin 3, 8 and 10 in the intestines of freshwater- and seawater-acclimated Japanese eels Anguilla japonica. Fish. Sci. 76, 695–702.
Konno, N., Hyodo, S., Yamaguchi, Y., Matsuda, K., and Uchiyama, M. (2010). Vasotocin/V2-type receptor/aquaporin axis exists in the African lungfish kidney but is functional only in terrestrial condition. Endocrinology 151, 1089–1096.
Liu, K., Nagase, H., Huang, C. G., Calamita, G., and Agre, P. (2006). Purification and functional characterization of aquaporin-8. Biol. Cell 98, 153–161.
MacIver, B., Cutler, C. P., Yin, J., Hill, M. G., Zeidel, M. L., and Hill, W. G. (2009). Expression and functional characterization of four aquaporin water channels from the European eel (Anguilla anguilla). J. Exp. Biol. 212, 2856–2863.
Manzon, L. A. (2002). The role of prolactin in fish osmoregulation: a review. Gen. Comp. Endocrinol. 125, 291–310.
Martinez, A. S., Cutler, C. P., Wilson, G. D., Phillips, C., Hazon, N., and Cramb, G. (2005). Cloning and expression of three aquaporin homologues from the European eel (Anguilla anguilla): effects of seawater acclimation and cortisol treatment on renal expression. Biol. Cell 97, 615–627.
McDonald, M. D. (2007). “The renal contribution to salt and water balance,” in Fish Osmoregulation, eds B. Baldisserotto, J. M. M. Romero, and B. G. Kapoor (Enfield, NH: Science Publishers), 322–345.
Miyazaki, H., Kaneko, T., Uchida, S., Sasaki, S., and Takei, Y. (2002). Kidney-specific chloride channel, OmClC-K, predominantly expressed in the diluting segment of freshwater-adapted tilapia kidney. Proc. Natl. Acad. Sci. U.S.A. 99, 15782–15787.
Mobasheri, A., Shakibaei, M., and Marples, D. (2004). Immunohistochemical localization of aquaporin 10 in the apical membranes of the human ileum: a potential pathway for luminal water and small solute absorption. Histochem. Cell Biol. 121, 463–471.
Nielsen, S., Frøkiær, J., Marples, D., Kwon, T. H., Agre, P., and Knepper, M. A. (2002). Aquaporins in the kidney: from molecules to medicine. Physiol. Rev. 82, 205–244.
Nishimura, H., and Imai, M. (1982). Control of renal function in freshwater and marine teleosts. Teleosts Fed. Proc. 41, 2355–2360.
Noda, Y., Sohara, E., Ohta, E., and Sasaki, S. (2010). Aquaporins in kidney pathophysiology. Nat. Rev. Nephrol. 6, 168–178.
Resende, A. D., Lobo-da-Cunha, A., Malhão, F., Franquinho, F., Monteiro, R. A. F., and Rocha, E. (2010). Histological and stereological characterization of brown trout (Salmo trutta f. fario) trunk kidney. Microsc. Microanal. 16, 677–687.
Santos, C. R., Estêvão, M. D., Fuentes, J., Cardoso, J. C., Fabra, M., Passos, A. L., Detmers, F. J., Deen, P. M., Cerdà, J., and Power, D. M. (2004). Isolation of a novel aquaglyceroporin from a marine teleost (Sparus auratus): function and tissue distribution. J. Exp. Biol. 207, 1217–1227.
Teranishi, K., and Kaneko, T. (2010). Spatial, cellular, and intracellular localization of Na+/K+-ATPase in the sterically disposed renal tubules of Japanese eel. J. Histochem. Cytochem. 58, 707–719.
Tingaud-Sequeira, A., Calusinska, M., Finn, R. N., Chauvigne, F., Lozano, J., and Cerdà, J. (2010). The zebrafish genome encodes the largest vertebrate repertoire of functional aquaporins with dual paralogy and substrate specificities similar to mammals. BMC Evol. Biol. 10, 38. doi: 10.1186/1471-2148-10-38
Tipsmark, C. K., Sørensen, K. J., and Madsen, S. S. (2010). Aquaporin expression dynamics in osmoregulatory tissues of Atlantic salmon during smoltification and seawater acclimation. J. Exp. Biol. 213, 368–379.
Keywords: kidney, aquaporin
Citation: Engelund MB and Madsen SS (2011) The role of aquaporins in the kidney of euryhaline teleosts. Front. Physio. 2:51. doi: 10.3389/fphys.2011.00051
Received: 27 June 2011; Accepted: 05 August 2011;
Published online: 23 August 2011.
Edited by:
Shigehisa Hirose, Tokyo Institute of Technology, JapanReviewed by:
Jonathan M. Wilson, Centre for Marine and Environmental Research, PortugalGordon Cramb, University of St Andrews, UK
Li-Yih Lin, National Taiwan Normal University, Taiwan
Copyright: © 2011 Engelund and Madsen. This is an open-access article subject to a non-exclusive license between the authors and Frontiers Media SA, which permits use, distribution and reproduction in other forums, provided the original authors and source are credited and other Frontiers conditions are complied with.
*Correspondence: Steffen S. Madsen, Institute of Biology, University of Southern Denmark, Campusvej 55, DK-5230 Odense M, Denmark. e-mail: steffen@biology.sdu.dk