- Division of Gene Therapy and Hepatology, Center for Applied Medical Research (CIMA), School of Medicine, University of Navarra, and Ciberehd, Pamplona, Spain
The Cl−/HCO−3anion exchanger 2 (AE2) is known to be involved in intracellular pH (pHi) regulation and transepithelial acid-base transport. Early studies showed that AE2 gene expression is reduced in liver biopsies and blood mononuclear cells from patients with primary biliary cirrhosis (PBC), a disease characterized by chronic non-suppurative cholangitis associated with antimitochondrial antibodies (AMA) and other autoimmune phenomena. Microfluorimetric analysis of the Cl−/HCO−3 anion exchange (AE) in isolated cholangiocytes showed that the cAMP-stimulated AE activity is diminished in PBC compared to both healthy and diseased controls. More recently, it was found that miR-506 is upregulated in cholangiocytes of PBC patients and that AE2 may be a target of miR-506. Additional evidence for a pathogenic role of AE2 dysregulation in PBC was obtained with Ae2−/−a,b mice, which develop biochemical, histological, and immunologic alterations that resemble PBC (including development of serum AMA). Analysis of HCO−3 transport systems and pHi regulation in cholangiocytes from normal and Ae2−/−a,b mice confirmed that AE2 is the transporter responsible for the Cl−/HCO−3exchange in these cells. On the other hand, both Ae2+/+a,b and Ae2−/−a,b mouse cholangiocytes exhibited a Cl−-independent bicarbonate transport system, essentially a Na+-bicarbonate cotransport (NBC) system, which could contribute to pHi regulation in the absence of AE2.
Introduction
Intracellular pH (pHi) regulation plays a critical role for most cellular processes and functions. Activation by environmental stimuli, DNA synthesis and cell proliferation, apoptosis, oxidative stress and cell metabolism are accompanied by changes in pHi (Gerson et al., 1982; Moolenaar et al., 1983; Burns and Rozengurt, 1984; Lagadic-Gossmann et al., 2004; Mulkey et al., 2004; Cardone et al., 2005). To minimize cytosolic pH disturbances, cells employ not only their intrinsic buffering capacity but have also a variety of ion carriers at the plasma membrane that maintain the pHi within a narrow physiological range (Boron et al., 2009; Casey et al., 2010). These include channels, pumps, exchangers, and cotransporters, all of which orchestrate the input and output of acid/base ions H+ and HCO−3. In this review, the major membrane ion carriers that contribute to the regulation of pHi in cholangiocytes (the biliary epithelial cells lining intrahepatic bile ducts) are described. A particular attention is paid to the anion exchanger 2 (AE2, also Slc4A2), a pH regulatory protein that is highly activated upon increased pHi (Stewart et al., 2001). AE2 is efficiently used by cholangiocytes to execute biliary HCO−3 secretion and its dysfunction is seemingly involved in the pathogenesis of primary biliary cirrhosis (PBC).
Membrane Ion Carriers that Regulate pHi in Cholangiocytes
Cholangiocytes are crucial for modifying the primary bile generated at the canaliculi, as they are capable of secretory and absorptive functions that results in bile fluidization and alkalinization (Tabibian et al., 2013). Under physiological conditions, a major function of cholangiocytes is the biliary secretion of HCO−3 (Banales et al., 2006b). As illustrated in Figure 1, cholangiocytes may accumulate HCO−3 through direct HCO−3 loading from the basolateral membrane and/or by ex-novo HCO−3 generation upon hydration of CO2 and subsequent H+ extrusion (Tabibian et al., 2013). CO2 hydration is catalyzed by carbonic anhydrases (CAs) (Alterio et al., 2012), several isoforms of which are expressed in the biliary tract. The cytosolic carbonic anhydrase type II (CA-II) is highly expressed in cholangiocytes and seems to be the major isoform participating in the ex-novo generation of HCO−3 (Tabibian et al., 2013). Membrane-bound CA-IV and CA-IX were localized in the biliary tract and could also participate in the process (Kivela et al., 2005).
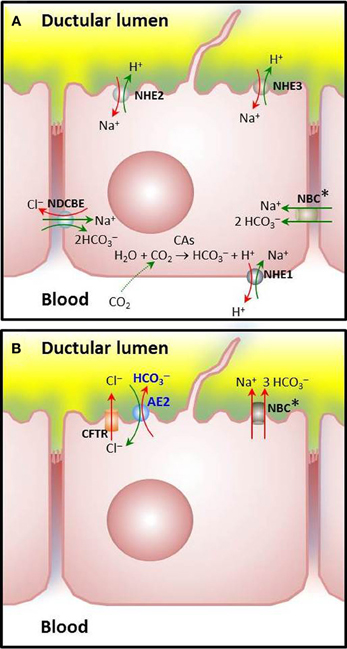
Figure 1. Major ion carriers involved in pHi regulation in cholangiocytes. (A) Acid extruders or HCO−3 loaders: Cells are loaded with HCO−3 via CO2 hydration catalyzed by carbonic anhydrases [CO2(g)+H2O(l) ↔ HCO3−(aq) + H+(aq)] and subsequent H+ extrusion through Na+/H+ exchange, mainly mediated by the basolateral amiloride-sensitive NHE1, that is recognized as a potent acid extruder. Amiloride-insensitive NHE2 and amiloride-sensitive NHE3 may also participate, though these apical exchangers seems to play a major role for NaCl and fluid absorption from the bile duct lumen (Strazzabosco, 1997; Spirlì et al., 1998; Mennone et al., 2001). Additionally, Na+: HCO−3 cotransporters (NBC) with a stoichiometry of 1:2 or a Na+-dependent Cl−/HCO−3 exchanger (NDCBE) may load HCO−3 (in rodent or human cholangiocytes, respectively). (B) Acid loaders: The Na+-independent Cl−/HCO−3 exchanger AE2 is the major acid loader in cholangiocytes. Physiologically, it extrudes HCO−3 in exchange with Cl− once a high outside to inside gradient has been established following stimulation of a variety of apical Cl− channels (the cAMP-activated CFTR and the Ca2+-dependent TMEM16A—illustrated in Figure 2—among other channels pending a complete characterization). Characteristically, mouse cholangiocytes possess an additional capability for acid loading through Na+: HCO−3 cotransport (putatively with a stoichiometry of 1:3) (Uriarte et al., 2010). The biliary epithelial cells have other ion carriers like those for Cl−, Na+, and K+ (not shown) which may contribute, at least indirectly, to pHi regulation and/or HCO−3 secretion. Asterisks are used to indicate that locations for NBC(s) remain to be definitely determined.
One of the mechanisms by which HCO−3 is secreted from cholangiocytes involves activation of Cl− channels and efflux of Cl− followed by its exchange with HCO−3 (Figure 2) (Alvaro et al., 1993; Banales et al., 2006b; Tabibian et al., 2013). But in order to maintain the overall HCO−3-secretory function, cholangiocytes are provided with specific ion membrane carriers like acid loaders and acid extruders (Figure 1) which allow them to maintain ion gradients and pHi (Strazzabosco et al., 1991; Banales et al., 2006b; Tabibian et al., 2013).
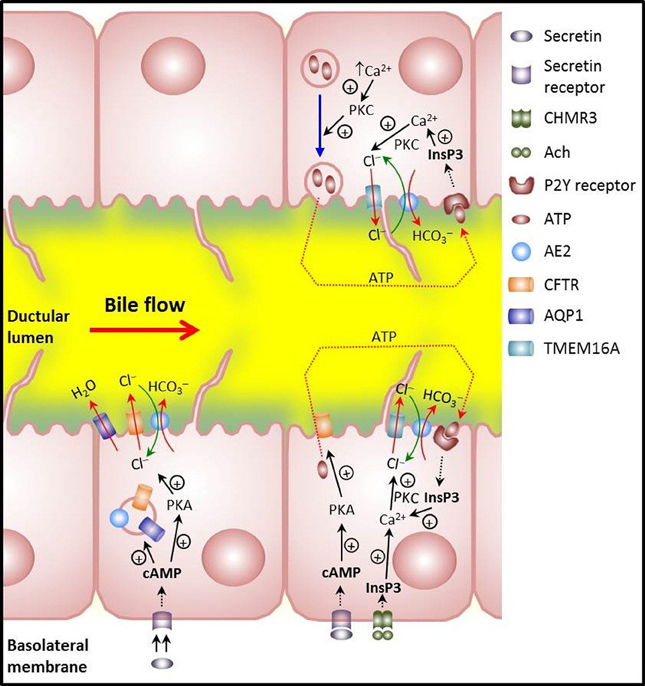
Figure 2. Main mechanisms involved in biliary HCO−3 secretion in cholangiocytes. Lower left: illustrates that the hormone secretin induces trafficking of vesicles with the chloride channel CFTR, the anion exchanger AE2/SLC4A2, and the water channel AQP1. Vesicle exocytosis at the apical membrane allows for bicarbonate-rich hydrocholeresis. Lower right: cholinergic stimulation of basolateral M3 muscarinic receptors increases InsP3 and leads to Ca2+ release. Activation of the apical Ca2+-dependent Cl− channel TMEM16A results in efflux of Cl− which is then exchanged with HCO−3 via AE2. Moreover, CFTR activation that follows secretin stimulation may induce apical release of ATP with further stimulation of apical P2Y receptors, increases in InsP3 and Ca2+, activation of apical Ca2+-dependent Cl− channel TMEM16A, Cl− efflux and final AE2-mediated Cl−/HCO−3 exchanger for apical HCO−3 secretion. Upper right: release of ATP that follows PKC-dependent exocytosis of ATP-enriched intracellular vesicles upon increases in cell volume. Further stimulation of apical P2Y receptors may end up with apical HCO−3 secretion as described for the CFTR-dependent release of ATP.
Acid Extruders in Cholangiocytes
Na+/H+ exchangers (NHEs)
The extrusion of H+ occurs through Na+/H+ exchangers (Donowitz et al., 2013). NHE1-3/SLC9A1-3—the three major isoforms reported in rat cholangiocytes—differ in their functional properties, sensitivity to inhibitors, regulatory mechanisms, and/or membrane polarity (see Figure 1A and references therein). The basolateral NHE1 protects cholangiocytes from intracellular H+ accumulation due to cell metabolism, ex-novo generation of H+ after CO2 hydration, and additional ion transport. Thus, NHE1 plays a crucial role for pHi homeostasis (in combination with diverse HCO−3 transporting systems like Na+- HCO−3 cotransporters and Na+-dependent and Na+-independent Cl−/HCO−3 exchangers), while the apical acid extruders NHE2 and NHE3 seems to play an important role for NaCl and fluid absorption from the bile duct lumen (see also references in Figure 1A).
Na+-bicarbonate cotransporters (NBCs)
The mechanisms leading to increased intracellular concentration of HCO−3 in cholangiocytes (through HCO−3 influx or ex-novo generation) all function as acid extruders. In rodent cholangiocytes HCO−3 influx is known to be mediated by Na+- HCO−3 cotransport (Strazzabosco et al., 1991). This HCO−3-loading function has been demonstrated for an isoform of the electrogenic Na+- HCO−3 cotransporter NBC1 (also referred to as SLC4A4 and NBCe1) expressed in the basolateral membrane of pancreatic ducts. This variant pNBC1 was found to mediate Na+ and HCO−3 influx by coupling the transport of 2 HCO−3 to the downhill flux of Na+, i.e., by operating with a Na+: HCO−3 stoichiometry of 1:2 (Gross and Kurtz, 2002). But the stoichiometry of NBC1 and the direction of the transmembrane Na+- HCO−3 cotransport may vary from one cell type to another (Gross and Kurtz, 2002). Also, it may change in the same cell depending on the intracellular levels of cAMP and PKA-dependent phosphorylation of the COOH terminus, that favor the 1:2 over the 1:3 stoichiometry (Gross and Kurtz, 2002; Pushkin and Kurtz, 2006) and on the intracellular concentration of calcium [Ca2+]i, the increase of which operates in the opposite direction (see below for NBC1 as a potential acid loader).
Na+-driven CL−/HCO−3 exchangers (NDCBEs)
In human cholangiocytes, the Na+- HCO−3 cotransport appears to be inactive at physiological pH, and HCO−3 influx is carried out through electroneutral Na+-dependent Cl−/HCO−3 anion exchange (Strazzabosco et al., 1997), which functions via the uptake of one Na+ and the equivalent of 2 HCO−3, together with the efflux of one Cl− (Romero et al., 2004). SLC4A8 is the only Na+-dependent Cl−/HCO−3 exchanger cloned in humans so far. Although early experiments of Northern blot failed to detect the SLC4A8 mRNA in whole liver (Grichtchenko et al., 2001), SLC4A8 expression cannot be discarded in cholangiocytes which represent only 5% of the liver cell population.
Acid Loaders in Cholangiocytes
The major acid-loading mechanism in cholangiocytes involves an apical electroneutral and Na+-independent Cl−/HCO−3 exchange (Strazzabosco et al., 1991; Spirlì et al., 1998) (Figure 1B). Since the direction of such an exchange is determined by the transmembrane gradient and the outside to inside gradient of Cl− is higher, the exchange normally functions secreting HCO−3 (Banales et al., 2006a,b). Several members of SLC4 and SLC26 families (SLC4A1, SLC4A2, SLC4A3 and SLC26A3, SLC26A4, and SLC26A6) could work in this regard (Pushkin and Kurtz, 2006; Dorwart et al., 2008). However, only SLC4A2 (also AE2) was encountered in cholangiocytes (see Medina, 2011). Moreover, AE2-knockdown experiments in human and rat cholangiocytes indicated that AE2 is the main effector of their Cl−/HCO−3 exchange activity (Banales et al., 2006a, 2012; Arenas et al., 2008). Also, Ae2−/−a,b mouse cholangiocytes, but not wildtype-control cells, lacked Cl−/HCO−3 exchange activity (Uriarte et al., 2010). Characteristically, mouse cholangiocytes exhibited electrogenic NBC activity and NBC1 expression that was increased in Ae2−/−a,b cholangiocytes (Uriarte et al., 2010). Ae2-deficient cells also showed elevated [Ca2+]i, known to favor the 1:3 stoichiometry of NBC1 that promotes an extruding Na+: HCO−3 cotransport (Muller-Berger et al., 2001). These data suggest that overexpressed NBC1 might attempt to replace AE2 for HCO−3 extrusion and intracellular acidification in the knockout cholangiocytes.
Another Na+- HCO−3 cotransporter, referred to as NBC4 (also SLC4A5/NBCe2) was reported to be expressed in cholangiocytes. Similarly to NBC1, NBC4 is electrogenic and may work with 1:3 and 1:2 stoichiometries. Among the six NBC4 splice variants (NBC4a–f) identified in humans, only the NBC4c variant was found to be expressed in rat liver, being basolateral in hepatocytes but apical in cholangiocytes (Abuladze et al., 2004; Pushkin and Kurtz, 2006). In human cholangiocytes, however, the Na+-HCO−3 cotransport mechanism is seemingly inactive at physiological pHi (Strazzabosco et al., 1997; Uriarte et al., 2010) and AE2 represents their major acid-loading mechanism.
Other Ion Transporters in Cholangiocytes
Cholangiocytes are equipped with additional ion transporters like those for Cl−, Na+, and K+, that may contribute at least indirectly, to pHi regulation. At the apical pole, for instance, cholangiocytes express not only the cAMP-responsive Cl− channel CFTR that is activated by secretin (SCT), but also the mechanosensitive and Ca2+-activated Cl−channel TMEM16A and other Ca2+- and/or PKC-dependent Cl− channels (Dutta et al., 2011, 2013). These Ca2+-dependent channels may contribute to Cl− secretion in response to diverse Ca2+-dependent stimuli such as ATP stimulation and cell volume increase. Cl−efflux is important for the outside to inside Cl− gradient that facilitates the electroneutral Cl−/HCO−3 exchange via AE2. Though this exchange partially restores intracellular Cl− levels, the basolateral Na+-K+-2 Cl− cotransporter NKCC1 (also referred to as SLC12A2) can allow for further Cl− influx accompanied by Na+ and K+ (Singh et al., 2001). A basolateral sodium pump Na+/K+ ATPase may achieve the efflux of 3 Na+ by the influx of 2 K+ (Rakowski et al., 1989; Scoazec et al., 1997), while cAMP and/or Ca2+-sensitive basolateral K+ channels can mediate K+ extrusion. The small conductance K+ channel SK2/KCNN2 has been detected in cholangiocytes both apical and basolateral, although functional studies revealed greater basolateral Ca2+-stimulated K+ conductance (Feranchak et al., 2004). The osmotic gradient generated in the bile duct lumen drives secretion of water via aquaporin 1 (AQP1) (Marinelli et al., 1999). Interestingly, AQP1 colocalizes with CFTR and AE2 in subapical vesicles that are exocytosed into the apical membrane upon cAMP-related stimulation (Tietz et al., 2003, 2006), which supports a coordinated contribution of these transporters for the generation of ductal bile flow. Finally, basolateral AQP4 makes possible transcellular water flux for bile fluidization by mediating the import of water from the peribiliary vascular plexus surrounding the bile duct (Marinelli et al., 2000).
The Role of AE2 for Biliary HCO−3 Secretion
Bicarbonate transporters of the SLC26 family that display cAMP- and Ca2+-dependent electroneutral Cl−/HCO−3 exchange (Romero et al., 2004; Garnett et al., 2011; Rode et al., 2011) have been reported to drive HCO−3 secretion in several epithelia. This is the case, for instance, in pancreatic and salivary glands, where those exchangers mediate apical HCO−3 secretion while AE2 is seemingly intended to regulate pHi changes from the basolateral site (Vázquez et al., 1995; Lee et al., 2012). But in the liver there is no evidence for the expression of any of those SLC26 transporters and AE2 appears to be the only operative Cl−/HCO−3 exchanger in the hepatobiliary cells. Compatible with its ability to secrete HCO−3 into bile, AE2 was localized with a monoclonal antibody at the apical domain of both cholangiocytes and hepatocytes (Martínez-Ansó et al., 1994; Medina et al., 1997). On the other hand, and in agreement with previous reports (Alper, 2009), the same antibody localized AE2 at the basolateral domain in choroid plexus (Martínez-Ansó et al., 1994), salivary glands (Vázquez et al., 1995), and kidney (Castillo et al., 2000). The characteristic apical targeting of AE2 in liver cells is further supported by data obtained in different rat models (Tietz et al., 2003; Aranda et al., 2004; Banales et al., 2008; Úriz et al., 2011).
In addition to the aforementioned AE2-knockdown experiments in human and rat cholangiocytes and assessments in Ae2-knockout mouse cholangiocytes indicating that AE2 is by far the major Cl−/HCO−3 exchanger in these biliary cells (see references above), in situ hybridization in human liver further confirmed that the AE2 gene is extensively expressed in cholangiocytes (García et al., 1998). Thus, AE2 is currently regarded as the Cl−/HCO−3 exchanger cholangiocytes have not only for pHi regulation but also as a key HCO−3 extruder (in close interaction with CFTR and other Cl− channels), for HCO−3 secretion to bile.
Biliary HCO−3 secretion is tightly regulated by local factors such as bile salts and purinergic agonists, particularly the potent secretagogue adenosine triphosphate (ATP) and by visceral neurohormonal factors including cholinergic and adrenergic agents, vasoactive intestinal peptide (VIP), glucagon, glucagon-like peptide-1, somatostatin and, above all, SCT (Alvaro et al., 2007; Beuers et al., 2010). In the case of SCT (see Figure 2), the interaction of the hormone with its receptor SCTR at the basolateral membrane of cholangiocytes results in functional stimulation of the SCT/SCTR/CFTR/Cl−/HCO−3—AE2 system (Úriz et al., 2011; Afroze et al., 2013) by increasing cAMP levels and protein kinase A activation (Alvaro et al., 1997b). Subsequent mobilization of AE2/CFTR/AQP1-containing intracellular vesicles toward the apical membrane is followed by vesicle endocytosis (Tietz et al., 2003) (Figure 2). Accompanying phosphorylation and activation of CFTR leads to apical efflux of Cl− that is ultimately exchanged with HCO−3 through AE2 (Alvaro et al., 1997b; Banales et al., 2006a,b). Moreover, increased cAMP levels can stimulate the AE activity in cholangicytes (Spirlì et al., 1998; Zsembery et al., 1998).
The choleretic effect of an increase in the levels of cAMP may be enhanced through a CFTR-associated release of ATP from cholangiocytes into bile (Minagawa et al., 2007) and autocrine/paracrine stimulation of apical purinergic P2Y receptors. The resultant increase in intracellular Ca2+ can activate the apical Ca2+-dependent Cl− channel TMEM16A (Dutta et al., 2011), promoting additional efflux of Cl− that will be exchanged with HCO−3 through AE2. Ursodeoxycholic acid (UDCA) is also able to stimulate CFTR-associated biliary ATP release leading to purinergic stimulation, [Ca2+]i increase and PKC activation, Cl− efflux and AE2-mediated exchange with HCO−3 (Fiorotto et al., 2007). Moreover, ATP may be released upon exocytosis of ATP-enriched intracellular vesicles in response to increases in cell volume in a PKC-dependent manner (Sathe et al., 2011). As mentioned above for the CFTR-associated ATP release, this type of purinergic stimulation—seemingly mediated by the vesicular nucleotide transporter SLC17A9—is expected to result in further Cl− efflux through TMEM16A. Whether the SLC17A9-dependent exocytosis of ATP-enriched intracellular vesicles and the CFTR-associated ATP release are closely related remains yet to be determined.
Acetylcholine and cholinergic stimulation may further assist biliary HCO−3 secretion through Ca2+-dependent Cl− efflux from cholangiocytes, thus potentiating the effect of SCT on both intracellular cAMP levels and Cl−/HCO−3 exchange in a calcineurin-dependent manner (Alvaro et al., 1997a; Minagawa et al., 2007).
All these data indicate the existence of a close mechanistic interplay between the cAMP/PKA and [Ca2+]i/PKC pathways when potent secretagogues exert their choleretic effect. A common mechanistic endpoint appears to be an increase in biliary Cl− efflux, and the fact that such an increase leads to augmented biliary HCO−3 secretion indicates that AE2-mediated Cl−/HCO−3 exchange is critical for the enhanced choleresis to occur.
AE2 interactions with cytosolic and/or membrane bound CAs, similar to those described for cells other than biliary cells (Sterling et al., 2001, 2002; Morgan et al., 2007), may also contribute to AE2-mediated biliary HCO−3 secretion.
How AE2 Deficiency may Lead to PBC?
PBC is a cholestatic liver disease of unknown etiopathogenesis which affects mainly middle-aged women and concurs with characteristic autoimmune phenomena (Hohenester et al., 2009; Poupon, 2010). In the early 90s we hypothesized that PBC pathogenesis could be related to alterations in the mechanisms of HCO−3 transport because: (i) most PBC patients improve the clinical course of the disease by continued treatment with UDCA (Corpechot et al., 2005); and (ii) the hydrophilic bile acid UDCA is known to produce a HCO−3-rich hydrocholeresis [see Medina (2011), for a review]. Interestingly, we could find reduced expression of AE2 in liver biopsies and peripheral blood mononuclear cells from patients with PBC (Prieto et al., 1993; Medina et al., 1997). Also, human cholangiocytes isolated from PBC patients showed a decreased response of the AE activity to cAMP stimulation (Melero et al., 2002), and more recent findings pointed to microRNA-506 being upregulated in cholangiocytes from PBC patients (Banales et al., 2012). This microRNA may bind to the 3'UTR region of AE2 mRNA and prevent protein translation leading to diminished AE2 activity (Banales et al., 2012). Additionally, many immunologic and hepatobiliary alterations characteristic of PBC are eventually reproduced in Ae2−/−a,b mice which indeed develop both immunologic and hepatobiliary PBC-like alterations (Salas et al., 2008). Interestingly, cholangiocytes isolated from these Ae2−/−a,b mice show no increase in resting pHi despite the AE2 deficiency, most probably because of their ability to upregulate the aforementioned NBC activity with acidifying potential (Uriarte et al., 2010). This acidifying cotransport activity is absent in human cholangiocytes (Arenas et al., 2008). Complete deficiency of AE2 would therefore be expected to result in intracellular alkalinization, but most PBC patients show diminished (rather than absent) AE2 expression (Medina et al., 1997), and residual AE2 activity may allow them to maintain normal resting pHi (Melero et al., 2002). In this regard, it can be assumed that upon situations of stimulated hydroionic transport, pHi homeostasis might undergo parallel abnormalities in human cholangiocytes from PBC patients and mouse cholangiocytes from Ae2−/−a,b mice.
Recently, Beuers et al. postulated a new and attractive hypothesis that may explain how AE2 deficiency could contribute to the pathogenesis of PBC and other human cholangiopathies. This hypothesis proposes that biliary epithelial cells develop a HCO−3 umbrella at their luminal membrane to protect themselves against bile-salt induced injury (Beuers et al., 2010). By maintaining an alkaline environment around the luminal membrane of cholangiocytes, the protonation of apolar hydrophobic bile salt monomers could be prevented, rendering those monomers unable to permeate membranes in an uncontrolled fashion and avoiding toxic effects on cholangiocytes. In a series of elegant experiments, the authors then demonstrated that an intact glycocalix at the apical membrane of cholangiocytes and adequate AE2 expression are crucial to maintain the biliary HCO−3 umbrella (Hohenester et al., 2012). Therefore, it appears that cholangiocytes use AE2 as a highly beneficial two-edged sword which allows them to fulfill: (i) the direct control of the pHi preventing, for instance, that any ATP-stimulated increase in NHE activity (Zsembery et al., 1998; Melero et al., 2002) leads to harmful intracellular alkalinization; and (ii) the immediate generation of the apical alkaline umbrella, preventing highly concentrated hydrophobic bile salt monomers to enter the cell.
In the case of PBC, dysfunctional lymphocytes play a crucial role in the pathogenesis of the disease. Of note, our Ae2−/−a,b mouse model (Salas et al., 2008) supports the view that these immune cells require AE2 for controlling their protective surveillance in a way that tolerance is preserved and autoimmunity does not come out. Peripheral blood mononuclear cells from PBC patients exhibit a decrease in AE2 (Prieto et al., 1993), and therefore the risk for a break of tolerance may be increased. In summary, diminished AE2 activity in cholangiocytes from PBC patients may lead to cell injury that makes them more provoking to the immune system. Additionally, diminished AE2 activity in PBC lymphocytes may contribute to these cells being more aggressive toward the provoking cholangiocytes, resulting in profound damage of the biliary tree.
Conflict of Interest Statement
The authors declare that the research was conducted in the absence of any commercial or financial relationships that could be construed as a potential conflict of interest.
Acknowledgments
Additionally, the authors acknowledge the support provided through the Spanish “UTE for CIMA project”, the Spanish Ministry of Science and Innovation (grant No. SAF20012-35455), and the Carlos III Institute of Health (Ciberehd CB06/04/0067). Axel R. Concepcion had financial support from the Subprogram “Torres Quevedo” (Spanish Ministry of Science and Innovation and the European Union, PTQ-10-04247).
References
Abuladze, N., Pushkin, A., Tatishchev, S., Newman, D., Sassani, P., and Kurtz, I. (2004). Expression and localization of rat NBC4c in liver and renal uroepithelium. Am. J. Physiol. Cell Physiol. 287, C781–C789. doi: 10.1152/ajpcell.00590.2003
Afroze, S., Meng, F., Jensen, K., McDaniel, K., Rahal, K., Onori, P., et al. (2013). The physiological roles of secretin and its receptor. Ann. Transl. Med. 1, 29. doi: 10.3978/j.issn.2305-5839.2012.12.01
Alper, S. L. (2009). Molecular physiology and genetics of Na+-independent SLC4 anion exchangers. J. Exp. Biol. 212, 1672–1683. doi: 10.1242/jeb.029454
Alterio, V., di Fiore, A., D'Ambrosio, K., Supuran, C. T., and de Simone, G. (2012). Multiple binding modes of inhibitors to carbonic anhydrases: how to design specific drugs targeting 15 different isoforms? Chem. Rev. 112, 4421–4468. doi: 10.1021/cr200176r
Alvaro, D., Alpini, G., Jezequel, A. M., Bassotti, C., Francia, C., Fraioli, F., et al. (1997a). Role and mechanisms of action of acetylcholine in the regulation of rat cholangiocyte secretory functions. J. Clin. Invest. 100, 1349–1362. doi: 10.1172/JCI119655
Alvaro, D., Mennone, A., and Boyer, J. L. (1997b). Role of kinases and phosphatases in the regulation of fluid secretion and Cl−/HCO−3 exchange in cholangiocytes. Am. J. Physiol. 273, G303–G313.
Alvaro, D., Cho, W. K., Mennone, A., and Boyer, J. L. (1993). Effect of secretion on intracellular pH regulation in isolated rat bile duct epithelial cells. J. Clin. Invest. 92, 1314–1325. doi: 10.1172/JCI116705
Alvaro, D., Mancino, M. G., Glaser, S., Gaudio, E., Marzioni, M., Francis, H., et al. (2007). Proliferating cholangiocytes: a neuroendocrine compartment in the diseased liver. Gastroenterology 132, 415–431. doi: 10.1053/j.gastro.2006.07.023
Aranda, V., Martínez, I., Melero, S., Lecanda, J., Banales, J. M., Prieto, J., et al. (2004). Shared apical sorting of anion exchanger isoforms AE2a, AE2b1, and AE2b2 in primary hepatocytes. Biochem. Biophys. Res. Commun. 319, 1040–1046. doi: 10.1016/j.bbrc.2004.05.080
Arenas, F., Hervías, I., Úriz, M., Joplin, R., Prieto, J., and Medina, J. F. (2008). Combination of ursodeoxycholic acid and glucocorticoids upregulates the AE2 alternate promoter in human liver cells. J. Clin. Invest. 118, 695–709. doi: 10.1172/JCI33156
Banales, J. M., Arenas, F., Rodríguez-Ortigosa, C. M., Sáez, E., Uriarte, I., Doctor, R. B., et al. (2006a). Bicarbonate-rich choleresis induced by secretin in normal rat is taurocholate-dependent and involves AE2 anion exchanger. Hepatology 43, 266–275. doi: 10.1002/hep.21042
Banales, J. M., Prieto, J., and Medina, J. F. (2006b). Cholangiocyte anion exchange and biliary bicarbonate excretion. World J. Gastroenterol. 12, 3496–3511. doi: 10.3748/wjg.v12.i22.3496
Banales, J. M., Masyuk, T. V., Bogert, P. S., Huang, B. Q., Gradilone, S. A., Lee, S. O., et al. (2008). Hepatic cystogenesis is associated with abnormal expression and location of ion transporters and water channels in an animal model of autosomal recessive polycystic kidney disease. Am. J. Pathol. 173, 1637–1646. doi: 10.2353/ajpath.2008.080125
Banales, J. M., Sáez, E., Úriz, M., Sarvide, S., Urribarri, A. D., Splinter, P., et al. (2012). Upregulation of mir-506 leads to decreased AE2 expression in biliary epithelium of patients with primary biliary cirrhosis. Hepatology 56, 687–697. doi: 10.1002/hep.25691
Beuers, U., Hohenester, S., De Buy Wenniger, L. J., Kremer, A. E., Jansen, P. L., and Elferink, R. P. (2010). The biliary HCO−3 umbrella: a unifying hypothesis on pathogenetic and therapeutic aspects of fibrosing cholangiopathies. Hepatology 52, 1489–1496. doi: 10.1002/hep.23810
Boron, W. F., Chen, L., and Parker, M. D. (2009). Modular structure of sodium-coupled bicarbonate transporters. J. Exp. Biol. 212, 1697–1706. doi: 10.1242/jeb.028563
Burns, C. P., and Rozengurt, E. (1984). Extracellular Na+ and initiation of DNA synthesis: role of intracellular pH and K+. J. Cell Biol. 98, 1082–1089. doi: 10.1083/jcb.98.3.1082
Cardone, R. A., Casavola, V., and Reshkin, S. J. (2005). The role of disturbed pH dynamics and the Na+/H+ exchanger in metastasis. Nat. Rev. Cancer 5, 786–795. doi: 10.1038/nrc1713
Casey, J. R., Grinstein, S., and Orlowski, J. (2010). Sensors and regulators of intracellular pH. Nat. Rev. Mol. Cell Biol. 11, 50–61. doi: 10.1038/nrm2820
Castillo, J. E., Martínez-Ansó, E., Malumbres, R., De Alava, E., García, C., Medina, J. F., et al. (2000). In situ localization of anion exchanger-2 in the human kidney. Cell Tissue Res. 299, 281–287. doi: 10.1007/s004410050026
Corpechot, C., Carrat, F., Bahr, A., Chretien, Y., Poupon, R. E., and Poupon, R. (2005). The effect of ursodeoxycholic acid therapy on the natural course of primary biliary cirrhosis. Gastroenterology 128, 297–303. doi: 10.1053/j.gastro.2004.11.009
Donowitz, M., Ming Tse, C., and Fuster, D. (2013). SLC9/NHE gene family, a plasma membrane and organellar family of Na+/H+ exchangers. Mol. Aspects Med. 34, 236–251. doi: 10.1016/j.mam.2012.05.001
Dorwart, M. R., Shcheynikov, N., Yang, D., and Muallem, S. (2008). The solute carrier 26 family of proteins in epithelial ion transport. Physiology (Bethesda) 23, 104–114. doi: 10.1152/physiol.00037.2007
Dutta, A. K., Khimji, A. K., Kresge, C., Bugde, A., Dougherty, M., Esser, V., et al. (2011). Identification and functional characterization of TMEM16A, a Ca2+-activated Cl− channel activated by extracellular nucleotides, in biliary epithelium. J. Biol. Chem. 286, 766–776. doi: 10.1074/jbc.M110.164970
Dutta, A. K., Woo, K., Khimji, A. K., Kresge, C., and Feranchak, A. P. (2013). Mechanosensitive Cl− secretion in biliary epithelium mediated through TMEM16A. Am. J. Physiol. Gastrointest. Liver Physiol. 304, G87–G98. doi: 10.1152/ajpgi.00154.2012
Feranchak, A. P., Doctor, R. B., Troetsch, M., Brookman, K., Johnson, S. M., and Fitz, J. G. (2004). Calcium-dependent regulation of secretion in biliary epithelial cells: the role of apamin-sensitive SK channels. Gastroenterology 127, 903–913. doi: 10.1053/j.gastro.2004.06.047
Fiorotto, R., Spirli, C., Fabris, L., Cadamuro, M., Okolicsanyi, L., and Strazzabosco, M. (2007). Ursodeoxycholic acid stimulates cholangiocyte fluid secretion in mice via CFTR-dependent ATP secretion. Gastroenterology 133, 1603–1613. doi: 10.1053/j.gastro.2007.08.071
García, C., Montuenga, L. M., Medina, J. F., and Prieto, J. (1998). In situ detection of AE2 anion-exchanger mRNA in the human liver. Cell Tissue Res. 291, 481–488. doi: 10.1007/s004410051017
Garnett, J. P., Hickman, E., Burrows, R., Hegyi, P., Tiszlavicz, L., Cuthbert, A. W., et al. (2011). Novel role for pendrin in orchestrating bicarbonate secretion in cystic fibrosis transmembrane conductance regulator (CFTR)-expressing airway serous cells. J. Biol. Chem. 286, 41069–41082. doi: 10.1074/jbc.M111.266734
Gerson, D. F., Kiefer, H., and Eufe, W. (1982). Intracellular pH of mitogen-stimulated lymphocytes. Science 216, 1009–1010. doi: 10.1126/science.6281887
Grichtchenko, I. I., Choi, I., Zhong, X., Bray-Ward, P., Russell, J. M., and Boron, W. F. (2001). Cloning, characterization, and chromosomal mapping of a human electroneutral Na+-driven Cl−-HCO−3 exchanger. J. Biol. Chem. 276, 8358–8363. doi: 10.1074/jbc.C000716200
Gross, E., and Kurtz, I. (2002). Structural determinants and significance of regulation of electrogenic Na+-HCO−3 cotransporter stoichiometry. Am. J. Physiol. Renal Physiol. 283, F876–F887. doi: 10.1152/ajprenal.00148.2002
Hohenester, S., Oude Elferink, R. P. J., and Beuers, U. (2009). Primary biliary cirrhosis. Semin. Immunopathol. 31, 283–307. doi: 10.1007/s00281-009-0164-5
Hohenester, S., Wenniger, L. M., Paulusma, C. C., Van Vliet, S. J., Jefferson, D. M., Elferink, R. P., et al. (2012). A biliary HCO−3 umbrella constitutes a protective mechanism against bile acid-induced injury in human cholangiocytes. Hepatology 55, 173–183. doi: 10.1002/hep.24691
Kivela, A. J., Kivela, J., Saarnio, J., and Parkkila, S. (2005). Carbonic anhydrases in normal gastrointestinal tract and gastrointestinal tumours. World J. Gastroenterol. 11, 155–163.
Lagadic-Gossmann, D., Huc, L., and Lecureur, V. (2004). Alterations of intracellular pH homeostasis in apoptosis: origins and roles. Cell Death Differ. 11, 953–961. doi: 10.1038/sj.cdd.4401466
Lee, M. G., Ohana, E., Park, H. W., Yang, D., and Muallem, S. (2012). Molecular mechanism of pancreatic and salivary gland fluid and HCO−3 secretion. Physiol. Rev. 92, 39–74. doi: 10.1152/physrev.00011.2011
Marinelli, R. A., Pham, L. D., Tietz, P. S., and Larusso, N. F. (2000). Expression of aquaporin-4 water channels in rat cholangiocytes. Hepatology 31, 1313–1317. doi: 10.1053/jhep.2000.7986
Marinelli, R. A., Tietz, P. S., Pham, L. D., Rueckert, L., Agre, P., and Larusso, N. F. (1999). Secretin induces the apical insertion of aquaporin-1 water channels in rat cholangiocytes. Am. J. Physiol. 276, G280–G286.
Martínez-Ansó, E., Castillo, J. E., Díez, J., Medina, J. F., and Prieto, J. (1994). Immunohistochemical detection of chloride/bicarbonate anion exchangers in human liver. Hepatology 19, 1400–1406. doi: 10.1002/hep.1840190613
Medina, J. F. (2011). Role of the anion exchanger 2 in the pathogenesis and treatment of primary biliary cirrhosis. Dig. Dis. 29, 103–112. doi: 10.1159/000324144
Medina, J. F., Martínez-Ansó, E., Vázquez, J. J., and Prieto, J. (1997). Decreased anion exchanger 2 immunoreactivity in the liver of patients with primary biliary cirrhosis. Hepatology 25, 12–17. doi: 10.1002/hep.510250104
Melero, S., Spirli, C., Zsembery, A., Medina, J. F., Joplin, R. E., Duner, E., et al. (2002). Defective regulation of cholangiocyte Cl−/HCO−3 and Na+/H+ exchanger activities in primary biliary cirrhosis. Hepatology 35, 1513–1521. doi: 10.1053/jhep.2002.33634
Mennone, A., Biemesderfer, D., Negoianu, D., Yang, C. L., Abbiati, T., Schultheis, P. J., et al. (2001). Role of sodium/hydrogen exchanger isoform NHE3 in fluid secretion and absorption in mouse and rat cholangiocytes. Am. J. Physiol. Gastrointest. Liver Physiol. 280, G247–G254.
Minagawa, N., Nagata, J., Shibao, K., Masyuk, A. I., Gomes, D. A., Rodrigues, M. A., et al. (2007). Cyclic AMP regulates bicarbonate secretion in cholangiocytes through release of ATP into bile. Gastroenterology 133, 1592–1602. doi: 10.1053/j.gastro.2007.08.020
Moolenaar, W. H., Tsien, R. Y., Van Der Saag, P. T., and De Laat, S. W. (1983). Na+/H+ exchange and cytoplasmic pH in the action of growth factors in human fibroblasts. Nature 304, 645–648. doi: 10.1038/304645a0
Morgan, P. E., Pastorekova, S., Stuart-Tilley, A. K., Alper, S. L., and Casey, J. R. (2007). Interactions of transmembrane carbonic anhydrase, CAIX, with bicarbonate transporters. Am. J. Physiol. Cell Physiol. 293, C738–C748. doi: 10.1152/ajpcell.00157.2007
Mulkey, D. K., Henderson, R. A., 3rd, Ritucci, N. A., Putnam, R. W., and Dean, J. B. (2004). Oxidative stress decreases pHi and Na+/H+ exchange and increases excitability of solitary complex neurons from rat brain slices. Am. J. Physiol. Cell Physiol. 286, C940–C951. doi: 10.1152/ajpcell.00323.2003
Muller-Berger, S., Ducoudret, O., Diakov, A., and Fromter, E. (2001). The renal Na+-HCO−3 cotransporter expressed in Xenopus laevis oocytes: change in stoichiometry in response to elevation of cytosolic Ca2+ concentration. Pflugers Arch. 442, 718–728. doi: 10.1007/s004240100592
Poupon, R. (2010). Primary biliary cirrhosis: a 2010 update. J. Hepatol. 52, 745–758. doi: 10.1016/j.jhep.2009.11.027
Prieto, J., Qian, C., García, N., Díez, J., and Medina, J. F. (1993). Abnormal expression of anion exchanger genes in primary biliary cirrhosis. Gastroenterology 105, 572–578.
Pushkin, A., and Kurtz, I. (2006). SLC4 base (HCO−3, CO2−3) transporters: classification, function, structure, genetic diseases, and knockout models. Am. J. Physiol. Renal Physiol. 290, F580–F599. doi: 10.1152/ajprenal.00252.2005
Rakowski, R. F., Gadsby, D. C., and De Weer, P. (1989). Stoichiometry and voltage dependence of the sodium pump in voltage-clamped, internally dialyzed squid giant axon. J. Gen. Physiol. 93, 903–941. doi: 10.1085/jgp.93.5.903
Rode, B., Dirami, T., Bakouh, N., Rizk-Rabin, M., Norez, C., Lhuillier, P., et al. (2011). The testis anion transporter TAT1 (SLC26A8) physically and functionally interacts with the cystic fibrosis transmembrane conductance regulator channel: a potential role during sperm capacitation. Hum. Mol. Genet. 21, 1287–1298. doi: 10.1093/hmg/ddr558
Romero, M. F., Fulton, C. M., and Boron, W. F. (2004). The SLC4 family of HCO−3 transporters. Pflugers Arch. 447, 495–509. doi: 10.1007/s00424-003-1180-2
Salas, J. T., Banales, J. M., Sarvide, S., Recalde, S., Ferrer, A., Uriarte, I., et al. (2008). Ae2a,b-deficient mice develop antimitochondrial antibodies and other features resembling primary biliary cirrhosis. Gastroenterology 134, 1482–1493. doi: 10.1053/j.gastro.2008.02.020
Sathe, M. N., Woo, K., Kresge, C., Bugde, A., Luby-Phelps, K., Lewis, M. A., et al. (2011). Regulation of purinergic signaling in biliary epithelial cells by exocytosis of SLC17A9-dependent ATP-enriched vesicles. J. Biol. Chem. 286, 25363–25376. doi: 10.1074/jbc.M111.232868
Scoazec, J. Y., Bringuier, A. F., Medina, J. F., Martínez-Ansó, E., Veissiere, D., Feldmann, G., et al. (1997). The plasma membrane polarity of human biliary epithelial cells: in situ immunohistochemical analysis and functional implications. J. Hepatol. 26, 543–553. doi: 10.1016/S0168-8278(97)80419-9
Singh, S. K., Mennone, A., Gigliozzi, A., Fraioli, F., and Boyer, J. L. (2001). Cl−-dependent secretory mechanisms in isolated rat bile duct epithelial units. Am. J. Physiol. Gastrointest. Liver Physiol. 281, G438–G446.
Spirlì, C., Granato, A., Zsembery, K., Anglani, F., Okolicsanyi, L., Larusso, N. F., et al. (1998). Functional polarity of Na+/H+ and Cl−/HCO−3 exchangers in a rat cholangiocyte cell line. Am. J. Physiol. 275, G1236–G1245.
Sterling, D., Alvarez, B. V., and Casey, J. R. (2002). The extracellular component of a transport metabolon. extracellular loop 4 of the human AE1 Cl−/HCO−3 exchanger binds carbonic anhydrase IV. J. Biol. Chem. 277, 25239–25246. doi: 10.1074/jbc.M202562200
Sterling, D., Reithmeier, R. A., and Casey, J. R. (2001). A transport metabolon. Functional interaction of carbonic anhydrase II and chloride/bicarbonate exchangers. J. Biol. Chem. 276, 47886–47894. doi: 10.1074/jbc.M105959200
Stewart, A. K., Chernova, M. N., Kunes, Y. Z., and Alper, S. L. (2001). Regulation of AE2 anion exchanger by intracellular pH: critical regions of the NH2-terminal cytoplasmic domain. Am. J. Physiol. Cell Physiol. 281, C1344–C1354.
Strazzabosco, M. (1997). Transport systems in cholangiocytes: their role in bile formation and cholestasis. Yale J. Biol. Med. 70, 427–434.
Strazzabosco, M., Joplin, R., Zsembery, A., Wallace, L., Spirli, C., Fabris, L., et al. (1997). Na+-dependent and -independent Cl−/HCO−3 exchange mediate cellular HCO−3 transport in cultured human intrahepatic bile duct cells. Hepatology 25, 976–985. doi: 10.1002/hep.510250431
Strazzabosco, M., Mennone, A., and Boyer, J. L. (1991). Intracellular pH regulation in isolated rat bile duct epithelial cells. J. Clin. Invest. 87, 1503–1512. doi: 10.1172/JCI115160
Tabibian, J. H., Masyuk, A. I., Masyuk, T. V., O'hara, S. P., and Larusso, N. F. (2013). Physiology of cholangiocytes. Compr. Physiol. 3, 541–565. doi: 10.1002/cphy.c120019
Tietz, P. S., Marinelli, R. A., Chen, X. M., Huang, B., Cohn, J., Kole, J., et al. (2003). Agonist-induced coordinated trafficking of functionally related transport proteins for water and ions in cholangiocytes. J. Biol. Chem. 278, 20413–20419. doi: 10.1074/jbc.M302108200
Tietz, P. S., Mcniven, M. A., Splinter, P. L., Huang, B. Q., and Larusso, N. F. (2006). Cytoskeletal and motor proteins facilitate trafficking of AQP1-containing vesicles in cholangiocytes. Biol. Cell 98, 43–52. doi: 10.1042/BC20040089
Uriarte, I., Banales, J. M., Sáez, E., Arenas, F., Oude Elferink, R. P. J., Prieto, J., et al. (2010). Bicarbonate secretion of mouse cholangiocytes involves Na+-HCO−3 cotransport in addition to Na+-independent Cl−/HCO−3 exchange. Hepatology 51, 891–902. doi: 10.1002/hep.23403
Úriz, M., Sáez, E., Prieto, J., Medina, J. F., and Banales, J. M. (2011). Ursodeoxycholic acid is conjugated with taurine to promote secretin-stimulated biliary hydrocholeresis in the normal rat. PLoS ONE 6:e28717. doi: 10.1371/journal.pone.0028717
Vázquez, J. J., Vázquez, M., Idoate, M. A., Montuenga, L., Martínez-Ansó, E., Castillo, J. E., et al. (1995). Anion exchanger immunoreactivity in human salivary glands in health and Sjogren's syndrome. Am. J. Pathol. 146, 1422–1432.
Keywords: bile flow, biliary HCO−3 secretion, cholangiocytes, Cl−/HCO−3 anion exchange, primary biliary cirrhosis
Citation: Concepcion AR, Lopez M, Ardura-Fabregat A and Medina JF (2014) Role of AE2 for pHi regulation in biliary epithelial cells. Front. Physiol. 4:413. doi: 10.3389/fphys.2013.00413
Received: 01 October 2013; Paper pending published: 18 October 2013;
Accepted: 27 December 2013; Published online: 17 January 2014.
Edited by:
Ebbe Boedtkjer, Aarhus University, DenmarkReviewed by:
Dominique Eladari, Institut National de la Santé et de la Recherche Médicale, FranceJens Leipziger, Aarhus University, Denmark
Copyright © 2014 Concepcion, Lopez, Ardura-Fabregat and Medina. This is an open-access article distributed under the terms of the Creative Commons Attribution License (CC BY). The use, distribution or reproduction in other forums is permitted, provided the original author(s) or licensor are credited and that the original publication in this journal is cited, in accordance with accepted academic practice. No use, distribution or reproduction is permitted which does not comply with these terms.
*Correspondence: Juan F. Medina, Division of Gene Therapy and Hepatology, Center for Applied Medical Research (CIMA), School of Medicine, University of Navarra, Ave. Pio XII, 55, E-31008 Pamplona, Spain e-mail:amZtZWRpbmFAdW5hdi5lcw==