- 1 Department of Orthodontics, Institute of Dentistry, University of Helsinki, Helsinki, Finland
- 2 Finnish Graduate School of Oral Sciences, Turku, Finland
- 3 Center of Medical Biotechnology, University of Duisburg-Essen, Essen, Germany
- 4 Oral and Maxillofacial Diseases, Helsinki University Central Hospital, Helsinki, Finland
Greig cephalopolysyndactyly syndrome (GCPS) is an autosomal dominant disorder with polydactyly and syndactyly of the limbs and a broad spectrum of craniofacial abnormalities. Craniosynostosis of the metopic suture (interfrontal suture in mice) is an important but rare feature associated with GCPS. GCPS is caused by mutations in the transcription factor GLI3, which regulates Hedgehog signaling. The Gli3 loss-of-function (Gli3Xt-J/Xt-J) mouse largely phenocopies the human syndrome with the mice exhibiting polydactyly and several craniofacial abnormalities. Here we show that Gli3Xt-J/Xt-J mice exhibit ectopic ossification in the interfrontal suture and in the most severe cases the suture fuses already prior to birth. We show that abnormalities in frontal bones occur early in calvarial development, before the establishment of the interfrontal suture. It provides a model for the metopic suture pathology that can occur in GCPS.
Introduction
Heterozygous mutations in the gene encoding GLI3 causes Greig cephalopolysyndactyly syndrome (GCPS, MIM 175700; Vortkamp et al., 1991). GCPS patients have limb and craniofacial defects; typically broad base of the nose, mild hypertelorism, prominent forehead, and macrocephaly. Recent findings indicate that premature fusion, craniosynostosis, of the metopic suture is an infrequent, but significant feature of GCPS (McDonald-McGinn et al., 2010; Hurst et al., 2011). The metopic suture is the first calvarial suture to fuse in humans and this normally occurs during the first 12 months after birth. In mice, the interfrontal suture corresponds to the human metopic suture and its posterior part is the only suture that fuses, taking place on the 15th postnatal day (Sahar et al., 2005).
The Gli3 extra-toe mouse (Gli3Xt-J/Xt-J) is an animal model for the GCPS (Vortkamp et al., 1992). Mice have polydactylous and syndactylous limbs that lack digit identity (Hui and Joyner, 1993). Gli3Xt-J/Xt-J mice have extensive brain malformations (Tole et al., 2000; Blaess et al., 2008). Eyes are severely truncated and ears are misplaced. Exencephaly and oedema are also commonly detected. In the original description of the Gli3Xt-J/Xt-J mice the skull is reported to be abnormal in shape (Johnson, 1967). We have previously shown that premature fusion of the lambdoid suture is a fully penetrant feature of Gli3Xt-J/Xt-J mice (Rice et al., 2010).
The Gli3Xt-J/Xt-J mouse has a 51.5-kb intragenic deletion of the Gli3 gene (Maynard et al., 2002). This deletion disrupts the open reading frame of Gli3 resulting in an abnormal transcript truncated in the first zinc finger domain (Buscher et al., 1998). This is predicted to prevent any specific binding to DNA and thus cause loss-of-function of Gli3. Gli3 is a transcription factor functioning as a downstream mediator of Hh signaling and the presence of Hh ligand modulates its activity. When Hh ligand is absent, Gli3 is proteolytically cleaved into a repressor form, which actively inhibits transcription of Hh target genes. In the presence of Hh ligand the cleavage of Gli3 into the truncated form is prevented and the accumulating full-length Gli3 is able to function as an activator of Hh target gene transcription (Wang et al., 2000).
Sonic hedgehog (Shh) and Indian hedgehog (Ihh) are the Hh ligands expressed in the head during development. Shh acts as a vital organizer of the head in mice; Shh−/− mice have an early and a very severe head defect, which restricts investigation of its other roles later in development (Chiang et al., 1996). Deletion of Shh solely from neural crest cells causes a significant truncation of the neural crest derived frontal bones (Jeong et al., 2004). In the absence of Ihh, intramembranous calvarial bones are smaller (St-Jacques et al., 1999). There is evidence that this may be due to accelerated early differentiation of osteoprogenitor cells (Abzhanov et al., 2007). However, loss of Ihh has also been shown to reduce Bmp expression in the calvaria and Ihh is postulated to be a positive regulator of intramembranous ossification (Lenton et al., 2011).
Here we describe in detail the effect the loss-of-function of Gli3 has on the frontal bone development in mice. We show that heterotopic ossification occurs in the interfrontal suture of Gli3Xt-J/Xt-J mice. The phenotype varies, but in severe cases loss-of-function of Gli3 leads to premature interfrontal suture fusion. We also show that structural abnormalities in the brain associate with abnormalities in frontal bone development. The Gli3Xt-J/Xt-J mouse can thus be used as a model to investigate the mechanism by which the pathogenic suture closes.
Materials and Methods
Animals
Gli3+/Xt-J mice maintenance and PCR genotyping has been described previously (Rice et al., 2010). We generated Gli3Xt-J/Xt-J mice by mating Gli3+/Xt-J mice, which were maintained on C57BL/6J background. Wt littermates were used as controls. All experiments were approved by the University of Helsinki, Helsinki University Hospital and the Southern Finland Council animal welfare and ethics committees.
Skeletal, Toluidine Blue, and ALP Staining
Heads of Gli3Xt-J/Xt-J and Wt littermates aged embryonic day (E)16.5, E17.5, and E18.5 were fixed in 95% ethanol overnight and stained with Alcian blue and Alizarin red and then cleared in 1% KOH and transferred to glycerol, as described in detail previously (Rice et al., 2003b). As Gli3Xt-J/Xt-J mice die at birth further assessment of later stages of development was not possible. Toluidine blue staining to stain cartilage was performed with 1% aqueous solution on sections. Whole mount alkaline phosphatase staining was carried out on E13.5 aged Wt and Gli3Xt-J/Xt-J heads, as previously reported (Ishii et al., 2003). Tissue was fixed in 4% paraformaldehyde overnight at 4°C, washed in NTMT (0.1 M NaCl + 0.1 M Tris–HCl pH 9.5 + 50 mM Magnesium chloride + 0.1% Tween-20) and then stained using NBT/BCIP (Roche). Alkaline phosphatase staining was also done on 7 μm thick paraffin sections of 4% paraformaldehyde fixed E13.5 aged heads. After deparaffinization the sections were washed in NTMT and stained with NBT/BCIP (Roche) and then mounted with UltraKitt (J.T.Baker). P-values were calculated by t-test.
In situ Hybridization
E16.5 aged Gli3Xt-J/Xt-J and Wt heads were fixed overnight in 4% paraformaldehyde at 4°C, embedded in paraffin wax and further sectioned at 7 μm intervals. Runx2 (Rice et al., 2003a) and Bsp (Rice et al., 1999) 35S-UTP riboprobes were prepared and in situ hybridization was performed as previously described (Rice et al., 2000).
Results
Metopic synostosis is a significant feature of GCPS, which is caused by mutations in GLI3. Therefore we investigated the frontal bone development of Gli3Xt-J/Xt-J mice. Staining of wild type (Wt) and Gli3Xt-J/Xt-J mice heads with Alcian blue and Alizarin red S revealed that at E18.5 the frontal bone morphology of Gli3Xt-J/Xt-J mice was abnormal (Figures 1A–J). At E18.5 there were heterotopic bones of varying size and shape in the interfrontal suture of all Gli3Xt-J/Xt-J mice [Figure 1E (schematic); red domain, 1G and H; double-lined arrows]. In specimens with numerous, fused heterotopic bones, the interfrontal suture exhibited synostosis anteriorly (Figures 1D,G). The medial edge of the frontal bones had a cleft like hypoplasia anteriorly in all samples (Figures 1G,H; black arrow, Figures 1I,J). In some samples, there was less heterotopic ossification and the frontal bones were hypoplastic and the suture width was increased, especially anteriorly (Figure 1I; black arrow head). At E18.5, the length of the Gli3Xt-J/Xt-J head was similar to that of Wt mice [mean length of Wt head (anterior of nasal bones to occiput): 9.1 ± 0.2 mm, and Gli3Xt-J/Xt-J head: 8.7 ± 0.5 mm, P = 0.2; Figure 1E; green arrow]. At the level of anterior interfrontal suture, however, the Gli3Xt-J/Xt-J head was significantly wider (mean width in Wt mice: 2.8 ± 0.1 mm, and Gli3Xt-J/Xt-J mice: 3.5 ± 0.2 mm, P < 0.001; Figure 1E; green arrow). Similarly in GCPS patients with metopic synostosis the distance between the eyes was not decreased even though metopic synostosis is usually associated with hypotelorism (Hurst et al., 2011).
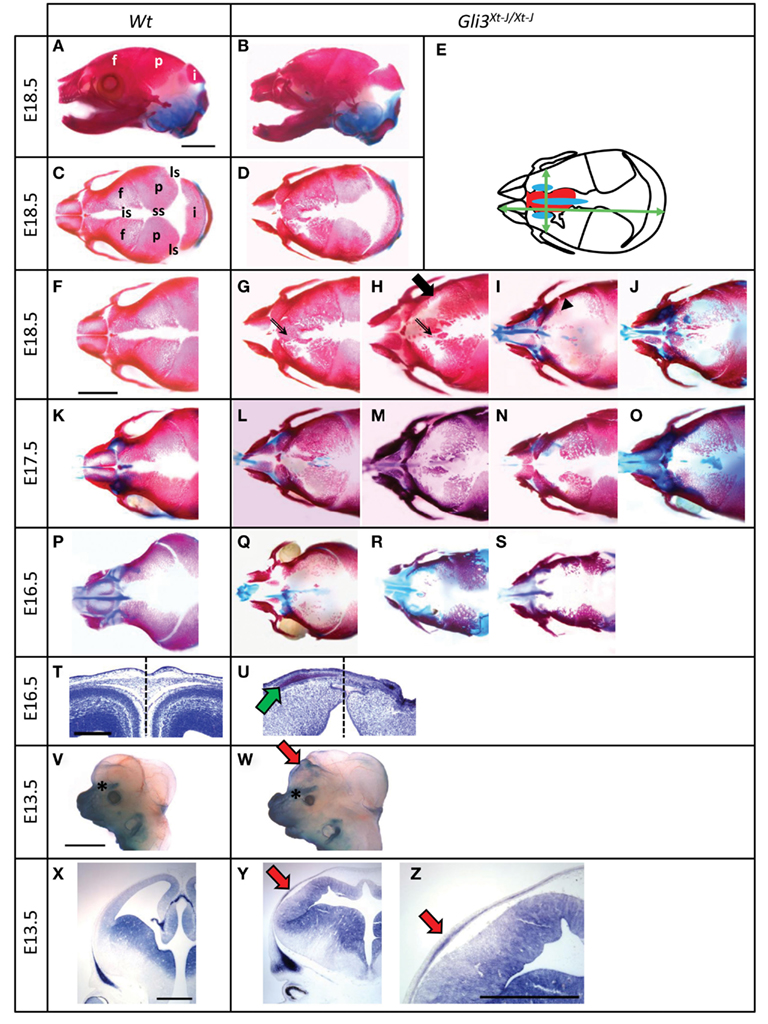
Figure 1. Frontal bone development in Gli3Xt-J/Xt-J mice. Alcian blue and Alizarin red S stained heads of Wt (A,C,F,K,P) and Gli3Xt-J/Xt-J(B,D,G–J,L–O,Q–S) embryos and a schematic of Gli3Xt-J/Xt-J head indicating heterotopic ossification (red), heterotopic cartilage formation (blue), and measurements of the head (green arrows) (E). At E18.5 Gli3Xt-J/Xt-J frontal bones are abnormally shaped (black arrow in H) and in the interfrontal suture there are heterotopic bones [(G,H); double-lined arrow] that have fused in some samples causing suture synostosis. In samples with less heterotopic bones the suture is wider compared to Wt [(I); arrowhead]. At E17.5 Gli3Xt-J/Xt-J frontal bones have similar features as at E18.5. At E16.5 Gli3Xt-J/Xt-J frontal bones are hypoplastic, but ectopic ossification is already evident. Ectopic cartilage is seen in the interfrontal suture of Gli3Xt-J/Xt-J mice [(I,J,L,N,O,Q,R,U); green arrow]. Toluidine blue stained frontal sections through the posterior interfrontal suture of Wt (T) and Gli3Xt-J/Xt-J (U) heads at E16.5. Morphology of the Gli3Xt-J/Xt-J brain is abnormal (U). Dash line in T and U indicate the midline of the head. Alkaline phosphatase stained E13.5 heads of Wt (V,X) and Gli3Xt-J/Xt-J (W,Y,Z) embryos, where heterotopic osteoblast differentiation is detected in Gli3Xt-J/Xt-J mice [(W,Y,Z); red arrow]. f, Frontal bone; i, interparietal bone; is, interfrontal suture; ls, lambdoid suture; p, parietal bone; ss, sagittal suture. Scale bars: 2 mm, except 300 μm in (T) and 500 μm in (X,Z).
At E17.5, the abnormalities seen in the Gli3Xt-J/Xt-J frontal bone morphology (Figures 1K–O) were similar to the features observed at E18.5. In two specimens (n = 8), however, no heterotopic ossification was detected at this stage (Figures 1N,O). At E16.5 the frontal bones of Gli3Xt-J/Xt-J mice (Figures 1P–S) were all hypoplastic compared to Wt, and already at this stage heterotopic ossification was seen in the interfrontal suture (Figures 1Q,R).
Heterotopic cartilage was also detected in the interfrontal suture of Gli3Xt-J/Xt-J mice (Figure 1E; blue domains, Figures 1I,J,L,N,O,Q,R,U; green arrow). However, endochondral ossification was not apparent. Cartilage formation was not detected in controls at any stage examined (Figures 1F,K,P,T).
We investigated if Gli3 participates in earlier frontal bone development, prior to mineralization, by performing alkaline phosphatase staining (Figures 1V–Z). At E13.5 similar sized frontal bone primordia were present above the eye in Gli3Xt-J/Xt-J and Wt mice. However, an additional alkaline phosphatase domain was seen more apically in Gli3Xt-J/Xt-J samples (Figures 1W,Y,Z; red arrows). Gli3 is normally expressed in the region of ectopic ossification; in the undifferentiated mesenchyme (Huang et al., 2008; Rice et al., 2010).
We studied osteoblast differentiation in Gli3Xt-J/Xt-J mice by examining the expression of osteoblastic markers at E16.5 (Figures 2A–D). The early osteoblastic marker; Runx2 as well as a later marker; Bone sialoprotein (Bsp) were expressed in the developing frontal bones of Gli3Xt-J/Xt-J mice at comparable levels to Wt samples. However, already at this early stage, Runx2 was additionally expressed almost across the whole Gli3Xt-J/Xt-J suture (Figure 2B; arrow). Bsp was also expressed ectopically in the Gli3Xt-J/Xt-J interfrontal suture (Figure 2D; arrows), but by smaller population of cells than those expressing Runx2.
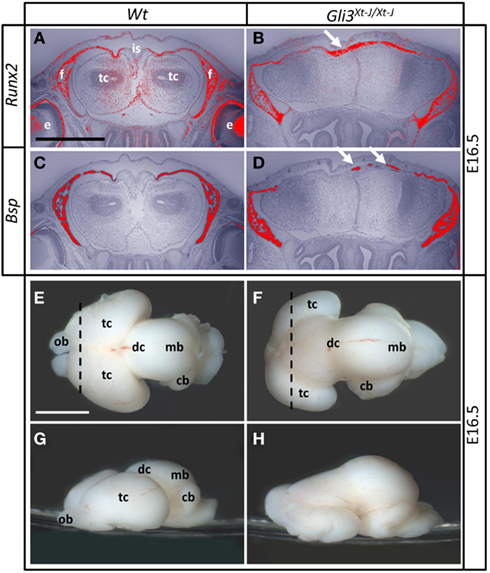
Figure 2. Osteoblast differentiation and brain morphology of Gli3Xt-J/Xt-J mice at E16.5. Frontal sections of Wt (A,C) and Gli3Xt-J/Xt-J heads (B,D). (A–D) The osteoblastic markers Runx2 and Bsp are expressed in the frontal bones and ectopically in the interfrontal suture (arrows) of Gli3Xt-J/Xt-J mice. Dash line in (E,F) represent the plane of section in (A–D) respectively. Brains of Wt (E,G) and Gli3Xt-J/Xt-J(F,H) mice. The Gli3Xt-J/Xt-J brain has hypoplastic dorsal telencephalon and diencephalon resides more anteriorly. The cerebellum extends more ventrally. cb, Cerebellum; dc, diencephalon, f, frontal; is, interfrontal suture; e, eye; mb, midbrain; ob, olfactory bulb; tc, telencephalon. Scale bars are 1 mm in (A), 2 mm in (E).
It has been established that the brain interacts with the calvaria during development and that the anatomy of the Gli3Xt-J/Xt-J mouse brain is abnormal (Schowing, 1968; Opperman et al., 1993; Bradley et al., 1997; Aoto et al., 2002; Blaess et al., 2008). We therefore investigated the morphology of Gli3Xt-J/Xt-J brains at E16.5 to see how the calvarial phenotype correlates with the underlying brain abnormalities (Figures 2E–H). The olfactory bulbs were absent in Gli3Xt-J/Xt-J mice. The dorsomedial telencephalon was hypoplastic and the diencephalon extended more anteriorly compared to Wt littermates. The midbrain was enlarged and the cerebellum was wider extending more ventrally. The structure of the Gli3Xt-J/Xt-J brain is reported to be similar few days later, at the time of birth (Blaess et al., 2008). Sections across the frontal bone region at E16.5 showed an abnormal relation of the frontal bones to the underlying brain in Gli3Xt-J/Xt-J mice (Figures 2A–D). In Wt mice the frontal bone was superior to the cerebral hemisphere and the interfrontal suture had formed between the two frontal bones above the falx cerebri that separates the two hemispheres. Gli3Xt-J/Xt-J cerebral hemispheres were not clearly separated as the falx cerebri was absent. The Gli3Xt-J/Xt-J frontal bones were detected on either side of the developing brain, but ossification was also present in the midline. Additionally, in the Wt mice the widest cross sectional part of the frontal bones resided where the brain was widest horizontally. The Gli3Xt-J/Xt-J frontal bones, however, were widest more caudally, below the greatest diameter of the brain.
Discussion
Metopic synostosis is a significant feature of GCPS, which is caused by mutations in GLI3. In many aspects Gli3Xt-J/Xt-J mice model GCPS and in this study we show that Gli3Xt-J/Xt-J mice present heterotopic ossification in the interfrontal suture that in severe cases leads to its premature fusion. As in humans, the phenotype in mice varies; at E18.5 some of the sutures have already fused, while in others the ossification is delayed. Yet in all of the studied Gli3Xt-J/Xt-J mice the frontal bone morphology is abnormal and heterotopic bones are detected in all samples at E18.5.
Markers of early and late osteoblast differentiation are expressed normally in the developing frontal bones of Gli3Xt-J/Xt-J mice and also in the heterotopic bones indicating that Gli3 is not vital for intramembranous osteoblast differentiation. We have previously shown that Gli3 regulates osteoprogenitor proliferation as well as osteoblast differentiation during calvarial bone development. In the absence of Gli3, hedgehog (Hh) signaling is activated ectopically in the sutural mesenchyme and in these cells the expression of the basic helix-loop-helix transcription factor, Twist1, is reduced, while transcription of osteoblast regulatory gene, Runx2, is upregulated leading to premature fusion of the lambdoid suture (Rice et al., 2010). Here we further show that in the interfrontal suture of Gli3Xt-J/Xt-J mice there is not only heterotopic ossification, but the morphology of the frontal bones is also abnormal. The defect in the frontal bone development occurs early during development, prior to mineralization, as ectopic alkaline phosphatase staining is detected at E13.5 in Gli3Xt-J/Xt-J mice. These findings suggest that Gli3 may also function in calvarial bone patterning. The situation is similar during limb development; deletion of Gli3 causes patterning abnormalities (polydactyly; Litingtung et al., 2002; te Welscher et al., 2002), with only mild defects in endochondral ossification (Koziel et al., 2005).
Ectopic cartilage was detected in the interfrontal suture of Gli3Xt-J/Xt-J mice. During intramembranous ossification of calvarial bones cartilage is transiently detected in the sutures (Pritchard et al., 1956) and chondrocyte markers, Sox9, and type II collagen, are expressed in the calvaria (Aberg et al., 2005). Transient secondary cartilage rods in sutures may be a reaction to mechanical stress, particularly related to altered mechanical forces in the dura mater (Solem et al., 2011). Altered mechanical forces may indeed affect the interfrontal suture of Gli3Xt-J/Xt-J mice. In a mouse model of Apert syndrome (Fgfr2+/S252W) increased cartilage proliferation or altered progenitor cell differentiation has been postulated to cause heterotopic cartilage formation in the sagittal suture, prior to premature suture fusion (Wang et al., 2005). During endochondral ossification Gli3 represses an early stage of chondrocyte differentiation regulating the amount of proliferating chondrocytes (Koziel et al., 2005). Endodermal layer of posterior frontal suture in mice fuses through endochondral ossification and cartilage is observed at this site postnatally (Sahar et al., 2005). Gli3 may also function in the calvaria to restrict chondrocyte differentiation. The possible role of Gli3 during the posterior frontal suture fusion is, however, impossible to study in Gli3Xt-J/Xt-J mice as they die at birth.
We found that the abnormal morphology of the frontal bones and the interfrontal suture in Gli3Xt-J/Xt-J mice correlates with brain defects in Gli3Xt-J/Xt-J mice. Aberrant morphology of the Gli3Xt-J/Xt-J brain is likely to influence frontal bone development. The dura mater is shown to affect calvarial cell migration and differentiation by mechanical and biochemical signals (Roth et al., 1996; Gagan et al., 2007). It is also possible that the number and/or the migration of neural crest cells may underlie frontal bone abnormalities. Neural crest cells arise from the future caudal forebrain, midbrain, and prorhombomere A of the hindbrain (Jiang et al., 2002) and these structures are abnormal in Gli3Xt-J/Xt-J mice (Aoto et al., 2002). Some of the GCPS patients, although exact numbers of patients have not been reported, also have structural brain anomalies, such as bilateral frontal and parietal atrophy, mild cerebral ventriculomegaly and hypoplasia of corpus callosum (Hurst et al., 2011). The morphology of the brain in GCPS patients is not, however, extensively reported so it is not known how well the brain shape has been perceived. Although the brain and the calvaria develop in such close proximity little is known about how the brain influences calvaria development. Recent studies on humans and mice with craniosynostosis have shown that the pattern of premature suture fusion and the brain shape correlate surprisingly little (Richtsmeier et al., 2006; Aldridge et al., 2010). This indicates that the brain and the calvaria develop independently and their development has been programmed early. Gli3Xt-J/Xt-J mice provide an appealing model to study further the co-development of the brain and the calvaria as both organs are severely affected.
In conclusion, our results support the evidence that Gli3 has a significant role in frontal bone development as in Gli3Xt-J/Xt-J mice the patterning of the frontal bones is abnormal and heterotopic ossification in the interfrontal suture can lead to its premature fusion. We thus provide a valuable tool to study the mechanism of metopic suture fusion in GCPS patients. Our findings also encourage more careful examination of the metopic suture in infants with mutations in GLI3.
Conflict of Interest Statement
The authors declare that the research was conducted in the absence of any commercial or financial relationships that could be construed as a potential conflict of interest.
Acknowledgments
We thank Airi Sinkko and Marjatta Kivekäs for technical assistance. Work was supported by Biocentrum Helsinki, Helsinki University, Helsinki University Central Hospital, Sigrid Jusélius Foundation, Academy of Finland and FINDOS.
References
Aberg, T., Rice, R., Rice, D., Thesleff, I., and Waltimo-Siren, J. (2005). Chondrogenic potential of mouse calvarial mesenchyme. J. Histochem. Cytochem. 53, 653–663.
Abzhanov, A., Rodda, S. J., McMahon, A. P., and Tabin, C. J. (2007). Regulation of skeletogenic differentiation in cranial dermal bone. Development 134, 3133–3144.
Aldridge, K., Hill, C. A., Austin, J. R., Percival, C., Martinez-Abadias, N., Neuberger, T., Wang, Y., Jabs, E. W., and Richtsmeier, J. T. (2010). Brain phenotypes in two FGFR2 mouse models for Apert syndrome. Dev. Dyn. 239, 987–997.
Aoto, K., Nishimura, T., Eto, K., and Motoyama, J. (2002). Mouse GLI3 regulates Fgf8 expression and apoptosis in the developing neural tube, face, and limb bud. Dev. Biol. 251, 320–332.
Blaess, S., Stephen, D., and Joyner, A. L. (2008). Gli3 coordinates three-dimensional patterning and growth of the tectum and cerebellum by integrating Shh and Fgf8 signaling. Development 135, 2093–2103.
Bradley, J. P., Levine, J. P., McCarthy, J. G., and Longaker, M. T. (1997). Studies in cranial suture biology: regional dura mater determines in vitro cranial suture fusion. Plast. Reconstr. Surg. 100, 1091–9; discussion 1100–1102.
Buscher, D., Grotewold, L., and Ruther, U. (1998). The XtJ allele generates a Gli3 fusion transcript. Mamm. Genome 9, 676–678.
Chiang, C., Litingtung, Y., Lee, E., Young, K. E., Corden, J. L., Westphal, H., and Beachy, P. A. (1996). Cyclopia and defective axial patterning in mice lacking sonic hedgehog gene function. Nature 383, 407–413.
Gagan, J. R., Tholpady, S. S., and Ogle, R. C. (2007). Cellular dynamics and tissue interactions of the dura mater during head development. Birth Defects Res. C Embryo Today 81, 297–304.
Huang, X., Goudy, S. L., Ketova, T., Litingtung, Y., and Chiang, C. (2008). Gli3-deficient mice exhibit cleft palate associated with abnormal tongue development. Dev. Dyn. 237, 3079–3087.
Hui, C. C., and Joyner, A. L. (1993). A mouse model of greig cephalopolysyndactyly syndrome: the extra-toesJ mutation contains an intragenic deletion of the Gli3 gene. Nat. Genet. 3, 241–246.
Hurst, J. A., Jenkins, D., Vasudevan, P. C., Kirchhoff, M., Skovby, F., Rieubland, C., Gallati, S., Rittinger, O., Kroisel, P. M., Johnson, D., Biesecker, L. G., and Wilkie, A. O. (2011). Metopic and sagittal synostosis in Greig cephalopolysyndactyly syndrome: five cases with intragenic mutations or complete deletions of GLI3. Eur. J. Hum. Genet. 19, 757–762.
Ishii, M., Merrill, A. E., Chan, Y. S., Gitelman, I., Rice, D. P., Sucov, H. M., and Maxson, R. E. Jr. (2003). Msx2 and Twist cooperatively control the development of the neural crest-derived skeletogenic mesenchyme of the murine skull vault. Development 130, 6131–6142.
Jeong, J., Mao, J., Tenzen, T., Kottmann, A. H., and McMahon, A. P. (2004). Hedgehog signaling in the neural crest cells regulates the patterning and growth of facial primordia. Genes Dev. 18, 937–951.
Jiang, X., Iseki, S., Maxson, R. E., Sucov, H. M., and Morriss-Kay, G. M. (2002). Tissue origins and interactions in the mammalian skull vault. Dev. Biol. 241, 106–116.
Johnson, D. R. (1967). Extra-toes: anew mutant gene causing multiple abnormalities in the mouse. J. Embryol. Exp. Morphol. 17, 543–581.
Koziel, L., Wuelling, M., Schneider, S., and Vortkamp, A. (2005). Gli3 acts as a repressor downstream of Ihh in regulating two distinct steps of chondrocyte differentiation. Development 132, 5249–5260.
Lenton, K., James, A. W., Manu, A., Brugmann, S. A., Birker, D., Nelson, E. R., Leucht, P., Helms, J. A., and Longaker, M. T. (2011). Indian hedgehog positively regulates calvarial ossification and modulates bone morphogenetic protein signaling. Genesis 49, 784–796.
Litingtung, Y., Dahn, R. D., Li, Y., Fallon, J. F., and Chiang, C. (2002). Shh and Gli3 are dispensable for limb skeleton formation but regulate digit number and identity. Nature 418, 979–983.
Maynard, T. M., Jain, M. D., Balmer, C. W., and LaMantia, A. S. (2002). High-resolution mapping of the Gli3 mutation extra-toes reveals a 51.5-kb deletion. Mamm. Genome 13, 58–61.
McDonald-McGinn, D. M., Feret, H., Nah, H. D., Bartlett, S. P., Whitaker, L. A., and Zackai, E. H. (2010). Metopic craniosynostosis due to mutations in GLI3: a novel association. Am. J. Med. Genet. A 152A, 1654–1660.
Opperman, L. A., Sweeney, T. M., Redmon, J., Persing, J. A., and Ogle, R. C. (1993). Tissue interactions with underlying dura mater inhibit osseous obliteration of developing cranial sutures. Dev. Dyn. 198, 312–322.
Pritchard, J. J., Scott, J. H., and Girgis, F. G. (1956). The structure and development of cranial and facial sutures. J. Anat. 90, 73–86.
Rice, D. P., Aberg, T., Chan, Y., Tang, Z., Kettunen, P. J., Pakarinen, L., Maxson, R. E., and Thesleff, I. (2000). Integration of FGF and TWIST in calvarial bone and suture development. Development 127, 1845–1855.
Rice, D. P., Connor, E. C., Veltmaat, J. M., Lana-Elola, E., Veistinen, L., Tanimoto, Y., Bellusci, S., and Rice, R. (2010). Gli3Xt-J/Xt-J mice exhibit lambdoid suture craniosynostosis which results from altered osteoprogenitor proliferation and differentiation. Hum. Mol. Genet. 19, 3457–3467.
Rice, D. P., Kim, H. J., and Thesleff, I. (1999). Apoptosis in murine calvarial bone and suture development. Eur. J. Oral Sci. 107, 265–275.
Rice, D. P., Rice, R., and Thesleff, I. (2003a). Molecular mechanisms in calvarial bone and suture development, and their relation to craniosynostosis. Eur. J. Orthod. 25, 139–148.
Rice, R., Rice, D. P., Olsen, B. R., and Thesleff, I. (2003b). Progression of calvarial bone development requires Foxc1 regulation of Msx2 and Alx4. Dev. Biol. 262, 75–87.
Richtsmeier, J. T., Aldridge, K., DeLeon, V. B., Panchal, J., Kane, A. A., Marsh, J. L., Yan, P., and Cole, T. M. III. (2006). Phenotypic integration of neurocranium and brain. J. Exp. Zool. B Mol. Dev. Evol. 306, 360–378.
Roth, D. A., Bradley, J. P., Levine, J. P., McMullen, H. F., McCarthy, J. G., and Longaker, M. T. (1996). Studies in cranial suture biology: part II. Role of the dura in cranial suture fusion. Plast. Reconstr. Surg. 97, 693–699.
Sahar, D. E., Longaker, M. T., and Quarto, N. (2005). Sox9 neural crest determinant gene controls patterning and closure of the posterior frontal cranial suture. Dev. Biol. 280, 344–361.
Schowing, J. (1968). Demonstration of the inductive role of the brain in osteogenesis of the embryonic skull of the chicken. J. Embryol. Exp. Morphol. 19, 83–94.
Solem, R. C., Eames, B. F., Tokita, M., and Schneider, R. A. (2011). Mesenchymal and mechanical mechanisms of secondary cartilage induction. Dev. Biol. 356, 28–39.
St-Jacques, B., Hammerschmidt, M., and McMahon, A. P. (1999). Indian hedgehog signaling regulates proliferation and differentiation of chondrocytes and is essential for bone formation. Genes Dev. 13, 2072–2086.
te Welscher, P., Zuniga, A., Kuijper, S., Drenth, T., Goedemans, H. J., Meijlink, F., and Zeller, R. (2002). Progression of vertebrate limb development through SHH-mediated counteraction of GLI3. Science 298, 827–830.
Tole, S., Ragsdale, C. W., and Grove, E. A. (2000). Dorsoventral patterning of the telencephalon is disrupted in the mouse mutant extra-toes(J). Dev. Biol. 217, 254–265.
Vortkamp, A., Franz, T., Gessler, M., and Grzeschik, K. H. (1992). Deletion of GLI3 supports the homology of the human Greig cephalopolysyndactyly syndrome (GCPS) and the mouse mutant extra toes (Xt). Mamm. Genome 3, 461–463.
Vortkamp, A., Gessler, M., and Grzeschik, K. H. (1991). GLI3 zinc-finger gene interrupted by translocations in Greig syndrome families. Nature 352, 539–540.
Wang, B., Fallon, J. F., and Beachy, P. A. (2000). Hedgehog-regulated processing of Gli3 produces an anterior/posterior repressor gradient in the developing vertebrate limb. Cell 100, 423–434.
Wang, Y., Xiao, R., Yang, F., Karim, B. O., Iacovelli, A. J., Cai, J., Lerner, C. P., Richtsmeier, J. T., Leszl, J. M., Hill, C. A., Yu, K., Ornitz, D. M., Elisseeff, J., Huso, D. L., and Wang Jabs, E. (2005). Abnormalities in cartilage and bone development in the Apert syndrome FGFR2(+/S252W) mouse. Development 132, 3537–3548.
Keywords: craniosynostosis, Gli3, bone, developmental biology, suture
Citation: Veistinen L, Takatalo M, Tanimoto Y, Kesper DA, Vortkamp A and Rice DPC (2012) Loss-of-function of Gli3 in mice causes abnormal frontal bone morphology and premature synostosis of the interfrontal suture. Front. Physio. 3:121. doi: 10.3389/fphys.2012.00121
Received: 06 February 2012; Paper pending published: 20 February 2012;
Accepted: 12 April 2012; Published online: 03 May 2012.
Edited by:
Gianpaolo Papaccio, Second University of Naples, ItalyReviewed by:
Daniel Graf, University of Zurich, SwitzerlandJavier Catón, Universidad Complutense de Madrid, Spain
Copyright: © 2012 Veistinen, Takatalo, Tanimoto, Kesper, Vortkamp and Rice. This is an open-access article distributed under the terms of the Creative Commons Attribution Non Commercial License, which permits non-commercial use, distribution, and reproduction in other forums, provided the original authors and socurce are credited.
*Correspondence: David P. C. Rice, Department of Orthodontics, Institute of Dentistry, Room C229a, Biomedicum Helsinki, PL 63 (Haartmaninkatu 8), 00014 University of Helsinki, Helsinki, Finland. e-mail:ZGF2aWQucmljZUBoZWxzaW5raS5maQ==