- 1 General Psychology (Cognition), Institute of Psychology, University of Zürich, Zürich, Switzerland
- 2 Institute of Psychology, RWTH Aachen University, Aachen, Germany
The human cognitive system is equipped with various processes for dealing with everyday challenges. One of such processes is the inhibition of currently irrelevant goals or mental task-sets, which can be seen as a response to the critical event of information overflow in the cognitive system and challenging the cognitive system’s ability to keep track of ongoing demands. In two experiments, we investigate the flexibility of the inhibitory process by inserting rare non-critical events (25% of all trials), operationalized as univalent stimuli (i.e., unambiguous stimuli that call for only one specific task in a multitasking context), and by introducing the possibility to prepare for an upcoming task (Experiment 2). We found that the inhibitory process is not influenced by a cue informing subjects about the upcoming occurrence of a univalent stimulus. However, the introduction of univalent stimuli allowed preparatory processes to modify the impact of the inhibitory process. Therefore, our results suggest that inhibitory processes are engaged in a rather global manner, not taking into account variations in stimulus valence, which we took as operationalization of critical, conflict-inducing events in the ongoing stream of information processing. However, rare uncritical events, such as univalent stimuli that do not cause conflict and interference in the processing stream, appear to alter the way the cognitive system can take advantage of preparatory processes.
Introduction
Researchers in cognitive psychology usually refer to task activation and task inhibition processes when trying to explain human behavior in everyday life. Cognitive representations of tasks (i.e., task-sets; e.g., Rogers and Monsell, 1995; Monsell, 1996) are thought to comprise all elements of a task, such as the to be encountered stimuli, the required responses, and the cues used to indicate which task is the relevant one in the current trial. When investigating the application of these task-sets, cognitive psychologists assume that task-sets are activated and implemented in the cognitive system to guarantee the successful achievement of goals, such as writing an article or phoning a friend (see, e.g., Miller and Cohen, 2001). However, even though goal-directed behavior is successful in many situations, people also encounter situations in which the successful pursuit of the goal at hand becomes difficult and they fail.
One classical, widely studied critical situation is multitasking, where people are asked to switch rapidly from one task to another, such as keeping an eye on the children in the living room while preparing lunch for them. Furthermore, although activation of currently relevant task-sets is a powerful mechanism, competition among several activated task-sets causes interference and hampers the successful implementation of the currently appropriate task-set. This interference between task-sets has been suggested to be causal for the observed performance costs when there is a switch of task (i.e., a sequence like AB, where A and B denote to different tasks) compared to a task repetition (i.e., AA). These switch costs have been observed numerous times in task switching studies (see, e.g., Kiesel et al., 2010, for a review).
In task switching, interference among task-sets is thought to arise because of shared stimuli and responses which activate associated task-sets in a bottom-up manner, for instance when subjects are asked to judge a digit based on its magnitude (i.e., smaller vs. larger than five) or parity (i.e., odd vs. even, see, e.g., Koch and Allport, 2006). The notion of competition and interference among task-sets has fostered the proposal of inhibitory mechanisms that reduce the activation of a competing task-set and therefore help the selection of the now appropriate task-set and reduce interference (see, e.g., Monsell, 2003). The present study is aimed at examining inhibitory processes in task switching.
An empirical marker of inhibitory processes in the selection among various task-sets are the so-called n−2 task repetition costs (Mayr and Keele, 2000; see Koch et al., 2010, for a review). These costs are calculated as the performance difference between n−2 task switches (i.e., sequences such as CBA) and n−2 repetitions (sequences such as ABA). An account that assumes activation only would predict that switching back to a task that was recently (i.e., two trials earlier) performed would facilitate performance due to repetition priming (i.e., residual activation) and thus decrease reaction time (RTs). In contrast, an inhibition account predicts exactly the opposite, namely that after having performed task A, subjects have to inhibit the task to successfully switch to task B. When encountering task A again, its activation is still reduced below baseline, so that activating task A again (and thereby overcoming residual inhibition) takes longer and increases RTs relative to the n−2 non-repetition control condition (e.g., Mayr and Keele, 2000; Gade and Koch, 2007). This is exactly what has been found in many studies (see Koch et al., 2010, for a review), establishing n−2 task repetition costs as a valid marker for inhibitory mechanisms in the performance of task sequences.
One question that has arisen shortly after the discovery of n−2 repetition costs was related to the conditions that trigger inhibitory mechanisms in multitasking. Studies aiming at the identification of the triggering conditions of n−2 repetition costs investigated response-related factors such as response selection or execution (for example Schuch and Koch, 2003; Gade and Koch, 2007; Philipp et al., 2007a; Schneider and Verbruggen, 2008), while other studies identified cue-related factors (Houghton et al., 2009; Grange and Houghton, 2011) or stimulus-related factors (Arbuthnott, 2008; Sdoia and Ferlazzo, 2008) that gave rise to n−2 repetition costs. Furthermore, n−2 repetition costs were found to be largely insensitive to variations in preparation time (Mayr and Keele, 2000; Schuch and Koch, 2003; Gade and Koch, 2008), suggesting that engaging in active cognitive preparation of a task-set does not usually help to overcome the residual inhibition (but see Grange and Houghton, 2011). Moreover, n−2 task repetition costs were found even though subjects knew that they will have to perform the just inhibited task again (Mayr and Keele, 2000; Koch et al., 2006). To conclude, inhibition of competing tasks, which can be measured as n−2 task repetition costs, is a powerful means of the cognitive system to deal with critical situations of task conflict, that is, situations involving several active task-sets and the need to select one of them to successfully to meet the situational requirements. However, the triggering conditions are not yet fully understood.
In fact, although being reliably obtained, the size of n−2 task repetition costs varied widely across studies. This may suggest that the amount of inhibition (as reflected in the size of n−2 repetition costs) is tied to the amount of conflict experienced in the trial before the current trial (i.e., trial n−1). In line with this suggestion, Gade and Koch (2005) found that the size of n−2 repetition costs was linked to the time elapsed between the response in the preceding trial and the next cue (the response-cue interval). They observed a decrease in n−2 repetition costs with increasing RCI and attributed it to decay of residual task activation, which then produces less competing activation of the recently abandoned task (Gade and Koch, 2005; see Horoufchin et al., 2011, for a discussion of the issue of RCI effects in task switching). Next to decreased task activation, also the dominance of a task has been found to influence the size of n−2 repetition costs. Using a speech production paradigm requiring the naming of visually presented digits, Philipp et al. (2007b) asked their subjects to switch among three languages and found significantly larger n−2 repetition costs for the more dominant language (L1; i.e., German, for German subjects) compared to the other languages assessed in the experiment (see also Arbuthnott, 2008, for a related finding). Furthermore, Grange and Houghton (2010) could show that an increase in intertrial-conflict by the change in cue-task mapping increased n−2 repetition costs. Finally, Philipp and Koch (2006) observed that the introduction of immediate (i.e., n−1) task repetitions lead to reduced n−2 repetition costs. They interpreted their finding according to a balance between activation and inhibition processes ensuring the optimal equilibrium of activation and inhibition in the cognitive system (Philipp and Koch, 2006).
To summarize this brief review, there is evidence that the size of n−2 repetition costs is related to the amount of processing conflict among tasks. For this article, which is about the notion of criticality in living systems, we take the concept of conflict as equivalent to the idea of a critical event, and cognitive conflicts can be suitably explored in multitasking situations, such as in task switching. Therefore, we take changes in processing demands that reduce conflict among tasks (and consequently the size of n−2 repetition costs) as those variables that reduce criticality of the ongoing processing demands. Thus, we use an operational definition of critical events by means of conflict among tasks.
In the present study, we set out to investigate whether inhibitory mechanisms leading to n−2 repetition costs are influenced by stimulus valence. Previous task switching research has shown that stimulus valence is a powerful trigger of conflict among tasks (see Kiesel et al., 2010). We were specifically interested in whether a univalent stimulus modulates the size of n−2 repetition cost. Univalent stimuli are stimuli that call only for one task and thus do not afford task conflict. Stimulus valence (i.e., multivalent vs. univalent) has been rarely assessed when investigating the triggering conditions of task inhibition.
In one of the rare studies, Sdoia and Ferlazzo (2008) had subjects perform three tasks (two numerical judgment tasks and one perceptual color matching short-term memory task) on either univalent or trivalent stimuli (i.e., colored digits, the stimulus material for the numerical judgment tasks). Sdoia and Ferlazzo (2008) found n−2 repetition costs only for trivalent stimuli, while there were no n−2 repetition costs for univalent stimuli (i.e., colored patches). They concluded that overlap on the level of the stimuli could also give rise to n−2 repetition costs. However, please note that in this study, the color matching task (i.e., same or different) was a purely perceptual task and therefore differed from the two numerical judgment tasks in terms of difficulty and memory engagement. This difference in tasks might account for the failure of finding n−2 repetition costs with univalent stimuli in the first place. In a different study, Gade and Koch (2007) were primarily interested in investigating the role of response set overlap (i.e., response valence), but they also used univalent stimuli in 25% of trials. However, like in Sdoia and Ferlazzo’s (2008) study, univalent stimuli where confounded with task identity and this specific task also had a different response set. That is, in both Sdoia and Ferlazzo’s (2008) study and Gade and Koch’s (2007) study, the data on the role of stimulus valence and its relation to n−2 task repetition costs were associated with a confound of task identity with stimulus valence, rendering clear conclusions difficult.
The present study was aimed to systematically examine the role of stimulus valence in task inhibition deconfounded with task identity or response set differences. In Experiment 1, we introduced 25% univalent trials, independent of task identity. That is, any of the three tasks was either performed on a trivalent stimulus (i.e., a stimulus consisting of members of all three stimulus sets, see Figure 1) or on a univalent stimulus that afforded only one task. If stimulus valence leads to less conflict among tasks, then we should see reduced n−2 repetition costs after a univalent stimulus in trial n−1 because univalent stimuli should not call for inhibitory processes to reduce task-set conflict. This assumption is based on the finding that univalent stimuli in two-tasks paradigms lead to less switch costs and shorter RTs, indicating facilitated processing (e.g., Meiran, 2008; Braverman and Meiran, 2010) and that bivalency of stimuli incurs a cost (Woodward et al., 2003).
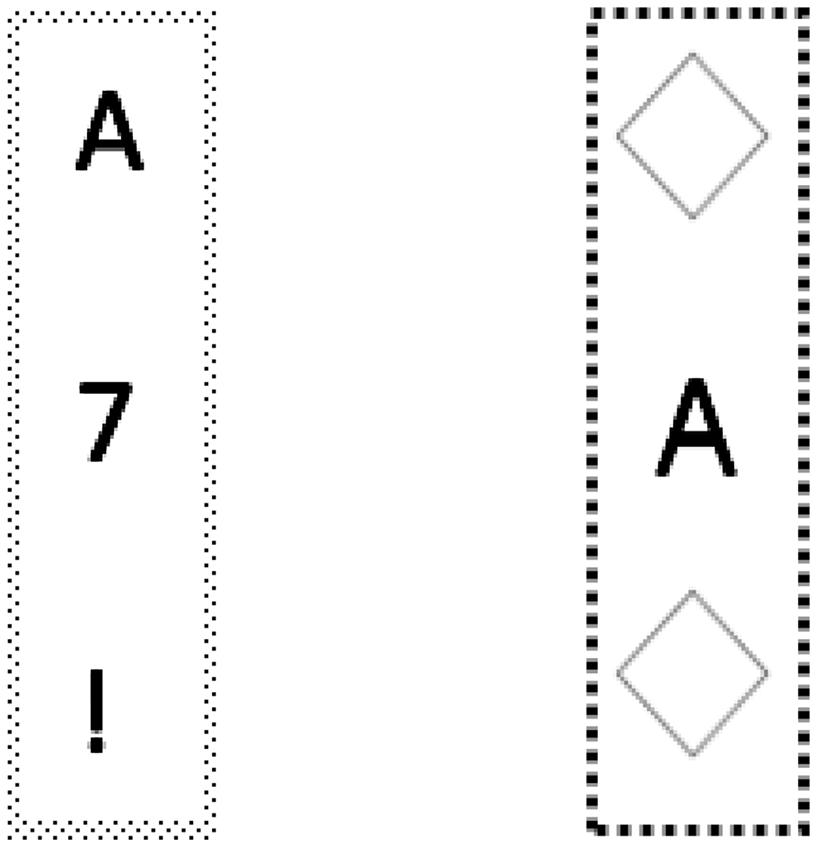
Figure 1. Stimulus display with trivalent stimuli (left) and univalent stimuli (right) in Experiment 1 and 2. Colored frames served as cues. In Experiment 2, the diamonds were replaced by straight lines.
However, to make sure that subjects knew in advance about the less critical, less conflict-inducing events, and thus to further reduce the potential task conflict, each univalent stimulus was indicated by a task-unspecific cue, informing the subject that the next stimulus would be univalent, but not announcing the identity of the upcoming task (which had to be inferred from the univalent stimulus). In all other trials, tasks were indicated by a task-specific cue.
Experiment 1
Method
Subjects
Thirty-two subjects (18 women, mean age 23.8 years) participated in the experiment and were paid 8 Euro.
Stimuli and tasks
Subjects performed a letter judgment (consonant vs. vowel), a digit judgment (odd vs. even), or a symbol judgment (text symbols vs. math symbols), as indicated by a cue. The stimuli were presented in the center of a 15″ color monitor connected to an IBM compatible PC. The stimulus display consisted of all three kinds of stimuli that were arranged in a column, so that subjects had to use the cue to decide which task to perform. The stimulus set for the letter task was (A, E, U, D, P, W). Stimuli for the digit task were (2, 4, 6, 3, 5, 7). For the symbol task, stimuli consisted of a set of symbols (!, ’, ., +, =, <). Stimulus position varied randomly from trial to trial. Cues were colored rectangles surrounding the stimulus display. A blue rectangle indicated the letter task, a green rectangle the digit task, and a yellow one the symbol task. These cues are further referred to as task-specific cues. However, in one fourth of the trials, subjects were presented with a red rectangle, merely indicating a task switch without informing about the task identity in the next trial. This cue is referred to as task-unspecific cue, and the subsequent stimulus display consisted of two diamonds and one stimulus being associated with one of the three tasks (see Figure 1). Because of practical reasons, one half of the subjects used the ALT and ALT GR keys of a German computer keyboard for responding, and the other half of subjects responded on an external keyboard by pressing one of the two horizontally aligned keys with the index finger of the right and left hand. External response keys measured approximately 1.7 cm and were spatially separated by 3.2 cm. Testing took place in a dimly lit room. Viewing distance was about 50 cm.
Procedure
The experiment was run in a single session with one subject at a time and took about 60 min. Written instructions appeared on the screen, and the experiment was also explained orally. Subjects were informed that they were required to perform three different tasks, depending on the cue surrounding the stimulus display. Subjects were instructed to make use of the cue because most stimulus displays would contain all three kinds of stimuli, requiring the information conveyed by the cue. The S-R mappings were explained for all tasks (with the mappings being counterbalanced across subjects) and a card containing these mappings was placed below the monitor. Subjects performed two practice blocks of 10 and 15 trials to get familiar with the cues and the tasks. In a third practice block, consisting of 25 trials, the task-unspecific cues were introduced. Task-unspecific cues were always followed by univalent stimuli. All three tasks occurred equally often within a block, and so did all possible task triplets. Immediate task repetitions were excluded. The task sequence was pseudo-random due to the constraints described above. The stimulus and response sequence was random with the constraint that each stimulus appeared equally often within one block. Direct stimulus repetition did not occur. The instruction emphasized both speed and accuracy. Subjects received feedback when they committed an error.
A trial started with a blank screen for 500 ms. Then the cue appeared, followed by the stimulus display after 500 ms. Stimuli and cue remained on the screen until the response was given. An experimental block of trials consisted of 75 trials. After each block, subjects received feedback about their mean RT and were invited to take a small break before starting with the next block. Altogether, subjects performed nine experimental blocks followed by a post-experimental interview.
Design
To assess whether our manipulation of stimulus valence had the expected impact we compared performance in trial n for univalent and trivalent stimuli. Please note that according to the retroactive nature of task inhibition (Koch et al., 2010), our main analysis focuses on stimulus valence in trial n−1 and task sequence, leading to the two independent variables task sequence (n−2 repetition vs. n−2 switch) and stimulus valence in trial n−1 (univalent vs. trivalent). To accomplish this analysis, we constructed the triplets relevant for data analysis in such a way that both the task in trial n−2 and the task in trial n were performed on trivalent stimuli, whereas univalent stimuli were only allowed to occur in trial n−1. In each block, univalent stimuli, indicated by a task-unspecific cue, were randomly interspersed in one-fourth of the trials. Immediate repetition of trials with univalent stimuli was possible, but these trials were excluded from analysis. The dependent variables were RT and error rate.
Results
Only correct trials that were preceded by at least two correct trials were included in the data analysis. We standardized RTs for each subject separately and regarded RTs larger than z = 3 as outliers (1.6% of otherwise correct trials). The data from one subject were excluded because of an error rate over 15%; however, the overall data pattern did not change when including these data in the analysis.
To make sure that our manipulation of stimulus valence had the expected effect, we examined the effect of stimulus valence (univalent vs. trivalent) in trial n using a paired t-test. Subjects performed 127 ms faster on univalent stimuli compared to trivalent stimuli [1581 ms vs. 1709 ms, t(30) = 5.0, p < 0.05], indicating that our manipulation of stimulus valence was successful. The mean error rate for subjects was 1.5% for univalent trials, whereas it was 4.9% for trivalent trials [t(30) = 10.64, p < 0.05], further corroborating the idea that univalent stimuli induced less processing conflict in the current trial. Based on this empirically established effect of stimulus valence, we now turn to the analysis of the influence of stimulus valence as a triggering condition for task inhibition; that is, we examine performance as a function of stimulus valence in the preceding trial (trial n−1) to see whether it affects the degree of inhibition that we can measure (as an after-effect) as n−2 task repetition costs. RT and percentage error as a function of stimulus valence in trial n−1 and task sequence is shown in Table 1.
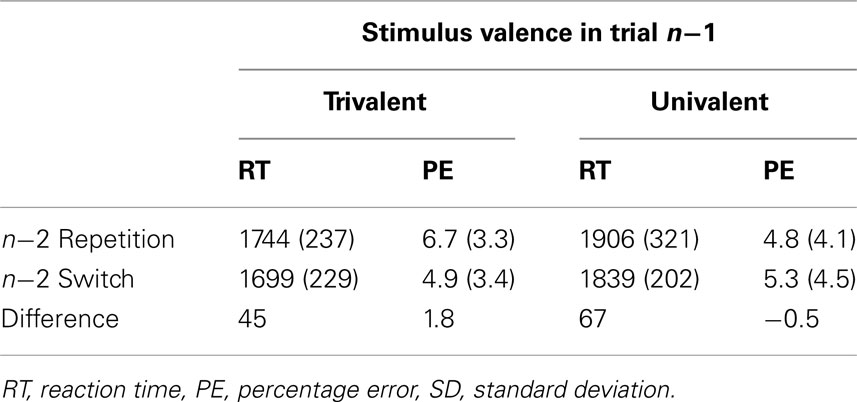
Table 1. RT (SD) and PE (SD) in Experiment 1 for trivalent stimuli in trial n as a function of task sequence (n−2 repetition vs. n−2 switch) and stimulus valence in trial n−1 (trivalent vs. univalent).
An analysis of variance (ANOVA) on RT using stimulus valence in trial n−1 (trivalent vs. univalent) and task sequence (n−2 repetition vs. n−2 switch) as independent variables revealed a main effect of stimulus valence in trial n−1, F(1, 30) = 40.62, p < 0.05, pη2 = 0.58. RT was higher after a trial with a univalent stimulus than after a trial with a trivalent stimulus (1906 vs. 1839 ms), which is probably due to the change from the univalent stimulus display back to the multivalent display (see also Gade and Koch, 2007). Importantly, we also obtained a significant main effect of task sequence, F(1, 30) = 12.97, p < 0.05, pη2 = 0.30, indicating overall n−2 task repetition costs of 56 ms. However, contrary to the expectation based on the idea that univalent stimuli do not call for inhibitory processes to resolve task-set conflict (or do so much less), these n−2 task repetition costs were not at all larger after a trivalent stimuli than after a univalent stimulus but even numerically smaller (45 vs. 67 ms), but this difference was clearly non-significant (F < 1).
The overall error rate was 6.1% (see Table 1). There was no main effect of stimulus valence in trial n−1 or of task sequence (both Fs < 1.8, both ps > 0.20, pη2 < 0.1). Also the interaction effect was non-significant, F(1, 30) = 3.7, p > 0.06, pη2 = 0.11, even though there was in fact a numerical trend toward larger n−2 repetition costs after trivalent stimuli than after univalent stimuli. Note, however, that the absolute number of errors after the univalent stimulus was very small (as was the number of errors for univalent trials in general because these trials were infrequent relative to those trials following a trivalent cue, so that these error data are more variable, rendering the interpretation of error rates in this condition rather inconclusive.
Discussion
In Experiment 1, we found n−2 repetition costs both for trials after a univalent stimulus and trials after a trivalent stimulus. These costs did not differ as a function of stimulus valence, although our manipulation showed reduced RT and error rates for univalent stimuli in the current trial, so that we have empirical evidence for the clear effect of our experimental variation of stimulus valence.
However, one way to account for this lack of an effect of stimulus valence in the preceding trial might be that subjects have adopted a strategy to inhibit the just performed task when the task-unspecific cue came up. Therefore it is difficult to isolate the effect of stimulus valence from cue type (specific vs. unspecific). Experiment 2 allowed us to examine more directly the influence of stimulus valence by disentangling the variation of stimulus valence from the additional introduction of an attention-grabbing task-unspecific cue as used in Experiment 1.
In Experiment 2, we used task-specific cues only and still introduced 25% univalent stimuli randomly in the task sequence. In addition, to assess the degree to which subjects used the task-specific cues, we varied the cue-target interval (CTI). Previous task switching studies have demonstrated that both RT level generally and switch costs particularly are decreased with a long CTI, suggesting a process of active task preparation (see Kiesel et al., 2010, for a review). Hence, in Experiment 2 we could establish empirically whether subjects actively process the cue prior to stimulus onset.
Experiment 2
Method
Subjects
Twenty-four new subjects participated either for course credit or the payment of 15 CHF. Subjects had a mean age of 23.7 years and 21 of them were female.
Stimuli, tasks, and procedure
Stimuli, tasks, and procedure were the same as in Experiment 1, but we omitted the task-unspecific cues. Moreover, to make sure that subjects used the task-specific cues we introduced a CTI manipulation. Subjects performed blocks with long (i.e., 900 ms) CTI and blocks with short (i.e., 100 ms) CTI in alternating order. CTI order was counterbalanced across subjects. The first block was regarded as practice and was not further analyzed. Subjects performed 12 blocks of 75 trials followed by a post-experimental interview. As in Experiment 1, 25% of trials were performed on univalent stimuli.
Design
The independent within-subjects variables were stimulus valence in trial n−1 (trivalent vs. univalent), CTI (100 vs. 900 ms), and task sequence (n−2 repetition vs. n−2 switch). The dependent variables were RT and error rate.
Results
Only correct trials that were preceded by at least two correct trials were included in the data analysis. We standardized RTs for each subject separately and regarded RTs larger than z = 3 as outliers (1.6% of otherwise correct trials). RT and percentage error as a function of stimulus in trial n−1, CTI, and task sequence is shown in Table 2.
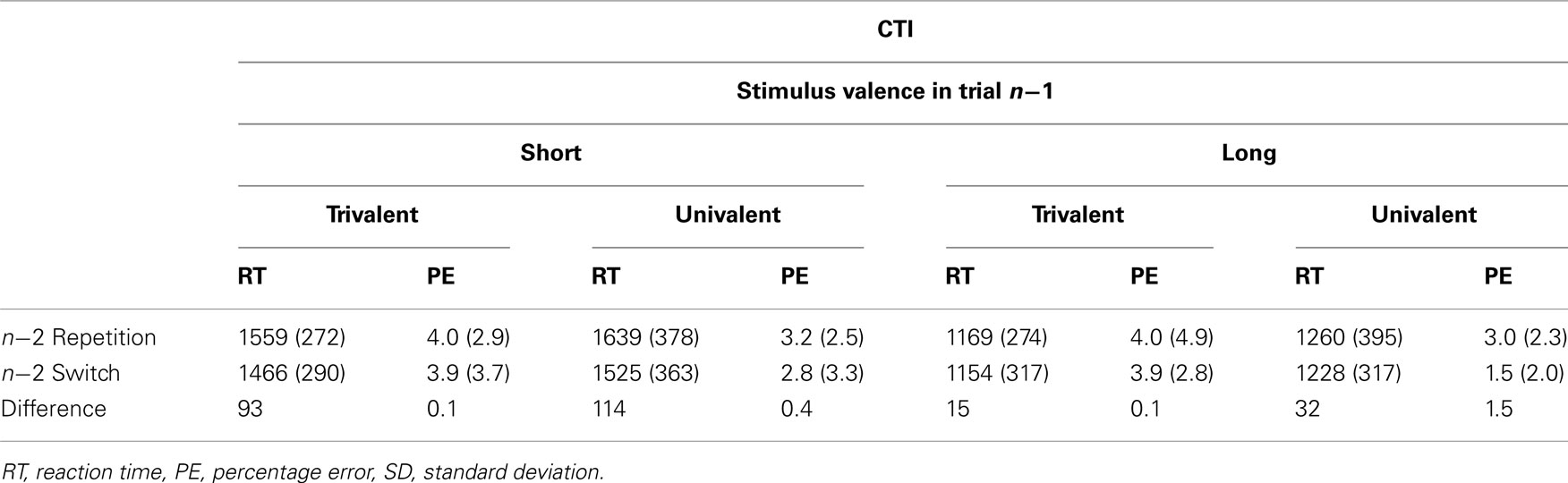
Table 2. RT (SD) and PE (SD) in Experiment 2 for trivalent stimuli in trial n as a function of task sequence (n−2 repetition vs. n−2 switch), stimulus valence in trial n−1 (trivalent vs. univalent), and cue-target interval (short vs. long).
To establish that our manipulation of stimulus valence had the intended effect of reducing task conflict, we analyzed performance in trial n depending on stimulus valence. Subjects were 285 ms faster when the current stimulus was univalent compared to a trivalent stimulus [1082 vs. 1367 ms, t(23) = 8.78, p < 0.05], so again the introduction of univalent stimuli led to faster performance. Accuracy was not affected by stimulus valence (0.6% for both types of trials).
An ANOVA on RT using stimulus valence in trial n−1 (univalent vs. trivalent), CTI (100 vs. 900 ms), and task sequence (n−2 repetition vs. n−2 switch) as within-subjects variables revealed a main effect of task sequence, F(1, 23) = 10.66, p < 0.05, pη2 = 0.31, indicating overall n−2 repetition costs of 64 ms. Stimulus valence in trial n−1 lead to a main effect [F(1, 23) = 12.45, pη2 = 35.1], indicating that subjects were, like in Experiment 1, slower after a univalent stimulus in trial n−1 (1337 vs. 1413 ms, for trivalent and univalent stimuli in trial n−1, respectively). CTI also yielded a main effect, F(1, 23) = 304.30, p < 0.05, pη2 = 0.93, suggesting that subjects engaged in preparation for the upcoming task although this might turn out to be a trial with a univalent stimulus, in which case cue processing is not required because the stimulus affords only one task. Importantly, like in Experiment 1, the interaction of stimulus valence and task sequence was not significant (F < 1). n−2 Repetition costs were 50 ms after trivalent stimuli in trial n−1 [t(23) = 3.9, p < 0.05] and 83 ms after univalent stimuli in trial n−1 [t(23) = 3.9, p < 0.05]. Interestingly, the interaction of task sequence and CTI was significant, F(1, 23) = 7.19, p < 0.05, pη2 = 0.24, indicating a reduction of n−2 repetition cost with long CTI (104 vs. 24 ms, for short vs. long CTI, respectively). The residual n−2 task repetition costs of 24 ms were no longer significant [t(23) = 0.90, p > 0.30]. This preparatory reduction of the inhibition effect occurred regardless of stimulus valence, that is, neither the three-way interaction nor any other interaction was significant (all Fs < 1, see Figure 2).
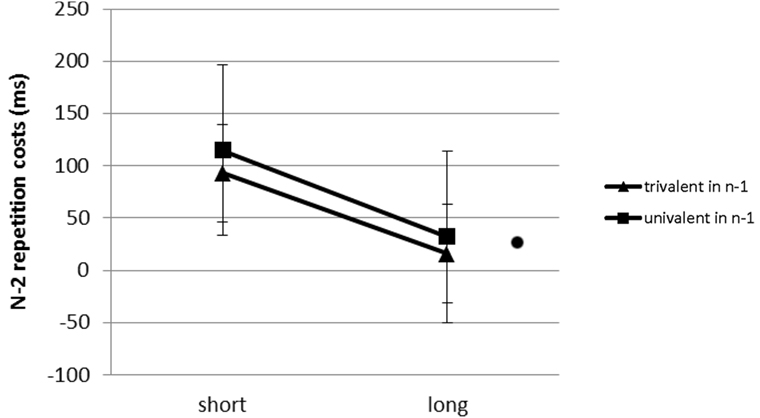
Figure 2. n−2 Repetition cost as a function of stimulus valence in trial n−1 and CTI for Experiment 2. Error bars denote 95% within-subject confidence intervals.
The overall error rate was 3.6% (see Table 2). Only stimulus valence in trial n−1 yielded a significant main effect [F(1, 23) = 12.96, p < 0.05, pη2 = 0.36], indicating more errors after a trivalent stimulus (4%) than after a univalent stimulus (2.7%). (Note that this effect indicates a speed-accuracy tradeoff because RTs were actually significantly shorter after a trivalent stimulus than after a univalent stimulus.) No other significant results were obtained (all ps > 0.15).
Discussion
In Experiment 2, we used only task-specific cues. Our manipulation of stimulus valence showed the intended effect in trial n, so that performance was faster with univalent stimuli, suggesting reduced task conflict (see Braverman and Meiran, 2010, for related results). Importantly, we found n−2 task repetition costs regardless of stimulus valence in the preceding trial (i.e., n−1); again, like in Experiment 1 these were descriptively even somewhat larger after a univalent stimulus. The results of Experiment 2 thus closely replicate those of Experiment 1, suggesting that the finding of similar task inhibition effects after univalent and trivalent stimuli in Experiment 1 was not due to some strategic adaptation based on the task-unspecific warning cues.
Note that the substantial effect of the CTI suggests that subjects actively prepared the upcoming task based on the cues regardless of the fact that the stimulus could have been univalent. However, we also found that prolonged preparation time reduced the n−2 task repetition costs, rendering these costs non-significant with long CTI. The issue of why the introduction of univalent stimuli apparently favored an influence of cue-based preparation on n−2 task repetition costs is discussed in the Section “General Discussion.”
General Discussion
The present study was aimed to examine the degree of processing conflict as a triggering condition for task inhibition in multitasking situations requiring rapid task switching. To this end, we examined the influence of stimulus valence in the preceding trial on the occurrence of n−2 task repetition costs in two experiments. In Experiment 1, we could show that presenting a task-unspecific warning cue followed by a univalent stimulus in trial n−1 did not abolish n−2 repetition costs measured in trial n. In Experiment 2, we examined the impact of univalent stimuli more directly and could rule out that the task-unspecific warning cue, used in Experiment 1, gave rise to the pattern of comparable n−2 repetition costs regardless of stimulus valence in the preceding trial. To summarize our results, the random insertion of 25% of univalent stimuli did not abolish n−2 repetition costs, neither when they occurred with a warning cue nor when they occurred completely unpredictably.
The experimental variation of stimulus valence was based on the notion that task conflict should be small when being faced with univalent stimuli, because such stimuli afford only one task (Woodward et al., 2003). Empirically we could establish this expected effect of stimulus valence. Hence, if the level of conflict in the current trial determines the strength of inhibition of the most competing task (which is usually the immediately preceding, just performed task) that we can measure as n−2 repetition costs, then we should have observed reduced n−2 repetition costs after univalent stimuli as did Sdoia and Ferlazzo (2008). However, this was clearly not the case in two experiments. Both experiments had reasonable statistical power, but the data trend actually at least numerically even opposed our prediction in both experiments. Please note that our study differed from the study of Sdoia and Ferlazzo (2008) and the work of Gade and Koch (2007) in that we did not assign a specific task to univalent stimuli or a specific, different response set, which might provide alternative explanations for the finding obtained by Sdoia and Ferlazzo (2008). In another way, the data therefore support the conclusion drawn by Gade and Koch (2007) in their third experiment, namely critical and a triggering condition for task inhibition is indeed the overlap in response set.
This finding suggests that task inhibition is a powerful means of the cognitive system to deal with critical events, such as conflict among, but it seems to be a very inflexible mechanism that does not respond to the level of conflict induced by the stimulus. Hence, even though we could demonstrate empirically that univalent stimuli lead to less task conflict, it appears that the task conflict that still remains based on overlap of the response sets across tasks (Gade and Koch, 2007) is sufficient to trigger the n−2 task repetition costs in full size, unmodulated by the variation of stimulus valence.
However, univalent stimuli, although not directly influencing the size of n−2 repetition costs, might none the less have altered performance of the ongoing task sequence by indirect means (for instance by a change in task-set shielding; Dreisbach and Wenke, 2011). We found increased RTs after a univalent trial in both experiments, which suggests that the exposition to a univalent stimulus interrupted the routines subjects had developed for solving the task requirements (see Lien et al., 2006, for a related finding in a two-task paradigm). Therefore, although on its own univalent trials might be experienced as easier than multivalent trials, the data suggest that variations in stimulus valence apparently lead to changes in the way people process the encountered tasks.
Adaptation to current processing demands is widely documented in the task switching literature, for example by the impact of stimulus congruency (that is whether or not the two dimensions of a bivalent stimulus afford the same response or different responses) on task switch costs (Goschke, 2000), or vice versa by the influence of switching demands on congruency of otherwise irrelevant stimulus features (Dreisbach and Wenke, 2011). In this context, it is an important finding that in Experiment 2, we found that prolonged preparation time decreased n−2 task repetition costs, which seems to be an adaptive effect, too.
This preparatory reduction of n−2 task repetition costs is a somewhat atypical finding, as the majority of previous studies observed that long preparation time does not significantly reduce the size of n−2 repetition costs (e.g., Mayr and Keele, 2000; Schuch and Koch, 2003; Gade and Koch, 2008; see Grange and Houghton, 2011, for discussion). Yet, two other studies found a similar reduction of n−2 repetition costs with prolonged preparation time. In one study, Philipp et al., 2007b, Experiment 2) assessed n−2 repetition costs when switching among languages, and in a second study Koch et al. (2004) assessed n−2 repetition costs in switching of response mode. It should be noted that these two studies, like the present study, had experimental features that distinguished them from the standard paradigm.
That is, there was a clear dominance relationship among languages in the study by Philipp et al. (2007b), there were two choice-response tasks but one simple-response task in the study by Koch et al. (2004), and there were 25% univalent stimuli in the present study. Please note that preparation time benefits were not solely observed for the altered tasks but were found to affect all three tasks in our present study, as it was in the study of Koch et al. (2004) and of Philipp et al. (2007b).
We think it is likely that the introduction of univalent trials, which fostered adaptations such as a refocusing of attention after a univalent trial (Meiran, 2008), might have also altered the way prolonged preparation time influences the n−2 repetition costs, by rendering variations in preparation time more salient. While we assume that usually n−2 repetition costs are insensitive to prolonged preparation time (Schuch and Koch, 2003, for a more detailed argument), having to adapt to infrequent changes in stimulus valence like in this study, or to different response effectors (Koch et al., 2004), or to strong dominance hierarchies (Philipp et al., 2007b) might encourage subjects to use prolonged preparation time more efficiently (Gade and Koch, 2008; see Grange and Houghton, 2011, for a similar argument). Further research will have to examine the underlying mechanism of this particular preparatory phenomenon in more detail.
In sum, our data suggest the means, for example the inhibition of the last performed task, by which the cognitive system deals with critical events are used in a rather global fashion (i.e., for all trials in a complete block or even experiment) and therefore are inflexible to adapt to rare non-critical events.
Conflict of Interest Statement
The authors declare that the research was conducted in the absence of any commercial or financial relationships that could be construed as a potential conflict of interest.
Acknowledgments
The authors would like to thank Denise Nadine Stephan, Maret Kassner, Katrin Rolffs, and Olivia Schär for help in data collection.
References
Arbuthnott, K. (2008). Asymmetric switch costs and backward inhibition: carryover activation and inhibition in switching between tasks of unequal difficulty. Can. J. Exp. Psychol. 62, 91–100.
Dreisbach, G., and Wenke, D. (2011). The shielding function of task-sets and its relaxation during task switching. J. Exp. Psychol. Learn. Mem. Cogn. 37, 1540–1546.
Gade, M., and Koch, I. (2005). Linking inhibition to activation in the control of task sequences. Psychon. Bull. Rev. 12, 530–534.
Gade, M., and Koch, I. (2007). The influence of overlapping response sets on task inhibition. Mem. Cognit. 35, 603–609.
Gade, M., and Koch, I. (2008). Dissociating cue-related and task-related processes in task inhibition: evidence from using a 2:1 cue-to-task mapping. Can. J. Exp. Psychol. 62, 51–55.
Goschke, T. (2000). “Intentional reconfiguration and involuntary persistence in task-set switching,” in Control of Cognitive Processes: Attention and Performance, Vol. XVIII, eds S. Monsell and J. Driver (Cambridge, MA: MIT Press), 331–355.
Grange, J. A., and Houghton, G. (2010). Heightened conflict in cue-target translation increases backward inhibition in set-switching. J. Exp. Psychol. Learn. Mem. Cogn. 36, 1003–1009.
Grange, J. A., and Houghton, G. (2011). Task preparation and task inhibition: a comment on Koch, Gade, Schuch and Philipp (2010). Psychon. Bull. Rev. 18, 211–216.
Horoufchin, H., Philipp, A. M., and Koch, I. (2011). The dissipating task repetition benefit in cued task switching: task-set decay or temporal distinctiveness? J. Exp. Psychol. Hum. Percept. Perform. 37, 455–472.
Houghton, G., Pritchard, R., and Grange, J. A. (2009). The role of cue-target translation in backward inhibition of attentional set. J. Exp. Psychol. Learn. Mem. Cogn. 35, 466–476.
Kiesel, A., Steinhauser, M., Wendt, M., Falkenstein, M., Jost, K., Philipp, A. M., and Koch, I. (2010). Control and interference in task switching – a review. Psychol. Bull. 136, 849–874.
Koch, I., and Allport, A. (2006). Cue-based preparation and stimulus-based priming of tasks in task switching. Mem. Cognit. 34, 433–444.
Koch, I., Gade, M., and Philipp, A. M. (2004). Inhibition of response mode in task switching. Exp. Psychol. 51, 52–58.
Koch, I., Gade, M., Schuch, S., and Philipp, A. M. (2010). The role of task inhibition in task switching – a review. Psychon. Bull. Rev. 17, 1–14.
Koch, I., Philipp, A. M., and Gade, M. (2006). Chunking in task sequences modulates task inhibition. Psychol. Sci. 17, 346–350.
Lien, M. C., Ruthruff, E., and Kuhns, D. (2006). On the difficulty of task switching: assessing the role of task-set inhibition. Psychon. Bull. Rev. 13, 530–535.
Mayr, U., and Keele, S. W. (2000). Changing internal constraints on action: the role of backward inhibition. J. Exp. Psychol. Gen. 129, 4–26.
Meiran, N. (2008). The dual implication of dual affordance. Stimulus-task binding and attentional focus changing during task preparation. Exp. Psychol. 55, 252–260.
Miller, E. K., and Cohen, J. D. (2001). An integrative theory of prefrontal cortex function. Annu. Rev. Neurosci. 24, 167–202.
Monsell, S. (1996). “Control of mental processes,” in Unsolved Mysteries of the Mind: Tutorial Essays in Cognition, ed. V. Bruce (Hove: Erlbaum), 93–148.
Philipp, A. M., Jolicoeur, P., Falkenstein, M., and Koch, I. (2007a). Response selection and response execution in task switching: evidence from a go-signal paradigm. J. Exp. Psychol. Hum. Percept. Perform. 33, 1062–1075.
Philipp, A. M., Gade, M., and Koch, I. (2007b). Inhibitory processes in language switching? Evidence from switching language-defined response sets. Eur. J. Cogn. Psychol. 19, 395–416.
Philipp, A. M., and Koch, I. (2006). Task inhibition and task repetition in task switching. Eur. J. Cogn. Psychol. 18, 624–639.
Rogers, R. D., and Monsell, S. (1995). Costs of a predictable switch between simple cognitive tasks. J. Exp. Psychol. Gen. 124, 207–231.
Schneider, D. W., and Verbruggen, F. (2008). Inhibition of irrelevant category-response mappings. Q. J. Exp. Psychol. (Hove) 61, 1629–1640.
Schuch, S., and Koch, I. (2003). The role of response selection for inhibition of task-sets in task shifting. J. Exp. Psychol. Hum. Percept. Perform. 29, 92–105.
Sdoia, S., and Ferlazzo, F. (2008). Stimulus-related inhibition of task-set during task switching. Exp. Psychol. 55, 322–327.
Keywords: cognitive control, multitasking, interference
Citation: Gade M and Koch I (2012) Inhibitory processes for critical situations – the role of n−2 task repetition costs in human multitasking situations. Front. Physio. 3:159. doi: 10.3389/fphys.2012.00159
Received: 13 January 2012; Accepted: 07 May 2012;
Published online: 25 May 2012.
Edited by:
Zbigniew R. Struzik, The University of Tokyo, JapanReviewed by:
Boris Podobnik, University of Rijeka, CroatiaFabio Ferlazzo, Sapienza University of Rome, Italy
Copyright: © 2012 Gade and Koch. This is an open-access article distributed under the terms of the Creative Commons Attribution Non Commercial License, which permits non-commercial use, distribution, and reproduction in other forums, provided the original authors and source are credited.
*Correspondence: Miriam Gade, General Psychology (Cognition), Institute of Psychology, University of Zürich, Binzmuehlestr. 14/Box 22, CH-8050 Zürich, Switzerland. e-mail:bS5nYWRlQHBzeWNob2xvZ2llLnV6aC5jaA==