- 1Department of Endocrinology and Internal Medicine, University of Arkansas for Medical Sciences, Little Rock, AR, USA
- 2GIM Foundation, Little Rock, AR, USA
- 3Department of Internal Medicine and Hospital Medicine, Christus Trinity Mother Frances Hospital, Tyler, TX, USA
- 4Department of Policy, University of Arkansas for Little Rock, Little Rock, AR, USA
- 5Tutwiler Clinic, Tutwiler, MS, USA
- 6Department of Pulmonary and Critical Care Medicine, University of Arkansas for Medical Sciences, Little Rock, AR, USA
- 7Winthrop P. Rockefeller Cancer Institute, University of Arkansas for Medical Sciences, Little Rock, AR, USA
- 8St. Vincent Infirmary (Catholic Health Initiative), Little Rock, AR, USA
- 9The Wright Center, Scranton, PA, USA
A commentary on
ATP: The crucial component of secretory vesicles
by Estévez-Herrera, J., Domínguez, N., Pardo, M. R., González-Santana, A., Westhead, E. W., Borges, R., et al. (2016). Proc. Natl. Acad. Sci. U.S.A. 113, E4098–E4106. doi: 10.1073/pnas.1600690113
We are aware of concepts of osmotic and oncotic pressure in clinical physiology. Per Starling's law, the freely movable ions do not significantly contribute to the retention of vascular volume, or the maintenance of interstitial fluid pressure (Jacob and Chappell, 2013). On the other hand, albumin, due to its molecular size and inefficiency of free diffusion through the transcellular pores, assumes a major role of maintaining oncotic pressure and retaining fluid within the vascular space. The implications are obvious: either the lack of protein synthesis in liver diseases or loss of protein through the leaky glomerulus in nephrotic conditions leads to deficiency of oncotic pressure, fluid leak and its accumulation in tissue spaces.
In a recent manuscript by Estévez-Herrera et al. (2016), a relatively newly appreciated biophysical role of ATP is elucidated. Namely, the authors provide evidence using in vitro chromaffin cells that ATP agglomerates peptides and amines within the vesicles, thus reducing their particle numbers and effectively reducing the osmotic pressure within the vesicles. This may be critically important for maintaining isotonicity and survival of the vesicles in the cytosolic matrix.
ATP is a highly negatively charged molecule. It cannot simply diffuse through the membrane. Though the existence of a vesicular nucleotide transporter (VNUT) was predicted for a long time (Stiernet et al., 2006), the solute carrier protein SLC17A9 was only recently identified as the channel that transports ATP through the membrane (Sawada et al., 2008). Since its discovery, several studies have shown existence of SLC17A9 in enteric synaptic vesicles (Chaudhury et al., 2012), biliary epithelial microvesicles (Sathe et al., 2011), in the central nervous system (Larsson et al., 2012) and in the beta cells of the pancreas (Sakamoto et al., 2014).
In the beta cells of the pancreas, the insulin granules are not freely soluble molecules in the cytosol but rather exists as packaged vesicles. Electron micrographs of beta cells and physiological experiments have revealed that these insulin granules undergo regulated exocytosis to a glucose load, rather than random secretion (Dean, 1973). Potassium-sensitive ATP channels have been shown to play an important role for sensing of the glucose load and initiation of the exocytosis (Rutter and Hill, 2006).
Recently, it has been suggested that the exocytosis involving insulin granules resembles remarkable similarity to the pattern of inhibitory enteric neuromuscular neurotransmission (Chaudhury, 2014). Insulin granule exocytosis involves sequential release of ATP and the gaseous nitric oxide (NO), which may have important regulatory roles.
The SLC17A9 channel transports ATP within the insulin containing dense core vesicles (Sawada et al., 2008). In VNUT−/− mice, the number and appearances of secretory granules in islet β-cells of knockout mice were normal (Sakamoto et al., 2014). However, insulin exocytosis was accelerated (Sakamoto et al., 2014) The recent manuscript by Estévez-Herrera et al. (2016) may potentially explain this significant observation. It is well known that insulin hypersecretion is an early stage pathophysiology which occurs in genetic obesity and prediabetes (Gonzalez et al., 2013; Irles et al., 2015). When VNUT is absent or deficient, ATP fails to enter the beta cells, or may load the vesicles suboptimally (Sakamoto et al., 2014; Figure 1). The decrease of ATP within the vesicle may alter the consistency of the dense crystalline core of the insulin. Thus, the vesicular osmotic pressure may be considerably elevated, leading to rapid exocytosis. Additionally, due to the lack of insulin polymer formation with ATP, the granular exocytosis may be sped up. These aspects remains to be examined.
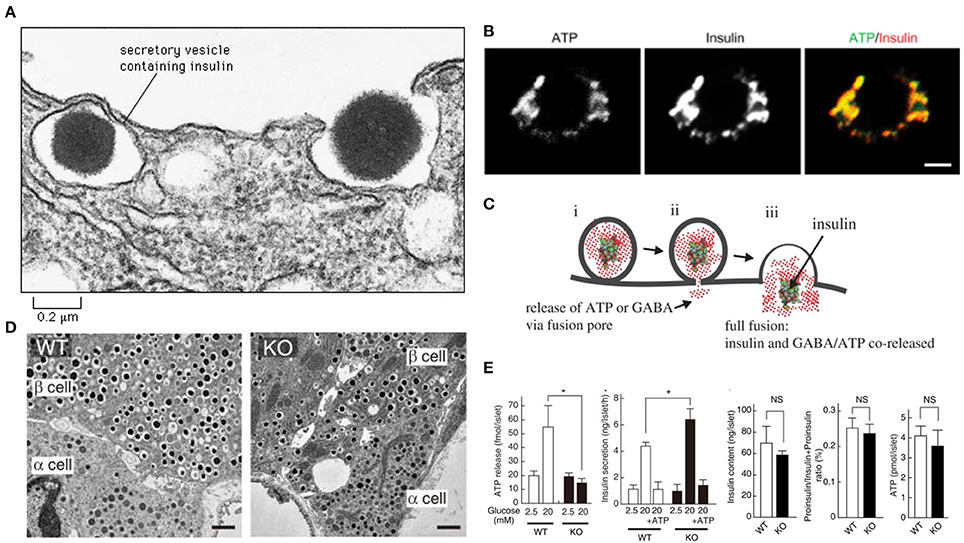
Figure 1. ATP may contribute to colligative property of insulin containing vesicles (A). Note the exocytotic insulin vesicle and the dense insulin cluster in the electron micrograph. Recent study by Estévez-Herrera et al. (2016) suggest that ATP may contribute to colligative and osmotic balance of peptide containing vesicles. Such may be true for insulin vesicles (B). ATP and insulin colocalize in beta cells of pancreas. Scale bar, 2 μm (C). During exocytosis, both ATP and insulin are co-released. This co-release may have wide implications as discussed in the commentary (D). In SLC17A9 knockout, the insulin containing vesicles are not altered in number or appearance (E). In SLC17A9 knockout, the ATP and insulin contents of vesicles are decreased. But note that insulin exocytosis in SLC17A9 knockout is accelerated. This may occur due to lack of formation of ATP-(insulin)n polymer. This rapid exocytosis is a feature of early prediabetic state, as well as seen in progressive type II diabetes mellitus. Reproduced with permission from Sakamoto et al. (2014) and Liu et al. (2014). *Significance.
Type II diabetes mellitus is characterized by progressive exhaustion of the beta cells of the pancreas (Butler et al., 2016). This leads to increased insulin resistance and finally leads to an insulin-dependent state. The rates of beta cell damage are stochastic, and are likely multifactorial, including the effect of ongoing treatment with anti-diabetic medications. The ATP content in SLC17A9 knockout mice was reduced, though not significantly (Sakamoto et al., 2014; Figure 1). It may be remembered that insulin granule exocytosis is very complex and may involve several protein interactors, association with the cellular cytoskeleton, motor proteins like myosin Va, complex spatial negotiations at the subcellular cortex and finally the interaction with the membrane, where the dynamic pore size may be a critical determinant of exocytosis of the insulin, which is released either as a monomer or dimer (Ashcroft et al., 1973; Rothman, 1994; Li et al., 1995; Sudhof, 1995; Ivarsson et al., 2005; Braun et al., 2009; Karanauskaite et al., 2009; Wang and Thurmond, 2009; Solimena and Speier, 2010; Galvanovskis et al., 2011; MacDonald, 2011; Andersson et al., 2012; Schvartz et al., 2012; Seino, 2012; Rosengren et al., 2012; Wiseman and Thurmond, 2012; Kalwat and Thurmond, 2013; Moghadam and Jackson, 2013; Rorsman and Braun, 2013; Stamper and Wang, 2013; Xie et al., 2013; Bergeron et al., 2014; Gaisano, 2014; Heaslip et al., 2014; Satoh, 2014; Sun et al., 2014; Hoang Do and Thorn, 2015; Rutter et al., 2015; Schumacher et al., 2015; Takahashi, 2015; Takahashi et al., 2015; Wuttke, 2015; Thorn et al., 2016). Some or all of these pathways may be affected or altered in SLC17A9 channelopathy.
In this commentary, we advance the hypothesis that SLC17A9 dysfunction may contribute to the ongoing inefficacy of insulin exocytosis, leading to progressive diabetes. The lack of entry of ATP through a dysfunctional SLC17A9 (Sakamoto et al., 2014; Estévez-Herrera et al., 2016) may lead to a labile insulin vesicle formation, with enhanced rates of exocytosis. This may lead to increased demand on the cell for further insulin biogenesis, which may lead to a cascade of genomic stress and ultimate beta cell death. The mechanisms involved in the rapid dissolution of the insulin crystal before and during exocytosis, is not well-studied. Newer methodologies may be employed to study the insulin porosome (Jena, 2009).
What causes SLC17A9 dysfunction is not clear. Interestingly, SLC17A9 is gated by ATP itself (Sawada et al., 2008). Thus, ATP generation may be a limiting factor. Recent evidence suggest the inefficiency of mitochondrial coupling function with progressive diabetes, leading to diminished ATP production (Maechler, 2013; Peiris et al., 2016). This may be a potential underlying mechanism for the SLC17A9 dysfunction. Also, the channel may be gated by NO. NO downstream effectors are present in the beta cells and NO is known to inhibit ATP loading of dense vesicles (Machado et al., 2000). Using a range of biophysical methods including dynamic light scattering, turbidimetry, circular dichroism and atomic force microscopy, an elegant study has demonstrated that the amino acid L-arginine (Arg) has the ability to influence insulin aggregation propensity (Smirnova et al., 2015). The modification of the net charge of insulin induced by increase in the pH level of the incubation medium resulted in major alterations clustering of Arg-insulin. Using pH markers like pHluorin, vesicle pH may be assessed, which may serve as a surrogate for the vesicle osmotic pressure, which is otherwise a challenging technique to perform. Here, we may recollect that acidification of the vesicles is the main driver of ATP entry within the vesicles (Sawada et al., 2008).
There have been recent advances of novel markers that can tag synaptic proteins, especially those acting on the membranes. PET imaging may detect these global aspects of neurotransmission (Finnema et al., 2016). It is possible that such novel imaging may be applied for estimating beta cell mass in progressive diabetes mellitus. This is a huge area of unmet need in diabetes mellitus management. SLC17A9 may be tagged and imaged with similar novel methodologies to estimate whether their quantitative distribution is altered in progressive diabetes. Currently, C peptide analyses is used as a crude method to estimate disease status and functional beta cell reserve. Our thesis provides a novel paradigm in approaching management of diabetes mellitus from a precision medicine perspective.
Author Contributions
ArC, conceptualized and development of manuscript, drafted manuscript. CD, major intellectual contribution. VD, major intellectual contribution. CM, important discussion. NS, important discussion. WM, important discussion and conceptualizations. RR, important discussion. AM, important discussion, manuscript recheck. AdR, important discussion and prospects. All authors read and approved final version of manuscript.
Conflict of Interest Statement
The authors declare that the research was conducted in the absence of any commercial or financial relationships that could be construed as a potential conflict of interest.
References
Andersson, S. A., Olsson, A. H., Esguerra, J. L., Heimann, E., Ladenvall, C., Edlund, A., et al. (2012). Reduced insulin secretion correlates with decreased expression of exocytotic genes in pancreatic islets from patients with type 2 diabetes. Mol. Cell Endocrinol. 364, 36–45. doi: 10.1016/j.mce.2012.08.009
Ashcroft, S. J., Weerasinghe, L. C., and Randle, P. J. (1973). Interrelationship of islet metabolism, adenosine triphosphate content and insulin release. Biochem. J. 132, 223–231.
Bergeron, A., Pucci, L., Bezzi, P., and Regazzi, R. (2014). Analysis of synaptic-like microvesicle exocytosis of B-cells using a live imaging technique. PLoS ONE 9:e87758. doi: 10.1371/journal.pone.0087758
Braun, M., Ramracheya, R., Johnson, P. R., and Rorsman, P. (2009). Exocytotic properties of human pancreatic beta-cells. Ann. N.Y. Acad. Sci. 1152, 187–193. doi: 10.1111/j.1749-6632.2008.03992.x
Butler, A. E., Dhawan, S., Hoang, J., Cory, M., Zeng, K., Fritsch, H., et al. (2016). β-Cell deficit in obese type 2 diabetes, a minor role of β-Cell dedifferentiation and degranulation. J. Clin. Endocrinol. Metab. 101, 523–532. doi: 10.1210/jc.2015-3566
Chaudhury, A. (2014). Similarity in Transcytosis of nNOSα in Enteric Nerve Terminals and Beta Cells of Pancreatic Islet. Front. Med. (Lausanne). 1:20. doi: 10.3389/fmed.2014.00020
Chaudhury, A., He, X. D., and Goyal, R. K. (2012). Role of myosin Va in purinergic vesicular neurotransmission in the gut. Am. J. Physiol. Gastrointest. Liver. Physiol. 302, G598–G607. doi: 10.1152/ajpgi.00330.2011
Estévez-Herrera, J., Domínguez, N., Pardo, M. R., González-Santana, A., Westhead, E. W., Borges, R., et al. (2016). ATP: The crucial component of secretory vesicles. Proc. Natl. Acad. Sci. U.S.A. 113, E4098–E4106. doi: 10.1073/pnas.1600690113
Finnema, S. J., Nabulsi, N. B., Eid, T., Detyniecki, K., Lin, S. F., Chen, M. K., et al. (2016). Imaging synaptic density in the living human brain. Sci Transl Med. 8:348ra96. doi: 10.1126/scitranslmed.aaf6667
Gaisano, H. Y. (2014). Here come the newcomer granules, better late than never. Trends Endocrinol. Metab. 25, 381–388. doi: 10.1016/j.tem.2014.03.005
Galvanovskis, J., Braun, M., and Rorsman, P. (2011). Exocytosis from pancreatic β-cells: mathematical modelling of the exit of low-molecular-weight granule content. Interface Focus. 1, 143–152. doi: 10.1098/rsfs.2010.0006
Gonzalez, A., Merino, B., Marroqu,í, L., Ñeco, P., Alonso-Magdalena, P., Caballero-Garrido, E., et al. (2013). Insulin hypersecretion in islets from diet-induced hyperinsulinemic obese female mice is associated with several functional adaptations in individual β-cells. Endocrinology 154, 3515–3524. doi: 10.1210/en.2013-1424
Heaslip, A. T., Nelson, S. R., Lombardo, A. T., Beck Previs, S., Armstrong, J., and Warshaw, D. M. (2014). Cytoskeletal dependence of insulin granule movement dynamics in INS-1 beta-cells in response to glucose. PLoS ONE 9:e109082. doi: 10.1371/journal.pone.0109082
Hoang Do, O., and Thorn, P. (2015). Insulin secretion from beta cells within intact islets: location matters. Clin. Exp. Pharmacol. Physiol. 42, 406–414. doi: 10.1111/1440-1681.12368
Irles, E., Ñeco, P., Lluesma, M., Villar-Pazos, S., Santos-Silva, J. C., Vettorazzi, J. F., et al. (2015). Enhanced glucose-induced intracellular signaling promotes insulin hypersecretion: pancreatic beta-cell functional adaptations in a model of genetic obesity and prediabetes. Mol. Cell Endocrinol. 404, 46–55. doi: 10.1016/j.mce.2015.01.033
Ivarsson, R., Jing, X., Waselle, L., Regazzi, R., and Renström, E. (2005). Myosin 5a controls insulin granule recruitment during late-phase secretion. Traffic 6, 1027–1035. doi: 10.1111/j.1600-0854.2005.00342.x
Jacob, M., and Chappell, D. (2013). Reappraising Starling: the physiology of the microcirculation. Curr. Opin. Crit. Care 19, 282–289. doi: 10.1097/MCC.0b013e3283632d5e
Jena, B. P. (2009). Secretory vesicles transiently dock and fuse at the porosome to discharge contents during cell secretion. Cell Biol. Int. 34, 3–12. doi: 10.1042/CBI20090161
Kalwat, M. A., and Thurmond, D. C. (2013). Signaling mechanisms of glucose-induced F-actin remodeling in pancreatic islet β cells. Exp. Mol. Med. 45:e37. doi: 10.1038/emm.2013.73
Karanauskaite, J., Hoppa, M. B., Braun, M., Galvanovskis, J., and Rorsman, P. (2009). Quantal ATP release in rat beta-cells by exocytosis of insulin-containing LDCVs. Pflug. Arch. 458, 389–401. doi: 10.1007/s00424-008-0610-6
Larsson, M., Sawada, K., Morland, C., Hiasa, M., Ormel, L., Moriyama, Y., et al. (2012). Functional and anatomical identification of a vesicular transporter mediating neuronal ATP release. Cereb. Cortex 22, 1203–1214 doi: 10.1093/cercor/bhr203
Li, C., Ullrich, B., Zhang, J. Z., Anderson, R. G., Brose, N., and Sudhof, T. C. (1995). Ca2+-dependent and -independent activities of neural and non-neural synaptotagmins. Nature 375, 594–599.
Liu, T., Li, H., Gounko, N. V., Zhou, Z., Xu, A., Hong, W., et al. (2014). Detection of insulin granule exocytosis by an electrophysiology method with high temporal resolutionreveals enlarged insulin granule pool in BIG3-knockout mice. Am. J. Physiol. Endocrinol. Metab. 307, E611–E618. doi: 10.1152/ajpendo.00208.2014
MacDonald, P. E. (2011). Signal integration at the level of ion channel and exocytotic function in pancreatic β-cells. Am. J. Physiol. Endocrinol. Metab. 301, E1065–E1069. doi: 10.1152/ajpendo.00426.2011
Machado, J. D., Segura, F., Brioso, M. A., and Borges, R. (2000). Nitric oxide modulates a late step of exocytosis. J. Biol. Chem. 275, 20274–20279. doi: 10.1074/jbc.M000930200
Maechler, P. (2013). Mitochondrial function and insulin secretion. Mol. Cell Endocrinol. 379, 12–18. doi: 10.1016/j.mce.2013.06.019.
Moghadam, P. K., and Jackson, M. B. (2013). The functional significance of synaptotagmin diversity in neuroendocrine secretion. Front. Endocrinol. (Lausanne). 4:124. doi: 10.3389/fendo.2013.00124
Peiris, H., Duffield, M. D., Fadista, J., Jessup, C. F., Kashmir, V., Genders, A. J., et al. (2016). A syntenic cross species aneuploidy genetic screen links RCAN1 expression to β-Cell mitochondrial dysfunction in Type 2 diabetes. PLoS Genet. 12:e1006033. doi: 10.1371/journal.pgen.1006033
Rorsman, P., and Braun, M. (2013). Regulation of insulin secretion in human pancreatic islets. Annu. Rev. Physiol. 75, 155–179. doi: 10.1146/annurev-physiol-030212-183754
Rosengren, A. H., Braun, M., Mahdi, T., Andersson, S. A., Travers, M. E., Shigeto, M., et al. (2012). Reduced insulin exocytosis in human pancreatic β-cells with gene variants linked to type 2 diabetes. Diabetes 61, 1726–1733. doi: 10.2337/db11-1516
Rutter, G. A., and Hill, E. V. (2006). Insulin vesicle release: walk, kiss, pause…then run. Physiology (Bethesda) 21, 189–196. doi: 10.1152/physiol.00002.2006
Rutter, G. A., Pullen, T. J., Hodson, D. J., and Martinez-Sanchez, A. (2015). Pancreatic β-cell identity, glucose sensing and the control of insulin secretion. Biochem. J. 466, 203–218. doi: 10.1042/BJ20141384
Sakamoto, S., Miyaji, T., Hiasa, M., Ichikawa, R., Uematsu, A., Iwatsuki, K., et al. (2014). Impairment of vesicular ATP release affects glucose metabolism and increases insulin sensitivity. Sci. Rep. 4:6689. doi: 10.1038/srep06689
Sathe, M. N., Woo, K., Kresge, C., Bugde, A., Luby-Phelps, K., Lewis, M. A., et al. (2011). Regulation of purinergic signaling in biliary epithelial cells by exocytosis of SLC17A9-dependent ATP-enriched vesicles. J. Biol. Chem. 286, 25363–25376. doi: 10.1074/jbc.M111.232868
Satoh, T. (2014). Rho GTPases in insulin-stimulated glucose uptake. Small GTPases. 5:e28102. doi: 10.4161/sgtp.28102
Sawada, K., Echigo, N., Juge, N., Miyaji, T., Otsuka, M., Omote, H., et al. (2008). Identification of a vesicular nucleotide transporter. Proc. Natl. Acad. Sci. U.S.A. 105, 5683–5686. doi: 10.1073/pnas.0800141105
Schumacher, K., Matz, M., Brüning, D., Baumann, K., and Rustenbeck, I. (2015). Granule mobility, fusion frequency and insulin secretion are differentially affected by insulinotropic stimuli. Traffic 16, 493–509. doi: 10.1111/tra.12261
Schvartz, D., Brunner, Y., Couté, Y., Foti, M., Wollheim, C. B., and Sanchez, J. C. (2012). Improved characterization of the insulin secretory granule proteomes. J. Proteomics 75, 4620–4631. doi: 10.1016/j.jprot.2012.04.023
Seino, S. (2012). Cell signalling in insulin secretion: the molecular targets of ATP, cAMP and sulfonylurea. Diabetologia 55, 2096–2108. doi: 10.1007/s00125-012-2562-9
Smirnova, E., Safenkova, I., Stein-Margolina, V., Shubin, V., Polshakov, V., and Gurvits, B. (2015). pH-responsive modulation of insulin aggregation and structural transformation of the aggregates. Biochimie 109, 49–59. doi: 10.1016/j.biochi.2014.12.006
Solimena, M., and Speier, S. (2010). Insulin release: shedding light on a complex matter. Cell Metab. 12, 5–6. doi: 10.1016/j.cmet.2010.06.002
Stamper, I. J., and Wang, X. (2013). Mathematical modeling of insulin secretion and the role of glucose-dependent mobilization, docking, priming and fusion of insulin granules. J. Theor. Biol. 318, 210–225. doi: 10.1016/j.jtbi.2012.11.002
Stiernet, P., Guiot, Y., Gilon, P., and Henquin, J.-C. (2006). Glucose acutely decreases pH of secretory granules in mouse pancreatic islets. Mechanisms and influence oninsulin secretion. J. Biol. Chem. 281, 22142–22151. doi: 10.1074/jbc.M513224200
Sudhof, T. C. (1995). The synaptic vesicle cycle: a cascade of protein-protein interactions. Nature 375, 645–653.
Sun, Y., Chiu, T. T., Foley, K. P., Bilan, P. J., and Klip, A. (2014). Myosin Va mediates Rab8A-regulated GLUT4 vesicle exocytosis in insulin-stimulated muscle cells. Mol. Biol. Cell. 25, 1159–1170. doi: 10.1091/mbc.E13-08-0493
Takahashi, N. (2015). Imaging analysis of insulin secretion with two-photon microscopy. Biol. Pharm. Bull. 38, 656–662. doi: 10.1248/bpb.b14-00880
Takahashi, N., Sawada, W., Noguchi, J., Watanabe, S., Ucar, H., Hayashi-Takagi, A., et al. (2015). Two-photon fluorescence lifetime imaging of primed SNARE complexes in presynaptic terminals and β cells. Nat. Commun. 6:8531. doi: 10.1038/ncomms9531
Thorn, P., Zorec, R., Rettig, J., and Keating, D. J. (2016). Exocytosis in non-neuronal cells. J. Neurochem. 137, 849–859. doi: 10.1111/jnc.13602
Wang, Z., and Thurmond, D. C. (2009). Mechanisms of biphasic insulin-granule exocytosis - roles of the cytoskeleton, small GTPases and SNARE proteins. J. Cell Sci. 122(Pt 7), 893–903. doi: 10.1242/jcs.034355
Wiseman, D. A., and Thurmond, D. C. (2012). The good and bad effects of cysteine S-nitrosylation and tyrosine nitration upon insulin exocytosis: a balancing act. Curr. Diab. Rev. 8, 303–315. doi: 10.2174/157339912800840514
Wuttke, A. (2015). Lipid signalling dynamics at the β-cell plasma membrane. Basic Clin. Pharmacol. Toxicol. 116, 281–290. doi: 10.1111/bcpt.12369
Keywords: exocytosis, SLC17A9, diabetes mellitus, cell secretion, ATP, purinergic
Citation: Duvoor C, Dendi VS, Marco A, Shekhawat NS, Chada A, Ravilla R, Musham CK, Mirza W and Chaudhury A (2017) Commentary: ATP: The crucial component of secretory vesicles: Accelerated ATP/insulin exocytosis and prediabetes. Front. Physiol. 8:53. doi: 10.3389/fphys.2017.00053
Received: 22 July 2016; Accepted: 19 January 2017;
Published: 02 February 2017.
Edited by:
Ghanshyam Upadhyay, City College of New York (CUNY), USAReviewed by:
Nagaraja Nagre, Eastern Virginia Medical School, USASantosh Kumar Maurya, Sanford Burnham Prebys Medical Discovery Institute, USA
Copyright © 2017 Duvoor, Dendi, Marco, Shekhawat, Chada, Ravilla, Musham, Mirza and Chaudhury. This is an open-access article distributed under the terms of the Creative Commons Attribution License (CC BY). The use, distribution or reproduction in other forums is permitted, provided the original author(s) or licensor are credited and that the original publication in this journal is cited, in accordance with accepted academic practice. No use, distribution or reproduction is permitted which does not comply with these terms.
*Correspondence: Arun Chaudhury, YXJ1bmNoYXVkaHVyeS5ib3N0b25AZ21haWwuY29t
†These authors have contributed equally to this work.