- 1Department of Pharmacology, Division of Pathological Sciences, Kyoto Pharmaceutical University, Kyoto, Japan
- 2Institute of Resource Development and Analysis, Kumamoto University, Kumamoto, Japan
The alkaline pH-activated, two-pore domain K+ channel K2P5.1 (also known as TASK2/KCNK5) plays an important role in maintaining the resting membrane potential, and contributes to the control of Ca2+ signaling in several types of cells. Recent studies highlighted the potential role of the K2P5.1 K+ channel in the pathogenesis of autoimmune diseases such as rheumatoid arthritis and multiple sclerosis. The aim of the present study was to elucidate the pathological significance of the K2P5.1 K+ channel in inflammatory bowel disease (IBD). The degrees of colitis, colonic epithelial damage, and colonic inflammation were quantified in the dextran sulfate sodium-induced mouse IBD model by macroscopic and histological scoring systems. The expression and functional activity of K2P5.1 in splenic CD4+ T cells were measured using real-time PCR, Western blot, and fluorescence imaging assays. A significant increase was observed in the expression of K2P5.1 in the splenic CD4+ T cells of the IBD model. Concomitant with this increase, the hyperpolarization response induced by extracellular alkaline pH was significantly larger in the IBD model with the corresponding intracellular Ca2+ rises. The expression of K2P5.1 was higher in CD4+CD25− T cells than in CD4+CD25+ regulatory T cells. The knockout of K2P5.1 in mice significantly suppressed the disease responses implicated in the IBD model. Alternations in intracellular Ca2+ signaling following the dysregulated expression of K2P5.1 were associated with the disease pathogenesis of IBD. The results of the present study suggest that the K2P5.1 K+ channel in CD4+CD25− T cell subset is a potential therapeutic target and biomarker for IBD.
Introduction
Due to the overlap in the pathogeneses and pharmacological treatments, Crohn's disease (CD) and ulcerative colitis (UC) are types of inflammatory bowel diseases (IBD) (Bouma and Strober, 2003; McCole, 2014). Dextran sulfate sodium (DSS) is known to chemically induce the pathogenesis of CD and UC in rodents (Okayasu et al., 1990; Dieleman et al., 1994). A genome-wide expression profiling study showed that DSS-associated genes in mice correlated with data obtained from IBD patients (Fang et al., 2011). Therefore, the DSS-induced IBD model is a commonly used model of IBD in mice, and a number of studies have used the DSS-induced IBD model to investigate the pathogenesis of CD and UC.
An elevation in intracellular Ca2+ by the release of Ca2+ from intracellular Ca2+ stores and Ca2+ influx through store-operated Ca2+ channels is one of the essential triggering signals for T cell activation (Cahalan and Chandy, 2009; Hogan et al., 2010). The K+ channel is also one of the key molecules that modulates Ca2+ signaling in T cells because it provides an electrochemical gradient to drive Ca2+ influx, and two major K+ channels (the voltage-gated, KV1.3 and intermediate-conductance Ca2+-activated, KCa3.1) function in T and B lymphocytes (Cahalan and Chandy, 2009). Recent studies including our previous study demonstrated that the upregulation of ion channels (Orai/STIM, TRPV1, TRPA1, and KCa3.1) in CD4+ T cells was involved in the pathogenesis of IBD, while their pharmacological blockade elicited a significant decrease in IBD disease severity (Di Sabatino et al., 2009; Di et al., 2010; Bertin et al., 2014; Kun et al., 2014; Ohya et al., 2014).
The background or leak two-pore domain K+ (K2P) channel superfamily includes 16 members and plays a crucial role in diverse physiological functions through the regulation of cell excitability (Enyedi and Czirják, 2010; Es-Salah-Lamoureux et al., 2010). The K2P5.1 K+ channel (also known as TASK2/KCNK5) is activated by extra- and intracellular alkalization, and plays an important role in maintaining the resting membrane potential and the control of Ca2+ signaling in various types of cells. K2P5.1 has been shown to control diverse physiological and pathophysiological processes including innate immunity and cancer progression (Bittner et al., 2010b; Clark et al., 2011; Cid et al., 2013), and is, therefore, expected to become a potential therapeutic target for the treatment of autoimmune, inflammatory, and allergic diseases as well as several types of cancers. Recent studies described the pathophysiological impact of the upregulation of K2P5.1 in CD4+ and CD8+ T cells on the pathogenesis of autoimmune diseases such as rheumatoid arthritis and multiple sclerosis (Bittner et al., 2010a, 2011). K2P5.1 is inhibited by non-selective K+ channel inhibitors such as quinidine, lidocaine, and clofilium and is activated by volatile anesthetics (Wulff and Zhorov, 2008); however, the lack of selective blockers is represents an important difficulty in experimental studies on K2P5.1.
In the present study, we examined the involvement of the K2P5.1 K+ channel in CD4+ T lymphocytes in the pathogenesis of IBD using a mouse model of chemically-induced IBD. We further compared histopathologies between the DSS-induced IBD model using wild-type and K2P5.1-deficient mice.
Materials and Methods
Preparation of the Dextran Sulfate Sodium (DSS)-induced Mouse IBD Model
Male C57BL/6J (6–7 weeks of age) mice were purchased from Shimizu Laboratory Supplies (Kyoto, Japan), and were acclimatized for 1 week before the experiment. They were given distilled water containing 5.0% (wt/vol) dextran sulfate sodium 5000 (DSS) (Wako Pure Chemical, Osaka, Japan) ad libitum. Control mice were given drinking water only. Seven days after the administration of DSS, mice were sacrificed, tissue samples were taken, and colitis and inflammation were assessed macroscopically and scored as previously reported (Ohya et al., 2014). All experiments were carried out in accordance with the guiding principles for the care and use of laboratory animals in Kyoto Pharmaceutical University, and the protocols were approved by the committee on the Ethics of Animal Research of Kyoto Pharmaceutical University (Permit Number: 15-12-091). K2P5.1 heterozygous knockout mice bred in a C57BL6 background [B6;CB-Kcnk5Gt(pU-21)81Imeg] were established by the exchangeable gene trap method (Araki et al., 2014). Homozygous (K2P5.1−/−) knockout mice were generated by crossing heterozygous (K2P5.1+/−) males with K2P5.1+/− females (Cid et al., 2013). Genomic DNA was isolated from finger or tail biopsies following a 3-h digestion at 50°C in buffer containing 50 mM Tris-HCl (pH 8.0), 100 mM EDTA, 100 mM NaCl, 0.1% SDS, and 1 mg/mL proteinase K, followed by heat inactivation. PCR was performed using the primer pairs to distinguish the K2P5.1 wild type (forward: 5′-GCTGAGAACAATAGGGACAG-3′ and reverse: 5′-TCACCCAGCTTTGGGATTCC-3′) and gene trapped (forward: 5′-GCTGAGAACAATAGGGACAG-3′and reverse: 5′-TACAGGCATCGTGGTGTCAC-3′). PCR conditions were as follows: 30 cycles at 94°C for 30 s, 60°C for 30 s, and 68°C for 30 s. The amplified products were separated on 1.0% agarose gels, and visualized by ethidium bromide staining. DSS-induced IBD model mice were prepared using male and female K2P5.1−/− (6–10 weeks of age) and K2P5.1+/− (6 weeks of age) mice.
Histological Scoring
Inflammation and crypt damage in hematoxylin and eosin (H&E)-stained sections were assessed as reported previously (Dieleman et al., 1994; Ohya et al., 2014). Briefly, for histological assessments, 1 cm of tissue collected from the distal colon was fixed in 10% buffered formalin, embedded in a paraffin block, cut into 5-μm-thick sections, and stained with H&E. The inflammation score was determined as a multiplication of the severity grade of inflammation (grade 0, none; grade 1, slight; grade 2, moderate; grade 3, severe) as well as its extent (grade 0, none; grade 1, mucosa; grade 2, mucosa, and submucosa; grade 3, transmural). The crypt damage score was determined as a multiplication of the damage grade of the crypt (grade 0, none; grade 1, basal 1/3 damage; grade 2, basal 2/3 damage; grade 3 only the surface epithelium intact; grade 4, entire crypt, and epithelium lost) and the percent area score (grade 1, 1–25%; grade 2, 25–50%; grade 3, 51–75%; grade 4, 76–100%). Data were obtained from three sections of the colon measured at least 200 μm apart per animal from four individual mice per group.
Cell Isolation, RNA Extraction, Reverse Transcription, and Real-time PCR
Total RNA was extracted and reverse transcribed from the T cells of mouse tissues (Ohya et al., 2013, 2014). Single cell suspensions were prepared by pressing the spleen (also the thymus and mesenteric lymph nodes) with a frosted grass slide and then filtering through a nylon mesh. CD4+ cells were isolated from cell suspensions by Dynabeads® FlowComp™ Mouse CD4+ (Invitrogen, Grand Island, NY, USA), according to the experimental manual supplied by Invitrogen, and were then collected in phosphate buffered saline (PBS) supplemented with 0.1% bovine serum albumin. A flow cytometric analysis confirmed that more than 95% of the purified T cells were CD4+. CD4+CD25− and CD4+CD25+ cells were also isolated from cell suspensions by Dynabeads® FlowComp™ Mouse CD4+ CD25+ Treg Cells (Invitrogen). A flow cytometric analysis confirmed that more than 90% of purified T cells were CD4+CD25− and CD4+CD25+. The resulting cDNA products were amplified with gene-specific primers, and primers were designated using Primer Express™ software (Ver 1.5, Applied Biosystems, Foster City, CA, USA). Quantitative, real time PCR was performed using Syber Green chemistry (SYBR® Premix Ex Taq™ II) (TaKaRa BIO, Osaka, Japan) on an ABI 7500 sequence detector system (Applied Biosystems) as previously reported (Ohya et al., 2014). The following PCR primers for mouse clones were used for real-time PCR: K2P5.1 (GenBank accession number: NM_021542, 722-844), amplicon = 123 bp; K2P3.1 (AF065162, 692-812), 121 bp; K2P9.1 (NM_001033876, 757-877), 121 bp; K2P16.1 (NM_029006, 191-312), 122 bp; interferon (IFN)-γ (NM_008337, 222-323), 102 bp; interleukin-4 (IL-4) (NM_021283, 126-246), 121 bp; IL-10 (NM_010548, 245-355), 111 bp; CD25 (NM_008367, 522-642), 121 bp; IL-17A (NM_010552, 165-277), 113 bp; β-actin (ACTB) (NM_031144, 419-519), 101 bp. Regression analyses of the mean values of three multiplex RT-PCRs for log10 diluted cDNA were used to generate standard curves (Ohya et al., 2014). Unknown quantities relative to the standard curve for a particular set of primers were calculated, yielding the transcriptional quantitation of gene products relative to the endogenous standard, ACTB. The following PCR primers were used for real-time PCR to confirm the knockout of K2P5.1 transcripts in T cells from K2P5.1−/− mice: K2P5.1 (NM_021542, 472-567), 96 bp.
Western Blotting
Splenic CD4+ T cells were solubilized with lysis buffer with 1% SDS. The same amount of proteins (10 μg for each) were subjected to 10% SDS-PAGE (Ohya et al., 2014). The blots were incubated with an anti-K2P5.1 antibody (G-14 or H-170, Santa Cruz Biotechnology, Santa Cruz, CA, USA), and then incubated with anti-rabbit or anti-goat horseradish peroxidase-conjugated IgG (Millipore). An enhanced chemiluminescence detection system (GE Healthcare, Buckinghamshire, England) was used to detect the bound antibody. The resulting images were analyzed by a LAS-3000mini device (Fujifilm, Tokyo, Japan). The blots were also probed with an anti-β-actin (ACTB) antibody (Medical & Biological Laboratories, Nagoya, Japan).
Measurement of the Membrane Potential and Intracellular Ca2+ Concentrations by Fluorescent Indicators
The membrane potential was measured using the fluorescent voltage-sensitive dye DiBAC4(3), as previously reported (Ohya et al., 2013, 2014). Glass bottom tissue culture dishes were coated with fibronectin (Wako Pure Chemical Industries, Osaka, Japan) in Ca2+ and Mg2+-free PBS at 50 μg/mL for 1 μg/cm2 at 4°C overnight. Cells were seeded onto fibronectin-coated dishes and cultured for 2 h at 37°C in 5% CO2 humidified incubator. Prior to the fluorescence measurements with DiBAC4(3), cells were incubated in normal HEPES buffer containing 100 nM DiBAC4(3) for 20 min at room temperature. The cells were continuously incubated with 100 nM DiBAC4(3) throughout the experiments. In membrane potential imaging, cells loaded with DiBAC4(3) were illuminated at a wavelength of 490 nm, and fluorescence images were recorded on the ORCA-Flash2.8 digital camera (Hamamatsu Photonics, Hamamatsu, Japan). Additionally, intracellular Ca2+ concentrations were measured using the fluorescent Ca2+ indicator dye Fura 2-AM. Cells were incubated with 10 μM Fura 2-AM in normal HEPES solution for 30 min at room temperature. Cells loaded with Fura 2-AM were alternatively illuminated at wavelengths of 340 and 380 nm, and fluorescence images were recorded. The fluorescent intensity of Fura 2 was expressed as measured 340/380 nm fluorescence ratios after background subtraction. Data collection and analyses were performed using an HCImage system (Hamamatsu Photonics). Images were measured every 5 s, and the values of fluorescent intensity (F) were determined by measuring the average for 1 min (12 images).
Flow Cytometric Analysis
Cell surface markers were analyzed with BD FACSCalibur (BD Biosciences, San Jose, CA, USA), which acquired at least 10,000 events, and gated according to forward- and side-scatter (Ohya et al., 2013). Data were analyzed using CellQuest software (BD Biosciences). The lymphocyte gate was established by analyzing the forward angle vs. right angle light scatter. The percentage of positive-staining cells was determined by comparing the test histograms with those obtained using a fluorescein isothiocyanate (FITC) or phycoerythrin (PE)-conjugated anti-CD4 antibody (FITC/PE-CD4), FITC-conjugated CD3 (FITC-CD3), PE-conjugated CD8 (PE-CD8), and PE-conjugated CD25 (PE-CD25) (BD Biosciences). After being incubated with the antibodies for 1 h at room temperature, excess antibodies were removed by repeated washing with PBS.
Chemicals
The sources of pharmacological agents were as follows: clofilium tosylate (Sigma-Aldrich, Tokyo, Japan), DiBAC4(3), (Dojindo, Kumamoto, Japan), Fura 2-AM (Invitrogen), and 2-APB (Tocris Bioscience, Ellisville, MO, USA). All others were obtained from Sigma-Aldrich or Wako Pure Chemical Industries.
Statistical Analysis
Significant differences between two and among multiple groups were evaluated using the Student's t- and Welch's t-tests or Tukey's test after the F-test or ANOVA. Data that were non-normal distributed were analyzed using the Mann-Whitney U-test. Significance at P < 0.05 and P < 0.01 was indicated in the figures. Data are presented as means ± SE.
Results
Upregulated Expression of the K2P5.1 K+ Channel in Splenic CD4+ T Cells from the Mouse Model of DSS-induced Inflammatory Bowel Disease
Mice were sacrificed 7 days after the administration of 5.0% (wt/vol) DSS and tissue samples were collected. Control mice were given drinking water only. As previously reported (Ohya et al., 2014), severe macroscopic symptoms (colitis and fecal blood) were detected in DSS-induced IBD model mice, and the scores of colonic inflammation and crypt damage were significantly higher in IBD model mice than in control mice (not shown). As reported by Pereira et al. (1987), enlargement of the spleen, “splenomegaly,” was detected in IBD model mice (Figure 1A), and a significant increase in the transcriptional expression of the Th1 cytokine interferon-γ (IFN-γ) and Th17 cytokine interleukin-17 (IL-17) was detected (Figures 1B,C), without any changes in the Th2 cytokine IL-4 (not shown). The flow cytometric analysis showed no significant differences of the CD4+ phenotypic ratio between isolated splenic CD3+ T cells from both groups (Figure 1H).
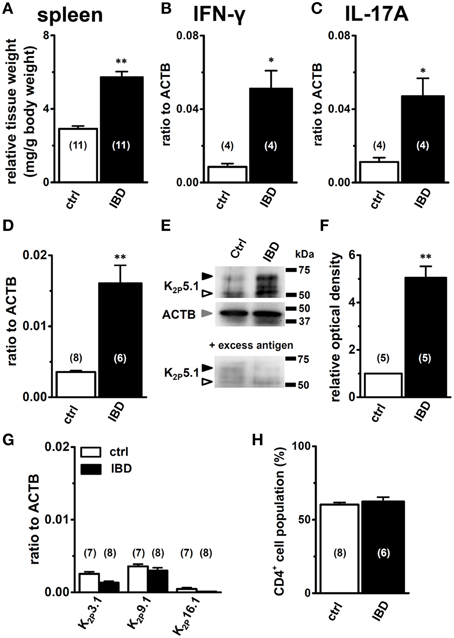
Figure 1. Expression levels of K2P5.1 and pro-inflammatory cytokines in splenic CD4+ T lymphocytes of chemically-induced IBD model mice. (A) spleen weight relative to body weight in control and IBD mice. (B–D) quantitative, real-time PCR assay for the expression of IFN-γ (B), IL-17A (C), and K2P5.1 (D) transcripts in the splenic CD4+ cells of control and IBD model mice. Expression levels are expressed as a ratio to β-actin (ACTB). (E) splenic CD4+ cell lysates were probed by immunoblotting with an anti-K2P5.1 (G-14) antibody. Molecular weight standards are shown in kilodaltons (kDa) on the right. Arrowheads indicate the migrating positions of K2P5.1 and ACTB proteins (open: K2P5.1, closed: glycosylated K2P5.1, gray: ACTB). Lower panel shows signals after the pre-incubation of a primary antibody with excess antigens. (F) summarized data obtained from (E) as the optical density of the band signal for K2P5.1 (open arrowhead) in the IBD model relative to that in normal mice. (G) Expression of alkaline pH-activated K2P K+ channel subtype (K2P3.1, 9.1, and 16.1) transcripts in the splenic CD4+ T cells of control and IBD model mice. (H) the percentages of the CD4+ T cell population relative to CD3+ T cells were analyzed by flow cytometry, acquiring at least 10,000 events. Results were expressed as means ± SEM. Numbers used for the experiments are shown in parentheses. *, **P < 0.05, 0.01 vs. control mice (ctrl).
The quantitative, real-time PCR assay showed that the expression level of the K2P5.1 transcripts was significantly higher in the splenic CD4+ T cells of IBD model mice (IBD) than in control mice (ctrl) (Figure 1D). The expression levels of K2P5.1 relative to ACTB (in arbitrary units) were 0.0036 ± 0.0002 and 0.0161 ± 0.0025 in the splenic CD4+ T cells of control mice (n = 8) and IBD model mice (n = 6, P < 0.01), respectively. The western blot examination showed that the amount of the K2P5.1 protein (~50 kDa) in the cell lysate of splenic CD4+ T cells was higher in IBD model mice than in control mice (Figure 1E, upper panel, open arrowhead), which was consistent with the results obtained by real-time PCR examination. The amount of the glycosylated K2P5.1 protein (~65 kDa, Figure 1E, upper panel, closed arrowhead) was also higher in the IBD model. The optical density for the K2P5.1 protein band signal (~50 kDa) relative to that for ACTB (Figure 1E, middle panel) was calculated using Image J software (Ver. 1.42, NIH), and its protein expression level in control mice was expressed as 1.00. The relative optical density in the IBD model was 5.05 ± 0.48 (n = 5 for each, P < 0.01) (Figure 1F). The two band signals specific for an anti-K2P5.1 antibody (G-14) disappeared with a pre-incubation with excess antigens (Figure 1E, lower panel). Similar results were obtained when another anti-K2P5.1 antibody (H-170) was used as the primary antibody (Supplementary Figure 1). In addition, the expression levels of the other alkaline pH-activated K2P channel subtypes, K2P3.1/TASK1, K2P9.1/TASK3, and K2P16.1/TALK1 transcripts, were less abundant in the IBD model: < 0.004 in arbitrary units (Figure 1G).
Enhancement in Alkaline pHe-induced Hyperpolarizing Responses in Splenic CD4+ T Cells of IBD Model Mice
To perform functional analysis of K2P5.1 in splenic CD4+ T cells, we measured the hyperpolarizing responses induced by the alkalization of extracellular pH (pHe) (pHe8.0 and 8.5) using the membrane potential-sensitive dye, DiBAC4(3). To confirm living cells, 140 mM high K+-induced depolarization was measured at the end of protocol. As shown in Figure 2A, the fluorescence intensity of DiBAC4(3) decreased with the application of alkaline pHe (pHe8.5). The peak amplitude of the relative fluorescence intensity of DiBAC4(3) was lower in IBD model mice than in control mice (Figure 2B). When alkaline pHe-induced hyperpolarizing responses (pHe8.0 and 8.5) were expressed as changes in the relative fluorescence intensity of DiBAC4(3) [Δ relative fluorescence intensity of DiBAC4(3)], they were significantly larger in IBD model mice than in control mice (Figure 2C). Under alkaline pHe conditions, high K+-induced increases in the fluorescence intensity of DiBAC4(3) were almost the same as those under normal pHe (pHe7.4) conditions (Figure 2B). In the presence of the non-specific K2P5.1 blocker, clofilium (5 μM), alkaline pHe (pHe8.5)-induced hyperpolarizing responses disappeared in both groups. Significantly larger depolarizing responses were observed in IBD model mice (Figure 2D), suggesting that alkaline pHe-induced hyperpolarizing responses via the activation of K2P5.1 in IBD model mice were underestimated. Clofilium also inhibits voltage-gated K+ channel KV1.3, one of main K+ channels in T cells, however, 4-aminopyridine (5 mM), a KV1.3 blocker, did not induce any significant differences in the alkaline pHe (pHe 8.5)-induced hyperpolarizing responses [Δ relative fluorescence intensity of DiBAC4(3) = −0.17±0.04 (n = 19)]. A recent study demonstrated that the Ca2+-release activated Ca2+ (CRAC) channel, Orai1, which contributes to the store-operated entry of Ca2+ into T cells, was activated by alkaline pH (Beck et al., 2014), suggesting that the function of Orai1 was upregulated in the splenic CD4+ T cells of IBD model mice. However, depolarizing responses were not suppressed by the pre-treatment with the Orai1 blocker 2-APB (10 μM) for more than 5 min (P < 0.01) (Figure 2D), and the expression levels of Orai1 transcripts were also not changed between the two groups (not shown). We subsequently observed a relationship between alkaline pHe-induced hyperpolarization responses and [Ca2+]i rises via CRAC channels in the splenic CD4+ T cells of IBD model mice by simultaneously measuring DiBAC4(3) and Fura 2 signals (Figure 2E). As shown in Figure 2F, alkaline pHe-induced hyperpolarization responses positively correlated with [Ca2+]i rises in the splenic CD4+ T cells of IBD model mice (R2 = 0.41). The alkaline pHe-induced [Ca2+]i rises were suppressed by about 40% by the pre-treatment with 2-APB (10 μM) (not shown).
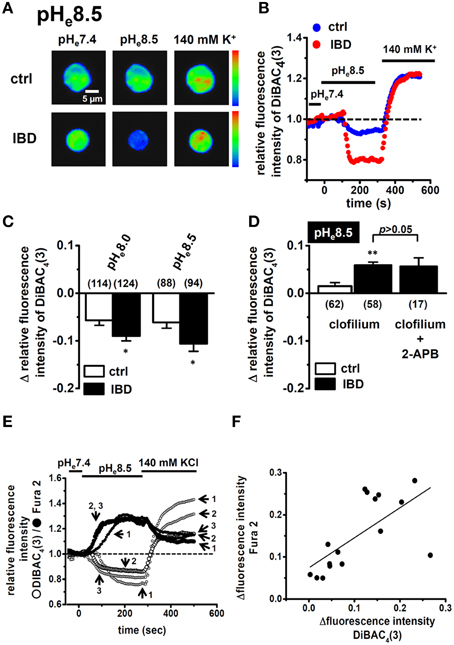
Figure 2. Effects of alkaline pHe on membrane potential and intracellular Ca2+ mobilization in splenic CD4+ T lymphocytes of IBD model mice. (A,B) measurement of alkaline pHe (pHe 8.5)-induced hyperpolarizing responses in splenic CD4+ T cells using the voltage-sensitive fluorescent dye DiBAC4(3). Images (A) are shown in pseudo-colors from blue (low intensity) to red (high intensity) in the bar. (B) shows the time course of changes in the fluorescence intensity of DiBAC4(3). The fluorescence intensity of DiBAC4(3) at 0 s (pHe 7.4) is expressed as 1.0. (C) summarized data are shown as alkaline pHe (pHe 8.0 and 8.5)-induced fluorescence changes [Δrelative fluorescence intensity of DiBAC4(3)] in splenic CD4+ T cells of control and IBD model mice. (D) effects of clofilium (5 μM) and the combination of clofilium (5 μM) and 2-APB (10 μM) on alkaline-pHe (pHe 8.5)-induced hyperpolarizing responses in the splenic CD4+ T cells of control and IBD model mice. Cells were pre-treated with clofilium and/or 2-APB for 30 min. (E,F) simultaneous measurements of changes in the membrane potential and intracellular Ca2+ concentrations by hyperpolarizing (pHe 8.5) and depolarizing (140 mM KCl) stimulations using DiBAC4(3) and Fura 2, a Ca2+ indicator. (E) shows the time course of changes in their fluorescence intensities, and summarizing data are shown in (F). Results were expressed as means ± SEM. Numbers used for the experiments are shown in parentheses. *, **P < 0.05, 0.01 vs. control mice (ctrl).
Higher Expression Level of K2P5.1 in the Inflammatory CD4+CD25− Subset than in the Regulatory CD4+CD25+ Subset
When K2P5.1 is upregulated in Th1/Th17 cells producing IFN-γ/IL-17, the inhibition of K2P5.1 may suppress the disease progression of IBD. However, when K2P5.1 is upregulated in regulatory T cells producing IL-10, the inhibition of K2P5.1 may promote the disease progression of IBD. In order to clarify the pathophysiological significance of the K2P5.1 K+ channel in IBD, we examined differences in the expression levels of K2P5.1 between the splenic CD4+CD25− and CD4+CD25+ subsets in IBD model mice using a real-time PCR assay. As shown in Figure 3, the proinflammatory Th1/Th17 cytokines IFN-γ (Figure 3B) and IL-17A (Figure 3C) transcripts were expressed at high levels in the CD4+CD25− subset of IBD model mice, whereas the natural regulatory T cell (Treg) markers CD25 (Figure 3D), IL-10 (Figure 3E), and Foxp3 (not shown) were predominantly expressed in the CD4+CD25+ subset. As shown in Figure 3A, K2P5.1 transcripts were expressed at significantly higher levels in the CD4+CD25− subset in IBD model mice than in control mice. Significant increases in the expression levels of the K2P5.1 transcripts were also found in the CD4+CD25+ subset of IBD model mice (P < 0.01 vs. control) (Figure 3A); however, these increases were significantly lower than those observed in the CD4+CD25− subset of IBD model mice (P < 0.01). Correspondingly, similar levels of alkaline pHe-induced hyperpolarizing responses (pHe8.5) were observed in the CD4+CD25− subset of IBD model mice but not in the CD4+CD25+ subset (not shown). These results suggested that the pharmacological inhibition of K2P5.1 is an effective strategy for the treatment of IBD.
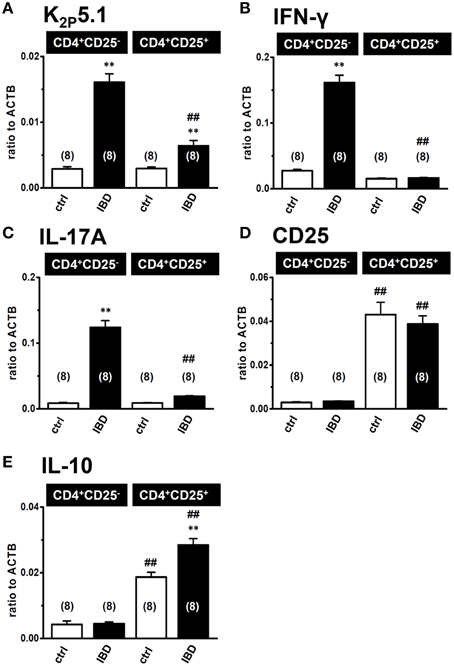
Figure 3. Expression levels of K2P5.1 and pro- and anti-inflammatory cytokine transcripts in splenic CD4+CD25− and CD4+CD25+ subsets of IBD model mice. (A–E) real-time PCR assay for the expression of K2P5.1 (A), IFN-γ (B), IL-17A (C), CD25 (D), and IL-10 (E) transcripts in the splenic CD4+CD25− and CD4+CD25+ T cells of control and IBD model mice. Results were expressed as means ± SEM. Numbers used for the experiments are shown in parentheses. **P < 0.01 vs. control mice (ctrl), ##P < 0.01 vs. the CD4+CD25− subset.
Decreased Susceptibility to the Pathogenesis of IBD in K2P5.1 Knockout Mice
As described above, the lack of selective pharmacological agents represents the main difficulty in carrying out experimental studies on K2P5.1. Therefore, we examined the effects of the genetic inhibition of K2P5.1 on inflammatory responses during acute IBD in K2P5.1 homozygous knockout (K2P5.1−/−) mice. As reported in our recent study (Ohya et al., 2014), severe clinical symptoms and enlargement of the spleen were observed in wild-type K2P5.1+/+ mice (Figures 4A–C, left columns). A significant protective effect against IBD was observed in K2P5.1−/− mice (Figures 4A,B, right columns). The diarrhea scores were 2.25 ± 0.16 (n = 8) and 1.41 ± 0.17 (n = 17) in K2P5.1+/+ and K2P5.1−/− mice, respectively (P < 0.01 vs. K2P5.1+/+). The degrees of splenomegaly was also significantly lower in K2P5.1−/− mice (P < 0.05 vs. K2P5.1+/+). Furthermore, colonic inflammation and crypt damage were quantified by colon weight/length ratio measurements and histological scoring. As shown in Figure 4D, colonic wall thickening was significantly increased in K2P5.1+/+ mice, whereas no significant differences between control and IBD were observed in K2P5.1−/− mice. Histological assessments of colonic inflammation and crypt damage also revealed that both scores were significantly lower in K2P5.1−/− mice than in K2P5.1+/+ mice (P < 0.01 for the inflammation score, P < 0.05 for the crypt damage score) (Figures 4E–G). No significant differences in the degrees of colitis, colonic inflammation, or crypt damage were observed in heterozygous knockout mice (K2P5.1+/−) [diarrhea score: 1.95 ± 0.20 (n = 22), visible fecal blood score: 2.32 ± 0.17 (n = 22), inflammation score: 10.43 ± 0.87 (n = 7), crypt damage score: 9.86 ± 0.86 (n = 7)]. In K2P5.1−/− and K2P5.1+/− mice drinking water only for 7 days, all scores assessed in this study were 0 (not shown). In addition, in the splenic CD4+CD25− and CD4+CD25+ cells of K2P5.1−/− mice, the expression level of K2P5.1 transcripts almost disappeared (Figure 4H), and alkaline pHe (pHe8.5)-induced hyperpolarizing responses were also very small in the splenic CD4+ T cells of K2P5.1−/− mice (Figure 4I). These results suggest that K2P5.1 inhibitors may be efficacious in patients with IBD.
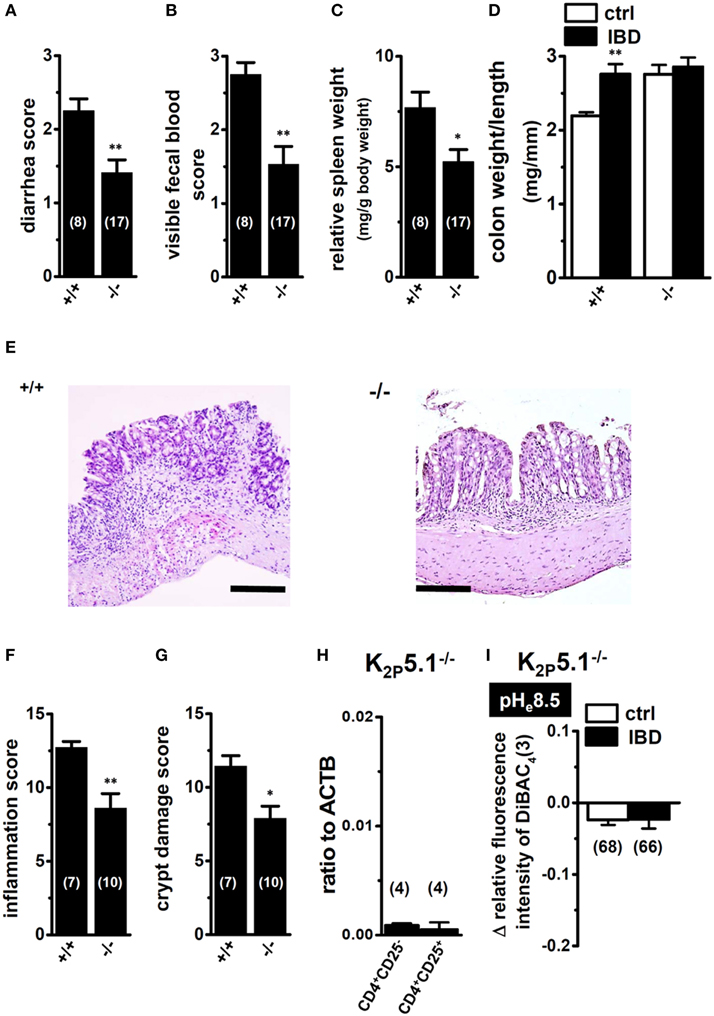
Figure 4. Macroscopic disease activities and histopathological assessments in the IBD model from wild-type (+/+) and homozygous K2P5.1 knockout (-/-) mice. (A,B) Seven days after DSS exposure, diarrhea, and visible fecal blood severities were scored on a 0–3 scale (0, Normal pellets; 1, Slightly loose feces; 2, Loose feces; 3, Watery diarrhea) and a 0–3 scale (0, Normal; 1, Slightly bloody; 2, Bloody; 3, Blood in the whole colon), respectively, in K2P5.1+/+ and K2P5.1−/− mice. (C) spleen weights relative to body weights in K2P5.1+/+ and K2P5.1−/− mice. (D) the colon weight/length ratio was measured in K2P5.1+/+ and K2P5.1−/− mice. (E–G) Photographs of colon sections from K2P5.1+/+ and K2P5.1−/− mice with H&E staining (magnification, X100) (E). Scale bar, 100 μm. Colonic inflammation (F) and crypt damage (G) were assessed histologically. (H) Expression of K2P5.1 transcripts relative to ACTB transcripts in CD4+CD25− and CD4+CD25+ subsets from K2P5.1−/− mice. (I) summarized data are shown as alkaline pHe (pHe 8.5)-induced hyperpolarizing responses in the splenic CD4+ T cells of control and IBD model mice. Results were expressed as means ± SEM. The numbers used for the experiments are shown in parentheses. *, **P < 0.05, 0.01 vs. K2P5.1+/+ (D).
Discussion
The activation of K+ channels in T cells promotes Ca2+ influx, thereby indirectly modulating Ca2+ signaling (Vig and Kinet, 2009). Two K+ channel subtypes, the voltage-gated KV1.3 and intermediate-conductance Ca2+-activated K+ channel KCa3.1, are known to mainly function in T and B lymphocytes (Di Sabatino et al., 2009). The physiological role of the two-pore domain K+ channel K2P5.1 was recently clarified in lymphocytes (Nam et al., 2011; Cid et al., 2013; Shin et al., 2014), and several studies have shown the pathophysiological impact of the upregulation of the two-pore domain K+ channel K2P5.1 in CD4+ T cells on the pathogenesis of autoimmune diseases such as rheumatoid arthritis and multiple sclerosis (Bittner et al., 2010a, 2011). However, the pathophysiological impact of K2P5.1 in diseases associated with Th1 and Th17 cytokine profiles has not yet been examined using in vivo animal models. This is the first study to employ chemically-induced IBD model mice to characterize the pathophysiological role of K2P5.1 in mature CD4+ T cells. The main results of the present study were as follows: (1) Facilitation of the expression level and functional activity of the K2P5.1 K+ channel in splenic CD4+ T cells of IBD model mice, especially in the CD4+CD25− subset (Figures 1, 2), (2) Decreased disease activity index (diarrhea, bloody feces, and weight loss) and histopathological scores (colonic inflammation and crypt damage) in homozygous K2P5.1-deficient (K2P5.1−/−) mice (Figure 4). We were unable to elucidate the mechanism underlying the transcription regulation of K2P5.1 in the splenic CD4+ T cells of IBD model mice. The voltage-gated K+ channel KV1.3 and Ca2+-activated K+ channel KCa3.1 are the main K+ conductance channels in CD4+ T cells. Although both transcripts were expressed in splenic CD4+CD25− cells at high levels, no significant differences were observed in their expression levels between control and IBD model mice (not shown). These results suggested that the upregulation of K2P5.1 played an important role in the changes observed in T cell Ca2+ signaling in IBD pathogenesis, and may affect the facilitation of proliferation and infiltration of splenic CD4+ T cells. K2P5.1 also contributes to the regulation of osmotic volume, regulatory volume decreases (RVD), in several types of cells including T cells (Niemeyer et al., 2001; Bobak et al., 2011; Andronic et al., 2013), which participate in cell cycle progression. Therefore, a dysfunction in cellular volume homeostasis via the upregulation of K2P5.1 may also be involved in dysregulated cellular functions in the CD4+ T cells of IBD model mice.
As shown in Figure 3A, the upregulation of K2P5.1 was greater in splenic CD4+CD25− T cells of IBD model mice than in CD4+CD25+ T cells. CD4+CD25− T cells include pro-inflammatory Th1 and Th17 subsets, and an increase was observed in the transcription of IFN-γ and IL-17A in the splenic CD4+CD25− T cells of IBD model mice (Figures 3B,C). Due to the lack of a selective K2P5.1 inhibitor, we were unable to examine the effects of its in vivo administration on the disease activity index and histopathological scores in IBD model mice in order to clarify the pathophysiological significance of K2P5.1 in IBD. However, we showed that the genetic knockdown of K2P5.1 significantly protected against IBD via the amelioration of severe colitis and colonic inflammation using homozygous K2P5.1 knockdown K2P5.1−/− mice (Figure 4). Furthermore, the expression levels of the IFN-γ transcripts from the pro-inflammatory Th1 subset were significantly lower in the CD4+CD25− cells of the IBD model from K2P5.1−/− mice than from K2P5.1+/+ mice (Figure 5A), and no significant differences were noted in the expression levels of IL-17 in CD4+CD25− cells and IL-10 in CD4+CD25+ cells between IBD models from K2P5.1+/+ and K2P5.1−/− mice (Figures 5B,C). Similar findings were obtained with the genetic and pharmacological knockdown of the Ca2+-activated K+ channel KCa3.1 in an IBD model (Di et al., 2010; Ohya et al., 2014; Ohya and Imaizumi, 2014). It has been reported that the function of Th1 was impaired in an IBD model from KCa3.1−/− mice, whereas that of Th17 was normal (Di et al., 2010). We recently demonstrated the inhibition of IFN-γ transcription, but not that of IL-17A by the in vivo administration of selective KCa3.1 inhibitors in a DSS-induced IBD model (Ohya et al., 2014). Therefore, the development of small molecule K2P5.1 modulators may represent therapeutic and/or preventative strategies for the treatment of inflammatory and autoimmune disorders and cancer, and the establishment of a novel high-throughput screening system to detect these modulators currently required (Bagriantsev et al., 2013). Furthermore, novel findings on the regulatory molecules of K2P5.1 will provide insights into the inhibition of K2P5.1.
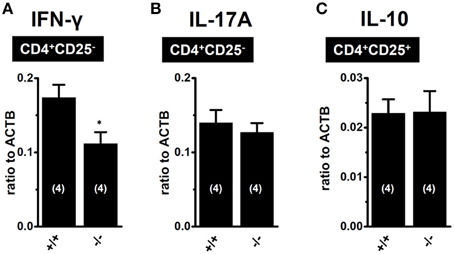
Figure 5. Expression levels of IFN-γ and IL-17A transcripts in CD4+CD25− subset of IBD models from K2P5.1+/+ (+/+) and K2P5.1−/− (-/-) mice (A,B), and IL-10 transcripts CD4+CD25+ subset of IBD models from K2P5.1+/+ (+/+) and K2P5.1−/− mice (-/-) (C). Expression levels were measured using a real-time PCR assay. Results were expressed as means ± SE. Numbers used for the experiments are shown in parentheses. *P < 0.01 vs. K2P5.1+/+ mice.
Regulatory T (Treg) cells play an important role in the maintenance of intestinal homeostasis, and Treg cells are considered to be beneficial for IBD therapy (Boden and Snapper, 2008; Himmel et al., 2012; Gibson et al., 2013; Mayne and Williams, 2013). As shown in Figure 3A, the expression levels of K2P5.1 transcripts of K2P5.1 were significantly increased in the splenic CD4+CD25+ T cells of IBD model mice. This subset also included Foxp3-positive cells (not shown) and, thus, is referred to as naturally-occurring regulatory T cells. In B lymphocytes, the production of the anti-inflammatory cytokine IL-10 was shown to be stimulated by Ca2+ influx via the CRAC channel (Matsumoto et al., 2011; Baba et al., 2014). Therefore, the upregulation of K2P5.1 may strengthen the production of IL-10 in the natural Treg cells of IBD model mice, and the activation of K2P5.1 may improve the pathogenesis of IBD. In the present study, the expression of IL-10 was significantly higher in the natural Treg cells of IBD model mice than in those of control mice (Figure 3E). No significant changes in the expression of IL-10 were found in natural Treg cells of the IBD model from K2P5.1−/− mice (Figure 5C); however, the long-lasting dysregulation of Ca2+ signaling elicited by a K2P5.1 deficiency may be compensated by the upregulation of other K+ channel(s), and, thus, the expression of IL-10 may be maintained within normal ranges in the Treg cells of the IBD model from K2P5.1−/− mice. Further studies are needed in order to clarify the pathophysiological role of K2P5.1 in the natural Treg cells of IBD.
In conclusion, the results of the present study suggested that the background K+ channel K2P5.1 in splenic CD4+ T cells was involved in the pathogenesis of IBD using a chemically-induced IBD model and provided evidence for the K2P5.1 K+ channel as a potential therapeutic target for suppressing the progression of IBD. Dysregulated K2P5.1 may stimulate the Th1 imbalance in the process of intestinal inflammation. The lack of selective K2P5.1 blockers may facilitate future research on a novel high-throughput screening system to detect small molecule K2P5.1 modulators and the regulatory mechanisms underlying K2P5.1 transcription, translation, and protein degradation in IBD.
Author Contributions
SN, HK, and SO participated in research design. SN, MM, HK, MF, and SO conducted molecular biological and biochemical experiments and performed data analyses. SN, MM, AS, MI, and HK conducted fluorescence imaging experiments and performed data analyses. SN, HN, SM, MM conducted H&E staining experiments and performed data analyses. SN, AS, HK, EK, SM, MM, NK, MA, KA, and SO contribute to the maintenance of knockout mice and preparation of IBD model mice. SN, HK, and SO contributed to the writing of the manuscript.
Funding
This work was supported by a Grant-in Aid for Scientific Research (C) (No. 25460111) by The Japan Society for the Promotion of Science (JSPS), a grant for the Supported Program for the Strategic Research Foundation at Private Universities, 2013-2017 (S1311035) from the Ministry of Education, Culture, Sports, Science and Technology (MEXT), the Mochida Memorial Foundation for Medical and Pharmaceutical Research, and the Uehara Memorial Foundation (to SO), a research grant from The Promotion and Mutual Aid Cooperation for Private Schools of Japan (Kyoto Pharmaceutical University and Aichi-Gakuin University).
Conflict of Interest Statement
The authors declare that the research was conducted in the absence of any commercial or financial relationships that could be construed as a potential conflict of interest.
Acknowledgments
We thank to Sayo Matsuba and Toshifumi Sato for their technical assistance. K2P5.1 heterozygous knockout mice [B6;CB-Kcnk5Gt(pU-21)81Imeg] were provided from the Center for Animal Resource and Development (CARD) (Kumamoto University, Kumamoto, Japan). Medical English Service (Kyoto, Japan) reviewed the manuscript prior to its submission.
Supplementary Material
The Supplementary Material for this article can be found online at: http://journal.frontiersin.org/article/10.3389/fphys.2015.00299
Abbreviations
K2P, two-pore domain K+ (channel); TASK, TWIK-related acid-sensitive K+ (channel); TWIK, tandem of P-domains in a weakly inward rectifying K+ (channel); TALK, TWIK-related alkaline pH-activated K+ (channel); IBD, inflammatory bowel disease; DSS, dextran sodium sulfate; HEPES, 4-(2-hydroxyethyl)-1-piperazineethanesulfonic acid; DiBAC4(3), bis-(1,3-dibutylbarbituric acid)trimethine oxonol; Fura 2-AM, acetoxymethyl 2-[5-[bis[(acetoxymethoxy-oxo-methyl)methyl]amino]-4-[2-[2-[bis[(acetoxymethoxy-oxo-methyl)methyl]amino]-5-methyl-phenoxy]ethoxy]benzofuran-2-yl]oxazole-5-carboxylate; FCM, flow cytometry; IFN, interferon; IL, interleukin; pHe, extracellular pH; CD, cluster of differentiation or Crohn's disease; UC, ulcerative colitis; FITC, fluorescein isothiocyanate; PE, Phycoerythrin; 2-APB, 2-aminoethoxydiphenyl borate; Orai, Ca2+ release-activated Ca2+ (channel); STIM, stromal interaction molecule; TRPV, transient receptor potential, vanilloid; TRPA, transient receptor potential, subfamily A; KCa, Ca2+-activated K+ (channel); KV, voltage-gated K+ (channel).
References
Andronic, J., Bobak, N., Bittner, S., Ehling, P., Kleinschnitz, C., Herrmann, A. M., et al. (2013). Identification of two-pore domain potassium channels as potent modulators of osmotic volume regulation in human T lymphocytes. Biochim. Biophys. Acta 1828, 699–707. doi: 10.1016/j.bbamem.2012.09.028
Araki, M., Nakahara, M., Muta, M., Itou, M., Yanai, C., Yamazoe, F., et al. (2014). Database for exchangeable gene trap clones: pathway and gene ontology analysis of exchangeable gene trap clone mouse lines. Dev. Growth Differ. 56, 161–174. doi: 10.1111/dgd.12116
Baba, Y., Matsumoto, M., and Kurosaki, T. (2014). Calcium signaling in B cells: regulation of cytosolic Ca2+ increase and its sensor molecules, STIM1 and STIM2. Mol. Immunol. 62, 3339–3343. doi: 10.1016/j.molimm.2013.10.006
Bagriantsev, S. N., Ang, K. H., Gallardo-Godoy, A., Clark, K. A., Arkin, M. R., Renslo, A. R., et al. (2013). A high-throughput functional screen identifies small molecule regulators of temperature- and mechano-sensitive K2P channels. ACS Chem. Biol. 8, 1841–1851. doi: 10.1021/cb400289x
Beck, A., Fleig, A., Penner, R., and Peinelt, C. (2014). Regulation of endogenous and heterologous Ca2+ release-activated Ca2+ currents by pH. Cell Calcium 56, 235–243. doi: 10.1016/j.ceca.2014.07.011
Bertin, S., Aoki-Nonaka, Y., de Jong, P. R., Nohara, L. L., Xu, H., Stanwood, S. R., et al. (2014). The ion channel TRPV1 regulates the activation and proinflammatory properties of CD4+ T cells. Nat. Immunol. 15, 1055–1063. doi: 10.1038/ni.3009
Bittner, S., Bobak, N., Feuchtenberger, M., Herrmann, A. M., Göbel, K., Kinne, R. W., et al. (2011). Expression of K2P5.1 potassium channels on CD4+ T lymphocytes correlates with disease activity in rheumatoid arthritis patients. Arthritis Res. Ther. 13:R21. doi: 10.1186/ar3245
Bittner, S., Bobak, N., Herrmann, A. M., Göbel, K., Meuth, P., Höhn, K. G., et al. (2010a). Upregulation of K2P5.1 potassium channels in multiple sclerosis. Ann. Neurol. 68, 58–69. doi: 10.1002/ana.22010
Bittner, S., Budde, T., Wiendl, H., and Meuth, S. G. (2010b). From the background to the spotlight: TASK channels in pathological conditions. Brain Pathol. 20, 999–1009. doi: 10.1111/j.1750-3639.2010.00407.x
Bobak, N., Bittner, S., Andronic, J., Hartmann, S., Mühlpfordt, F., Schneider-Hohendorf, T., et al. (2011). Volume regulation of murine T lymphocytes relies on voltage-dependent and two-pore domain potassium channels. Biochim. Biophys. Acta 1808, 2036–2044. doi: 10.1016/j.bbamem.2011.04.013
Boden, E. K., and Snapper, S. B. (2008). Regulatory T cells in inflammatory bowel disease. Curr. Opin. Gastroenterol. 24, 733–741. doi: 10.1097/MOG.0b013e328311f26e
Bouma, G., and Strober, W. (2003). The immunological and genetic basis of inflammatory bowel disease. Nat. Rev. Immunol. 3, 521–533. doi: 10.1038/nri1132
Cahalan, M. D., and Chandy, K. G. (2009). The functional network of ion channels in T lymphocytes. Immunol. Rev. 231, 59–87. doi: 10.1111/j.1600-065X.2009.00816.x
Cid, L. P., Roa-Rojas, H. A., Niemeyer, M. I., Gonzalez, W., Araki, M., and Sepulveda, F. V. (2013). TASK-2: a K2P K+ channel with complex regulation and diverse physiological functions. Front. Physiol. 4:198. doi: 10.3389/fphys.2013.00198
Clark, R. B., Kondo, C., Belke, D. D., and Giles, W. R. (2011). Two-pore domain K+ channels regulate membrane potential of isolated human articular chondrocytes. J. Physiol. (Lond). 589, 5071–5089. doi: 10.1113/jphysiol.2011.210757
Di, L., Srivastava, S., Zhdanova, O., Ding, Y., Li, Z., Wulff, H., et al. (2010). Inhibition of the K+ channel KCa3.1 ameliorates T cell-mediated colitis. Proc. Natl. Acad. Sci. U.S.A. 10, 1541–1546. doi: 10.1073/pnas.0910133107
Dieleman, L. A., Ridwan, B. U., Tennyson, G. S., Beagley, K. W., Bucy, R. P., and Elson, C. O. (1994). Dextran sulfate sodium-induced colitis occurs in severe combined immunodeficient mice. Gastroenterology 107, 1643–1652.
Di Sabatino, A., Rovedatti, L., Kaur, R., Spencer, J. P., Brown, J. T., Morisset, V. D., et al. (2009). Targeting gut T cell Ca2+ release-activated Ca2+ channels inhibits T cell cytokine production and T-box transcription factor T-bet in inflammatory bowel disease. J. Immunol. 183, 3454–3462. doi: 10.4049/jimmunol.0802887
Enyedi, P., and Czirják, G. (2010). Molecular background of leak K+ currents: two-pore domain potassium channels. Physiol. Rev. 90, 559–605. doi: 10.1152/physrev.00029.2009
Es-Salah-Lamoureux, Z., Steele, D. F., and Fedida, D. (2010). Research into the therapeutic roles of two-pore-domain potassium channels. Trends Pharmacol. Sci. 31, 587–595. doi: 10.1016/j.tips.2010.09.001
Fang, K., Bruce, M., Pattillo, C. B., Zhang, S., Stone, R. II, Clifford, J., et al. (2011). Temporal genome wide expression profiling of DSS colitis reveals novel inflammatory and angiogenesis genes similar to ulcerative colitis. Physiol. Genomics 43, 43–56. doi: 10.1152/physiolgenomics.00138.2010
Gibson, D. J., Ryan, E. J., and Doherty, G. A. (2013). Keeping the bowel regular: the emerging role of Treg as a therapeutic target in inflammatory bowel disease. Inflamm. Bowel Dis. 19, 2716–2724. doi: 10.1097/MIB.0b013e31829ed7df
Himmel, M. E., Yao, Y., Orban, P. C., Steiner, T. S., and Levings, M. K. (2012). Regulatory T-cell therapy for inflammatory bowel disease: more questions than answers. Immunology 136, 115–122. doi: 10.1111/j.1365-2567.2012.03572.x
Hogan, P. G., Lewis, R. S., and Rao, A. (2010). Molecular basis of calcium signaling in lymphocytes: STIM and ORAI. Annu. Rev. Immunol. 38, 491–533. doi: 10.1146/annurev.immunol.021908.132550
Kun, J., Szitter, I., Kemény, A., Perkecz, A., Kereskai, L., Pohóczky, K., et al. (2014). Upregulation of the transient receptor potential anlyrin 1 ion channel in the inflamed human and mouse colon and its protective roles. PLoS ONE 9:e108164. doi: 10.1371/journal.pone.0108164
Matsumoto, M., Fujii, Y., Baba, A., Hikida, M., Kurosaki, T., and Baba, Y. (2011). The calcium sensors STIM1 and STIM2 control B cell regulatory function through interleukin-10 production. Immunity 34, 703–714. doi: 10.1016/j.immuni.2011.03.016
Mayne, C. G., and Williams, C. B. (2013). Induced and natural regulatory T cells in the development of inflammatory bowel disease. Inflamm. Bowel Dis. 19, 1772–1788. doi: 10.1097/mib.0b013e318281f5a3
McCole, D. F. (2014). IBD candidate genes and intestinal barrier regulation. Inflamm. Bowel Dis. 20, 1829–1849. doi: 10.1097/MIB.0000000000000090
Nam, J. H., Shin, D. H., Zheng, H., Lee, D. S., Park, S. J., Park, K. S., et al. (2011). Expression of TASK-2 and its upregulation by B cell receptor stimulation in WEHI-231 mouse immature B cells. Am. J. Physiol. Cell Physiol. 300, C1013–C1022. doi: 10.1152/ajpcell.00475.2010
Niemeyer, M. I., Cid, L. P., Barros, L. F., and Sepúlveda, F. V. (2001). Modulation of the two-pore domain acid sensitive K+ channel TASK-2 (KCNK5) by changes in cell volume. J. Biol. Chem. 276, 43166–43174. doi: 10.1074/jbc.M107192200
Ohya, S., Fukuyo, Y., Kito, H., Shibaoka, R., Matsui, M., Niguma, H., et al. (2014). Upregulation of KCa3.1 K+ channel in mesenteric lymph node CD4+ T lymphocytes from a mouse model of dextran sodium sulfate-induced inflammatory bowel disease. Am. J. Physiol. Gastrointest. Liver Physiol. 306, G873–G885. doi: 10.1152/ajpgi.00156.2013
Ohya, S., and Imaizumi, Y. (2014). Intermediate-conductance Ca2+-activated K+ channel KCa3.1 and its related molecules in T-lymphocytes. Inflamm. Cell Signal. 1:e327. doi: 10.14800/ics.327
Ohya, S., Nakamura, E., Horiba, S., Kito, H., Matsui, M., Yamamura, H., et al. (2013). Role of the KCa3.1 K+ channel in auricular lymph node CD4+ T-lymphocyte function of the delayed-type hypersensitivity model. Br. J. Pharmacol. 169, 1011–1023. doi: 10.1111/bph.12215
Okayasu, I., Hatakeyama, S., Yamada, M., Ohkusa, T., Inagaki, Y., and Nakaya, R. (1990). A novel method in the induction of reliable experimental acute and chronic ulcerative colitis in mice. Gastroenterology 98, 694–702.
Pereira, J. L., Hughes, L. E., and Young, H. L. (1987). Spleen size in patients with inflammatory bowel disease. Does it have any clinical significance? Dis. Colon Rectum 30, 403–409. doi: 10.1007/BF02556485
Shin, D. H., Lin, H., Zheng, H., Kim, K. S., Kim, J. Y., Chun, Y. S., et al. (2014). HIF-1α-mediated upregulation of TASK-2 K+ channels augments Ca2+ signaling in mouse B cells under hypoxia. J. Immunol. 193, 4924–4933. doi: 10.4049/jimmunol.1301829
Vig, M., and Kinet, J. P. (2009). Calcium signaling in immune cells. Nat. Immunol. 10, 21–27. doi: 10.1038/ni.f.220
Keywords: background K+ channel, K2P5.1, CD4+ T cell, inflammatory bowel disease, Ca2+ influx, cytokine production
Citation: Nakakura S, Matsui M, Sato A, Ishii M, Endo K, Muragishi S, Murase M, Kito H, Niguma H, Kurokawa N, Fujii M, Araki M, Araki K and Ohya S (2015) Pathophysiological significance of the two-pore domain K+ channel K2P5.1 in splenic CD4+CD25− T cell subset from a chemically-induced murine inflammatory bowel disease model. Front. Physiol. 6:299. doi: 10.3389/fphys.2015.00299
Received: 26 May 2015; Accepted: 09 October 2015;
Published: 27 October 2015.
Edited by:
Mauricio Antonio Retamal, Universidad del Desarrollo, ChileReviewed by:
Péter Béla Hajdu, University of Debrecen, HungaryPhanindra Velisetty, University of Tennessee Health Science Center, USA
Copyright © 2015 Nakakura, Matsui, Sato, Ishii, Endo, Muragishi, Murase, Kito, Niguma, Kurokawa, Fujii, Araki, Araki and Ohya. This is an open-access article distributed under the terms of the Creative Commons Attribution License (CC BY). The use, distribution or reproduction in other forums is permitted, provided the original author(s) or licensor are credited and that the original publication in this journal is cited, in accordance with accepted academic practice. No use, distribution or reproduction is permitted which does not comply with these terms.
*Correspondence: Susumu Ohya, c29oeWFAbWIua3lvdG8tcGh1LmFjLmpw