- 1 Applied Molecular Hepatology Laboratory, Department of Visceral, Transplantation, Thoracic and Vascular Surgery, University Hospital Leipzig, Leipzig, Germany
- 2 Translational Centre for Regenerative Medicine, Leipzig, Germany
Without therapeutic intervention acute liver failure (ALF) is the consequence of a progredient destruction of the liver parenchyma due to metabolic exhaustion of the hepatocytes. Perivenous hepatocytes are responsible for the detoxification of noxious compounds via the cytochrome P450 enzyme system. Liver transplantation is the only remaining therapeutic option in the end-stage of the disease. Assuming that metabolic capacity could be provided by healthy hepatocytes and thus substitute for the genuine parenchymal cells hepatocyte transplantation since quite some time is considered to be an alternative to whole liver transplantation. While this hypothesis achieved proof-of-concept in animal trials clinical breakthrough is still awaiting success, the reasons of which are ongoing matter of debate. In recent times mesenchymal stem cells (MSC) came into focus as a transplantable cell source to treat ALF. Interestingly, as demonstrated in various rodent animal models their mode of action is rather based on trophic support of hepatocytes remaining in the damaged host parenchyma rather than substitution of tissue loss. Mechanistically, either direct or indirect paracrine effects from the transplanted cells acting pro-proliferative, anti-apoptotic, and anti-inflammatory seem to trigger the regenerative response of the residual healthy hepatocytes in the otherwise lethally injured liver parenchyma. Thus, allogeneic MSC may be the best choice for the treatment of ALF taking advantage of their short-term benefit to sustain the critical phase of the acute insult avoiding long-term immunosuppression.
Molecular Principles of Tissue Toxicity in ALF Induced by Paracetamol
Acute liver failure (ALF) is characterized by an initial devastating hepatic insult followed by gross parenchymal dysfunction, which leads to a multitude of systemic organ failures due to the missing metabolic homeostasis normally provided by the healthy liver. The most common causes of ALF are viral hepatitis, idiosyncratic side reactions, chronic liver diseases, autoimmune hepatitis, and dose-dependent drug-induced ALF. The disease occurs rapidly and in general requires intensive care with the known high risk of mortality. Whole liver transplantation very often is the only therapy option of choice (Ostapowicz and Lee, 2000; Gill and Sterling, 2001; Rahman and Hodgson, 2001; O’Grady, 2005). The incidence of acetaminophen (paracetamol)-induced ALF is rather high in the US and in the UK related both to therapy-associated and suicide-driven overdosage of the drug (Reuben et al., 2010; Lee et al., 2011). In the liver acetaminophen is metabolized by the cytochrome P450 enzyme system located predominantly in the hepatocytes surrounding the distal branches of the liver sinusoids, the so-called perivenous hepatocytes (Jungermann and Kietzmann, 2000; Benhamouche et al., 2006; Burke and Tosh, 2006; Hailfinger et al., 2006; Gebhardt and Hovhannisyan, 2010). There are two principle ways of detoxification: (1) conjugation by sulfation and/or glucuronidation followed by elimination and (2) cytochrome P450-dependent oxidation and formation of N-acetyl-p-benzoquinonimine (NAPQI), which is then conjugated to glutathione and finally eliminated with the bile. Yet, sustained NAPQI formation eventually causes depletion of glutathione, which then in turn leads to formation of protein adducts as well as reactive nitrogen and oxygen species (Figure 1). Very likely mitochondrial dysfunction and increased permeability of the mitochondrial membranes contribute to the formation of reactive nitrogen and oxygen metabolites such as peroxynitrate and hydrogen peroxide besides others, which in turn mediate protein nitration and oxidative stress (Jaeschke et al., 2002; James et al., 2003; Jaeschke and Bajt, 2006; Doi and Ishida, 2009). Obviously, besides the hepatocytes non-parenchymal cells such as Kupffer cells and sinusoidal endothelial cells seem to be involved in the generation of reactive nitrogen and oxygen species thus augmenting protein and lipid peroxidation. Since these reactions are ultimately mediated by the perivenous cytochrome P450 enzyme system, apoptotic cell death followed by centrilobular necrosis is a hallmark of acetaminophen-induced hepatotoxicity (Figure 2). The inflammatory environment produced during ALF is also responsible for the activation of hepatic stellate cells probably mediated by IL1, which respond with an increase in expression of α-smooth muscle actin and matrix metalloproteinases, mainly MMP9. This seems to favor the remodeling of the extracellular matrix, thus augmenting hepatocyte cell death (Yan et al., 2008; Dechene et al., 2010).
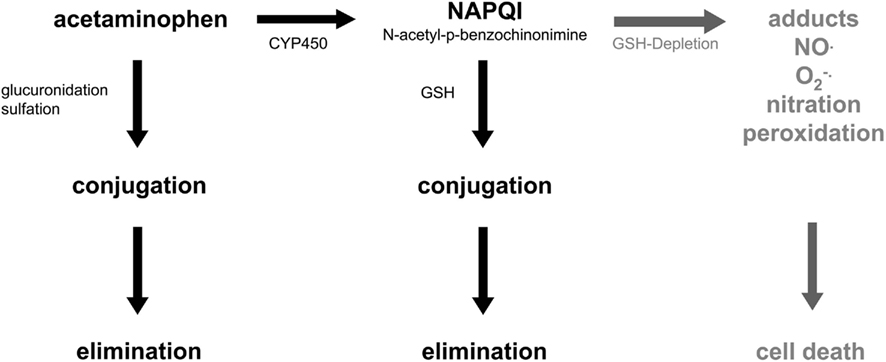
Figure 1. Acetaminophen and hepatotoxicity. Acetaminophen (paracetamol) is detoxified in the liver by conjugation or cytochrome P450-dependent oxidation followed by conjugation to glutathione (GSH). Depletion of GSH leads to formation of reactive nitrogen and oxygen species, which in turn causes cell death. For further details see text.
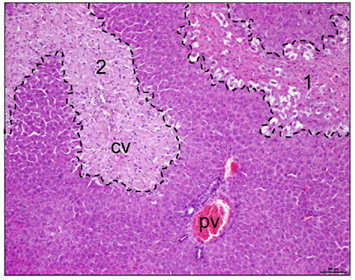
Figure 2. Pericentral necrosis after acetaminophen intoxication. Rats were treated with a repeated oral dose of 4 g/kg body weight of acetaminophen. Eighteen hours later livers were explanted and slices prepared for hemalaun–eosin staining. Dashed lines exemplify initial (area 1) and final necrotic perivenous areas (area 2, cv, central vein) of the liver tissue. Please note that areas around the portal vein (pv) are void of tissue damage.
Regenerative Response to Acute Liver Injury
In the normal healthy liver tissue turnover is in the range of 0.01%. Without any challenge this rather low regenerative rate would reconstitute the whole liver parenchyma within about 1 year (Steiner et al., 1966; Koniaris et al., 2003). One might suspect then that the liver had a poor regenerative potential, which is also corroborated by the fact that after partial hepatectomy the liver is rebuilt to the original organ size, only. After 2/3 partial hepatectomy this would be accomplished by the 1.5-times cell division of the remaining hepatocytes. However, this situation does not reflect the real regenerative potential of hepatocytes. It has been shown in serial transplantation experiments in the albumin promoter-urokinase plasminogen activator (uPA) transgenic mouse that hepatocytes feature a nearly unlimited regenerative capacity. In this model, the intracellular activation of the protease plasmin causes hepatocyte damage and perinatal lethality (Heckel et al., 1990). Eventually, mice survived due to the substitution of hepatocytes bearing the transgene by healthy hepatocytes, which obviously had a survival advantage. Transplantation of these hepatocytes having escaped the lethal phenotype into the livers of transgenic mice revealed the efficient repopulation of the diseased host liver by the donor hepatocytes, thus rescuing the lethal phenotype. This indicates an enormous mitotic potential of hepatocytes (Sandgren et al., 1991; Rhim et al., 1994). In another mouse model, the knockout of fumarylacetoacetate hydrolase (FAH) leads to the accumulation of tyrosine intermediates, which cause toxic insult of hepatocytes. Transplanted healthy hepatocytes display a proliferative advantage over the diseased host hepatocytes, thus achieving nearly complete replacement of the original transgenic hepatocytes by the transplanted cells. In this model, serial transplantation of hepatocytes derived from mutant livers colonized with transplanted wildtype cells revealed that 6 rounds of liver repopulation required a minimum of 69 cell divisions (Overturf et al., 1997, 1999; Wang et al., 2001). Thus, obviously adult hepatocytes have a high replicative and repopulation capacity. This in turn means, that they have the potential of self-renewal and of functional tissue formation in vivo, which are ultimate stem cell characteristics.
Experimentally, ALF might be triggered by the use of chemical noxious compounds such as carbon tetrachloride or acetaminophen as mentioned above. As long as the hepatocytes dispose of sufficient metabolic capacity to detoxify the drugs no obvious tissue lesions emerge. Yet, the production of reactive metabolites followed by covalent protein and lipid modification due to metabolic overload as mentioned above finally results in cellular dysfunction, initial cell damage and tissue injury. Depending on the dose applied tissue damage proceeds. The initial insult resulting in injury progression is the mitotic challenge for the hepatocytes to restore the tissue loss by functional hepatocyte progeny. Again, dependent on the dose of the noxious compounds the regenerative potential of the liver is either sufficient for injury regression or overwhelmed resulting in injury progression followed by ALF (Mehendale, 2005; Palmes et al., 2005; Figure 3). Tissue regeneration is accomplished by the hepatocytes themselves as long as a minimal liver tissue mass is compliant with a certain threshold of functional tissue loss. Yet, if this threshold is surpassed the regenerative capacity of the hepatocytes does not suffice for functional tissue restoration. In this case a progenitor cell compartment is activated giving rise to so-called oval cells in rodents, which are agreed upon to be the progeny of adult hepatic stem cells in the liver (Sell, 2001; Kofman et al., 2005). Oval cells appear in the periportal areas after massive liver injury adjacent to the canals of Hering, structural links between the terminal biliary branches and the periportal hepatocytes (Fausto, 2004; Santoni-Rugiu et al., 2005; Oertel and Shafritz, 2008). It is noteworthy that both hepatocytes and hepatic progenitor cells may differentiate into hepatocytes and biliary cells as well indicating their bipotent differentiation capacity. Hence, both cell types meet the minimal definition criteria of a stem cell, i.e., the potential of self-renewal to maintain the stem cell reserve, and a multiple differentiation potential giving rise to progeny of at least two different lineages. In the latter case it is self-evident that proliferation and differentiation of the offspring cells provide the functional backup for tissue repair after injury.
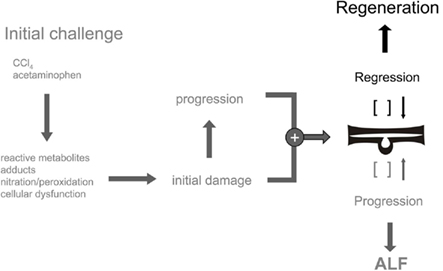
Figure 3. Balance of tissue homeostasis after acute liver injury. The regenerative response of the liver after acute intoxication is triggered by the emergence of initial tissue damage and progression. Dependding on the dose of the noxa and the regenerative capacity of the hepatocytes injury regression and regeneration or progression and ALF develop.
Hepatocyte Transplantation in ALF
In ALF, liver transplantation is the gold standard of treatment. However, about one third of patients on the waiting list for liver transplantation in Europe do not profit because of the unavailability of suitable donor organs1. The hepatocyte is the smallest functional unit of the liver executing the complete metabolic orchestra, which is provided by the liver as a whole. Therefore, transplantation of hepatocytes might be possible to substitute for the functional tissue loss in ALF provided the donor cells take over hepatocyte functions in the deteriorated host parenchyma for at least the critical period in time either required to bridge to organ transplantation or to allow for tissue recovery from the toxic insult (Najimi and Sokal, 2008; Oertel and Shafritz, 2008; Smets et al., 2008; Ito et al., 2009; Puppi and Dhawan, 2009). Technically, in rodent small animal models the cells are delivered to the liver either after intraportal or intrasplenic injection. It is assumed that the cells distribute homogeneously in the liver by passage with the blood stream where they are entrapped in the sinusoids and eventually penetrate the endothelia, integrate, proliferate, and spread into the host parenchyma. This concept has been verified in various rodent animal models of ALF (for recent reviews, see Fox and Roy-Chowdhury, 2004; Shafritz et al., 2006; Seppen et al., 2009; Weber et al., 2009). There is one major constraint, which probably seriously hampers the clinical translation of hepatocyte transplantation in ALF. Under non-stimulating conditions the repopulation of an acutely injured liver by transplanted hepatocytes is rather low, i.e., in the range of 1–5% of the total liver mass (Ponder et al., 1991; Rajvanshi et al., 1996; Gupta et al., 1999; Fox and Roy-Chowdhury, 2004; Fisher and Strom, 2006). However, if the recipient liver is challenged by a growth stimulus and the proliferation of host hepatocytes is impaired then a significant repopulation by transplanted hepatocytes is achieved. There is an elegant animal model available allowing for the identification of the transplanted cells in the host parenchyma. In this rat model the natural mutation in the CD26 gene leads to the expression of a non-functional protein, however, without obvious pathophysiological consequences. Transplanted wildtype donor cells may then be identified histologically in the host parenchyma by the detection of CD26. Providing selective pressure conditions by partial hepatectomy as a mitotic stimulus and pre-treatment with alkaloids such as retrorsine to inhibit host hepatocyte proliferation a repopulation rate for up to nearly 100% may be achieved in this rat model (Laconi et al., 1998, 1999). Similarly, high rates were obtained using rat fetal liver epithelial cells but without applying selective growth conditions for the transplanted cells (Sandhu et al., 2001; Oertel et al., 2006).
Acute liver failure in mice and rats may be induced under various experimental settings, the most common in use are those acutely applying paracetamol or carbon tetrachloride. In general, when adult hepatocytes or oval cells isolated from donor livers under various inducing conditions are used for transplantation without further selective pressure repopulation of the host liver by the transplanted cells is poor, i.e., in the range of less than 5%. However, cells are functional and survive long-term in the recipient liver indicating support of liver regeneration after acute hepatotoxic injury. If in addition to the acute injury regeneration by host hepatocytes is abrogated by the beforehand treatment with mitotoxins such as the pyrrolizidine alkaloid retrorsine much higher repopulation rates may be achieved, which clearly suffice to substitute for the loss of metabolic capacity due to the toxic parenchymal damage. Similar results were obtained using fetal (ED12.5) rat hepatoblasts. Yet, using ED14 mouse hepatoblasts 10- to 20-fold higher repopulation rates were achieved without applying selective repopulation conditions. A comprehensive summary of models and conditions used to study liver repopulation by transplanted hepatocytes or hepatocyte progenitor cells under normal and injury conditions is available (Sancho-Bru et al., 2009; Shafritz and Oertel, 2011). To summarize, transplanted cells integrate into the host parenchyma and even at low repopulation rates display hepatocyte functions. Thus, hepatocyte transplantation in ALF aims at tissue substitution of the recipient liver in order to functionally reconstitute the injured parenchyma by healthy donor cells.
Stem Cell-Derived Hepatocyte Transplantation in ALF
In respect to the clinical application of hepatocyte transplantation in ALF the major hurdle is probably the scarcity of donor organs to isolate human hepatocytes in sufficient quality and quantity. Therefore, one feasible alternative to human adult hepatocytes is the use of stem cell-derived hepatocytes. The bone marrow harbors adult stem cells, both hematopoietic and non-hematopoietic, which are clearly superior in choice over embryonic stem cells for clinical application because of their less ethical constraints and the lack of teratoma formation after tissue implantation. Adult stem cells may differentiate into hepatocyte-like cells. In the mouse model of FAH deficiency, hematopoietic bone marrow derived cells rescued the diseased phenotype by complementation of the defective FAH gene with the wildtype gene in the transplanted cells (Lagasse et al., 2000; Wang et al., 2002). It is an open question as to whether hepatocytes derived from the bone marrow are the product of differentiation from hematopoietic stem cells or of the fusion with host hepatocytes (Alvarez-Dolado et al., 2003; Newsome et al., 2003; Vassilopoulos et al., 2003; Wang et al., 2003; Camargo et al., 2004; Jang et al., 2004). In recent years studies in rats (Wang et al., 2004; Lange et al., 2005), mice (Jiang et al., 2002), and humans (Schwartz et al., 2002; Lee et al., 2004; Hong et al., 2005; Seo et al., 2005; Taléns-Visconti et al., 2006; Aurich et al., 2007; Banas et al., 2007) verified that mesenchymal stem cells (MSC) from various tissues like bone marrow, umbilical cord blood, or adipose tissue may differentiate into hepatocyte-like cells following specified growth and differentiation regimens in vitro. Yet, under acute injury conditions causing either periportal liver damage induced by allyl alcohol (Sato et al., 2005) or perivenous damage by the use of carbon tetrachloride (Seo et al., 2005; Banas et al., 2007; Yukawa et al., 2009) or acetaminophen (Stock et al., 2009), MSC-derived hepatocyte-like cells integrated into the diseased host liver, though repopulation rates were rather low, i.e., in the range of 1% of the total liver mass.
Reasoning that MSC feature immunomodulatory functions in that they are able to suppress the immune response mediated through T and B cells, dendritic cells and other immune cells (Chamberlain et al., 2007; Götherström, 2007; Krampera et al., 2007; Le Blanc and Ringden, 2007) it might not be surprising that the action of MSC in ALF is rather paracrine than direct tissue support by the transplanted cells. D-galactosamine-induced fulminant hepatic failure in rats was attenuated by MSC-derived molecules through inhibition of apoptosis, stimulation of hepatocyte proliferation, and minimization of the inflammatory response (Parekkadan et al., 2007a; van Poll et al., 2008). The paracrine mode of action of MSC was also corroborated by the amelioration of systemic inflammation induced by LPS or burn indicating in addition pleiotropic effects of the MSC (Yagi et al., 2010). Ectopic recruitment of MSC from the bone marrow to the liver has been shown in mice challenged by acute intoxication with carbon tetrachloride or 2-acetylaminofluorene indicating chemotactic activation of the MSC very likely mediated by stromal cell-derived factor-1 (Jin et al., 2009; Chen et al., 2010). Our own data substantiated that MSC are able to home to and integrate into an acutely injured liver. We treated rats with acetaminophen to induce acute liver damage. MSC derived from rat peritoneal adipose tissue were pre-differentiated into hepatocyte-like cells according to our standard protocol (Stock et al., 2010) and then the cells were administered to the diseased animals via tail vein injection. Eighteen hours after cell delivery donor-derived cells were detected in the liver (unpublished) where they significantly decreased acetaminophen-induced apoptosis as shown immunohistochemically by the TUNEL assay and stimulated proliferation of host hepatocytes as shown by Ki67 staining (Figure 4) to regenerate the liver tissue after acute injury (unpublished). Besides their anti-inflammatory and immunomodulatory impact MSC seem also to communicate with target cells by the exchange of mRNA or miRNA molecules (Collino et al., 2010; Deregibus et al., 2010). Thus, genetic material is exchanged, which then might affect the regenerative response of the host tissue cells on the one and the differentiation of donor MSC at the site of their engraftment into the host tissue on the other hand. This, however, means that transplanted cells might be imprinted by their target tissue and the molecular microenvironment induced by a specific type of injury. Acute liver injury may trigger paracrine effects due to the inflammatory environment of the diseased liver, whereas liver regeneration after, e.g., partial hepatectomy is achieved by the engraftment and functional tissue replacement by the MSC differentiated into hepatocytes at the site of their engraftment. This potential pleiotropic mode of action makes MSC ideal candidates for stem cell therapy of different liver diseases (Enns and Millan, 2008; Haridass et al., 2008; Alison et al., 2009; Flohr et al., 2009; Soto-Gutierrez et al., 2009).
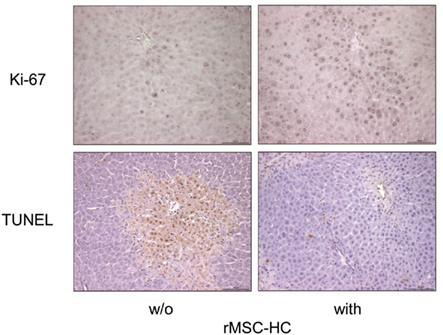
Figure 4. Anti-apoptotic and pro-proliferative action of MSC after acetaminophen intoxication of the rat liver. Rats were treated with a repeated dose of 4 g/kg body weight of acetaminophen. Eighteen hours after the last dose the animals were sacrificed and the livers prepared for the detection of apoptotic cells (dark nuclei) by the TUNEL assay (lower panels) or proliferating cells (dark nuclei) by the Ki67 stain (upper panels). Where indicated animals received adipose tissue-derived rat MSC pre-differentiated into hepatocyte-like cells (rMSC-HC) 6 h after the last dose of acetaminophen. It is obvious that the number of apoptotic cells was significantly lower but of proliferating cells was higher in the livers with MSC (right panels) indicating the anti-apoptotic and pro-proliferative action of the MSC.
Clinical Implications
It is obvious that experimental settings in animal models aimed to enhance liver repopulation by transplanted hepatocytes are not suited for clinical translation. Thus, the lack of a survival and/or a proliferative advantage of donor vs. host hepatocytes is probably the mechanistical reason for the poor clinical progress of hepatocyte transplantation. The low success rate is augmented by the fact that human hepatocytes are isolated from marginal donor livers not allocated for transplantation. Yet, as outlined above MSC might be an alternate cell resource to generate hepatocyte-like cells. MSC display hepatocyte differentiation potential, which was substantiated both in vitro and in vivo. Even if biological and biochemical differences might exist between MSC from various tissues they share typical MSC characteristics like marker expression, multiple differentiation capacity, and growth on plastic surfaces, which finally determine quantitative, not qualitative, variability in their hepatocyte differentiation potential. It is feasible to suppose that in respect to ethical, technical and biological aspects the transplantation of stem cell-derived hepatocytes follows the principles of hepatocyte transplantation (Fisher and Strom, 2006). MSC might even open a broader spectrum of activity compared with primary hepatocytes because of their versatile properties such as low immunogenicity as well as their anti-inflammatory, anti-apoptotic, and pro-proliferative activities, which not only substitute the tissue damaged but also actively might temper the inflammatory response, e.g., after toxic or chronic injury. Recently, a couple of clinical trials – most in China – has been initiated or even completed to demonstrate safety and efficacy of the site of application of MSCs concentrating on autologous stem cell transplantation in patients suffering from chronic liver failure (Table 1) or acute decompensation after ample liver resection2. Yet, so far no published results are available. In these studies, undifferentiated cells have been used bearing a potential tumor-promoting risk (Karnoub et al., 2007), which, however, has not been verified.
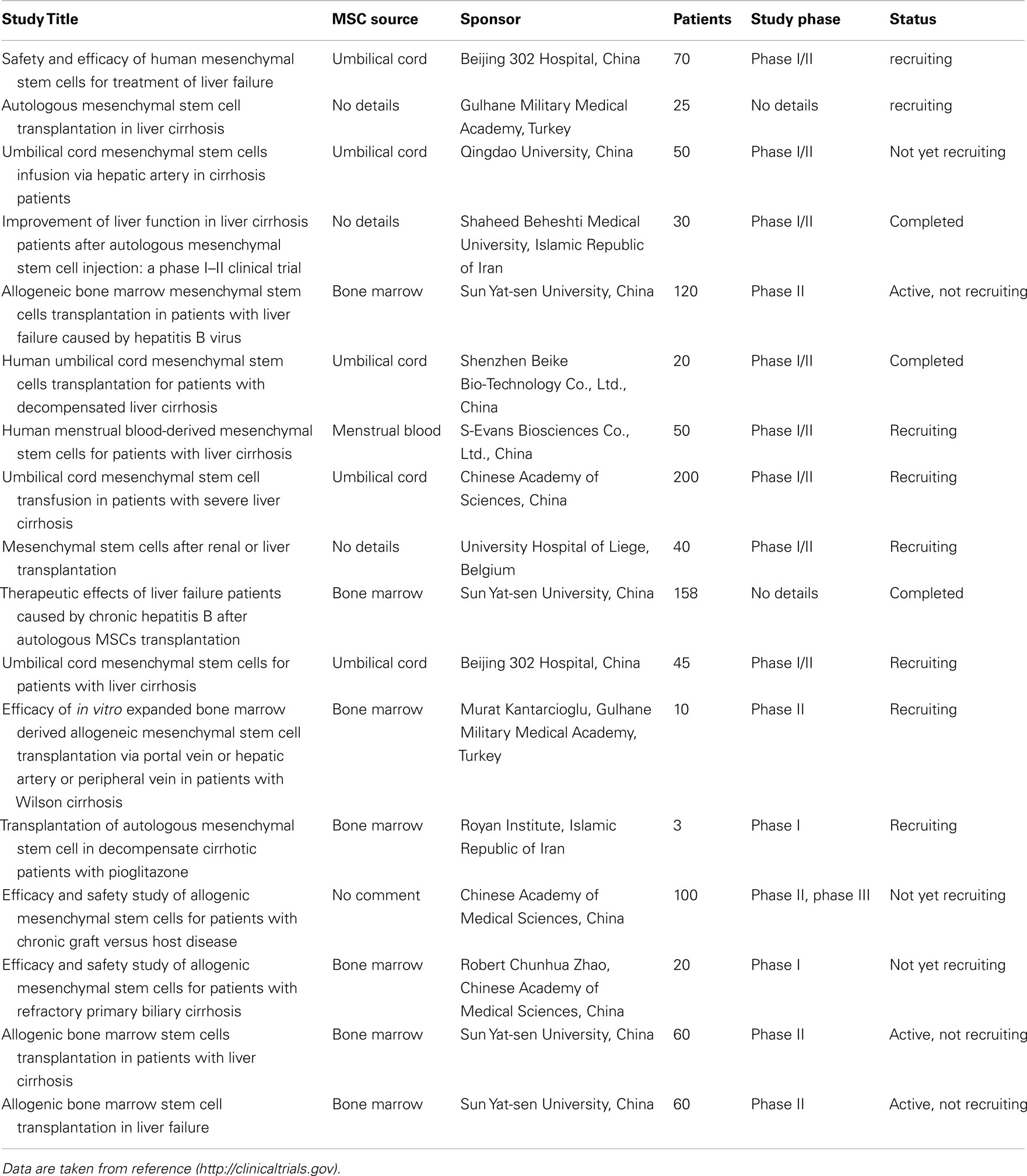
Table 1. Summary of clinical trials involving mesenchymal stem cells of different tissue sources for the treatment of chronic liver diseases.
Taking ethical considerations into account these clinical conditions may be adequate to assess safety of hepatic MSC transplantation. However, to take advantage of the cells’ immunomodulatory, chemotactic, and anti-inflammatory properties, ALF offering a highly inflammatory environment in the liver may be the disease situation of choice for the use of MSC. In this case even the use of allogeneic cell sources may not be a serious problem since only the short-term beneficial actions of the MSC might warrant support of liver regeneration in the critical phase of acute poisoning. Immunosuppression may be applied from the beginning of treatment on or even continued as long as the recovering of the liver is ongoing but then may be ceased, thus avoiding the theoretical risks of potential sensitization of the host for future organ grafts or promoting life-threatening septic episodes during long-term stay in the intensive care units.
Conclusion
To overcome the shortage of donor organs for liver transplantation in ALF cell therapy approaches seem to be feasible, which must achieve two principle goals. (1) The loss of metabolic capacity must be substituted by the healthy donor cells, and (2) the emergence of the inflammatory environment in ALF must be decelerated in order to protect hepatocytes from progression into cell death. It is obvious that the first goal might best be reached using primary hepatocytes, which, however, do not have a survival advantage in the deteriorated ALF liver. The second goal might best be met by the use of MSC taking advantage of their anti-inflammatory and – apoptotic as well as pro-proliferative features, which, however, promises no therapeutic benefit in the case that tissue damage has surpassed the lower threshold needed to maintain body metabolic homeostasis. Thus, it might be worthwhile thinking whether a combination of hepatocytes and MSC might be the cell therapeutic of best choice. Indeed, there is evidence that the performance of hepatocytes is improved in co-culture with MSC (Ijima et al., 2008; Shi et al., 2009; Chen et al., 2012), and vice versa MSC differentiation into hepatocyte-like cells is promoted by inflammatory liver injury conditions (Dong et al., 2010; Li et al., 2010). Recent data even demonstrated that not MSC themselves but as yet unequivocally unidentified soluble factors secreted by MSC exert the beneficial effects on hepatocytes under ALF conditions in mice and rats (Parekkadan et al., 2007b; van Poll et al., 2008; Zagoura et al., 2011). The anti-inflammatory cytokine IL10 secreted by MSC seemed to play a major role in alleviating liver damage after acute injury induced by carbon tetrachloride in the NOD/SCID mouse model (Zagoura et al., 2011). Thus, the identification of these factors might open even cell-free therapeutical options for the treatment of ALF with MSC-derived molecules.
Animal models for cell therapy approaches to treat ALF as described above enable us to earn knowledge on the mechanisms of interactions between donor and host cells both on the molecular and cellular level, to identify the hepatotropic effects esp. mediated by MSC and their impact on the noxious challenge in order to optimize integration of transplanted cells into the recipient tissue thereby to support efficacy of cell transplantation and thus optimize the therapeutical outcome.
Conflict of Interest Statement
The authors declare that the research was conducted in the absence of any commercial or financial relationships that could be construed as a potential conflict of interest.
Acknowledgments
Original work presented in this paper (Figures 2 and 4) was made possible by funding from the German Research Foundation (Ch 109/15-2) as well as by the German Federal Ministry of Education and Research (BMBF, PtJ-Bio, 0315883). The careful assistance of Madlen Hempel is greatly acknowledged.
Footnotes
References
Alison, M. R., Islam, S., and Lim, S. M. (2009). Cell therapy for liver disease. Curr. Opin. Mol. Ther. 11, 364–374.
Alvarez-Dolado, M., Pardal, R., Garcia-Verdugo, J. M., Fike, J. R., Lee, H. O., Pfeffer, K., Lois, C., Morrison, S. J., and Alvarez-Buylla, A. (2003). Fusion of bone-marrow-derived cells with Purkinje neurons, cardiomyocytes and hepatocytes. Nature 425, 968–973.
Aurich, I., Mueller, L. P., Aurich, H., Luetzkendorf, J., Tisljar, K., Dollinger, M. M., Schormann, W., Walldorf, J., Hengstler, J. G., Fleig, W. E., and Christ, B. (2007). Functional integration of hepatocytes derived from human mesenchymal stem cells into mouse livers. Gut 56, 405–415.
Banas, A., Teratani, T., Yamamoto, Y., Tokuhara, M., Takeshita, F., Quinn, G., Okochi, H., and Ochiya, T. (2007). Adipose tissue-derived mesenchymal stem cells as a source of human hepatocytes. Hepatology 46, 219–228.
Benhamouche, S., Decaens, T., Godard, C., Chambrey, R., Rickman, D. S., Moinard, C., Vasseur-Cognet, M., Kuo, C. J., Kahn, A., Perret, C., and Colnot, S. (2006). Apc tumor suppressor gene is the zonation-keeper of mouse liver. Dev. Cell 10, 759–770.
Burke, Z. D., and Tosh, D. (2006). The Wnt/beta-catenin pathway: master regulator of liver zonation? Bioessays 28, 1072–1077.
Camargo, F. D., Finegold, M., and Goodell, M. A. (2004). Hematopoietic myelomonocytic cells are the major source of hepatocyte fusion partners. J. Clin. Invest. 113, 1266–1270.
Chamberlain, G., Fox, J., Ashton, B., and Middleton, J. (2007). Concise review: mesenchymal stem cells: their phenotype, differentiation capacity, immunological features, and potential for homing. Stem Cells 25, 2739–2749.
Chen, X., Zhang, S., Liu, T., Liu, Y., and Wang, Y. (2012). Maintenance of rat hepatocytes under inflammation by coculture with human orbital fat-derived stem cells. Cell. Mol. Biol. Lett. 1–14. doi: 10.2478/s11658-012-0004-9. [Epub ahead of print].
Chen, Y., Xiang, L.-X., Shao, J.-Z., Pan, R.-L., Wang, Y.-X., Dong, X.-J., and Zhang, G.-R. (2010). Recruitment of endogenous bone marrow mesenchymal stem cells towards injured liver. J. Cell. Mol. Med. 14, 1494–1508.
Collino, F., Deregibus, M. C., Bruno, S., Sterpone, L., Aghemo, G., Viltono, L., Tetta, C., and Camussi, G. (2010). Microvesicles derived from adult human bone marrow and tissue specific mesenchymal stem cells shuttle selected pattern of miRNAs. PLoS ONE 5, e11803. doi:10.1371/journal.pone.0011803
Dechene, A., Sowa, J.-P., Gieseler, R. K., Jochum, C., Bechmann, L. P., El Fouly, A., Schlattjan, M., Saner, F., Baba, H. A., Paul, A., Dries, V., Odenthal, M., Gerken, G., Friedman, S. L., and Canbay, A. (2010). Acute liver failure is associated with elevated liver stiffness and hepatic stellate cell activation. Hepatology 52, 1008–1016.
Deregibus, M. C., Tetta, C., and Camussi, G. (2010). The dynamic stem cell microenvironment is orchestrated by microvesicle-mediated transfer of genetic information. Histol. Histopathol. 25, 397–404.
Doi, K., and Ishida, K. (2009). Diabetes and hypertriglyceridemia modify the mode of acetaminophen-induced hepatotoxicity and nephrotoxicity in rats and mice. J. Toxicol. Sci. 34, 1–11.
Dong, X. J., Zhang, H., Pan, R. L., Xiang, L. X., and Shao, J. Z. (2010). Identification of cytokines involved in hepatic differentiation of mBM-MSCs under liver-injury conditions. World J. Gastroenterol. 16, 3267–3278.
Enns, G. M., and Millan, M. T. (2008). Cell-based therapies for metabolic liver disease. Mol. Genet. Metab. 95, 3–10.
Fausto, N. (2004). Liver regeneration and repair: hepatocytes, progenitor cells, and stem cells. Hepatology 39, 1477–1487.
Fisher, R. A., and Strom, S. C. (2006). Human hepatocyte transplantation. Transplantation 82, 441–449.
Flohr, T. R., Bonatti, H. J., Brayman, K. L., and Pruett, T. L. (2009). The use of stem cells in liver disease. Curr. Opin. Organ Transplant. 14, 64–71.
Gebhardt, R., and Hovhannisyan, A. (2010). Organ patterning in the adult stage: the role of Wnt/ß-catenin signaling in liver zonation and beyond. Dev. Dyn. 239, 45–55.
Götherström, C. (2007). Immunomodulation by multipotent mesenchymal stromal cells. Transplantation 84, S35–S37.
Gupta, S., Rajvanshi, P., Aragona, E., Lee, C.-D., Yerneni, P. R., and Burk, R. D. (1999). Transplanted hepatocytes proliferate differently after CCl4 treatment and hepatocyte growth factor infusion. Am. J. Physiol. 276, G629–G638.
Hailfinger, S., Jaworski, M., Braeuning, A., Buchmann, A., and Schwarz, M. (2006). Zonal gene expression in murine liver: lessons from tumors. Hepatology 43, 407–414.
Haridass, D., Narain, N., and Ott, M. (2008). Hepatocyte transplantation: waiting for stem cells. Curr. Opin. Organ Transplant. 13, 627–632.
Heckel, J. L., Sandgren, E. P., Degen, J. L., Palmiter, R. D., and Brinster, R. L. (1990). Neonatal bleeding in transgenic mice expressing urokinase-type plasminogen activator. Cell 62, 447–456.
Hong, S. H., Gang, E. J., Jeong, J. A., Ahn, C., Hwang, S. H., Yang, I. H., Park, H. K., Han, H., and Kim, H. (2005). In vitro differentiation of human umbilical cord blood-derived mesenchymal stem cells into hepatocyte-like cells. Biochem. Biophys. Res. Commun. 330, 1153–1161.
Ijima, H., Matsuo, T., and Kawakami, K. (2008). The mixed coculture effect of primary rat hepatocytes and bone marrow cells is caused by soluble factors derived from bone marrow cells. J. Biosci. Bioeng. 105, 226–231.
Ito, M., Nagata, H., Miyakawa, S., and Fox, I. J. (2009). Review of hepatocyte transplantation. J. Hepatobiliary Pancreat. Surg. 16, 97–100.
Jaeschke, H., and Bajt, M. L. (2006). Intracellular signaling mechanisms of acetaminophen-induced liver cell death. Toxicol. Sci. 89, 31–41.
Jaeschke, H., Gores, G. J., Cederbaum, A. I., Hinson, J. A., Pessayre, D., and Lemasters, J. J. (2002). Mechanisms of hepatotoxicity. Toxicol. Sci. 65, 166–176.
James, L. P., Mayeux, P. R., and Hinson, J. A. (2003). Acetaminophen-induced hepatotoxicity. Drug Metab. Dispos. 31, 1499–1506.
Jang, Y.-Y., Collector, M. I., Baylin, S. B., Diehl, A. M., and Sharkis, S. J. (2004). Hematopoietic stem cells convert into liver cells within days without fusion. 6, 532–539.
Jiang, Y., Jahagirdar, B. N., Reinhardt, R. L., Schwartz, R. E., Keene, C. D., Ortiz-Gonzalez, X. R., Reyes, M., Lenvik, T., Lund, T., Blackstad, M. D. J., Aldrich, S., Lisberg, A., Low, W. C., Largaespada, D. A., and Verfaillie, C. M. (2002). Pluripotency of mesenchymal stem cells derived from adult marrow. Nature 418, 41–49.
Jin, S. Z., Meng, X. W., Han, M. Z., Sun, X., Sun, L. Y., and Liu, B. R. (2009). Stromal cell derived factor-1 enhances bone marrow mononuclear cell migration in mice with acute liver failure. World J. Gastroenterol. 15, 2657–2664.
Jungermann, K., and Kietzmann, T. (2000). Oxygen: modulator of metabolic zonation and disease of the liver. Hepatology 31, 255–260.
Karnoub, A. E., Dash, A. B., Vo, A. P., Sullivan, A., Brooks, M. W., Bell, G. W., Richardson, A. L., Polyak, K., Tubo, R., and Weinberg, R. A. (2007). Mesenchymal stem cells within tumour stroma promote breast cancer metastasis. Nature 449, 557–563.
Kofman, A. V., Morgan, G., Kirschenbaum, A., Osbeck, J., Hussain, M., Swenson, S., and Theise, N. D. (2005). Dose- and time-dependent oval cell reaction in acetaminophen-induced murine liver injury. Hepatology 41, 1252–1261.
Koniaris, L. G., McKillop, I. H., Schwartz, S. I., and Zimmers, T. A. (2003). Liver regeneration. J. Am. Coll. Surg. 197, 634–659.
Krampera, M., Sartoris, S., Liotta, F., Pasini, A., Angeli, R., Cosmi, L., Andreini, A., Mosna, F., Bonetti, B., Rebellato, E., Testi, M. G., Frosali, F., Pizzolo, G., Tridente, G., Maggi, E., Romagnani, S., and Annunziato, F. (2007). Immune regulation by mesenchymal stem cells derived from adult spleen and thymus. Stem Cells Dev. 16, 797–810.
Laconi, E., Oren, R., Mukhopadhyay, D. K., Hurston, E., Laconi, S., Pani, P., Dabeva, M. D., and Shafritz, D. A. (1998). Long-term, near-total liver replacement by transplantation of isolated hepatocytes in rats treated with retrorsine. Am. J. Pathol. 153, 319–329.
Laconi, S., Curreli, F., Diana, S., Pasciu, D., Filippo, G. D., Sarma, D. S. R., Pani, P., and Laconi, E. (1999). Liver regeneration in response to partial hepatectomy in rats treated with retrorsine: a kinetic study. J. Hepatol. 31, 1069–1074.
Lagasse, E., Connors, H., Al-Dhalimy, M., Reitsma, M., Dohse, M., Osborne, L., Wang, X., Finegold, M., Weissman, I. L., and Grompe, M. (2000). Purified hematopoietic stem cells can differentiate into hepatocytes in vivo. Nat. Med. 6, 1229–1234.
Lange, C., Bassler, P., Lioznov, M. V., Bruns, H., Kluth, D., Zander, A. R., and Fiegel, H. C. (2005). Hepatocytic gene expression in cultured rat mesenchymal stem cells. Transplant. Proc. 37, 276–279.
Le Blanc, K., and Ringden, O. (2007). Immunomodulation by mesenchymal stem cells and clinical experience. J. Intern. Med. 262, 509–525.
Lee, K. D., Kuo, T. K., Whang-Peng, J., Chung, Y. F., Lin, C. T., Chou, S. H., Chen, J. R., Chen, Y. P., and Lee, O. K. (2004). In vitro hepatic differentiation of human mesenchymal stem cells. Hepatology 40, 1275–1284.
Lee, W. M., Stravitz, R. T., and Larson, A. (2011). Introduction to the revised AASLD position paper on acute liver failure 2011. Hepatology 55, 965–967.
Li, J., Tao, R., Wu, W., Cao, H., Xin, J., Guo, J., Jiang, L., Hong, X., Demetriou, A. A., Farkas, D., and Li, L. (2010). Transcriptional profiling and hepatogenic potential of acute hepatic failure-derived bone marrow mesenchymal stem cells. Differentiation 80, 166–174.
Mehendale, H. M. (2005). Tissue repair: an important determinant of final outcome of toxicant-induced injury. Toxicol. Pathol. 33, 41–51.
Najimi, M., and Sokal, E. M. (2008). Cell transplantation in the treatment of liver diseases. 12, 6–13.
Newsome, P. N., Johannessen, I., Boyle, S., Dalakas, E., McAulay, K. A., Samuel, K., Rae, F., Forrester, L., Turner, M. L., Hayes, P. C., Harrison, D. J., Bickmore, W. A., and Plevris, J. N. (2003). Human cord blood-derived cells can differentiate into hepatocytes in the mouse liver with no evidence of cellular fusion. Gastroenterology 124, 1891–1900.
Oertel, M., Menthena, A., Dabeva, M. D., and Shafritz, D. A. (2006). Cell competition leads to a high level of normal liver reconstitution by transplanted fetal liver stem/progenitor cells. Gastroenterology 130, 507–520.
Oertel, M., and Shafritz, D. A. (2008). Stem cells, cell transplantation and liver repopulation. Biochim. Biophys. Acta 1782, 61–74.
Ostapowicz, G., and Lee, W. M. (2000). Acute hepatic failure: a Western perspective. J. Gastroenterol. Hepatol. 15, 480–488.
Overturf, K., Al-Dhalimy, M., Finegold, M., and Grompe, M. (1999). The repopulation potential of hepatocyte populations differing in size and prior mitotic expansion. Am. J. Pathol. 155, 2135–2143.
Overturf, K., al-Dhalimy, M., Ou, C. N., Finegold, M., and Grompe, M. (1997). Serial transplantation reveals the stem-cell-like regenerative potential of adult mouse hepatocytes. Am. J. Pathol. 151, 1273–1280.
Palmes, D., Skawran, S., and Spiegel, H.-U. (2005). Acute liver failure: from bench to bedside. Transplant. Proc. 37, 1628–1631.
Parekkadan, B., van Poll, D., Megeed, Z., Kobayashi, N., Tilles, A. W., Berthiaume, F., and Yarmush, M. L. (2007a). Immunomodulation of activated hepatic stellate cells by mesenchymal stem cells. Biochem. Biophys. Res. Commun. 363, 247–252.
Parekkadan, B., van Poll, D., Suganuma, K., Carter, E. A., Berthiaume, F., Tilles, A. W., and Yarmush, M. L. (2007b). Mesenchymal stem cell-derived molecules reverse fulminant hepatic failure. PLoS ONE 2, e941. doi:10.1371/journal.pone.0000941
Ponder, K. P., Gupta, S., Leland, F., Darlington, G., Finegold, M., DeMayo, J., Ledley, F. D., Chowdhury, J. R., and Woo, S. L. (1991). Mouse hepatocytes migrate to liver parenchyma and function indefinitely after intrasplenic transplantation. Proc. Natl. Acad. Sci. U.S.A. 88, 1217–1221.
Puppi, J., and Dhawan, A. (2009). Human hepatocyte transplantation overview. Methods Mol. Biol. 481, 1–16.
Rahman, T., and Hodgson, H. (2001). Clinical management of acute hepatic failure. Intensive Care Med. 27, 467–476.
Rajvanshi, P., Kerr, A., Bhargava, K., Burk, R., and Gupta, S. (1996). Efficacy and safety of repeated hepatocyte transplantation for significant liver repopulation in rodents. Gastroenterology 111, 1092–1102.
Reuben, A., Koch, D. G., and Lee, W. M. (2010). Drug-induced acute liver failure: results of a U.S. multicenter, prospective study. Hepatology 52, 2065–2076.
Rhim, J. A., Sandgren, E. P., Degen, J. L., Palmiter, R. D., and Brinster, R. L. (1994). Replacement of diseased mouse liver by hepatic cell transplantation. Science 263, 1149–1152.
Sancho-Bru, P., Najimi, M., Caruso, M., Pauwelyn, K., Cantz, T., Forbes, S., Roskams, T., Ott, M., Gehling, U., Sokal, E., Verfaillie, C. M., and Muraca, M. (2009). Stem and progenitor cells for liver repopulation: can we standardise the process from bench to bedside? Gut 58, 594–603.
Sandgren, E. P., Palmiter, R. D., Heckel, J. L., Daugherty, C. C., Brinster, R. L., and Degen, J. L. (1991). Complete hepatic regeneration after somatic deletion of an albumin-plasminogen activator transgene. Cell 66, 245–256.
Sandhu, J. S., Petkov, P. M., Dabeva, M. D., and Shafritz, D. A. (2001). Stem cell properties and repopulation of the rat liver by fetal liver epithelial progenitor cells. Am. J. Pathol. 159, 1323–1334.
Santoni-Rugiu, E., Jelnes, P., Thorgeirsson, S. S., and Bisgaard, H. C. (2005). Progenitor cells in liver regeneration: molecular responses controlling their activation and expansion. APMIS 113, 876–902.
Sato, Y., Araki, H., Kato, J., Nakamura, K., Kawano, Y., Kobune, M., Sato, T., Miyanishi, K., Takayama, T., Takahashi, M., Takimoto, R., Iyama, S., Matsunaga, T., Ohtani, S., Matsuura, A., Hamada, H., and Niitsu, Y. (2005). Human mesenchymal stem cells xenografted directly to rat liver are differentiated into human hepatocytes without fusion. Blood 106, 756–763.
Schwartz, R. E., Reyes, M., Koodie, L., Jiang, Y., Blackstad, M., Lund, T., Lenvik, T., Johnson, S., Hu, W. S., and Verfaillie, C. M. (2002). Multipotent adult progenitor cells from bone marrow differentiate into functional hepatocyte-like cells. J. Clin. Invest. 109, 1291–1302.
Seo, M. J., Suh, S. Y., Bae, Y. C., and Jung, J. S. (2005). Differentiation of human adipose stromal cells into hepatic lineage in vitro and in vivo. Biochem. Biophys. Res. Commun. 328, 258–264.
Seppen, J., Filali, E. E., and Elferink, R. O. (2009). Small animal models of hepatocyte transplantation. Methods Mol. Biol. 481, 75–82.
Shafritz, D. A., and Oertel, M. (2011). Model systems and experimental conditions that lead to effective repopulation of the liver by transplanted cells. Int. J. Biochem. Cell Biol. 43, 198–213.
Shafritz, D. A., Oertel, M., Menthena, A., Nierhoff, D., and Dabeva, M. D. (2006). Liver stem cells and prospects for liver reconstitution by transplanted cells. Hepatology 43, S89–S98.
Shi, X.-L., Zhang, Y., Gu, J.-Y., and Ding, Y.-T. (2009). Coencapsulation of hepatocytes with bone marrow mesenchymal stem cells improves hepatocyte-specific functions. Transplantation 88, 1178–1185.
Smets, F., Najimi, M., and Sokal, E. M. (2008). Cell transplantation in the treatment of liver diseases. Pediatr. Transplant. 12, 6–13.
Soto-Gutierrez, A., Navarro-Alvarez, N., Yagi, H., and Yarmush, M. L. (2009). Stem cells for liver repopulation. Curr. Opin. Organ Transplant. 14, 667–673.
Steiner, J. W., Perz, Z. M., and Taichman, L. B. (1966). Cell population dynamics in the liver. A review of quantitative morphological techniques applied to the study of physiological and pathological growth. Exp. Mol. Pathol. 5, 146–181.
Stock, P., Bruckner, S., Ebensing, S., Hempel, M., Dollinger, M. M., and Christ, B. (2010). The generation of hepatocytes from mesenchymal stem cells and engraftment into murine liver. Nat. Protoc. 5, 617–627.
Stock, P., Ebensing, S., Hempel, M., Dollinger, M. M., Seufferlein, T., and Christ, B. (2009). Hepatocyte-like cells derived from human mesenchymal stem cells support regeneration of the mouse liver after acetaminophen-induced injury. Regen. Med. 4, S79.
Taléns-Visconti, R., Bonora, A., Jover, R., Mirabet, V., Carbonell, F., Castell, J. V., and Gómez-Lechón, M. J. (2006). Hepatogenic differentiation of human mesenchymal stem cells from adipose tissue in comparison with bone marrow mesenchymal stem cells. World J. Gastroenterol. 12, 5834–5845.
van Poll, D., Parekkadan, B., Cho, C. H., Berthiaume, F., Nahmias, Y., Tilles, A. W., and Yarmush, M. L. (2008). Mesenchymal stem cell-derived molecules directly modulate hepatocellular death and regeneration in vitro and in vivo. Hepatology 47, 1634–1643.
Vassilopoulos, G. P., Wang, R., and Russell, D. W. (2003). Transplanted bone marrow regenerates liver by cell fusion. Nature 422, 901–904.
Wang, P. P., Wang, J. H., Yan, Z. P., Hu, M. Y., Lau, G. K., Fan, S. T., and Luk, J. M. (2004). Expression of hepatocyte-like phenotypes in bone marrow stromal cells after HGF induction. Biochem. Biophys. Res. Commun. 320, 712–716.
Wang, X., Al-Dhalimy, M., Lagasse, E., Finegold, M., and Grompe, M. (2001). Liver repopulation and correction of metabolic liver disease by transplanted adult mouse pancreatic cells. Am. J. Pathol. 158, 571–579.
Wang, X., Montini, E., Al-Dhalimy, M., Lagasse, E., Finegold, M., and Grompe, M. (2002). Kinetics of liver repopulation after bone marrow transplantation. Am. J. Pathol. 161, 565–574.
Wang, X., Willenbring, H., Akkari, Y., Torimaru, Y., Foster, M., Al-Dhalimy, M., Lagasse, E., Finegold, M., Olson, S., and Grompe, M. (2003). Cell fusion is the principal source of bone-marrow-derived hepatocytes. Nature 422, 897–901.
Weber, A., Groyer-Picard, M.-T., Franco, D., and Dagher, I. (2009). Hepatocyte transplantation in animal models. Liver Transpl. 15, 7–14.
Yagi, H., Soto-Gutierrez, A., Kitagawa, Y., Tilles, A. W., Tompkins, R. G., and Yarmush, M. L. (2010). Bone marrow mesenchymal stromal cells attenuate organ injury induced by lps and burn. Cell Transplant. 19, 823–830.
Yan, C., Zhou, L., and Han, Y.-P. (2008). Contribution of hepatic stellate cells and matrix metalloproteinase 9 in acute liver failure. Liver Int. 28, 959–971.
Yukawa, H., Noguchi, H., Oishi, K., Takagi, S., Hamaguchi, M., Hamajima, N., and Hayashi, S. (2009). Cell Transplantation of adipose tissue-derived stem cells in combination with heparin attenuated acute liver failure in mice. Cell Transplant. 18, 611–618.
Zagoura, D. S., Roubelakis, M. G., Bitsika, V., Trohatou, O., Pappa, K. I., Kapelouzou, A., Antsaklis, A., and Anagnou, N. P. (2011). Therapeutic potential of a distinct population of human amniotic fluid mesenchymal stem cells and their secreted molecules in mice with acute hepatic failure. Gut. doi:10.1136/gutjnl-2011–300908. [Epub ahead of print].
Keywords: cell transplantation, liver stem cells, acute liver injury, stem cell-derived hepatocytes
Citation: Christ B and Brückner S (2012) Rodent animal models for surrogate analysis of cell therapy in acute liver failure. Front. Physio. 3:78. doi: 10.3389/fphys.2012.00078
Received: 07 February 2012; Accepted: 16 March 2012;
Published online: 02 April 2012.
Edited by:
Ali Canbay, University Hospital Essen, GermanyReviewed by:
Isabel Fabregat, University of Barcelona, SpainMartina Mueller-Schilling, University Hospital Regensburg, Germany
Copyright: © 2012 Christ and Brückner. This is an open-access article distributed under the terms of the Creative Commons Attribution Non Commercial License, which permits non-commercial use, distribution, and reproduction in other forums, provided the original authors and source are credited.
*Correspondence: Bruno Christ, Department of Visceral, Transplantation, Thoracic and Vascular Surgery, University Hospital of Leipzig, Liebigstraße 21, D-04103 Leipzig, Germany. e-mail:YnJ1bm8uY2hyaXN0QG1lZGl6aW4udW5pLWxlaXB6aWcuZGU=