- 1Department of Nephrology and Central Laboratory, Putuo Hospital, Shanghai University of Traditional Chinese Medicine, Shanghai, China
- 2Department of Pharmacology, School of Pharmacy, Fudan University, Shanghai, China
Large-conductance calcium-activated potassium (BK) channels are currently considered as vital players in a variety of renal physiological processes. In podocytes, BK channels become active in response to stimuli that increase local cytosolic Ca2+, possibly secondary to activation of slit diaphragm TRPC6 channels by chemical or mechanical stimuli. Insulin increases filtration barrier permeability through mobilization of BK channels. In mesangial cells, BK channels co-expressed with β1 subunits act as a major component of the counteractive response to contraction in order to regulate glomerular filtration. This review aims to highlight recent discoveries on the localization, physiological and pathological roles of BK channels in glomerulus.
Introduction
BK channels, also known as Maxi-K because of the large single-channel conductance (>200 pS in 100 mmol/L symmetrical K+), constitute a particular family of ion channels, which couple intracellular chemical signaling to membrane electrical signaling (Rothberg, 2012; Contreras et al., 2013). BK channels distributing in excitable as well as non-excitable cells are considered as key participants in a lot of physiological functions, including regulating neuronal firing, smooth muscle tone, endocrine cell secretion, cell proliferation and migration (Contreras et al., 2013).
Functional BK channels are homotetramers made up of pore-forming α subunits which are encoded by a single gene (Slo1 or Kcnma1). Distinct from homologous voltage-gated K+ (Kv) channels, BK α subunit possesses an additional hydrophobic segments containing a N-terminal transmembrane helix (S0) at the extracellular side of the plasma membrane as well as a long cytosolic C-terminal (S7–S10) which is comprised of two RCK (regulator of K+ conductance) domains and calcium bowl where putative Ca2+-binding sites reside (Yuan et al., 2010). Cooperating with tissue-specific auxiliary β (β1-4) and γ (γ1-4) subunits, BK channels are divided into several subfamilies and play specific roles in physiological actions of different organizations. The auxiliary subunits are responsible for modulating the kinetic behavior and Ca2+ sensitivity of BK as well as pharmacological responses to BK specific modulators (Tao et al., 2011; Contreras et al., 2013; Table 1).
In addition to their physiological functions, BK channels are also involved in a series of diseases such as hypertension, epilepsy, cancer and so on (Contreras et al., 2013). Recently, BK channel subtypes have been discovered in podocytes and mesangial cells (Morton et al., 2004; Ma et al., 2005; Piwkowska et al., 2015). In this review, we summarize the latest progress on the expression, function and regulatory mechanism of BK channel subtypes in glomerulus and evaluate the feasibility of therapeutical BK-specific ligands for chronic kidney disease (CKD).
BK Channels in Glomerular Podocytes
Podocytes, one of highly specialized cells encircling glomerular capillaries, extend the foot processes (or pedicels) forming finger-like projections, referred to as the slit diaphragm, interdigitate with that of neighboring cells. The sophisticated structure comprises an important component of the glomerular-filtration-barrier. The slit diaphragm containing cytoskeletal proteins and ion channels, such as nephrin, podocin, Neph1, F-actin, TRPC6, and BK channels, regulates podocyte function and cell body in response to fluid tresses (Dryer and Reiser, 2010; Lennon et al., 2014; Piwkowska et al., 2015).
Molecular Properties of Podocyte BK
BK channels and β subunits have been successively discovered expressed in mouse, rat and human podocytes (Morton et al., 2004; Dryer and Reiser, 2010; Piwkowska et al., 2015). Podocyte BK channels with slow kinetics and large conductance were observed in whole-cell as well as single-channel recordings readily, which was partially inhibited by iberiotoxin (IbTX), but completely blocked by paxilline and penetrem A (Morton et al., 2004; Kim et al., 2008). Iberiotoxin-insensitive BK (α+β4) is probably the main subtype in podocytes supported by the evidence above. It has been suggested that podocyte BK channels could be activated in response to membrane stretch at the positive potentials and high level of intracellular Ca2+. The opening probability of BK grew fourfold by applying modest negative pressure or decreasing the tonicity of bath solution (Morton et al., 2004). However, it is still unclear the mechanism underlying the activation of BK under physiological conditions, which is possibly associated with SD proteins of podocyte.
Interaction of Podocyte BK with SD Proteins and TRPC6
SD proteins (Figure 1A), interacting with BK C-terminal, include nephrin (Kim et al., 2008), Neph1 (Kim et al., 2009b), MAGI-1 (Ridgway et al., 2009), and synaptopodin (Kim et al., 2010). Down-regulating the expression of nephrin or synaptopodin decreases the number of functional surface BK channels in mouse podocytes (Kim et al., 2008, 2010). Co-expression of synaptopodin or nephrin with Slo1 increases surface expression of BK channels in HEK293T, which is the possible mechanism underlying the increment of BK protein expression during the maturity of podocytes (Yang et al., 2013). On the contrary, down-regulation of MAGI-1 mediates an increment in functional surface expression of BK in podocytes, co-expression of MAGI-1 suppresses surface expression of BK on the HEK293T cells (Ridgway et al., 2009). The situation with Neph1 is complicated because its regulation on BK surface expression depends on the cell type (Kim et al., 2009b). However, none of the proteins mentioned above facilitate the activation of BK channels. Thus, it is strongly suggested two possibilities for the activation of BK in non-excitable podocytes. One is SD proteins interacting with β subunits, the other is a stretch-dependent increment in intercellular Ca2+ concentration, for example BK interacting with mechanosensitive TRPC6 of which the stretch-evoked activation was markly regulated by podocin (Anderson et al., 2013).
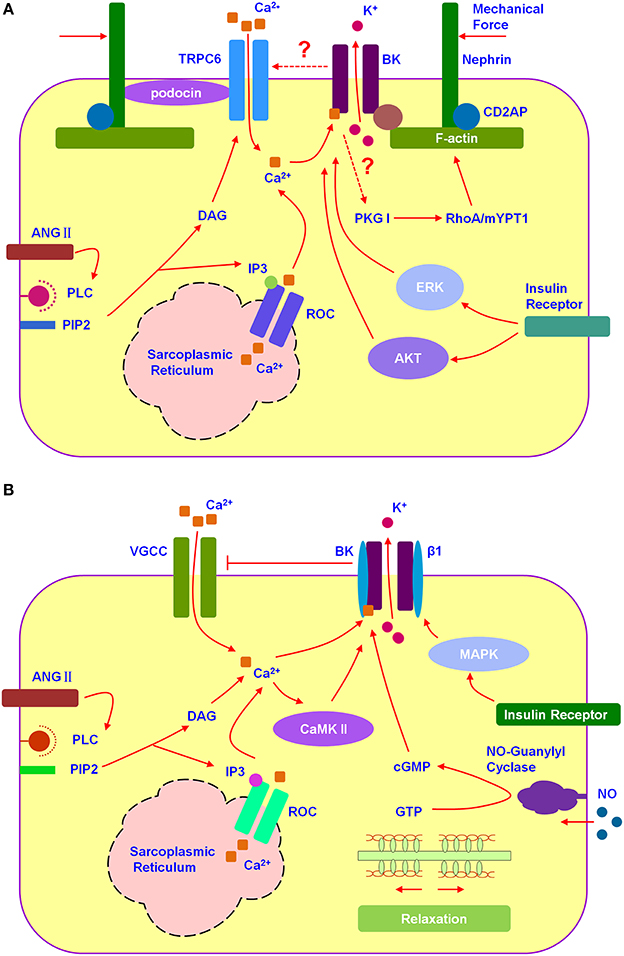
Figure 1. BK channels participated in the signal integration of podocytes and mesangial cells. (A) Hypothesized relation among TRPC6, BK, and SDs in podocyte. Podocyte BK participated in the cytoskeleton-related signal integration. (B) Hypothesized relation among VGCC and BK in mesangial cell. Mesangial BK participated in the signal integration of mesangial cell relaxion.
TRPC6 (Figure 1A), one of the non-selective cation channels, has been demonstrated co-expressing with BK channels and promoting its surface expression in podocytes (Kim et al., 2009a). TRPC6 permeates Ca2+ depending on the membrane hyperpolarization (Estacion et al., 2006). BK could be activated by TRPC6-induced calcium influx as well as membrane depolarization. Then, BK-caused membrane hyperpolarization provides positive feedback to TRPC6. Gain-of-function mutations in TRPC6 have been discovered, which mediate inherited glomerular disease such as focal segmental glomerulosclerosis (FSGS) (Reiser et al., 2005; Winn et al., 2005). Because of the close structure between each TRPCs member, there is still no specific blockers for TRPC6 (Dryer and Reiser, 2010). BK blockers seem to be an ideal way to control the glomerular sclerosis induced by TRPC6 hyperfunction. Among all, martentoxin, a selective blocker for podocyte BK (α+β4), is worthy of in-depth study for anti-FSGS (Tao et al., 2014).
Regulation of Hormones and Pathological Environment on Podocyte BK
Growing evidences have suggested that several hormones (Figure 1A), such as angiotensin II and insulin, as well as pathological environment regulating BK expression and function playing important roles in podocyte injury (Kim and Dryer, 2011; Gao et al., 2015; Piwkowska et al., 2015). Angiotensin II (Ang II) which could induce the oxidative stress and podocyte death not only inhibits the current amplitude of Podocyte BK, but also facilitates the BK activation (Gao et al., 2015). Insulin increases cell surface expression of podocyte BK channels, with accompanied by a corresponding increase in the current density, via ERK (extracellular signal-regulated kinase) and AKT (PKB, protein kinase B) signaling cascades. While, high glucose treatment decreases the number of functional surface BK channels and nephrin as well as abolishes the stimulatory effects of insulin on BK (Kim and Dryer, 2011). Podocyte BK is also considered as a key player mediating insulin-increased filtration barrier permeability along with PKGI-dependent transepithelial albumin flux through participating in the disruption of the actin cytoskeleton induced by insulin. IbTX blocked insulin-induced disruption of the actin cytoskeleton as well as inhibited the phosphorylation of PKG target proteins, RhoA and MYPT1 (Piwkowska et al., 2015). The exposure of podocytes to hypoxia environment caused an obvious reduction in BK currents and shifted BK activation range toward more depolarized potential and slowed its activation kinetics via increased BK β4-subunits expression (Zhang et al., 2012).
BK Channels in Glomerular Mesangial Cells
Mesangial cells (MC) in glomerulus have been proved participating in many physiological activities, such as producing growth factors, forming mesangial matrix as a structural support for capillaries and modulating glomerular hemodynamics through contractile properties. Confronted with glomerular injury induced by inflammation or hypertension, MCs often changes its phenotype as myofibroblasts expressing α-smooth muscle actin or interstitial collagens in addition to normal matrix constituents (Ma et al., 2005).
Molecular Properties and Cell Function of Mesangial BK
MCs have a lot of properties in common with smooth muscle cells, mainly expressing BK as well as VGCCs (voltage-gated calcium channels) (Ma et al., 2005; Figure 1B). Different from BK in podocyte, BK in MCs acts as a “brake” on Ca2+ signal. The activation of BK promotes membrane hyperpolarization, which provides negative feedback to VGCCs and the counteractive response to contraction of mesangial cell (Stockand and Sansom, 1996). Mesangial BK was composed by α and β1 subunit which has been shown to increase the sensitivity of BK to intercellular Ca2+ significantly (Kudlacek et al., 2003). MCs relax to elevate glomerular filtration rate (GFR) when the body is volume expanded. BK-β1 knockout mice have been observed a normal GFR under basal conditions, but they fail to elevate their GFR to the same extent as wild-type mice upon volume expansion (Pluznick et al., 2003). It has been demonstrated that BK-β1 gain-of-function variant-Glu65Lys could obviously influence GFR. From clinical investigation, the 65Lys carriers exhibit not only elevated baseline GFR, but also decline GFR more rapidly in CKD (Chen et al., 2010). Evidences above suggest that the BK (α+β1) is a key regulator for the tone of MCs. BK openers depending on the presence of β1 subunits have the potential to be a useful scaffold in the development of drugs for regulating GFR, for example newly discovered GoSlo-SR family (Large et al., 2015).
Besides VGCCs, store-operated Ca2+ (SOC) channels, including Orai1, are also functional expressed in MCs (Sours-Brothers et al., 2009; Wu et al., 2015). Orai1 could negatively regulate the expression of extracellular matrix protein, such as fibronectin and collagen IV, as well as mesangial expansion in the renal cortex (Wu et al., 2015). In recent years, store-operated Ca2+ channels have been reported to be associated with BK in many cells (Gueguinou et al., 2014). In contrast to VGCCs, Mesangial BK probably provides positive feedback to SOC channels and plays a reno-protective role in glomerular disease. However, there is no direct evidence that SOC channels are combined with BK in mesangial cells so far.
Regulation of Signaling Molecules on Mesangial BK
Signaling molecules activated BK could also be considered as ideal drug targets for regulating GFR. In MCs, pathways including protein kinase, hormones and gases are involved in regulating BK function (Figure 1B). It has been proved through patch clamping that BK could be activated by cGMP and PKG as well as by soluble gas and guanylyl cyclase stimulators such as atrial natriuretic peptide (ANP) and nitric oxide (NO), respectively (Ma et al., 2005). Insulin activates mesangial BK and upregulates BK expression not only in cellular but also in plasma membrane via MAPK pathway (Foutz et al., 2008). Ca2+/calmodulin-dependent kinase II (CaMKII) acts as an endogenous agonist to expand the Ang II-induced activation of BK and cause a feedback to MCs contraction (Sansom et al., 2000).
Concluding Remarks and Perspectives
In glomerular podocytes, BK channels, interacting with most SD proteins, participate in the increase of filtration barrier permeability induced by insulin as well as the remodeling pathway of cytoskeleton protein. β4 subunit is an important auxiliary subunit which controls the surface expression, dynamic characteristics and drug sensitivity of podocyte BK channels. BK channels, consisting of α and β4, could be used as a novel target for the treatment of glomerular disease. However, there are still a lot of mysteries of podocyte BK channels in suspense, (1) inactivation mechanism underlying the positive-feedback regulation of BK on Ca2+ signal in podocytes, (2) regulation of BK channel on the cytoskeleton of podocytes, (3) the reason why angiotensin II activated TRPC6, but inhibited the BK.
In glomerular mesangial cells, BK channels, co-expressed with β1, act as a major component of the counteractive response to contraction through negative-feedback regulating VGCCs. Therefore, specific openers of BK (α+β1) channels are expected to become the potential drugs for renal glomerular disease. It is a pity that suitable openers for BK (α+β1) haven't been found so far. Perhaps, agonists for cGMP and CaMKII pathway will become another way to solve this difficulty.
At last, because BK channels are widely expressed in most cells and tissues, the drugs designed for BK-related disease should be pay more attention to tissue-specific and BK subtype-specific properties in order to avoid the safety issues.
Author Contributions
Conceived the paper: XZ and WeP. Wrote the paper: JT, ZL, and YW. Drew the figure: JT. Revised the draft: HH, LT, and WaP.
Funding
This work was supported by National Science Foundation of China (No.81370979 and 81573478), Shanghai Science and Technology Innovation Grant (No.14140903202), Key Medical Discipline project of Shanghai Municipal Health Bureau (No.ZK2012A34), Innovation Program of Shanghai Municipal Education Commission (No.15ZZ063), Shanghai Municipal Commission of Health and Family Planning Fund for Young Scholars (No. 20134050) and Research Project of Putuo Hospital, Shanghai University of Traditional Chinese Medicine, China (No. 2014YJ002).
Conflict of Interest Statement
The authors declare that the research was conducted in the absence of any commercial or financial relationships that could be construed as a potential conflict of interest.
Abbreviations
BK, Large-conductance calcium-activated potassium channels; Kv, voltage-gated K+ channels; CKD, chronic kidney disease; HEK, human embryonic kidney; SD, slit diaphragm; IbTX, iberiotoxin; TRPC6, transient receptor potential canonical 6; FSGS, focal segmental glomerulosclerosis; Ang II, Angiotensin II; ERK, extracellular signal-regulated kinase; PKB, protein kinase B; PKG, cGMP-dependent protein kinase; MYPT1, myosin phosphatase target subunit 1; MC, Mesangial cells; VGCCs, voltage-gated Ca2+ channels; GFR, glomerular filtration rate; SOC, store-operated Ca2+ channels; ANP, atrial natriuretic peptide; NO, nitric oxide; MAPK, mitogen-activated protein kinase; CaMKII, calmodulin-dependent kinase II.
References
Anderson, M., Kim, E. Y., Hagmann, H., Benzing, T., and Dryer, S. E. (2013). Opposing effects of podocin on the gating of podocyte TRPC6 channels evoked by membrane stretch or diacylglycerol. Am. J. Physiol. Cell Physiol. 305, C276–C289. doi: 10.1152/ajpcell.00095.2013
Chen, Y., Salem, R. M., Rao, F., Fung, M. M., Bhatnagar, V., Pandey, B., et al. (2010). Common charge-shift mutation Glu65Lys in K+ channel beta(1)-Subunit KCNMB1: pleiotropic consequences for glomerular filtration rate and progressive renal disease. Am. J. Nephrol. 32, 414–424. doi: 10.1159/000320131
Contreras, G. F., Castillo, K., Enrique, N., Carrasquel-Ursulaez, W., Castillo, J. P., Milesi, V., et al. (2013). A BK (Slo1) channel journey from molecule to physiology. Channels 7, 442–458. doi: 10.4161/chan.26242
Dryer, S. E., and Reiser, J. (2010). TRPC6 channels and their binding partners in podocytes: role in glomerular filtration and pathophysiology. Am. J. Physiol. Renal Physiol. 299, F689–F701. doi: 10.1152/ajprenal.00298.2010
Estacion, M., Sinkins, W. G., Jones, S. W., Applegate, M. A., and Schilling, W. P. (2006). Human TRPC6 expressed in HEK 293 cells forms non-selective cation channels with limited Ca2+ permeability. J. Physiol. 572, 359–377. doi: 10.1113/jphysiol.2005.103143
Foutz, R. M., Grimm, P. R., and Sansom, S. C. (2008). Insulin increases the activity of mesangial BK channels through MAPK signaling. Am. J. Physiol. Renal Physiol. 294, F1465–F1472. doi: 10.1152/ajprenal.00012.2008
Gao, N., Wang, H., Zhang, X., and Yang, Z. (2015). The inhibitory effect of angiotensin II on BKCa channels in podocytes via oxidative stress. Mol. Cell. Biochem. 398, 217–222. doi: 10.1007/s11010-014-2221-1
Guéguinou, M., Chantome, A., Fromont, G., Bougnoux, P., Vandier, C., and Potier-Cartereau, M. (2014). KCa and Ca(2+) channels: the complex thought. Biochim. Biophys. Acta 1843, 2322–2333. doi: 10.1016/j.bbamcr.2014.02.019
Kim, E. Y., Alvarez-Baron, C. P., and Dryer, S. E. (2009a). Canonical transient receptor potential channel (TRPC)3 and TRPC6 associate with large-conductance Ca2+-activated K+ (BKCa) channels: role in BKCa trafficking to the surface of cultured podocytes. Mol. Pharmacol. 75, 466–477. doi: 10.1124/mol.108.051912
Kim, E. Y., Chiu, Y. H., and Dryer, S. E. (2009b). Neph1 regulates steady-state surface expression of Slo1 Ca(2+)-activated K(+) channels: different effects in embryonic neurons and podocytes. Am. J. Physiol. Cell Physiol. 297, C1379–C1388. doi: 10.1152/ajpcell.00354.2009
Kim, E. Y., Choi, K. J., and Dryer, S. E. (2008). Nephrin binds to the COOH terminus of a large-conductance Ca2+-activated K+ channel isoform and regulates its expression on the cell surface. Am. J. Physiol. Renal Physiol. 295, F235–F246. doi: 10.1152/ajprenal.00140.2008
Kim, E. Y., and Dryer, S. E. (2011). Effects of insulin and high glucose on mobilization of slo1 BKCa channels in podocytes. J. Cell. Physiol. 226, 2307–2315. doi: 10.1002/jcp.22567
Kim, E. Y., Suh, J. M., Chiu, Y. H., and Dryer, S. E. (2010). Regulation of podocyte BK(Ca) channels by synaptopodin, Rho, and actin microfilaments. Am. J. Physiol. Renal Physiol. 299, F594–F604. doi: 10.1152/ajprenal.00206.2010
Kudlacek, P. E., Pluznick, J. L., Ma, R., Padanilam, B., and Sansom, S. C. (2003). Role of hbeta1 in activation of human mesangial BK channels by cGMP kinase. Am. J. Physiol. Renal Physiol. 285, F289–F294. doi: 10.1152/ajprenal.00046.2003
Large, R. J., Kshatri, A., Webb, T. I., Roy, S., Akande, A., Bradley, E., et al. (2015). Effects of the novel BK (KCa 1.1) channel opener GoSlo-SR-5-130 are dependent on the presence of BKbeta subunits. Br. J. Pharmacol. 172, 2544–2556. doi: 10.1111/bph.13085
Lennon, R., Randles, M. J., and Humphries, M. J. (2014). The importance of podocyte adhesion for a healthy glomerulus. Front. Endocrinol. 5:160. doi: 10.3389/fendo.2014.00160
Ma, R., Pluznick, J. L., and Sansom, S. C. (2005). Ion channels in mesangial cells: function, malfunction, or fiction. Physiology (Bethesda) 20, 102–111. doi: 10.1152/physiol.00050.2004
Morton, M. J., Hutchinson, K., Mathieson, P. W., Witherden, I. R., Saleem, M. A., and Hunter, M. (2004). Human podocytes possess a stretch-sensitive, Ca2+-activated K+ channel: potential implications for the control of glomerular filtration. J. Am. Soc. Nephrol. 15, 2981–2987. doi: 10.1097/01.ASN.0000145046.24268.0D
Piwkowska, A., Rogacka, D., Audzeyenka, I., Kasztan, M., Angielski, S., and Jankowski, M. (2015). Insulin increases glomerular filtration barrier permeability through PKGIalpha-dependent mobilization of BKCa channels in cultured rat podocytes. Biochim. Biophys. Acta 1852, 1599–1609. doi: 10.1016/j.bbadis.2015.04.024
Pluznick, J. L., Wei, P., Carmines, P. K., and Sansom, S. C. (2003). Renal fluid and electrolyte handling in BKCa-beta1-/- mice. Am. J. Physiol. Renal Physiol. 284, F1274–F1279. doi: 10.1152/ajprenal.00010.2003
Reiser, J., Polu, K. R., Moller, C. C., Kenlan, P., Altintas, M. M., Wei, C., et al. (2005). TRPC6 is a glomerular slit diaphragm-associated channel required for normal renal function. Nat. Genet. 37, 739–744. doi: 10.1038/ng1592
Ridgway, L. D., Kim, E. Y., and Dryer, S. E. (2009). MAGI-1 interacts with Slo1 channel proteins and suppresses Slo1 expression on the cell surface. Am. J. Physiol. Cell Physiol. 297, C55–C65. doi: 10.1152/ajpcell.00073.2009
Rothberg, B. S. (2012). The BK channel: a vital link between cellular calcium and electrical signaling. Protein Cell 3, 883–892. doi: 10.1007/s13238-012-2076-8
Sansom, S. C., Ma, R., Carmines, P. K., and Hall, D. A. (2000). Regulation of Ca(2+)-activated K(+) channels by multifunctional Ca(2+)/calmodulin-dependent protein kinase. Am. J. Physiol. Renal Physiol. 279, F283–F288. Available online at: http://ajprenal.physiology.org/content/279/2/F283
Sours-Brothers, S., Ding, M., Graham, S., and Ma, R. (2009). Interaction between TRPC1/TRPC4 assembly and STIM1 contributes to store-operated Ca2+ entry in mesangial cells. Exp. Biol. Med. 234, 673–682. doi: 10.3181/0809-RM-279
Stockand, J. D., and Sansom, S. C. (1996). Role of large Ca(2+)-activated K+ channels in regulation of mesangial contraction by nitroprusside and ANP. Am. J. Physiol. 270, C1773–C1779.
Tao, J., Shi, J., Yan, L., Chen, Y., Duan, Y. H., Ye, P., et al. (2011). Enhancement effects of martentoxin on glioma BK channel and BK channel (alpha+beta1) subtypes. PLoS ONE 6:e15896. doi: 10.1371/journal.pone.0015896
Tao, J., Zhou, Z. L., Wu, B., Shi, J., Chen, X. M., and Ji, Y. H. (2014). Recombinant expression and functional characterization of martentoxin: a selective inhibitor for BK channel (alpha + beta4). Toxins 6, 1419–1433. doi: 10.3390/toxins6041419
Winn, M. P., Conlon, P. J., Lynn, K. L., Farrington, M. K., Creazzo, T., Hawkins, A. F., et al. (2005). A mutation in the TRPC6 cation channel causes familial focal segmental glomerulosclerosis. Science 308, 1801–1804. doi: 10.1126/science.1106215
Wu, P., Wang, Y., Davis, M. E., Zuckerman, J. E., Chaudhari, S., Begg, M., et al. (2015). Store-operated Ca2+ channels in mesangial cells inhibit matrix protein expression. J. Am. Soc. Nephrol. 26, 2691–2702. doi: 10.1681/ASN.2014090853
Yang, J., Xu, P., Xie, Y., Li, Z., Xu, J., Zhang, T., et al. (2013). Developmental changes of BKCa channels depend on differentiation status in cultured podocytes. In vitro Cell. Dev. Biol. Anim. 49, 205–211. doi: 10.1007/s11626-013-9590-8
Yuan, P., Leonetti, M. D., Pico, A. R., Hsiung, Y., and Mackinnon, R. (2010). Structure of the human BK channel Ca2+-activation apparatus at 3.0 A resolution. Science 329, 182–186. doi: 10.1126/science.1190414
Keywords: BK channels, podocytes, mesangial cells, β subunits, glomerular filtration
Citation: Tao J, Lan Z, Wang Y, Hei H, Tian L, Pan W, Zhang X and Peng W (2016) Large-Conductance Calcium-Activated Potassium Channels in Glomerulus: From Cell Signal Integration to Disease. Front. Physiol. 7:248. doi: 10.3389/fphys.2016.00248
Received: 22 March 2016; Accepted: 06 June 2016;
Published: 21 June 2016.
Edited by:
Christine Kranz, University of Ulm, GermanyReviewed by:
Stuart E. Dryer, University of Houston, USAArohan Subramanya, University of Pittsburgh School of Medicine, USA
Copyright © 2016 Tao, Lan, Wang, Hei, Tian, Pan, Zhang and Peng. This is an open-access article distributed under the terms of the Creative Commons Attribution License (CC BY). The use, distribution or reproduction in other forums is permitted, provided the original author(s) or licensor are credited and that the original publication in this journal is cited, in accordance with accepted academic practice. No use, distribution or reproduction is permitted which does not comply with these terms.
*Correspondence: Xuemei Zhang, eHVlbXpoYW5nQGZ1ZGFuLmVkdS5jbg==;
Wen Peng, cGVuZ3dlbl8wMUB2aXAuc2luYS5jb20=
†These authors have contributed equally to this work