- 1School of Medicine and Dentistry, Universidad Católica de Valencia San Vicente Mártir, Valencia, Spain
- 2Facultad de Ciencias de la Salud, Universidad Europea de Valencia, Valencia, Spain
- 3Department of Biomedical Sciences, Universidad Cardenal Herrera, CEU, Moncada, Spain
HIGHLIGHTS
• Ethanol, Periodontal ligament, Extracellular matrix, Orthodontic movement.
Alcohol is a legal drug present in several drinks commonly used worldwide (chemically known as ethyl alcohol or ethanol). Alcohol consumption is associated with several disease conditions, ranging from mental disorders to organic alterations. One of the most deleterious effects of ethanol metabolism is related to oxidative stress. This promotes cellular alterations associated with inflammatory processes that eventually lead to cell death or cell cycle arrest, among others. Alcohol intake leads to bone destruction and modifies the expression of interleukins, metalloproteinases and other pro-inflammatory signals involving GSKβ, Rho, and ERK pathways. Orthodontic treatment implicates mechanical forces on teeth. Interestingly, the extra- and intra-cellular responses of periodontal cells to mechanical movement show a suggestive similarity with the effects induced by ethanol metabolism on bone and other cell types. Several clinical traits such as age, presence of systemic diseases or pharmacological treatments, are taken into account when planning orthodontic treatments. However, little is known about the potential role of the oxidative conditions induced by ethanol intake as a possible setback for orthodontic treatment in adults.
Introduction
Since prehistoric ages alcohol (chemically known as ethyl alcohol or ethanol; EtOH) has been consumed by humans. In fact, EtOH is present in beer, wine, spirits and many other drinks. In fact, these products are usually consumed on a daily basis in several countries worldwide. EtOH dependence and abuse are the most abundant mental disorders worldwide. In America, approximately 14% of the population meets chronic alcoholic criteria during some period of their lives (Elkstrom and Ingelman-Sundberg, 1989; Caro and Cederbaum, 2004). EtOH is involved in almost 50% of traffic accidents, the majority of homicides, suicides and domestic violence cases (Graham et al., 1998; Ofori-Adjei et al., 2007). Additionally, EtOH is also implicated in several organic diseases as well as in diverse forms of cancer, including oral cancer (Nelson et al., 2013).
Many reports are available on EtOH-related oral health (Kranzler et al., 1990; Franceschi, 1993; Gelbier and Harris, 1996). There are some important studies on gingival margin recession and oral mucosae alterations (Harris et al., 1996, 2004; Khocht et al., 2003, 2013). Little evidence is reported about the direct effects of EtOH consumption on dental tissues, aside from cancer. One important reason to explain this lack of evidence is the existence of a diversity of factors in connection with alcoholism, e.g., vitamin/nutrients deficiency, other drugs of abuse, smoking, deficient oral care, caries, etc. All these elements impair the identification of concrete factors directly and exclusively related to EtOH in oral diseases (Gelbier and Harris, 1996; Marques et al., 2015).
According to the American Association of Orthodontists, around 4.8 million Americans wear braces. From 1994 to 2010, the percentage of adults getting braces rose from 680,000 to 1.1 million a year (58%). This fact suggests that a relevant number of adults getting braces or other tooth-related devices might consume EtOH along a great part of the orthodontic treatment.
There are several, and sometimes unknown, factors that determine orthodontic outcome. This review focuses on the potential role of EtOH consumption during orthodontic treatment as a plausible factor affecting orthodontic outcome. Surprisingly, EtOH exposure and orthodontic movement affect the same cellular and molecular signaling pathways, giving support to this hypothesis.
General and Local EtOH Metabolism: Oxidative Stress and EtOH-Related Diseases
Because the liver is the main EtOH-detoxifying organ, EtOH-induced alterations have been mostly studied in hepatic tissue. However, nervous tissue, diverse connective-related tissues and others are also affected. EtOH exerts its deleterious effects in several tissues via oxidative and non-oxidative metabolic pathways (Bondy and Guo, 1995) involving free radical production and lipid peroxidation (Sun et al., 1997; Bosch-Morell et al., 1998; Ramachandran et al., 2003; Almansa et al., 2013; Flores-Bellver et al., 2014). One of the most important factor in this toxic process deals with the properties of EtOH to promote reactive oxygen species (ROS). These ROS ultimately react with macromolecules, among them membrane lipids, producing aldehydes such as 4-Hydroxynonenal (4-HNE) and Malondialdehyde (MDA). It is well known that aldehydes and ROS can directly affect both proteins or DNA, leading to transcription-repression of concrete genes. In fact, the role of ROS and aldehydes seems to be a key factor for these alterations, partially confirmed by the fact that administration of antioxidants prevents these EtOH-induced cellular alterations (Herrera et al., 2003; Bati et al., 2015; Han et al., 2015).
Cytochrome P450 and alcohol dehydrogenase (ADH) are the most relevant enzymes involved in EtOH metabolism. Both enzymes can be found not only in liver but also in other tissues (detailed below). The cytochrome P450 family proteins are involved in the oxidative metabolism of both endogenous and xenobiotic products (Tsutsumi et al., 1993; Miksys and Tyndale, 2002). It is known that CYP2E1 isoform is specifically involved in EtOH oxidation; furthermore, CYP2E1 has more affinity for EtOH than alcohol dehydrogenase (ADH) (Albano, 2008). In fact, CYP2E1 assumes an important role in ethanol metabolism, being considered as a major component of the microsomal ethanol-oxidizing system (MEOS) (Lieber and DeCarli, 1970; Koop et al., 1982). Despite EtOH being mostly catabolized in the liver by CYP2E1, the presence of CYP2E1 and ADH in other tissues indicates that EtOH could also be processed by a non-hepatic route (Martinez-Gil et al., 2015).
CYP2E1 is present in the digestive system, one of the most threatening environments because it is continuously exposed to different media containing chemicals, toxins, etc. In fact, CYP2E1 and ADH are strongly expressed not only in liver and the digestive tract, but also in other human oral cells as gingival fibroblasts, pulp, tongue and osteoblasts (Redetzki, 1960; Dong et al., 1996; Chen et al., 2006; Reichl et al., 2010; Plapp et al., 2015). Interestingly enough, it is well established that there is a good relation between CYP2E1 and EtOH in several digestive-related forms of cancer, e.g., mouth, pharynx, esophagus, colorectum and liver cancer (reviewed by Seitz and Wang, 2013). The presence of CYP2E1 and ADH in other cell types could explain a local and direct EtOH-detoxifying process (Flores-Bellver et al., 2014). In this sense, ethanol diffuses rapidly into saliva. Thirty minutes after alcohol intake, EtOH salivary and plasmatic levels are equilibrated. At the same time the levels of acetaldehyde in saliva exceed the systemic blood levels. Acetaldehyde and ethanol from saliva easily reach all the local tissues (Waszkiewicz et al., 2011, 2012; Zalewska et al., 2011). So it seems reasonable that EtOH and acetaldehyde can directly affect oral related structures.
Despite the fact that ADH has lower affinity for EtOH than CYP2E1, ADH is also relevant for EtOH detoxification. CYP2E1 and ADH are both present in the liver (Redetzki, 1960; Plapp et al., 2015) and also expressed in human attached gingiva and tongue (Dong et al., 1996). Surprisingly enough, whereas ADH is expressed in stromal osteoblasts, CYP2E1 seems to be unexpressed (Chen et al., 2006). Although these enzymes are not ubiquitously present in all tissues, their presence in liver and other tissues, clearly indicates the existence of extra-hepatic EtOH metabolism and that it might be related with some EtOH-related forms of cancer (Seitz and Wang, 2013).
Periodontum, Extracellular Matrix, and Bone Dynamics
The periodontum must be briefly presented as a complex histological area surrounding teeth relevant for root-tooth stability. This periodontal structure includes fibroblasts surrounded by the extracellular matrix (ECM) of hyaluronic acid (HA) and other extracellular proteins as collagen, mostly produced by periodontal fibroblasts. The most abundant collagen form is the type I collagen (Bornstein and Sage, 1980; Zhang et al., 1993).
Other important components of the periodontal ligament (PDL) are the matrix metalloproteinase enzyme family (MMP's) that degrade collagen, and its counterpart, tissue inhibitor metalloproteinases (TIMP) that do inhibit MMP's, being MMP-1 enzyme the most abundant in PDL (Birkedal-Hansen et al., 1993). Obviously, the balance between collagen production and MMP's activity determines the PDL quality and consequently dental stability. MMP's also degrade collagen under pathological conditions and therefore MMP-1, MMP-8, MMP-2, MMP-13 are locally and temporarily expressed during tooth movement phases (Apajalahti et al., 2003; Ingman et al., 2005; Cantarella et al., 2006; Leonardi et al., 2006; Huang et al., 2008; Meeran, 2012).
Root and bone resorption are both directly regulated by a group of tumor necrosis factor (TNF)-related proteins with paracrine-regulatory properties (Schoppet et al., 2002). Osteoprotegerin (OPG) is a soluble protein secreted by osteoblasts that acts as an inhibitor of both osteoclast differentiation and resorptive activity, promoting osteoclast apoptosis (Oshiro et al., 2002). Receptor activator of nuclear factor kappa-b ligand (RANKL) is expressed on the cell surface of osteoblast precursors (Schoppet et al., 2002), whereas its receptor (RANK) is expressed by osteoblastic cell lineages and activated T-cells (Katagiri and Takahashi, 2002). RANKL acts, together with macrophage colony stimulating-factor (M-CSF), promoting osteoclast formation, differentiation and activation, enhancing bone resorption activities (Kong et al., 1999; Liu and Zhang, 2015; Martin and Sims, 2015).
One important step for osteoclast fusion and activation is the coupling of RANK to RANKL. This union can be blocked by OPG, so the balance “resorption vs. reposition” depends on the prevalence of RANK vs. OPG, respectively.
Orthodontic Forces Affect Periodontal Structures Modifying Intra- and Extra-Cellular Proteins
Orthodontic Forces Lead to Extracellular Modifications
During orthodontic movement, applied forces modulate both molecular and cellular configurations, e.g., those producing collagen (Bumann et al., 1997), modifying the periodontal structure and therefore dental position (Nakagawa et al., 1994; Krishnan and Davidovitch, 2006). Some evidence indicates that mechanical forces modulate the expression of integrins, MMP's or collagen (Bolcato-Bellemin et al., 2000; Von den Hoff, 2003; He et al., 2004). On the hypothetical model for periodontal remodeling summarized by Meikle (2006), tension and compressive sides present some similarities. In the tensile strain, periodontal fibroblasts release IL-1 and IL-6; these interleukins can stimulate MMP's and inhibit TIMP synthesis, so bone and matrix lose structure in order to facilitate bone and PDL regeneration. At the same time, mechanically activated fibroblasts can induce angiogenesis by vascular endothelial growth factor (VEGF) release, helping bone renewal. In the compression side, similarly to the tensile side, IL-1, IL-6, and MMP's are released. One of the differences between both complementary processes seems to be the prevalence of OPG vs. RANK, leading to bone reconstruction and bone destruction, respectively (Tyrovola et al., 2008).
This represents an interesting issue for orthodontics or periodontal management, since both conditions involve these type of cellular responses, e.g., during tooth movement or periodontal disease. In this regard, some reports have found different biological markers in the gingivo-crevicular fluid (GCF): elevated levels of Prostaglandin E, IL-1β, IL-6, TNF-α and epidermal growth factor (EGF) have been found in GCF during tooth movement or periodontal disease (Grieve et al., 1994; Uematsu et al., 1996). Hyaline material and sterile necrosis in local pressure zones have been found also after tooth movement (Kurol and Owman-Moll, 1998). Unfortunately, the significance of these changes is only partially known.
Extracellular matrix degradation facilitates cell proliferation and capillary growth leading to the synthesis of new PDL and bone structures. However, on the compression side, periodontal cells also release IL-1 and IL-6, up-regulating not only MMP's, but also RANKL, leading to osteoclast-mediated bone resorption (Nakano et al., 2011).
Cathepsins are lysosomal cysteine proteases that play an important role in bone resorption. Cathepsin B levels can be increased by orthodontic tooth movement, being involved in extracellular matrix degradation in response to mechanical stress (Maeda et al., 2007). Since Cathepsins K, B and L are over-expressed in the compression side, they may be related to bone resorption (Domon et al., 1999; Sugiyama et al., 2003).
Orthodontic Forces Lead to Intracellular Modifications
Mechanical strain generates diverse intracellular responses in cells during orthodontic movements that could be of clinical interest. Integrins are transmembrane proteins whose extracellular side connects to the ECM via fibronectin (Wang et al., 1993; Clarke and Brugge, 1995), and the intracellular one connects with actin of the cytoskeleton. In fact, this actin-cytoskeletal connection is mediated by proteins as paxillin, talin and vinculin leading to focal adhesions that are crucial for cell adhesion and migration (Sastry and Burridge, 2000; Meikle, 2006). Although little is known about the role of integrin receptors in ECM for cell adhesion, the intracellular side is associated to cAMP and inositol phosphate activation pathways both involved in downstream cell signaling (Wang et al., 1993; DeMali et al., 2003). Well known integrin-mediated extracellular signals are mitogen-activated protein kinases (MAPKs) and Rho pathways, both are activated by mechanic stimuli in periodontal fibroblasts and osteoblasts (Basdra et al., 1995; Peverali et al., 2001).
MAPKs regulate several cellular responses such as cell division, metabolic processes, survival-apoptosis and differentiation, among others. Five distinct groups of MAPKs have been characterized in mammals: extracellular signal-regulated kinases (ERKs) 1 and 2 (namely ERK1/2), c-Jun amino-terminal kinases (JNKs) 1, 2, and 3, p38 isoforms α, β, γ, and δ, ERKs 3 and 4, and ERK5 (reviewed by Chen et al., 2001; Kyriakis and Avruch, 2001; Roux and Blenis, 2004). ERK 1 and 2 have been described to, when activated, phosphorylate membrane proteins (CD120a, Syk, and calnexin), nuclear substrates and cytoskeletal proteins (neurofilaments and paxillin) (reviewed by Chen et al., 2001; Roux and Blenis, 2004). Cyclic tensile forces up-regulate bone marrow protein-2 (BMP-2) expression via ERK1/2 and p38 MAP kinase pathways, with COX and PGE2 implication, in human periodontal ligament cells (Suzuki et al., 2014) and promote the migration of periodontal cells via ERK signaling pathway activation (Pan et al., 2010). Moreover, multiple data indicates that mechanical forces modulate ERK activities in periodontal fibroblasts increasing type I collagen, ostepontin and MMP-1 production (Liedert et al., 2006; Jeon et al., 2009; Kook et al., 2009, 2011).
Also related to extracellular stimuli-mediated signaling, Rho is a family of serine/threonine kinases (Wennerberg et al., 2005; Bustelo et al., 2007) involved in cell recruitment-migration, proliferation and apoptosis (Ridley, 2001; Etienne-Manneville and Hall, 2002). It has been recently described that Rho is involved in experimental orthodontic tooth movement by increasing Rho-kinase (ROCK) activity on the tension side (Meng et al., 2015). This fits with the finding that ROCK1 acts as a suppressor of inflammatory cell migration by regulating PTEN phosphorylation (Vemula et al., 2010). In line with this, it has been reported that Rho-ROCK enhances the formation of actin stress fibers and focal adhesion in fibroblasts (Amano et al., 1997). More concretely, during experimental orthodontic movement, the tension areas showed increased expression of actin stress fibers as well as increased number of myofibroblasts in the periodontal area (Meng et al., 2010, 2007). These facts are related to focal adhesion phenomena related to dental movement.
Not related to integrin-mediated signaling, Toll-like receptors (TLRs) are transmembrane proteins playing a critical role in innate immune system. TLRs are made up of an extracellular and of a cytoplasmic domain, homologous to the cytoplasmic domain of the human IL-1 receptor (Medzhitov, 2001). TLRs can recognize different patterns, known as pathogen-associated molecular patterns (PAMP). These PAMPs include lipids, proteins, lipoproteins, nucleic acids, and lipopolysaccarides (LPS) (Medzhitov and Janeway, 1997; Yang et al., 1998). TLR4 is particularly interesting in oral tissues because it is highly expressed by periodontal fibroblasts and specifically recognizes Porphyromonas gingivalis LPS (Takeuchi and Akira, 2001). The activation of TLR4 promotes pro-inflammatory signaling processes leading to periodontal alterations, osteoclast activation-recruitment and cytokine expression (Kikkert et al., 2007; Gelani et al., 2009; Nussbaum et al., 2009). TLR4 has been recently associated with mechanical forces on fibroblasts: its activation increased the expression of MMP-1, 3, and 10, increased phosphorylation of p38, JNK, and NF-κB, strongly suggesting that TLR4 may play an important role during orthodontic treatment (Lisboa et al., 2013). Hyaluronic acid (HA) is a classic and abundant component of connective tissue also present in the PDL. HA is an endogenous ligand for TLR4 that promotes protective responses in skin and lung injury models (Jiang et al., 2005; Taylor et al., 2007). Although the anti-inflammatory properties of HA and its mechanisms are partially unknown, direct interactions with inflammatory cells and the physical properties of the molecule, seem to be implicated. It is shown that HA reduces TNF-α and IFN-γ production and induces NF-κB activation in macrophages (Noble et al., 1996; Wang et al., 2006). As an example, this TLR4-HA interaction seems to be related to Cox-2 and PGE2 production to protect the colon mucosa from injury (Chen et al., 2011). More research is needed to explain the concrete role and mechanism of the HA-TLR4 interaction that could make it be of interest for orthodontic and periodontal clinical care.
A graphic summary of the periodontal area with the extracellular processes is detailed in Figures 1, 2.
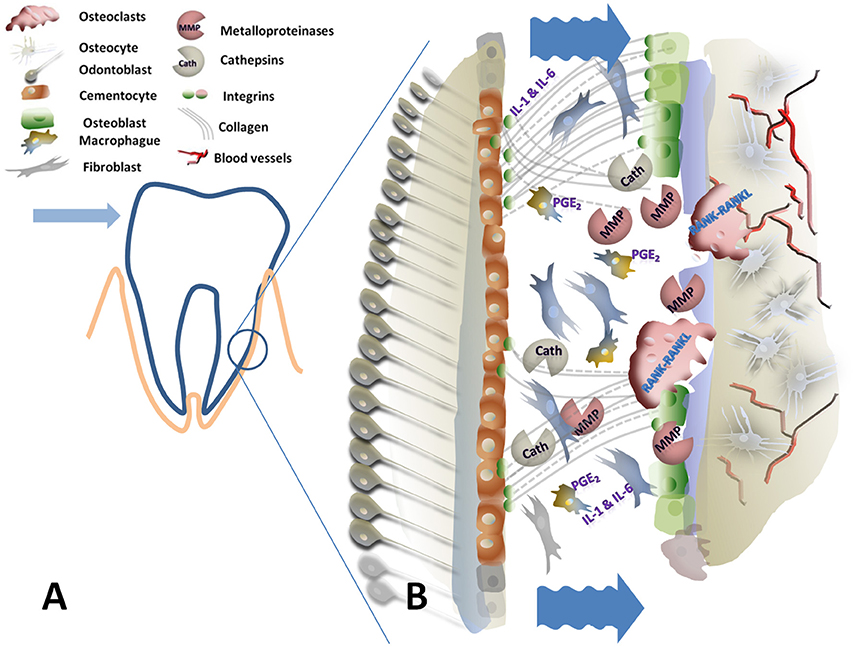
Figure 1. Graphical scheme of the periodontal ligament and alveolar bone under compressive forces. (A) Is the schematic representation of a tooth in the socket where the arrowhead indicates the sense of the applied force and the encircled area represents the compressive side. (B) Detailed view of the compressive side: Wrinkled arrows indicate the reduction on the periodontal gap due to compression. This mechanical signal affects cells and extracellular matrix components promoting extracellular release of matrix degrading enzymes as Metalloproteinases (MMP) and Cathepsins (Cath), macrophage activation (IL1, 6, and PGE2) and RANK-RANKL osteoclast activation. This results on bone resorption with periodontal destruction-reconstruction in the new dental position.
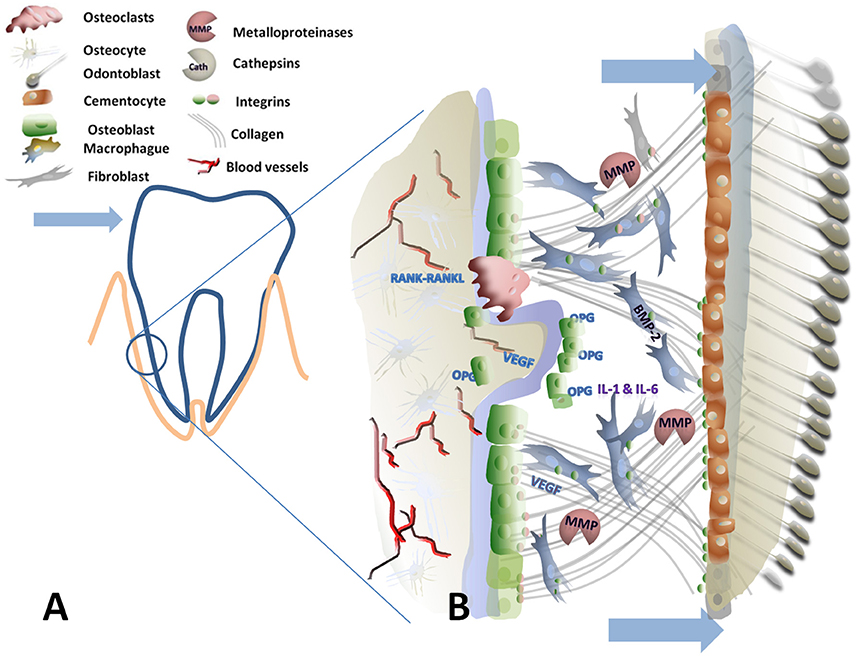
Figure 2. Graphical scheme of the periodontal ligament and alveolar bone under tensile forces. (A) Is the schematic representation of a tooth in the socket where the arrowhead indicate the applied force. The encircled area represents the tensile side. (B) Detailed view of the tensile side: Arrows indicate the increase on the periodontal gap due to tensile force. Tensile forces are transmitted via collagen-coupled Integrins to different cell types promoting new bone generation. Bone marrow protein 2 (BMP-2), osteoprotegerin (OPG), vascular endothelial growth factor (VEGF) are necessary for new bone formation.
EtOH Modulates Extracellular Proteins and Promotes Intracellular Changes
EtOH Modifies Extracellular Protein Activities and Bone Dynamics
It is well documented how EtOH affects osteoclastic/osteoblastic dynamics producing osteopenia and osteoporosis (Manolagas, 2000; Turner, 2000). Although the mechanisms are not fully understood, EtOH may promote bone loss inhibiting osteoblastogenesis (Friday and Howard, 1991) by altering bone remodeling-related genes (Chakkalakal, 2005; Callaci et al., 2009). It has also been shown an inverse correlation between EtOH intake and bone mineral density in both pre- and post-menopausal women (Turner and Sibonga, 2001). IL-6 seems to be responsible, at least in part, for this EtOH-induced bone loss (Dai et al., 2000). Interestingly enough, IL-6 is also increased during orthodontic movement (Grieve et al., 1994; Uematsu et al., 1996) and therefore it seems plausible that EtOH consumption during orthodontic treatment would affect the outcome of the intervention via IL-6. More research is needed to analyze the IL-6 levels and bone remodeling under these circumstances (EtOH+ orthodontic forces).
Some reports indicate that EtOH exposure preferentially alters the periodontal area, developing periodontitis by increasing the loss of attachment through recession of gingival margins (Khocht et al., 2003) or by altering the oral mucosa (Harris et al., 1996, 2004). Regarding the influence of EtOH and other drugs on tooth decay, some studies focus the attention on the EtOH-induced oral micro-flora alterations due to EtOH-acetaldehyde metabolism, leading to the progression of dental caries (Dasanayake et al., 2010; Rooban et al., 2011), and little is known about the role of EtOH on orthodontic movement.
Estrogens can protect from bone resorption (Kousteni et al., 2001; Chen et al., 2005) and this inhibitory effect seems to be related to the RANKL-RANK-OPG system (Syed and Khosla, 2005). In fact, estrogens can suppress RANKL expression in osteoblasts (Bord et al., 2003). Chen et al. (2006, 2008) found that the protective effects of estradiol on EtOH-induced bone loss was related to the inhibition of ROS production in osteoblasts. Additionally, NADPH oxidase (NOx) and estradiol would play a critical role on EtOH-induced bone loss via the ERK/STAT3/RANKL pathway.
It has been demonstrated that chronic EtOH consumption promotes bone loss, increases PGE2 expression and other inflammatory markers in rats (Dantas et al., 2012; Surkin et al., 2014). All these markers are related to periodontal disease, so the hypothesis of EtOH-induced oxidative burden as a modulator of the extracellular environment during tooth movement is supported.
Some reports indicate that MMP-1, Cathepsins K, B, and L are increased in the compression side during tooth movement (Domon et al., 1999; Sugiyama et al., 2003; Maeda et al., 2007). EtOH-induced osteoclastogenesis increases RANK and Cathepsin K activities (Domon et al., 1999). However, it has been shown that EtOH reduces proteolytic activity in hepatic Cathepsins B and L (Kharbanda et al., 1995, 1996). EtOH and tooth movement may act in the same way by increasing bone resorption and PDL remodeling in the compression side despite this tissue difference. Future studies should be addressed to know whether this fact is synergistic or accumulative leading to excessive bone resorption and eventually to root resorption.
EtOH Promotes Intracellular Responses
EtOH affects intestinal epithelial tight junction integrity via Ca++-mediated Rho/ROCK activation (Elamin et al., 2014). It has been described that EtOH exposure disorganizes actin-cytoskeleton in astrocytes and this process is mediated by RhoA signaling pathway (Guasch et al., 2003). Although nothing is known about the effect of EtOH on the cytoskeletal periodontal fibroblasts and osteoblasts, some evidence indicates that both EtOH and periodontal movement act in the same Rho-ROCK pathway. It seems reasonable that EtOH exposure during orthodontic movement may alter the cytoskeletal organization affecting orthodontic outcome.
ROS is a relevant extracellular ERK-triggering stimulus that up-regulates ERK-dependent genes such as RANKL (Torres, 2003). Supporting this fact, it was found that the administration of antioxidants such as N-Acetyl cysteine, estradiol or vitamin C, suppress RANKL mRNA expression and induces PDL progenitor cell differentiation via ERK activation pathway (Chen et al., 2008; Yan et al., 2013). In this regard, it is well documented that EtOH metabolism results in ROS production and subsequently leads to cell damage and eventually death (Johnsen-Soriano et al., 2007; Flores-Bellver et al., 2014). Even more, EtOH-induced oxidative stress seems to be crucial for these negative effects on cells, since the administration of antioxidants restores the oxidative misbalance and prevents the negative effects on cells (Herrera et al., 2003; Koch et al., 2004; Crews et al., 2007). ROS and EtOH activate MMP- 1,-2, and -9 via protein tyrosine kinase signaling, leading to basal membrane disruption (Haorah et al., 2007, 2008).
It is well documented that EtOH promotes inflammatory responses via TLR4 in different tissues, e.g., brain, lung and liver (Vaneker et al., 2008; Fernandez-Lizarbe et al., 2013; Zmijewski et al., 2014; Pascual et al., 2015). So, after considering the aforementioned data on TLR4, it seems plausible that EtOH exposure could be closely related to periodontal stability (see Figure 3) and therefore it becomes an important factor on clinical practice.
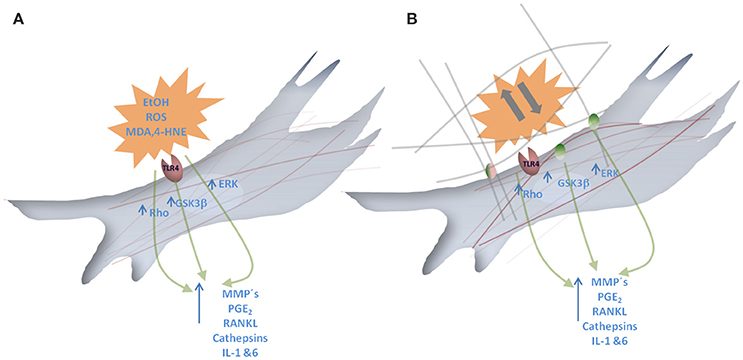
Figure 3. Graphical scheme of the molecular responses promoted by EtOH exposure and mechanical forces. (A) Represents a fibroblast where EtOH and EtOH-derived reactive oxygen species (ROS), lipid peroxidation products such as 4-hydroxynonenal (4-HNE) and Malondialdehyde (MDA) interact with Toll like receptor4 (TLR4). This interaction results in Rho, GSKβ and ERK pathway activation that leads to MMP/Cath, PGE2, RANKL and IL-1 and 6 release. (B) Representation of a fibroblast where mechanical forces affecting extracellular matrix can interact with integrins. This interaction results in Rho, GSKβ, and ERK pathway activation that leads to MMP/Cath, PGE2, RANKL and IL-1 and 6 release.
Glycogen synthase kinase 3 β (GSK3β) regulates the production of cytokines after TLR4 stimulation (Martin et al., 2005). TLR4-GSK3β route activation has been closely related to periodontal alterations induced by P. gingivalis and other pathogens (Wang et al., 2011). In this sense, and fitting with this, it has been shown how alcoholic fatty liver pathogenesis implicates GSK3β route activation (Zeng et al., 2014) and it has been demonstrated that GSK3β inhibition suppresses bacterial-induced periodontal bone loss (Adamowicz et al., 2012), supporting the idea that TLR4-GSK3β pathway could be particularly affected in alcohol-users. It is well documented that EtOH promotes inflammatory responses via TLR4 in different tissues, e.g., brain, lung and liver (Vaneker et al., 2008; Fernandez-Lizarbe et al., 2013; Zmijewski et al., 2014; Pascual et al., 2015). Considering the aforementioned data on TLR4, it seems plausible that EtOH exposure could be closely related to periodontal stability (see Figure 3) and therefore it becomes an important factor on clinical practice.
Final Discussion
Despite the fact that several studies indicate the potential deleterious effect of EtOH on periodontum and alveolar bone, there is only one report on the effects of ethanol during orthodontic movement. In this work, Araujo and collaborators describe less bone resorption at the end of tooth movement suggesting a delay of tooth movement in a rat model of binge drinking (Araujo et al., 2014). Obviously, this model simulates a drinking pattern where high EtOH concentrations are acutely consumed, which is different from the chronic pattern, where high EtOH levels are daily maintained for several weeks. According to the typically long lasting orthodontic treatments, mild and chronic EtOH exposure could interfere with this chronic orthodontic treatment by modifying the aforementioned proteins and genes leading to orthodontic failure or undesired outcome.
Systemic diseases are of relevance in oral medicine and dentistry. Diabetes mellitus (DM) is considered a common systemic disease with oral manifestations and profuse literature deals with the considerations of orthodontic treatment on diabetic patients (Burden et al., 2001; Vernillo, 2001; Bensch et al., 2003; McKenna, 2006). Experimental data widely show that DM promotes molecular and structural changes in the periodontal area after orthodontic treatment including MMP's or bone alterations (Feng et al., 2007; Abbassy et al., 2010; Braga et al., 2011; Villarino et al., 2011; Zhang et al., 2011). There are some similarities between alcohol exposure and DM in terms of molecular signaling and gene expression (Barcia et al., 2015). Additionally, experimental and clinical studies strongly indicate a close relationship between alcohol intake and risk of diabetes development (Cullmann et al., 2012; Kim et al., 2013). In consonance with this issue, orthodontic movement promotes intra and extracellular alterations, finally affecting periodontum and alveolar bone. Since the influence of DM in orthodontic treatment outcome seems clear, it seems appropriate to further investigate the effects of chronic EtOH exposure on orthodontic treatment.
As a hypothetical model, EtOH exposure during orthodontic movement may interfere with osteogenesis at the tension side, accepting that EtOH produces osteoblastogenesis inhibition (Friday and Howard, 1991). IL-6/ROS and PGE2 mediated bone loss is induced by EtOH (Dai et al., 2000; Chen et al., 2006) and it also increases RANKL (Chen et al., 2008). Additionally, as mentioned above, TLR4 over-activation (EtOH+ tensile strain) may lead to GSKβ activation, negatively affecting the periodontum (Kikkert et al., 2007; Gelani et al., 2009; Nussbaum et al., 2009). On the compression side, where bone destruction and reorganization takes place, probably bone resorption would be increased during EtOH metabolism leading to a rapid but unstable teeth position.
In view of the close similarities found between EtOH- and mechanical strain-induced responses on periodontal tissues, the aim of this review is to spark attention on the potential effect of EtOH consumption during orthodontic or periodontal treatment as a factor that needs to be considered in clinical practice. Further research is necessary to fully and experimentally support the actual indications suggesting that alcoholic beverages consumption should be discouraged during orthodontic treatment in adults.
Author Contributions
JB proposed the subject of the revision and distributed the tasks; supervised each of the topics that were revised. SP, VA, and LP revised the existing literature on tooth movement regulation and effects of alcohol on oral diseases. AU and GP supervised references content and the different perspectives studied. VV and VA supervised manuscript writing. FR supervised the whole manuscript and its final version.
Conflict of Interest Statement
The authors declare that the research was conducted in the absence of any commercial or financial relationships that could be construed as a potential conflict of interest.
Acknowledgments
Partially supported by grant Prometeo 94/2016 from the Generalitat Valenciana and by Funds from Fundación Universidad Catolica de Valencia San Vicente Martir and Universidad Europea de Valencia to FR.
References
Abbassy, M. A., Watari, I., and Soma, K. (2010). The effect of Diabetes mellitus on rat mandibular bone formation and microarchitecture. Eur. J. Oral Sci. 118, 364–369. doi: 10.1111/j.1600-0722.2010.00739.x
Adamowicz, K., Wang, H., Jotwani, R., Zeller, I., Potempa, J., and Scott, D. A. (2012). Inhibition of GSK3 abolishes bacterial-induced periodontal bone loss in mice. Mol. Med. 18, 1190–1196. doi: 10.2119/molmed.2012.00180
Albano, E. (2008). Oxidative mechanisms in the pathogenesis of alcoholic liver disease. Mol. Aspects Med. 29, 9–16. doi: 10.1016/j.mam.2007.09.004
Almansa, I., Barcia, J. M., López-Pedrajas, R., Muriach, M., Miranda, M., and Romero, F. J. (2013). Naltrexone reverses ethanol-induced rat hippocampal and serum oxidative damage. Oxid. Med. Cell. Longev. 2013:296898. doi: 10.1155/2013/296898
Amano, M., Chihara, K., Kimura, K., Fukata, Y., Nakamura, N., Matsuura, Y., et al. (1997). Formation of actin stress fibers and focal adhesions enhanced by rho-kinase. Science 275, 1308–1311. doi: 10.1126/science.275.5304.1308
Apajalahti, S., Sorsa, T., Railavo, S., and Ingman, T. (2003). The in vivo levels of matrix metalloproteinase-1 and -8 in gingival crevicular fluid during initial orthodontic tooth movement. J. Dent. Res. 82, 1018–1022. doi: 10.1177/154405910308201216
Araujo, C. M., Johann, A. C., Camargo, E. S., and Tanaka, O. M. (2014). The effects of binge-pattern alcohol consumption on orthodontic tooth movement. Dental Press J. Orthod. 19, 93–98. doi: 10.1590/2176-9451.19.6.093-098.oar
Barcia, J. M., Flores-Bellver, M., Muriach, M., Sancho-Pelluz, J., Lopez-Malo, D., Urdaneta, A. C., et al. (2015). Matching diabetes and alcoholism: oxidative stress, inflammation, and neurogenesis are commonly involved. Mediators Inflamm. 2015:624287. doi: 10.1155/2015/624287
Basdra, E. K., Papavassiliou, A. G., and Huber, L. A. (1995). Rab and rho GTPases are involved in specific response of periodontal ligament fibroblasts to mechanical stretching. Biochim. Biophys. Acta 1268, 209–213. doi: 10.1016/0167-4889(95)00090-F
Bati, B., Celik, I., and Dogan, A. (2015). Determination of hepatoprotective and antioxidant role of walnuts against ethanol-induced oxidative stress in rats. Cell. Biochem. Biophys. 71, 1191–1198. doi: 10.1007/s12013-014-0328-3
Bensch, L., Braem, M., Van Acker, K., and Willems, G. (2003). Orthodontic treatment considerations in patients with Diabetes mellitus. Am. J. Orthod. Dentofacial Orthop. 123, 74–78. doi: 10.1067/mod.2003.53
Birkedal-Hansen, H., Moore, W. G., Bodden, M. K., Windsor, L. J., Birkedal-Hansen, B., DeCarlo, A., et al. (1993). Matrix metalloproteinases: a review. Crit. Rev. Oral Biol. Med. 4, 197–250. doi: 10.1177/10454411930040020401
Bolcato-Bellemin, A. L., Elkaim, R., Abehsera, A., Fausser, J. L., Haikel, Y., and Tenenbaum, H. (2000). Expression of mRNAs encoding for α and β integrin subunits, MMPs, and TIMPs in stretched human periodontal ligament and gingival fibroblasts. J. Dent. Res. 79, 1712–1716. doi: 10.1177/00220345000790091201
Bondy, S. C., and Guo, S. X. (1995). Regional selectivity in ethanol-induced pro-oxidant events within the brain. Biochem. Pharmacol. 6, 69–72. doi: 10.1016/0006-2952(94)00442-O
Bord, S., Ireland, D. C., Beavan, S. R., and Compston, J. E. (2003). The effects of estrogen on osteoprotegerin, RANKL, and estrogen receptor expression in human osteoblasts. Bone 32, 136–141. doi: 10.1016/S8756-3282(02)00953-5
Bornstein, P., and Sage, H. (1980). Structurally distinct collagen types. Ann. Rev. Biochem. 49, 957–1003. doi: 10.1146/annurev.bi.49.070180.004521
Bosch-Morell, F., Martínez-Soriano, F., Colell, A., Fernández-Checa, J. C., and Romero, F. J. (1998). Chronic ethanol feeding induces cellular antioxidants decrease and oxidative stress in rat peripheral nerves. Effect of S-adenosyl-L-methionine and N-acetyl-L-cysteine. Free Radic. Biol. Med. 25, 365–368. doi: 10.1016/S0891-5849(98)00036-7
Braga, S. M., Taddei, S. R., Andrade, I. Jr., Queiroz-Junior, C. M., Garlet, G. P., Repeke, C. E., et al. (2011). Effect of diabetes on orthodontic tooth movement in a mouse model. Eur. J. Oral Sci. 119, 7–14. doi: 10.1111/j.1600-0722.2010.00793.x
Bumann, A., Carvalho, R. S., Schwarzer, C. L., and Yen, E. H. (1997). Collagen synthesis from human PDL cells following orthodontic tooth movement. Eur. J. Orthod. 19, 29–37. doi: 10.1093/ejo/19.1.29
Burden, D., Mullally, B., and Sandler, J. (2001). Orthodontic treatment of patients with medical disorders. Eur. J. Orthod. 23, 363–372. doi: 10.1093/ejo/23.4.363
Bustelo, X. R., Sauzeau, V., and Berenjeno, I. M. (2007). GTP-binding proteins of the Rho/Rac family: regulation, effectors and functions in vivo. Bioessays 29, 356–370. doi: 10.1002/bies.20558
Callaci, J. J., Himes, R., Lauing, K., Wezeman, F. H., and Brownson, K. (2009). Binge alcohol-induced bone damage is accompanied by differential expression of bone remodeling-related genes in rat vertebral bone. Calcif. Tissue Int. 84, 474–484. doi: 10.1007/s00223-009-9240-z
Cantarella, G., Cantarella, R., Caltabiano, M., Risuglia, N., Bernardini, R., and Leonardi, R. (2006). Levels of matrix metalloproteinases 1 and 2 in human gingival crevicular fluid during initial tooth movement. Am. J. Orthod. Dentofacial Orthop. 130, 568.e11–568.e16. doi: 10.1016/j.ajodo.2006.04.020
Caro, A. A., and Cederbaum, A. I. (2004). Oxidative stress, toxicology, and pharmacology of CYP2E1. Annu. Rev. Pharmacol. Toxicol. 44, 27–42. doi: 10.1146/annurev.pharmtox.44.101802.121704
Chakkalakal, D. A. (2005). Alcohol-induced bone loss and deficient bone repair. Alcohol Clin. Exp. Res. 29, 2077–2090. doi: 10.1097/01.alc.0000192039.21305.55
Chen, H., Mahaseth, M., and Zhang, Y. (2011). Hyaluronic acid as a rescue therapy for trinitrobenzene sulfonic acid-induced colitis through Cox-2 and PGE2 in a Toll-like receptor 4-dependent way. J. Zhejiang Univ. Sci. B. 12, 712–719. doi: 10.1631/jzus.B1000362
Chen, J. R., Haley, R. L., Hidestrand, M., Shankar, K., Liu, X., Lumpkin, C. K., et al. (2006). Estradiol protects against ethanol-induced bone loss by inhibiting up-regulation of receptor activator of nuclear factor-κB ligand in osteoblasts. J. Pharmacol. Exp. Ther. 319, 1182–1190. doi: 10.1124/jpet.106.109454
Chen, J. R., Plotkin, L. I., Aguirre, J. I., Han, L., Jilka, R. L., Kousteni, S., et al. (2005). Transient versus sustained phosphorylation and nuclear accumulation of ERKs underlie anti-versus pro-apoptotic effects of estrogens. J. Biol. Chem. 280, 4632–4638. doi: 10.1074/jbc.M411530200
Chen, J. R., Shankar, K., Nagarajan, S., Badger, T. M., and Ronis, M. J. (2008). Protective effects of estradiol on ethanol-induced bone loss involve inhibition of reactive oxygen species generation in osteoblasts and downstream activation of the extracellular signal-regulated kinase/signal transducer and activator of transcription 3/receptor activator of nuclear factor-κB ligand signaling cascade. J. Pharmacol. Exp. Ther. 324, 50–59. doi: 10.1124/jpet.107.130351
Chen, Z., Gibson, T. B., Robinson, F., Silvestro, L., Pearson, G., Xu, B., et al. (2001). MAP kinases. Chem. Rev. 101, 2449–2476. doi: 10.1021/cr000241p
Clarke, E. A., and Brugge, J. S. (1995). Integrins and signal transduction pathways: the road taken. Science 268, 233–239. doi: 10.1126/science.7716514
Crews, F., Nixon, K., Kim, D., Joseph, J., Shukitt-Hale, B., Qin, L., et al. (2007). BHT blocks NF-κB activation and ethanol-induced brain damage. Alcohol Clin. Exp. Res. 30, 1938–1949. doi: 10.1111/j.1530-0277.2006.00239.x
Cullmann, M., Hilding, A., and Östenson, C. G. (2012). Alcohol consumption and risk of pre-diabetes and type 2 diabetes development in a Swedish population. Diabet. Med. 29, 441–452. doi: 10.1111/j.1464-5491.2011.03450.x
Dai, J., Lin, D., Zhang, J., Habib, P., Smith, P., Murtha, J., et al. (2000). Chronic alcohol ingestion induces osteoclastogenesis and bone loss through IL-6 in mice. J. Clin. Invest. 106, 887–895. doi: 10.1172/JCI10483
Dantas, A. M., Mohn, C. E., Burdet, B., Zorrilla-Zubilete, M., Mandalunis, P. M., Elverdin, J. C., et al. (2012). Ethanol consumption enhances periodontal inflammatory markers in rats. Arch. Oral Biol. 57, 1211–1217. doi: 10.1016/j.archoralbio.2012.02.008
Dasanayake, A. P., Warnakulasuriya, S., Harris, C. K., Cooper, D. J., Peters, T. J., and Gelbier, S. (2010). Tooth decay in alcohol abusers compared to alcohol and drug abusers. Int. J. Dent. 2010:786503. doi: 10.1155/2010/786503
DeMali, K. A., Wennerberg, K., and Burridge, K. (2003). Integrin signaling to the actin cytoskeleton. Curr. Opin. Cell Biol. 15, 72–582. doi: 10.1016/S0955-0674(03)00109-1
Domon, S., Shimokawa, H., Matsumoto, Y., Yamaguchi, S., and Soma, K. (1999). In situ hybridization for matrix metalloproteinase-1 and cathepsin K in rat root-resorbing tissue induced by tooth movement. Arch. Oral Biol. 44, 907–915. doi: 10.1016/S0003-9969(99)00091-6
Dong, Y. J., Peng, T. K., and Yin, S. J. (1996). Expression and activities of class IV alcohol dehydrogenase and class III aldehyde dehydrogenase in human mouth. Alcohol 13, 257–262. doi: 10.1016/0741-8329(95)02052-7
Elamin, E., Masclee, A., Dekker, J., and Jonkers, D. (2014). Ethanol disrupts intestinal epithelial tight junction integrity through intracellular calcium-mediated Rho/ROCK activation. Am. J. Physiol. Gastrointest. Liver Physiol. 306, G677–G685. doi: 10.1152/ajpgi.00236.2013
Elkstrom, G., and Ingelman-Sundberg, M. (1989). Rat liver microsomal NADPH-supported oxidase activity and lipid peroxidation dependent on ethanol-inducible cytochrome P-450 (P-450IIEI). Biochem. Pharmacol. 38, 1313–1319. doi: 10.1016/0006-2952(89)90338-9
Etienne-Manneville, S., and Hall, A. (2002). Rho GTPases in cell biology. Nature 420, 629–635. doi: 10.1038/nature01148
Feng, Z. M., Wu, Y. S., and Yan, G. Y. (2007). Change of expression of matrix metalloproteinase-2 in the periodontal tissues of Diabetes mellitus rats during orthodontic toot movement. Hua Xi Kou Qiang Yi Xue Za Zhi. 25, 118–121.
Fernandez-Lizarbe, S., Montesinos, J., and Guerri, C. J. (2013). Ethanol induces TLR4/TLR2 association, triggering an inflammatory response in microglial cells. J. Neurochem. 126, 261–273. doi: 10.1111/jnc.12276
Flores-Bellver, M., Bonet-Ponce, L., Barcia, J. M., Garcia-Verdugo, J. M., Martinez-Gil, N., Saez-Atienzar, S., et al. (2014). Autophagy and mitochondrial alterations in human retinal pigment epithelial cells induced by ethanol: implications of 4-hydroxy-nonenal. Cell Death Dis. 5, e1328. doi: 10.1038/cddis.2014.288
Franceschi, S. (1993). Oesophageal and oral cancer: aetiological factors. Eur. J. Cancer Prev. 2, 2–3. doi: 10.1097/00008469-199301001-00007
Friday, K. E., and Howard, G. A. (1991). Ethanol inhibits human bone cell proliferation and function in vitro. Metabolism 40, 562–565. doi: 10.1016/0026-0495(91)90044-W
Gelani, V., Fernandes, A. P., Gasparoto, T. H., Garlet, T. P., Cestari, T. M., Lima, H. R., et al. (2009). The role of toll-like receptor 2 in the recognition of Aggregatibacter actinomycetemcomitans. J. Periodontol. 80, 2010–2019. doi: 10.1902/jop.2009.090198
Gelbier, S., and Harris, C. (1996). Oral and dental health in the alcohol misuser. Addict. Biol. 1, 165–169. doi: 10.1080/1355621961000124786
Graham, K., Leonard, K. E., Room, R., Wild, C., Pihl, R. O., Bois, C., et al. (1998). Alcohol and aggression: current directions in research on understanding and preventing intoxicated aggression. Addiction 93, 659–676. doi: 10.1046/j.1360-0443.1998.9356593.x
Grieve, W. G., Johnson, G. K., Moore, R. N., Reinhardt, R. A., and DuBois, L. M. (1994). Prostaglandin E (PGE) and interleukin-1β (IL-1β) levels in gingival crevicular fluid during human orthodontic tooth movement. Am. J. Orthod. Dentofacial Orthop. 105, 369–374. doi: 10.1016/S0889-5406(94)70131-8
Guasch, R. M., Tomas, M., Miñambres, R., Valles, S., Renau-Piqueras, J., and Guerri, C. (2003). RhoA and lysophosphatidic acid are involved in the actin cytoskeleton reorganization of astrocytes exposed to ethanol. J. Neurosci. Res. 15, 487–502. doi: 10.1002/jnr.10594
Han, J. H., Tian, H. Z., Lian, Y. Y., Yu, Y., Lu, C. B., Li, X. M., et al. (2015). Quetiapine mitigates the ethanol-induced oxidative stress in brain tissue, but not in the liver, of the rat. Neuropsychiatr. Dis. Treat. 15, 1473–1482. doi: 10.2147/NDT.S80505
Haorah, J., Schall, K., Ramirez, S. H., and Persidsky, Y. (2008). Activation of protein tyrosine kinases and matrix metalloproteinases causes blood-brain barrier injury: novel mechanism for neurodegeneration associated with alcohol abuse. Glia 56, 78–88. doi: 10.1002/glia.20596
Haorah, J., Ramirez, S. H., Schall, K., Smith, S., Pandya, R., and Persidsky, Y. (2007). Oxidative stress activates protein tyrosine kinase and matrix metalloproteinases leading to blood-brain barrier dysfunction. J. Neurochem. 101, 566–576. doi: 10.1111/j.1471-4159.2006.04393.x
Harris, C. K., Warnakulasuriya, K. A., Cooper, D. J., Peters, T. J., and Gelbier, S. (2004). Prevalence of oral mucosal lesions in alcohol misusers in south London. J. Oral Pathol. Med. 33, 253–259. doi: 10.1111/j.0904-2512.2004.00142.x
Harris, C. K., Warnakulasuriya, K. A., Johnson, N. W., Gelbier, S., and Peters, T. J. (1996). Oral health in alcohol misusers. Community Dent. Health. 13, 199–203.
He, Y., Macarak, E. J., Korostoff, J. M., and Howard, P. S. (2004). Compression and tension: differential effects on matrix accumulation by periodontal ligament fibroblasts in vitro. Connect. Tissue Res. 45, 28–39. doi: 10.1080/03008200490278124
Herrera, D. G., Yague, A. G., Johnsen-Soriano, S., Bosch-Morell, F., Collado-Morente, L., Muriach, M., et al. (2003). Selective impairment of hippocampal neurogenesis by chronic alcoholism: protective effects of an antioxidant. Proc. Natl. Acad. Sci. U.S.A. 100, 7919–7924. doi: 10.1073/pnas.1230907100
Huang, S. F., Li, Y. H., Ren, Y. J., Cao, Z. G., and Long, X. (2008). The effect of a single nucleotide polymorphism in the matrix metalloproteinase-1 (MMP-1) promoter on force-induced MMP-1 expression in human periodontal ligament cells. Eur. J. Oral Sci. 116, 319–323. doi: 10.1111/j.1600-0722.2008.00552.x
Ingman, T., Apajalahti, S., Mäntylä, P., Savolainen, P., and Sorsa, T. (2005). Matrix metalloproteinase 1 and 8 in GCF during orthodontic tooth movement: a pilot study during 1 month follow- up after fixed appliance activation. Eur. J. Orthod. 27, 202–207. doi: 10.1093/ejo/cjh097
Jeon, Y. M., Kook, S. H., Son, Y. O., Kim, E. M., Park, S. S., Kim, J. G., et al. (2009). Role of MAPK in mechanical force-induced up-regulation of type I collagen and osteopontin in human gingival fibroblasts. Mol. Cell. Biochem. 320, 45–52. doi: 10.1007/s11010-008-9897-z
Jiang, D., Liang, J., Fan, J., Yu, S., Chen, S., Luo, Y., et al. (2005). Regulation of lung injury and repair by Toll-like receptors and hyaluronan. Nat. Med. 11, 1173–1179. doi: 10.1038/nm1315
Johnsen-Soriano, S., Bosch-Morell, F., Miranda, M., Asensio, S., Barcia, J. M., Romá, J., et al. (2007). Ebselen prevents chronic alcohol-induced rat hippocampal stress and functional impairment. Alcohol. Clin. Exp. Res. 31, 486–492. doi: 10.1111/j.1530-0277.2006.00329.x
Katagiri, T., and Takahashi, N. (2002). Regulatory mechanisms of osteoblast and osteoclast differentiation. Oral Dis. 8, 147–159. doi: 10.1034/j.1601-0825.2002.01829.x
Kharbanda, K. K., McVicker, D. L., Zetterman, R. K., and Donohue, T. M. (1995). Ethanol consumption reduces the proteolytic capacity and protease activities of hepatic lysosomes. Biochim. Biophys. Acta 1245, 421–429. doi: 10.1016/0304-4165(95)00121-2
Kharbanda, K. K., McVicker, D. L., Zetterman, R. K., and Donohue, T. M. (1996). Ethanol consumption alters trafficking of lysosomal enzymes and affects the processing of procathepsin L in rat liver. Biochim. Biophys. Acta 1291, 45–52. doi: 10.1016/0304-4165(96)00043-8
Khocht, A., Janal, M., Schleifer, S., and Keller, S. (2003). The influence of gingival margin recession on loss of clinical attachment in alcohol-dependent patients without medical disorders. J. Periodontol. 74, 485–493. doi: 10.1902/jop.2003.74.4.485
Khocht, A., Schleifer, S., Janal, M., and Keller, S. (2013). Neutrophil function and periodontitis in alcohol-dependent males without medical disorders. J. Int. Acad. Periodontol. 15, 68–74.
Kikkert, R., Laine, M. L., Aarden, L. A., and van Winkelhoff, A. J. (2007). Activation of toll-like receptors 2 and 4 by gram-negative periodontal bacteria. Oral Microbiol. Immunol. 22, 145–151. doi: 10.1111/j.1399-302X.2007.00335.x
Kim, S. J., Ju, A., Lim, S. G., and Kim, D. J. (2013). Chronic alcohol consumption, type 2 Diabetes mellitus, insulin-like growth factor-I (IGF-I), and growth hormone (GH) in ethanol-treated diabetic rats. Life Sci. 93, 778–782. doi: 10.1016/j.lfs.2013.09.018
Koch, O. R., Pani, G., Borrello, S., Colavitti, R., Cravero, A., Farrè, S., et al. (2004). Oxidative stress and antioxidant defenses in ethanol-induced cell injury. Mol. Aspects Med. 25, 191–198. doi: 10.1016/j.mam.2004.02.019
Kong, Y. Y., Feige, U., Sarosi, I., Bolon, B., Tafuri, A., Morony, S., et al. (1999). Activated T cells regulate bone loss and joint destruction in adjuvant arthritis through osteoprotegerin ligand. Nature 402, 304–309. doi: 10.1038/46303
Kook, S. H., Hwang, J. M., Park, J. S., Kim, E. M., Heo, J. S., Jeon, Y. M., et al. (2009). Mechanical force induces type I collagen expression in human periodontal ligament fibroblasts through activation of ERK/JNK and AP-1. J. Cell. Biochem. 106, 1060–1067. doi: 10.1002/jcb.22085
Kook, S. H., Jang, Y. S., and Lee, J. C. (2011). Involvement of JNK-AP-1 and ERK-NF-κB signaling in tension-stimulated expression of Type I collagen and MMP-1 in human periodontal ligament fibroblasts. J. Appl. Physiol. 111, 1575–1583. doi: 10.1152/japplphysiol.00348.2011
Koop, D. R., Morgan, E. T., Tarr, G. E., and Coon, M. J. (1982). Purification and characterization of a unique isozyme of cytochrome P-450 from liver microsomes of ethanol-treated rabbits. J. Biol. Chem. 257, 8472–8480.
Kousteni, S., Bellido, T., Plotkin, L. I., O'Brien, C. A., Bodenner, D. L., Han, K., et al. (2001). Nongenotropic, sex-nonspecific signaling through the estrogen or androgen receptors: dissociation from transcriptional activity. Cell 104, 719–730. doi: 10.1016/s0092-8674(02)08100-x
Kranzler, H., Babor, T., Goldstein, L., and Gold, J. (1990). Dental pathology and alcohol related indicators in an out patient clinic sample. Community Dent. Oral Epidemiol. 18, 204–207. doi: 10.1111/j.1600-0528.1990.tb00058.x
Krishnan, V., and Davidovitch, Z. (2006). Cellular, molecular, and tissue-level reactions to orthodontic force. Am. J. Orthod. Dentofacial Orthop. 129, 469.e1–32. doi: 10.1016/j.ajodo.2005.10.007
Kurol, J., and Owman-Moll, P. (1998). Hyalinization and root resorption during early orthodontic tooth movement in adolescents. Angle Orthod. 68, 161–165.
Kyriakis, J. M., and Avruch, J. (2001). Mammalian mitogen-activated protein kinase signal transduction pathways activated by stress and inflammation. Physiol. Rev. 81, 807–869.
Leonardi, R., Talic, N. F., and Loreto, C. (2006). MMP-13 (collagenase 3) immunolocalisation during initial orthodontic tooth movement in rats. Acta Histochem. 109, 215–220. doi: 10.1016/j.acthis.2007.01.002
Lieber, C. S., and DeCarli, L. M. (1970). Hepatic microsomal ethanol-oxidizing system. In vitro characteristics and adaptive properties in vivo. J. Biol. Chem. 245, 2505–2512.
Liedert, A., Kaspar, D., Blakytny, R., Claes, L., and Ignatius, A. (2006). Signal transduction pathways involved in mechanotransduction in bone cells. Biochem. Biophys. Res. Commun. 349, 1–5. doi: 10.1016/j.bbrc.2006.07.214
Lisboa, R. A., Andrade, M. V., and Cunha-Melo, J. R. (2013). Toll-like receptor activation and mechanical force stimulation promote the secretion of matrix metalloproteinases 1,3 and 10 of human periodontal fibroblasts via p38, JNK and NF-κB. Arch. Oral Biol. 58, 731–739. doi: 10.1016/j.archoralbio.2012.12.009
Liu, W., and Zhang, X. (2015). Receptor activator of nuclear factor-κB ligand (RANKL)/RANK/osteoprotegerin system in bone and other tissues. Mol. Med. Rep. 11, 3212–3218. doi: 10.3892/mmr.2015.3152
Maeda, A., Soejima, K., Bandow, K., Kuroe, K., Kakimoto, K., Miyawaki, S., et al. (2007). Force-induced IL-8 from periodontal ligament cells requires IL-1α. J. Dent. Res. 86, 629–634. doi: 10.1177/154405910708600709
Manolagas, S. C. (2000). Birth and death of bone cells: basic regulatory mechanisms and implications for the pathogenesis and treatment of osteoporosis. Endocr. Rev. 21, 115–137. doi: 10.1210/er.21.2.115
Marques, T. C., Sarracini, K. L., Cortellazzi, K. L., Mialhe, F. L., de Castro-Meneghim, M., Pereira, A. C., et al. (2015). The impact of oral health conditions, socioeconomic status and use of specific substances on quality of life of addicted persons. BMC Oral Health 15:38. doi: 10.1186/s12903-015-0016-8
Martin, M., Rehani, K., Jope, R. S., and Michalek, S. M. (2005). Toll-like receptor-mediated cytokine production is differentially regulated by glycogen synthase kinase 3. Nat. Immunol. 6, 777–784. doi: 10.1038/ni1221
Martin, T. J., and Sims, N. A. (2015). RANKL/OPG; critical role in bone physiology. Rev. Endocr. Metab. Disord. 16, 131–139. doi: 10.1007/s11154-014-9308-6
Martinez-Gil, N., Flores-Bellver, M., Atienzar-Aroca, S., Lopez-Malo, D., Urdaneta-Alba, C., Sancho-Pelluz, F. J., et al. (2015). CYP2E1 in the human retinal pigment epithelium: expression, activity, and induction by ethanol. Invest. Ophthalmol. Vis. Sci. 11, 6855–6963. doi: 10.1167/iovs.14-16291
McKenna, S. (2006). Dental management of patients with diabetes. J. Dent. Clin. North Am. 50, 591–606. doi: 10.1016/j.cden.2006.06.008
Medzhitov, R. (2001). Toll-like receptors and innate immunity. Nat. Rev. Immunol. 1, 135–145. doi: 10.1038/35100529
Medzhitov, R., and Janeway, C. A. (1997). Innate immunity: impact on the adaptive immune response. Curr. Opin. Immunol. 9, 4–9. doi: 10.1016/S0952-7915(97)80152-5
Meeran, N. A. (2012). Biological response at the cellular level within the periodontal ligament on application of orthodontic force. An update. J. Orthodont. Sci. 1, 2–10. doi: 10.4103/2278-0203.94769
Meikle, M. C. (2006). The tissue, cellular, and molecular regulation of orthodontic tooth movement: 100 years after Carl Sandstedt. Eur. J. Orthod. 28, 221–240. doi: 10.1093/ejo/cjl001
Meng, R., Song, M., and Pan, J. (2015). Rho is involved in periodontal tissue remodelling with experimental tooth movement in rats. Arch. Oral Biol. 60, 923–931. doi: 10.1016/j.archoralbio.2015.01.017
Meng, Y., Bai, D., Han, X. L., Liu, M., Huang, L., and Bao, L. N. (2007). Proliferation of myofibroblast in periodental tension side during orthodontic tooth movement of beagle dogs. Sichuan Da Xue Xue Bao Yi Xue Ban. 38, 123–125.
Meng, Y., Han, X., Huang, L., Bai, D., Yu, H., He, Y., et al. (2010). Orthodontic mechanical tension effects on the myofibroblast expression of alpha-smooth muscle actin. Angle Orthodont. 80, 912–918. doi: 10.2319/101609-578.1
Miksys, S. L., and Tyndale, R. F. (2002). Drug-metabolizing cytochrome P450s in the brain. J. Psychiatry Neurosci. 27, 406–441.
Nakagawa, M., Kukita, T., Nakasima, A., and Kurisu, K. (1994). Expression of the type I collagen gene in rat periodontal ligament during tooth movement as revealed by in situ hybridization. Arch. Oral Biol. 39, 289–294. doi: 10.1016/0003-9969(94)90119-8
Nakano, Y., Yamaguchi, M., Fujita, S., Asano, M., Saito, K., and Kasai, K. (2011). Expressions of RANKL/RANK and M-CSF/c-fms in root resorption lacunae in rat molar by heavy orthodontic force. Eur. J. Orthod. 33, 335–343. doi: 10.1093/ejo/cjq068
Nelson, D. E., Jarman, D. W., Rehm, J., Greenfield, T. K., Rey, G., Kerr, W. C., et al. (2013). Alcohol-attributable cancer deaths and years of potential life lost in the United States. Am. J. Public Health. 103, 641–648. doi: 10.2105/AJPH.2012.301199
Noble, P. W., McKee, C. M., Cowman, M., and Shin, H. S. (1996). Hyaluronan fragments activate an NF-κB/I-κBα autoregulatory loop in murine macrophages. J. Exp. Med. 183, 2373–2378. doi: 10.1084/jem.183.5.2373
Nussbaum, G., Ben-Adi, S., Genzler, T., Sela, M., and Rosen, G. (2009). Involvement of Toll-like receptors 2 and 4 in the innate immune response to Treponema denticola and its outer sheath components. Infect. Immun. 77, 3939–3947. doi: 10.1128/IAI.00488-09
Ofori-Adjei, D., Casswell, S., Drummond, C., Wei, H., Medina-Mora, M. E., Ranganathan, S., et al. (2007). World Health Organization Expert Committee on Problems Related to Alcohol Consumption, Second Report. Geneva: WHO.
Oshiro, T., Shiotani, A., Shibasaki, Y., and Sasaki, T. (2002). Osteoclast induction in periodontal tissue during experimental movement of incisors in osteoprotegerin-deficient mice. Anat. Rec. 266, 218–225. doi: 10.1002/ar.10061
Pan, J. S., Han, Y., Chen, D. P., Xu, L., Qi, Y. X., and Yan, Z. Q. (2010). Cyclic strain promotes migration of human periodontal ligament cell via extracellular signal-regulated kinase (ERK) signaling pathway. Zhonghua Kou Qiang Yi Xue Za Zhi. 45, 80–84. doi: 10.3760/cma.j.issn.1002-0098.2010.02.005
Pascual, M., Baliño, P., Aragón, C. M. G., and Guerri, C. (2015). Cytokines and chemokines as biomarkers of ethanol-induced neuroinflammation and anxiety-related behavior: role of TLR4 and TLR2. Neuropharmacol. 89, 352–359. doi: 10.1016/j.neuropharm.2014.10.014
Peverali, F. A., Basdra, E. K., and Papavassiliou, A. G. (2001). Stretch-mediated activation of selective MAPK subtypes and potentiation of AP-1 binding in human osteoblastic cells. Mol. Med. 7, 68–78.
Plapp, B. V., Leidal, K. G., Murch, B. P., and Green, D. W. (2015). Contribution of liver alcohol dehydrogenase to metabolism of alcohols in rats. Chem. Biol. Interact. 178, 16–23. doi: 10.1016/j.cbi.2014.12.040
Ramachandran, V., Watts, L. T., Maffi, S. K., Chen, J., Schenker, S., and Henderson, G. (2003). Ethanol-induced oxidative stress precedes mitochondrially mediated apoptotic death of cultured fetal cortical neurons. J. Neurosci. Res. 74, 577–588. doi: 10.1002/jnr.10767
Redetzki, H. M. (1960). Alcohol-aldehyde transhydrogenation with liver alcohol dehydrogenase. Tex. Rep. Biol. Med. 18, 83–92.
Reichl, F. X., Seiss, M., Buters, J., Behrendt, H., Hickel, R., and Durner, J. (2010). Expression of CYP450-2E1 and formation of 2,3-epoxymethacrylic acid (2,3-EMA) in human oral cells exposed to dental materials. Dent. Mater. 26, 1151–1156. doi: 10.1016/j.dental.2010.08.005
Ridley, A. J. (2001). Rho proteins, PI 3-kinases, and monocyte/macrophage motility. FEBS Lett. 498, 168–171. doi: 10.1016/S0014-5793(01)02481-4
Rooban, T., Vidya, K., Joshua, E., Rao, A., Ranganathan, S., Rao, U. K., et al. (2011). Tooth decay in alcohol and tobacco abusers. J. Oral. Maxillofac. Pathol. 15, 14–21. doi: 10.4103/0973-029X.80032
Roux, P. P., and Blenis, J. (2004). ERK and p38 MAPK-activated protein kinases: a family protein kinases with diverse biological functions. Microbiol. Mol. Biol. Rev. 68, 320–344. doi: 10.1128/MMBR.68.2.320-344.2004
Sastry, S. K., and Burridge, K. (2000). Focal adhesions: a nexus for intracellular signaling and cytoskeletal dynamics. Exptl. Cell. Res. 261, 25–36. doi: 10.1006/excr.2000.5043
Schoppet, M., Preissner, K. T., and Hofbauer, L. C. (2002). RANK ligand and osteoprotegerin: paracrine regulators of bone metabolism and vascular function. Arterioscler. Thromb. Vasc. Biol. 22, 549–553. doi: 10.1161/01.ATV.0000012303.37971.DA
Seitz, H. K., and Wang, X. D. (2013). “The role of cytochrome P450 2E1 in ethanol-mediated carcinogenesis,” in Cytochrome P450 2E1: Its Role in Disease and Drug Metabolism, ed A. Dey (Dordrecht: Springer), 131–143.
Sugiyama, Y., Yamaguchi, M., Kanekawa, M., Yoshii, M., Nozoe, T., Nogimura, A., et al. (2003). The level of cathepsin B in GCF during human orthodontic tooth movement. Eur. J. Orthod. 25, 71–76. doi: 10.1093/ejo/25.1.71
Sun, A. Y., Chen, Y. M., James-Kracke, M., Wixom, P., and Cheng, Y. (1997). Ethanol-induced cell death by lipid peroxidation in PC12 cells. Neurochem. Res. 22, 1187–1192. doi: 10.1023/A:1021968526696
Surkin, P. N., Ossola, C. A., Mohn, C. E., Elverdin, J. C., and Fernández-Solari, J. (2014). Chronic alcohol consumption alters periodontal health in rats. Alcohol. Clin. Exp. Res. 38, 2001–2007. doi: 10.1111/acer.12436
Suzuki, R., Nemoto, E., and Shimauchi, H. (2014). Cyclic tensile force up-regulates BMP-2 expression through MAP kinase and COX-2/PGE2 signaling pathways in human periodontal ligament cells. Exp. Cell. Res. 323, 232–241. doi: 10.1016/j.yexcr.2014.02.013
Syed, F., and Khosla, S. (2005). Mechanisms of sex steroid effects on bone. Biochem. Biophys. Res. Commun. 328, 688–696. doi: 10.1016/j.bbrc.2004.11.097
Takeuchi, O., and Akira, S. (2001). Toll-like receptors: their physiological role and signal transduction system. Int. Immunopharmacol. 1, 625–635. doi: 10.1016/S1567-5769(01)00010-8
Taylor, K. R., Yamasaki, K., Radek, K. A., di Nardo, A., Goodarzi, H., Golenbock, D., et al. (2007). Recognition of hayluronan released in sterile injury involves a unique receptor complex dependent on Toll-like receptor 4, CD44, and MD-2. J. Biol. Chem. 282, 18265–18275. doi: 10.1074/jbc.M606352200
Torres, M. (2003). Mitogen-activated protein kinase pathways in redox signaling. Front. Biosci. 8, d369–d391. doi: 10.2741/999
Tsutsumi, M., Lasker, J. M., Takahashi, T., and Lieber, C. S. (1993). In vivo induction of hepatic P4502E1 by ethanol: role of increased enzyme synthesis. Arch. Biochem. Biophys. 304, 209–218. doi: 10.1006/abbi.1993.1341
Turner, R. T. (2000). Skeletal response to alcohol. Alcohol. Clin. Exp. Res. 24, 1693–1701. doi: 10.1111/j.1530-0277.2000.tb01971.x
Turner, R. T., and Sibonga, J. D. (2001). Effects of alcohol use and estrogen on bone. Alcohol. Res. Health 25, 276–281.
Tyrovola, J. B., Spyropoulos, M. N., Makou, M., and Perrea, D. (2008). Root resorption and the OPG/RANKL/RANK system: a mini review. J. Oral Sci. 50, 367–376. doi: 10.2334/josnusd.50.367
Uematsu, S., Mogi, M., and Deguchi, T. (1996). Interleukin (IL)-1β, IL-6, tumor necrosis factor-α, epidermal growth factor, and β2-microglobulinlevels are elevated in gingival crevicular fluid during human orthodontic tooth movement. J. Dent. Res. 75, 562–567. doi: 10.1177/00220345960750010801
Vaneker, M., Joosten, L. A., Heunks, L. M., Snijdelaar, D. G., Halbertsma, F. J., van Egmond, J., et al. (2008). Low-tidal-volume mechanical ventilation induces a toll-like receptor 4-dependent inflammatory response in healthy mice. Anesthesiology 109, 465–472. doi: 10.1097/ALN.0b013e318182aef1
Vemula, S., Shi, J., Hanneman, P., Wei, L., and Kapur, R. (2010). ROCK1 functions as a suppressor of inflammatory cell migration by regulating PTEN phosphorylation and stability. Blood 115, 1785–1796. doi: 10.1182/blood-2009-08-237222
Vernillo, A. T. (2001). Diabetes mellitus: relevance to dental treatment. Oral Surg. Oral Med. Oral Pathol. Oral Radiol Endod. 91, 263–270. doi: 10.1067/moe.2001.114002
Villarino, M. E., Lewicki, M., and Ubios, A. M. (2011). Bone response to orthodontic forces in diabetic Wistar rats. Am. J. Orthod. Dentofacial Orthop. 139 (4 Suppl.), S76–S82. doi: 10.1016/j.ajodo.2010.06.021
Von den Hoff, J. W. (2003). Effects of mechanical tension on matrix degradation by human periodontal ligament cells cultured in collagen gels. J. Periodontal Res. 38, 449–457. doi: 10.1034/j.1600-0765.2003.00404.x
Wang, H., Brown, J., and Martin, M. (2011). Glycogen synthase kinase 3: a point of convergence for the host inflammatory response. Cytokine 53, 130–140. doi: 10.1016/j.cyto.2010.10.009
Wang, M. J., Kuo, J. S., Lee, W. W., Huang, H. Y., Chen, W. F., and Lin, S. Z. (2006). Translational event mediates differential production of tumor necrosis factor-α in hyaluronan-stimulated microglia and macrophages. J. Neurochem. 97, 857–871. doi: 10.1111/j.1471-4159.2006.03776.x
Wang, N., Butler, J. P., and Ingber, D. E. (1993). Mechanotransduction across the cell surface and through the cytoskeleton. Science 269, 1124–1127. doi: 10.1126/science.7684161
Waszkiewicz, N., Zalewska, A., Szajda, S. D., Szulc, A., Kępka, A., Minarowska, A., et al. (2012). The effect of chronic alcohol intoxication and smoking on the activity of oral peroxidase. Folia Histochem. Cytobiol. 50, 450–455. doi: 10.5603/FHC.2012.0062
Waszkiewicz, N., Zalewska, A., Szulc, A., Kepka, A., Konarzewska, B., Zalewska-Szajda, B., et al. (2011). The influence of alcohol on the oral cavity, salivary glands and saliva. Pol. Merkur. Lekarski. 30, 69–74.
Wennerberg, K., Rossman, K. L., and Der, C. J. (2005). The Ras superfamily at a glance. J. Cell Sci. 118, 843–846. doi: 10.1242/jcs.01660
Yan, Y., Zeng, W., Song, S., Zhang, F., He, W., Liang, W., et al. (2013). Vitamin C induces periodontal ligament progenitor cell differentiation via activation of ERK pathway mediated by PELP1. Protein Cell. 4, 620–627. doi: 10.1007/s13238-013-3030-0
Yang, R. B., Mark, M. R., Gray, A., Huang, A., Xie, M. H., Zhang, M., et al. (1998). Toll-like receptor-2 mediates lipopolysaccharide-induced cellular signaling. Nature 395, 284–288. doi: 10.1038/26239
Zalewska, A., Waszkiewicz, N., Szajda, S. D., and Waszkiel, D. (2011). Impact of salivary flow and lysozyme content and output on the oral health of rheumatoid arthritis patients. Postepy. Hig. Med. Dosw. 65, 40–45. doi: 10.5604/17322693.932232
Zeng, T., Zhang, C. L., Song, F. Y., Zhao, X. L., and Xie, K. Q. (2014). CMZ reversed chronic ethanol-induced disturbance of PPAR-α possibly by suppressing oxidative stress and PGC-1α acetylation, and activating the MAPK and GSK3β pathway. PLoS ONE 9:e98658. doi: 10.1371/journal.pone.0098658
Zhang, L., Li, X., and Bi, L. J. (2011). Alterations of collagen-I, MMP-1 and TIMP-1 in the periodontal ligament of diabetic rats under mechanical stress. J. Periodontal. Res. 46, 448–455. doi: 10.1111/j.1600-0765.2011.01359.x
Zhang, X., Schuppan, D., Becker, J., Reichart, P., and Gelderblom, H. R. (1993). Distribution of undulin, tenascin, and fibronectin in the human periodontal ligament and cementum: comparative immunoelectron microscopy with ultra-thin cryosections. J. Histochem. Cytochem. 41, 245–251. doi: 10.1177/41.2.7678270
Keywords: ethanol, oxidative stress, orthodontic movement, periodontal ligament, orthodontic treatment
Citation: Barcia JM, Portolés S, Portolés L, Urdaneta AC, Ausina V, Pérez-Pastor GMA, Romero FJ and Villar VM (2017) Does Oxidative Stress Induced by Alcohol Consumption Affect Orthodontic Treatment Outcome? Front. Physiol. 8:22. doi: 10.3389/fphys.2017.00022
Received: 24 October 2016; Accepted: 10 January 2017;
Published: 25 January 2017.
Edited by:
Gareth Davison, Ulster University, UKReviewed by:
Deepesh Pandey, Johns Hopkins University, USAHelaine Mary Alessio, Miami University, USA
Copyright © 2017 Barcia, Portolés, Portolés, Urdaneta, Ausina, Pérez-Pastor, Romero and Villar. This is an open-access article distributed under the terms of the Creative Commons Attribution License (CC BY). The use, distribution or reproduction in other forums is permitted, provided the original author(s) or licensor are credited and that the original publication in this journal is cited, in accordance with accepted academic practice. No use, distribution or reproduction is permitted which does not comply with these terms.
*Correspondence: Francisco J. Romero, ZnJhbmNpc2NvamF2aWVyLnJvbWVyb0B1bml2ZXJzaWRhZGV1cm9wZWEuZXM=