- 1The Unit of the Theory of Physical Education, The Chair of Physical Education, Gdansk University of Physical Education and Sport, Gdańsk, Poland
- 2Department of Movement Physiology, Bogomoletz Institute of Physiology, National Academy of Sciences, Kyiv, Ukraine
- 3Department of Hypoxic States Investigation, Bogomoletz Institute of Physiology, National Academy of Sciences, Kyiv, Ukraine
- 4ESC “Institute of Biology and Medicine", Taras Shevchenko National University of Kyiv, Kyiv, Ukraine
- 5Institute of Chemistry and Biotechnology, Technical University of Ilmenau, Ilmenau, Germany
- 6Lesya Ukrainka East European National University, Lutsk, Ukraine
The aim of this study is to detect the effects of C60 fullerenes, which possess pronounced antioxidant properties, in comparison with the actions of the known exogenous antioxidants N-acetylcysteine (NAC) and β-Alanine in terms of exercise tolerance and contractile property changes of the m. triceps surae (TS) during development of the muscle fatigue in rats. The electrical stimulation of the TS muscle during four 30 min series in control rats led to total reduction of the muscle contraction force. Furthermore, the effects of prior intraperitoneal (i.p.) or oral C60FAS application and preliminary i.p. injection of NAC or β-Alanine on muscle contraction force under fatigue development conditions is studied. In contrast to control rats, animals with C60FAS, NAC, or β-Alanine administration could maintain a constant level of muscle effort over five stimulation series. The accumulation of secondary products and changes in antioxidant levels in the muscle tissues were also determined after the fatigue tests. The increased levels of lactic acid, thiobarbituric acid reactive substances and H2O2 after stimulation were statistically significant with respect to intact muscles. In the working muscle, there was a significant (p < 0.05) increase in the activity of endogenous antioxidants: reduced glutathione, catalase, glutathione peroxidase, and superoxide dismutase. Treated animal groups showed a decrease in endogenous antioxidant activity relative to the fatigue-induced animals (P < 0.05). Oral C60FAS administration clearly demonstrated an action on skeletal muscle fatigue development similar to the effects of i.p. injections of the exogenous antioxidants NAC or β-Alanine. This creates opportunities to oral use of C60FAS as a potential therapeutic agent. Due to the membranotropic activity of C60 fullerenes, non-toxic C60FAS has a more pronounced effect on the prooxidant-antioxidant homeostasis of muscle tissues in rats.
Introduction
Fullerenes are a new kind of organic compounds, consisting of carbon atoms with very attractive photo-, electrochemical and physical properties that can be used in many biological fields, which make fullerenes potential therapeutic agents for a wide range of applications in nanomedicine (Anilkumar et al., 2011; Dellinger et al., 2013; Castro et al., 2017). With respect to its electron donor and acceptor capability fullerenes can be effective antioxidants and radical scavengers (Bosi et al., 2003; Bakry et al., 2007). It was shown that under the influence of light, fullerene acts as a prooxidant (Kamat et al., 2000), generating singlet oxygen (Bosi et al., 2003), which can be used in photodynamic therapy of cancer and other diseases. Some fullerene derivatives exhibit inhibitory activity against human immunodeficiency virus reverse transcriptase and hepatitis C virus RNA polymerase (Mashino et al., 2005; Nakamura and Mashino, 2012), and can stabilize immune effector cells to prevent or inhibit the release of proinflammatory mediators, making them potential candidates for a variety of diseases, such as asthma, arthritis, and multiple sclerosis (Dellinger et al., 2013). Thus, fullerenes and their derivatives are excellent candidates for multiple functionalization.
It is known, that muscle fatigue is accompanied by ionic changes in action potentials (Allen et al., 2008) along with various metabolic disturbances in skeletal muscles, when reactive oxygen species (ROS) are formed (Halliwell and Gutteridge, 1989), excess lactic acid (LA) (Sahlin et al., 1987), and lipid peroxidation (Venditti and Di Meo, 1997). With excessive accumulation of these substances, oxidative stress occurs, which leads to significant functional disorders since various components of cells may be damaged. The damage could include changes in protein structures, nitrogenous bases, and destruction of membranes (Powers and Jackson, 2008). Muscle cells contain endogenous cellular defense mechanisms in the form of enzymatic and non-enzymatic antioxidants that can partially eliminate ROS (Sen et al., 1994; Banerjee et al., 2003). A changes in the level of enzymes (SOD GPx, and CAT) under loading was observed in both animals and humans (Sen, 1995). During exhausted loads, the amount of SOD increased in skeletal muscle (Powers et al., 1999), whereas the level of GPX activity had not changed (Brady et al., 1979) or was increased (Powers et al., 1999). Similar phenomena were observed concerning CAT activity when no changes were observed during exercise (Powers et al., 1994a), and there was an increase in activity under certain loads (Ji and Fu, 1992). Thus, the understanding of the mechanisms involved in the process of increasing antioxidant enzymes during exhaustive exercise has yet to be under debate.
Intense muscle loading contributes to rise of oxidative stress in muscle tissue (Powers et al., 1999). The presence of ROS inhibits the tricarboxylic acids cycle and disrupts the mitochondria electronic transport chain (Janero and Hreniuk, 1996). With intense physical activity, the rate of hydrolysis of ATP may exceed the rate of its resynthesis, which leads to a decrease in ATP and the formation of muscle fatigue effects (Graham et al., 1978; Sahlin, 1985). However, the synthesis of ATP during training can be supported by antioxidants supplementation, which accelerate the process of muscle recovery after fatigue. There are evidences demonstrated a moderately beneficial effect of NAC (Supinski et al., 1997; Sandstrom et al., 2006) and β-Alanine (Stout et al., 2007; Ghiasvand et al., 2012; Summermatter and Handschin, 2012; Schnuck et al., 2016) supplementation for exercise with a substantial contribution from oxidative metabolism. It was shown unspecific antioxidant activity of NAC with increase GSH synthesis and reduce muscle-derived ROS levels during contraction (Sandstrom et al., 2006). Another study suggested a delay accumulation of lactate during exercise and increasing time to exhaustion under β-Alanine supplementation (Summermatter and Handschin, 2012). In our previous study, we showed a facilitation effect of water-soluble C60 fullerenes on the removal of some symptoms of skeletal muscle fatigue (Prylutskyy et al., 2017). So, we consider that because of novel field of C60 fullerenes application (muscle fatigue) it was important to assessed the adequacy and efficiency of C60 fullerene protective properties against oxidative damage in comparison with the action of known antioxidant NAC and β-Alanine. It was hypothesized that due to its unique chemical structure and bioactivity a water-soluble C60 fullerenes would have predominant influence on prooxidant-antioxidant homeostasis of rat muscle tissue. In our the previous work we detected the effect of the C60 fullerenes in the short-term period after intramuscular injection (Prylutskyy et al., 2017), in this study we determined the most optimal way of its application and investigated the effect of C60 fullerenes after preliminary administration, which implies its preventive use in case of muscular fatigue.
Materials and Methods
Material Preparation and Characterization
A highly stable C60FAS at a maximum concentration of 0.15 mg/ml was prepared as described earlier (Scharff et al., 2004; Ritter et al., 2015). Briefly, for the preparation of C60FAS we used a saturated solution of pristine C60 fullerene (purity >99.99%) in toluene with a C60 molecule concentration corresponding to maximum solubility near 2.9 mg/ml, and the same amount of distilled water in an open beaker. The two phases formed were treated in ultrasonic bath. The procedure was continued until the toluene had completely evaporated and the water phase became yellow colored. Filtration of the aqueous solution allowed to separate the product from undissolved C60 fullerenes.
DLS Measurements
Measurements of the hydrodynamic size distribution for C60 fullerenes in aqueous solution were performed by dynamic light scattering (DLS) on a Zetasizer Nano-ZS90 (Malvern, Worcestershire, United Kingdom) at room temperature. A DLS instrument equipped with a HeNe laser (max 5 mW) operating at a wavelength of 633 nm was used. The measurements were performed at a 90° scattering angle. The autocorrelation function of the scattered light intensity was analyzed with Static Light Scattering software.
Zeta Potential Measurements
Zeta potential measurements for C60FAS were carried out on a Zetasizer Nano-ZS90 (Malvern, Worcestershire, United Kingdom) at room temperature. The results were evaluated using the Smoluchowski approximation, which is known to be valid only for spherical-like particles.
Procedure and Experimental Groups
Experiments were performed on male Wistar rats weighing 280–350 g with ages ranging from 4 to 6 months. The animals were purchased from a state-controlled animal farm through the common animal facility of Bogomoletz Institute of Physiology (Kyiv). The experimental animals were housed in Plexiglas cages (four rats per cage) and kept in an air-filtered and temperature-controlled (20–22°C) room. The rats received a standard pellet diet and water ad libitum. The present study was approved by the Ethics Committee of the Institute and performed according to the European Communities Council Directive of November 24, 1986 (86/609/EEC).
All animals were randomly divided into 7 groups: 1st – fatigue-induced animals (n = 6); 2nd – vehicle-injected [fatigue-induced rats with a preliminary intraperitoneal (i.p.) injection of 0.3 ml of saline solution, n = 6]; 3rd – C60FAS-injected [F-injection; fatigue-induced rats with a preliminary i.p. injection of 0.3 ml (0.14 mg/kg) of C60FAS, n = 6]; 4th – animals with oral administration of C60FAS [F-drinking; fatigue-induced rats with a preliminary (within 5 days, 0.225 mg/kg per day) oral introduction of C60FAS, n = 6]; 5th – NAC-injected (fatigue-induced rats with a preliminary i.p. injection of 150 mg/kg of NAC dissolved in saline solution, n = 6); 6th – β-Alanine-injected (β-Al-injection; fatigue-induced rats with a preliminary i.p. injection of 110 mg/kg of β-Alanine dissolved in saline solution, n = 6); and 7th – intact animals (rats were used only for biochemical studies, n = 6).
It should be noted that the toxicity of the pristine C60 fullerenes is strictly dependent on their size (the degree of aggregation in the water). In our previous works (Prylutska et al., 2009, 2017; Tolkachov et al., 2016), we investigated in detail the in vitro toxicity of C60FAS. We can conclude that at a maximum concentration of 0.15 mg/ml, C60FAS (the diameter of nanoparticles is up to 50 nm) does not exhibit any genotoxicity and cytotoxicity to various types of cells, including human ones. It is important to note that the doses of C60FAS used in this study do not present any acute or subacute toxicity in animals: they were significantly lower than the maximum tolerated dose of pristine C60 fullerene, which was found to be 5 g/kg both for oral or i.p. administration to rats (Gharbi et al., 2005). Toxic or lethal effects were not observed in the studies of the impact of C60 fullerenes after oral administration to rats at a total dose of 2 g/kg for 14 days (Mori et al., 2006). Moreover, according to our previous data (Tolkachov et al., 2016) C60FAS at the concentrations up to 24 μg/ml does not manifest any in vitro toxic effect on human mesenchymal stem cells. C60FAS (0.1 mg/ml) does not induce DNA strand breaks in the human lymphocytes as revealed by the comet assay (Prylutska et al., 2017). Finally, the authors (Świdwińska-Gajewska and Czerczak, 2016) state that oral exposure of pristine C60 fullerene nanoparticles does not lead to major adverse effects because they were not mutagenic and genotoxic in experimental research. The pristine C60 fullerene is characterized by low toxicity and it does not pose a risk in the occupational environment.
Fatigue of the TS of rat was induced by electrical stimulation of n. tibialis. C60FAS, NAC, β-Alanine or saline solution were administered 1 h prior to electrical stimulation. After the experiment, the TS of all animals in all groups were removed for biochemical analysis.
The animals were anesthetized with ketamine (100 mg/kg “Pfizer”, United States) combined with xylazine (10 mg/kg, “Interchemie,” Holland), tracheostomized and artificially ventilated (out of necessity). The left and right TS muscles were separated from the surrounding tissue, their tendons were detached at the distal insertions, and a small bone chip from the heel was left behind. The n. tibialis was separated from the tissue and cut proximally, and all branches of the nerve, except nerves innervating the TS, were cut. This nerve was mounted on a bipolar platinum wire electrode for electrical stimulation. The hindlimb muscles and nerves were covered with paraffin oil in a pool formed by the skin flaps. The ECG and heart rate were continuously monitored. Pools with mineral oil were maintained at 37–38°C using radiant heat. The TS muscle was connected via the Achilles tendon to the servo-control muscle puller. A linear motor under servo-control was used as the muscle puller. The muscle tension was measured by semi-conductor strain gauge resistors glued on a stiff steel beam mounted on the moving part of the linear motor. The stiffness of the puller exceeded 0.06 N/mm, whereas the time constants of the length transients did not exceed 60 ms.
To induce muscle fatigue, a series (30 min duration) of intermittent high-frequency electrical stimulations was used (Figures 1A–D), separated by rest intervals of 15 min. Four stimulation series were used for the rats in group 1, and 5 stimulation series were used for the other groups of animals. Each series consisted of trains of 0.2 ms rectangular pulses at a rate of 40 s-1 at a 12.4 s duration, and the series were separated by 5 s intervals of rest (Figure 1D, in a circle). The stimulus current was set to 1.3–1.4 times higher than the motor threshold. At the end of the 12.4 s stimulation, the muscle was stretched, and the changes in length had a bell-shaped form (one period of 4 Hz sinusoidal signal with corresponding phase locking) with a 3.5 mm amplitude and 2 s duration (Figure 1D, bottom row in a circle). The muscle reaction to the stretching appeared to be a tension increase after continuous stimulation. These stretches were applied before the post-stimulation twitches to remove, or at least diminish, the after-effects remaining from continuous stimulation (Kostyukov et al., 2000). The command signal to the muscle puller was derived from a DAC and was adjusted by a scaling amplifier and low-pass filter (0–100 Hz). In parallel, two analog signals (muscle tension and length) and pulse signals (stimulation pulses) were sampled via corresponding ADC channels. The signals were collected by PC using an input-output interface device (CED Power 1401) with 12-bit resolution.
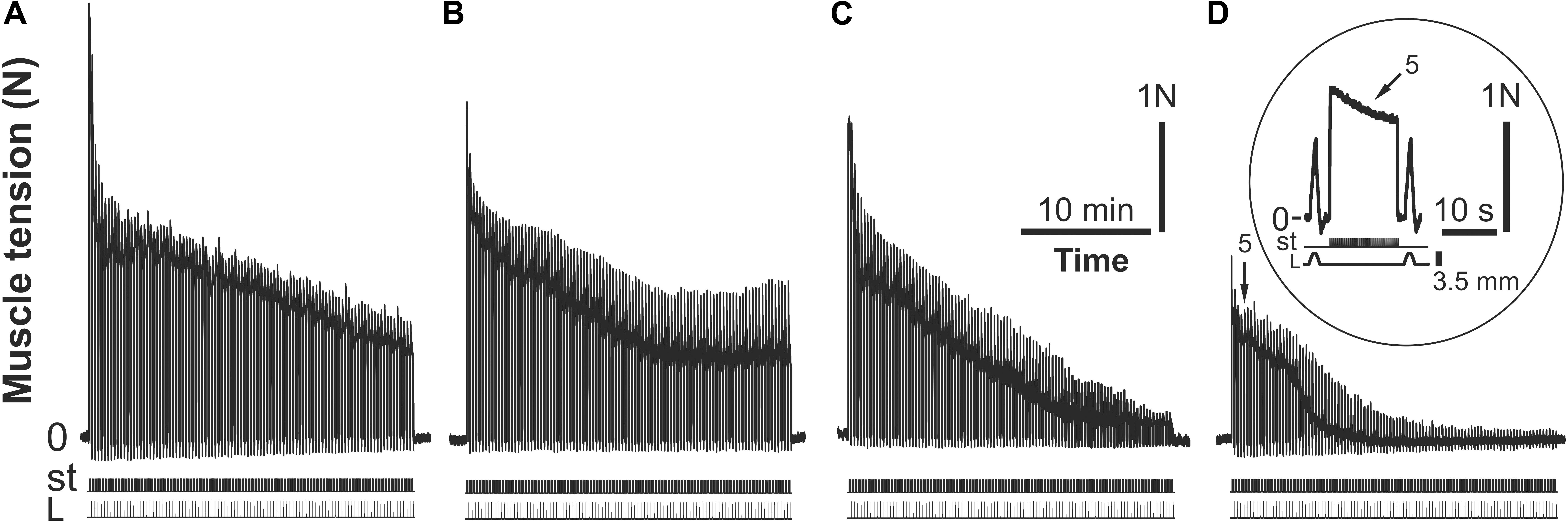
FIGURE 1. Strength of the m. triceps surae (TS) contractions during consecutive (I–IV) series of 30-min intermittent stimulations of the n. tibialis; the rat belongs to the fatigue-induced group of animals (group 1) (A–D). The 5th individual tetanic contraction of TS in the IV stimulation series is presented in a circle at a higher time scale on (D). P – muscle force (Newton, N), st – stimulation mark, L – muscle length (stretching, mm).
Data acquisition was performed using the program “Spike2” (CED). Input signals were digitized at rates of 5 kHz (muscle tension) and 1 kHz (other signals). Data analysis, including statistical treatment and graph plotting, was performed using the program Origin 8.0 (OriginLab Corp., United States).
Biochemical Experiment
After acute exercise, the excised muscles (soleus and gastrocnemius) were rapidly dissected, free of fat and tendons were removed, and the muscles were divided into several portions and stored in liquid N2. For GSH (reduced glutathione) analysis, the tissue samples were transferred to a medium containing 1N perchloric acid (1:10 w/v) and homogenized with a motor-driven Potter-Elvehjem glass homogenizer. Resultant homogenate was centrifuged at 10,000 g for 10 min (4°C). GSH content was measured spectrophotometrically (Sedlak and Lindsay, 1968). For the activities of enzymes, H2O2 and lipid peroxidation assays, the muscle samples were thawed and homogenized in 50 mM phosphate buffer with 2 mM EDTA (pH 7.4) at 4°C (1:9 w/v). The homogenates were then centrifuged then for 15 min at 15,000 g (4°C), and the post-mitochondrial supernatant was stored at -70°C.
Oxidative damage in tissue was measured using a TBARS assay. TBARS were isolated by boiling tissue homogenates for 15 min at 100°C with thiobarbituric acid reagent (0.5% 2-thiobarbituric acid/10% trichloroacetic acid/0.63 M/dm3 hydrochloric acid) and measuring the absorbance at 532 nm. The results were expressed as nM TBARS/mg protein using 𝜀 = 1.56 × 105 dm3/M/cm (Buege and Aust, 1978). The data on ROS formation were obtained from dichlorofluorescein (DCF) fluorescence. The tissue homogenates were loaded for 20 min at 37°C with non-fluorescent probe (2′,7′-dichlorodihydrofluorescein diacetate, DCFHDA) which is known to be decomposed in cells to give dichlorofluorescein upon oxidation by ROS, primarily hydroperoxide and superoxide anion. The final concentration of DCFH-DA was 10 μM. DCF formation was followed at the excitation wavelength of 488 nm and emission wavelength of 525 nm for 30 min by using a Hitachi F-2000 fluorescence spectrometer. The rate of DCFH-DA conversion to DCF was linear for at least 60 min, corrected with the autoxidation rate of DCFH-DA without protein. All assays were carried out in duplicates. Fluorescence was expressed as arbitrary fluorescence units.
H2O2 concentration in the tissue homogenates was measured using the FOX method, which is based on the peroxide-mediated oxidation of Fe2+, followed by a reaction of Fe3+ with xylenol orange (o-cresolsulfonephthalein 3′,3′′bis[methylimino] diacetic acid, sodium salt). This method is extremely sensitive and used to measure low levels of water-soluble hydroperoxide present in the aqueous phase. To determine the H2O2 concentration, 500 μL of the incubation medium was added to 500 μL of assay reagent (500 μm ammonium ferrous sulfate, 50 mm H2SO4, 200 μm xylenol orange, and 200 mm sorbitol). The absorbance of the Fe3+-xylenol orange complex (A560) was detected after 45 min. Standard curves of H2O2 were obtained for each independent experiment by adding variable amounts of H2O2 to 500 μL of basal medium mixed with 500 μL of assay reagent. The data were normalized and expressed as μm H2O2 per mg protein (Wolff, 1994).
Catalase activity was measured by decomposition of H2O2, which was determined by a decrease in absorbance at 240 nm (Aebi, 1983).
Reduced glutathione was determined using Ellman’s reagent. One milliliter of supernatant was treated with 0.5 ml of Ellman’s reagent (5.5′-dithio-bis-nitrobenzoic acid in abs. ethanol) and of 0.4 M Tris HCl buffer with 2 mM EDTA, pH 8.9. The absorbance was read at 412 nm in a spectrophotometer (Sedlak and Lindsay, 1968).
Manganese-superoxide dismutase (Mn-SOD) activity was estimated by the method of Misra and Fridovich (1972), which is based on the inhibition of autoxidation of adrenaline to adrenochrome by the SOD contained in the examined samples. The mitochondrial samples were preincubated at 0°C for 60 min with 6 mM KCN, which produces total inhibition of Cu, Zn-SOD activity. The results were expressed as the specific activity of the enzyme in units per mg protein. One unit of SOD activity was defined as the amount of protein causing 50% inhibition of the conversion rate of adrenaline to adrenochrome under specified conditions.
The activity of selenium-dependent GPx was determined according to the method of Flohé and Günzler (1984). Briefly, the reaction mixtures consisted of 50 mM KPO4 (pH 7.0), 1 mM EDTA, 1 mM NaN3, 0.2 mM NADPH, 1 mM GSH, 0.25 mM H2O2, and 226 U/ml glutathione reductase, and the rates of NADPH oxidation followed at 340 nm.
Lactic acid was determined in muscle tissue after deproteinization with 6% (wt/wt) perchloric acid by sitting the tissue on ice for 15 min and then centrifuging the tissue at 14,000 g for 5 min. The supernatant was neutralized with 5 M K2CO3, clarified again to remove potassium perchlorate, and stored at -70°C. The assay mixtures contained glycine/EDTA/hydrazine hydrate buffer (pH 9.5), 0.05 mM NAD, 10 units of lactate dehydrogenase, and sample (100 μl), and the mixtures were incubated at 37°C for 20 min. The LA concentration was determined spectrophotometrically at 340 nm in a 1.0-ml total reaction volume (Hohorst, 1970).
Protein concentration was estimated with the Bradford method using bovine serum albumin as a standard. All chemicals were purchased from Sigma, Fluka and Merck and had the highest available purity.
Statistical Analysis
In the electrophysiological study, each stimulation series was averaged (100 stimulations in one series). The average value of the first series was set to 100%, and the other series were normalized in relation to this and presented graphically for one of hindlimb. During experiments, it was found that till the moment of a drastic decrease of TS contraction force control animals maintain a certain force level of muscular contraction only during three series of electrical stimulation. To confirm the muscle fatigue factor, data from four stimulation series were taken for the study. Some animals from groups 3–6 to maintain a certain force level of muscular contraction until 5–6 series of electrical stimulation. Therefore, for further analysis, data from the first 5 series were taken.
Mean values (mean ± SD) of the TS muscle strength after C60FAS, NAC, β-Alanine, saline solution induction or without any induction were compared using two-way statistical analysis of variance (ANOVA). The factors of variation included two conditions: time and the effects of the C60FAS (NAC, β-Alanine or non-injected). A Bonferroni post hoc analysis was used to determine the differences between groups. The level of significance was set at P < 0.05.
Biochemical data are expressed as the means ± SEM for each group. The differences among experimental groups were detected by one-way analysis of variance (ANOVA) followed by Bonferroni’s multiple comparison test. Values of P < 0.05 were considered significant.
Results
It has been established that the size of water-soluble C60 fullerene particles directly correlates with their cytotoxicity and biological properties (Lyon et al., 2006; Prylutska et al., 2009; Song et al., 2011; Lalwani and Sitharaman, 2013; Zhang et al., 2015). Depending on the size, water-soluble C60 fullerene particles can penetrate through the plasma membrane into the cell or be adsorbed on the surface of the membrane (Foley et al., 2002; Schuetze et al., 2011; Franskevych et al., 2017). In this regard, the main advantage of using pristine C60 fullerenes as powerful antioxidants (Gharbi et al., 2005; Prylutska et al., 2008) is their ability to be localized preferentially to mitochondria, which generates a substantial amount of cellular ROS (Foley et al., 2002; Youle and Karbowski, 2005). Thus, since the size of C60 fullerene particles and stability of their aqueous solution (the degree of aggregation in water) may influence their bioactivity, the DLS and zeta potential studies of C60FAS were performed.
DLS and Zeta Potential Studies
The DLS results for investigated C60FAS clearly demonstrate that there was a monomodal nanoparticle size distribution in a (15–30) nm range, i.e., these nanoparticles in particular have specific bioactivity. This result is similar to our previous probe microscopic data, which directly correlate with C60 fullerene bioactivities (Prylutska et al., 2014; Skamrova et al., 2014).
The magnitude of the zeta potential is related to the stability of colloid dispersions because it determines the degree and nature of the interaction between the particles of the dispersal system. The value of zeta potential for C60FAS was equal to -23 mV, which agrees well with our previously published data (Ritter et al., 2015). A high negative charge for colloid nanoclusters (or, more strictly, the electrostatic repulsion between the negatively charged nanoclusters) seems to play a significant role in the stabilization of C60FAS (i.e., it disfavors the aggregation and makes the solution electrically stable).
Electrophysiological Experiments
As a result of intermittent high-frequency electrical stimulation (30 min duration, 40 Hz) of TS muscle in the rats of the first and second groups during four series, the reduction of the force contraction was recorded until the muscle ceased to demonstrate clear contractions. Figure 1 shows an example of the TS force response changes in one animal of the first group during fatigue development. In this case, the dynamics of changes in muscle force reflected fatigue development and a drop in amplitude in single contractions. In the first series of stimulations, a gradual reduction of TS activity level was observed over 30 min. In the second series of stimulations, which were started after a 15 min rest, the amplitudes of tetanic contractions were somewhat recovered; however, they did not reach the initial level, continuing to decrease. Within the third series of stimulations (after 90 min of electrical stimulation), an abrupt drop in the muscle force was observed. Within this time interval, the maximum significant strength level decrease (P < 0.05) was observed with respect to the first series of stimulations (Figure 2). After another interruption between stimulations, the isometric muscle force contraction continued to decline without further recovery. At the same time, such a decrease in muscle force response was statistically significant (P < 0.05) within the IV series of stimulations with respect to that one in the I, II, and III series (Figure 2). Note that statistically significant differences (P > 0.05) in the strength of muscle contractions between rats in groups 1 and 2 were not found.
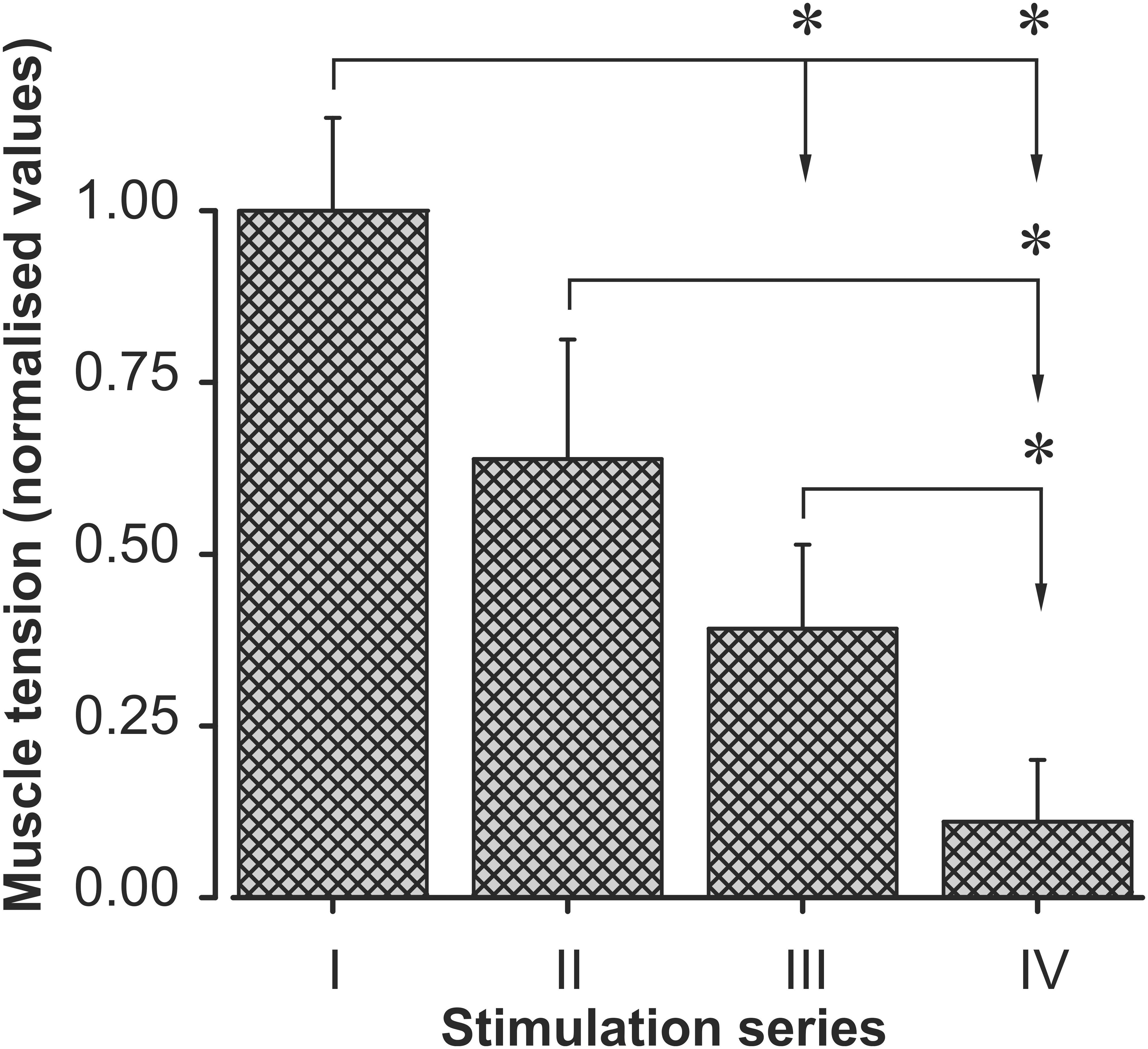
FIGURE 2. Changes in the TS muscle strength in the I–IV series of fatigue stimulation. There are normalized averaged force (mean ± SD) (normalization with respect to the first series) values. The averaging procedure has been applied to the data registered in six animals of the fatigue-induced group of rats (group 1), and the data were averaged with respect to the corresponding stimulation series. Asterisks denote significant differences (P < 0.05) between the muscle strength of different electrical stimulation series.
For assessments of the effectiveness of administration method of the C60FAS under conditions of fatigue stimulation it was made a comparison of the changes in the force levels developed by muscles in animals with C60 fullerene i.p. injection (F-injection) and in animals that drank C60 fullerene for 5 days (F-drinking) (Figure 3). The analysis did not reveal any significant differences in the muscle strength after studying the effects of these substance administration methods on muscle fatigue (F-injection vs. F-drinking). In this case, under conditions of both F-injection and F-drinking, the muscle maintained the same constant force level during the first series of fatigue stimulation (30 min). In animals with a C60 fullerene i.p. injection during the third series of stimulations, the force level was slightly higher compared to the group of animals that drank C60FAS. Under conditions of further fatigue stimulation (V series) with F-injection and F-drinking, the difference in the level of the developed effort decreased (Figure 3). Thus, the absence of statistically significant differences (P > 0.05) made it possible for us to conduct further analyses of fatigue development using only F-drinking administration, since it is non-invasive and potentially more practical for future application.
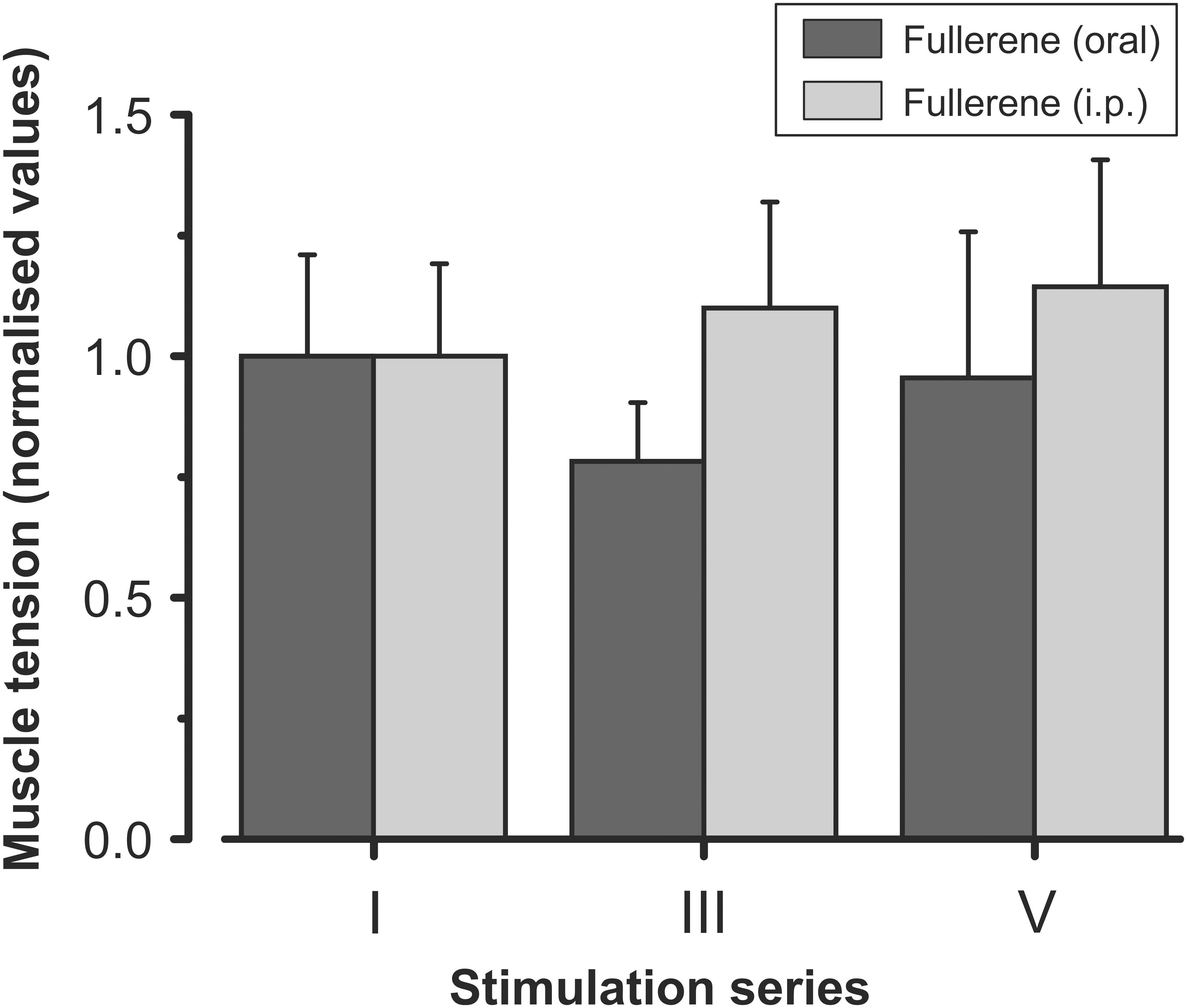
FIGURE 3. Averaged characteristics (mean ± SD) of the normalized (with respect to the first stimulation series) values of the muscle strength after preliminary intraperitoneal injection and oral administration of C60FAS (dark gray and light gray bars, respectively). I, III, and V – series of electrical fatiguing stimulation. Values of six animals in each group were averaged according to stimulation series. Note the absence of significant differences between data obtained in rats of groups 3 and 4.
Further analysis suggested the force fatigue contractions on the background of a separate action of C60FAS attach be compared to exogenous antioxidants NAC and β-Alanine (Figure 4). The data obtained in these experiments (Figures 4B,F) indicate that a reduction in the developed force in the animals of the F-drinking, the NAC-injected and the β-Al-injected group was slower compared to fatigue-induced or vehicle-injected animals. Significant differences (P < 0.05) in muscle strength changes between control animals (groups 1 and 2) and experimental rats (groups 4–6) appeared after the second stimulation series. In groups 4–6, the muscle maintained a constant level of developed effort through the whole fatigue stimulation, which was demonstrated using native records of its force characteristics (Figures 4A,C,E). The animals of the F-drinking group held a stationary level of force longer than the NAC-injected and β-Al-injected animals. The dynamics of the force changes was similar in almost all animals of this group (Figure 4B). In the NAC-injected animals during the III series, there was decrease in the muscle strength amplitude along with further stabilization, but these effects were not significant (Figure 4C). It should be noted that in two animals of this experimental group, recovery of the muscle contraction force level to the force level at the initial fatigue stimulation stages occurred (Figures 4C,D). At the same time, in the F-drink group during the V series of fatigue stimulation, muscle strength recovery was also observed, and in some animals, its increase was recorded (Figure 4B). In the β-Al-injected group, the developed force was maintained at a steady-state level within each series of stimulations (Figure 4E). At the end of the I series of stimulation, there was a slight decrease in muscle force with some recovery in the III series of stimulations. However, during further stimulation (V series), the level of the muscle force was slightly lower compared to previous series (Figure 4E). In this case, in all animals of this experimental group a similar change in muscle force was observed (Figure 4F).
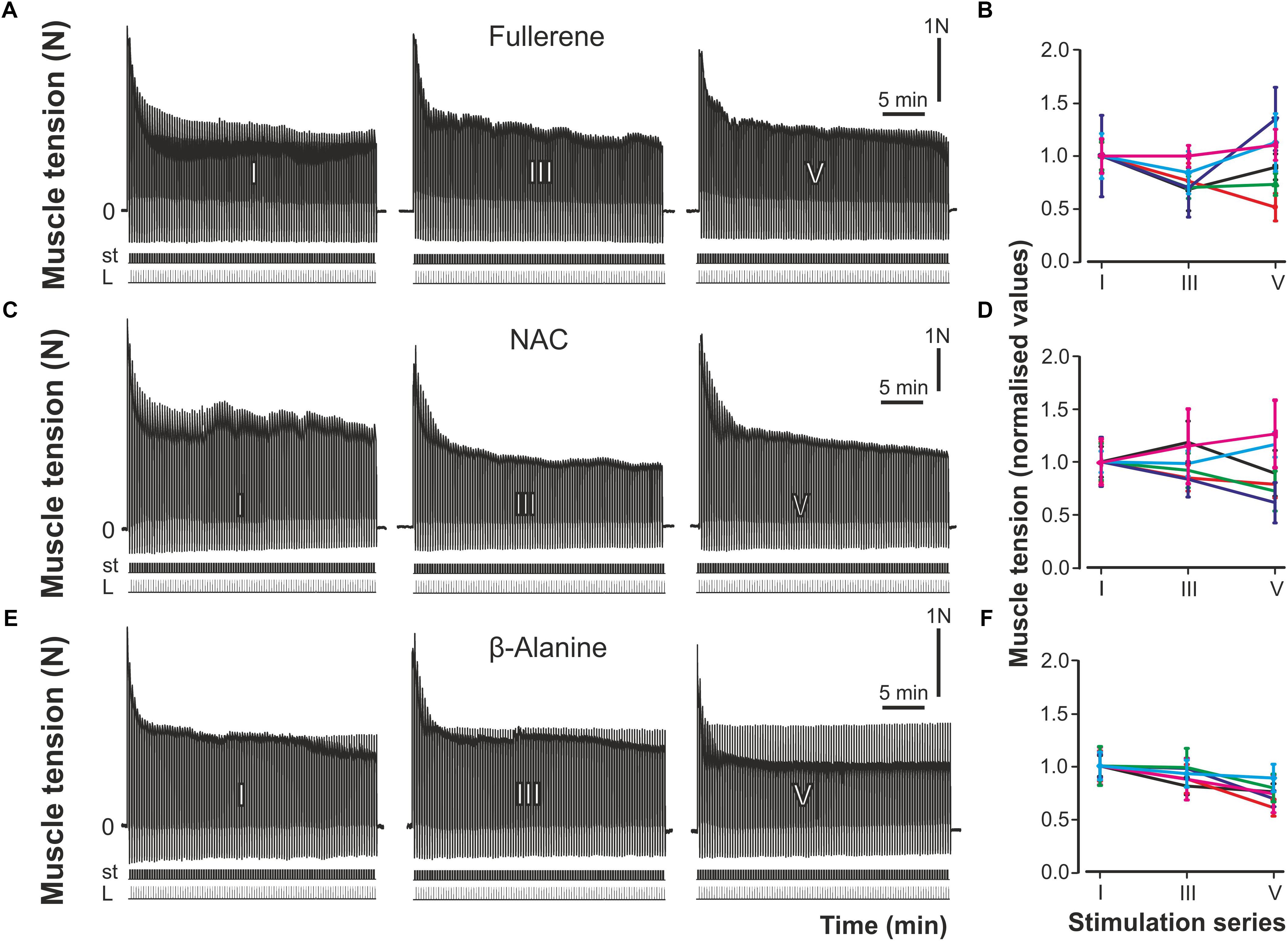
FIGURE 4. Strength of the muscle contractions after preliminary oral administration of C60FAS and injection of NAC and β-Alanine. (A,C,E) Protocol of registration of the muscle contractions after preliminary C60FAS oral administration, NAC- and β-Al-injection, respectively; (B,D,F) – results of six fatigue tests in the groups of animals with preliminary C60FAS oral administration, NAC- and β-Al-injection, respectively. I, III, and V – series of electrical fatigue stimulations, P – muscle force (Newton, N), st – stimulation mark, L – muscle length (stretching).
To compare the action of the C60FAS and antioxidants on the force of contractions during fatigue development, a statistical analysis was performed (Figure 5). In NAC-injected animals, during the initial series of stimulation, there was maintenance of the force at a constant level followed by some decrease. The characteristics of fatiguing muscle contractions were somewhat different in the F-drinking group. In these experiments, the level of muscle force, after some initial reduction in the I series of stimulations, was further recovered and almost reached the control values. In the β-Al-injected group, a gradual decrease of the muscle force was observed. However, it should be noted that there was no significant difference in the muscle force for series conducted within the same animal group, as well as between groups (NAC-injected, F-drink, β-Al-injected) (Figure 5).
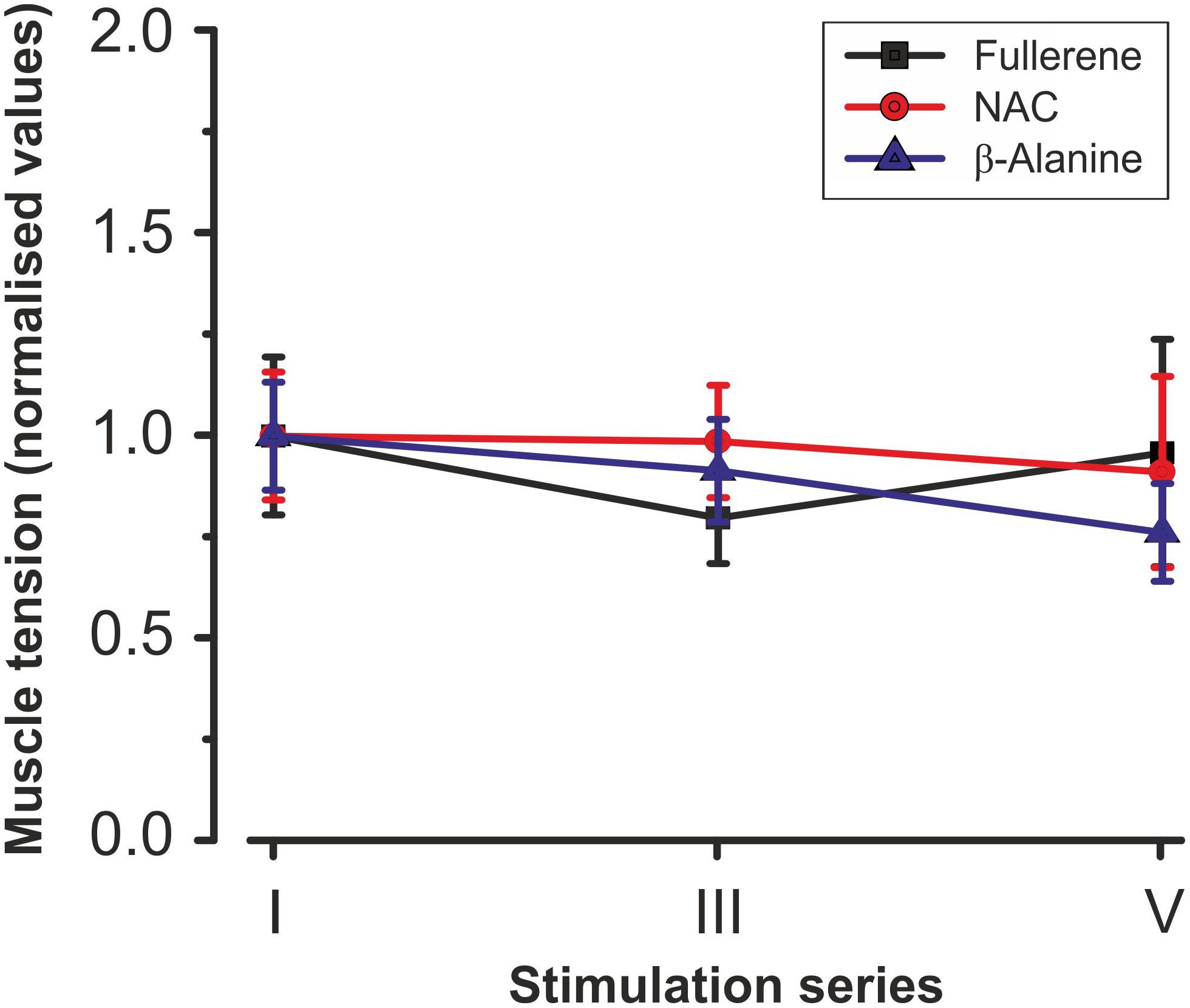
FIGURE 5. Averaged characteristics (mean ± SD) of normalized (with respect to the average values in the first stimulation series) values of the muscle strength after preliminary C60FAS oral administration, NAC- and β-Al-injection. Values of six animals in each group were averaged by stimulation series. Note the absence of significant differences between the data obtained in the rats of these groups. I, III, and V – a series of electrical fatigue stimulations.
Biochemical Experiments
After the fatiguing tests, accumulation of secondary products and changes in antioxidant levels in the muscle tissues were determined (Figure 6). The obtained data clearly demonstrate the increased level of metabolic product (LA), markers of peroxidative and oxidative stress (TBARS, H2O2) and ROS formation after stimulation that indicates the occurrence of muscle fatigue (Figure 6). This increase was statistically significant with respect to the intact muscles (“norm”) and consisted of 61% for ROS formation, 78% for TBARS, 115% for H2O2, and 198% (P < 0.05) for LA. In turn, in response to these changes in the working muscle, there was a significant (P < 0.05) increase in endogenous antioxidant activity, including GSH (71%), CAT (18%), GPx (28.5%), and SOD (34%).
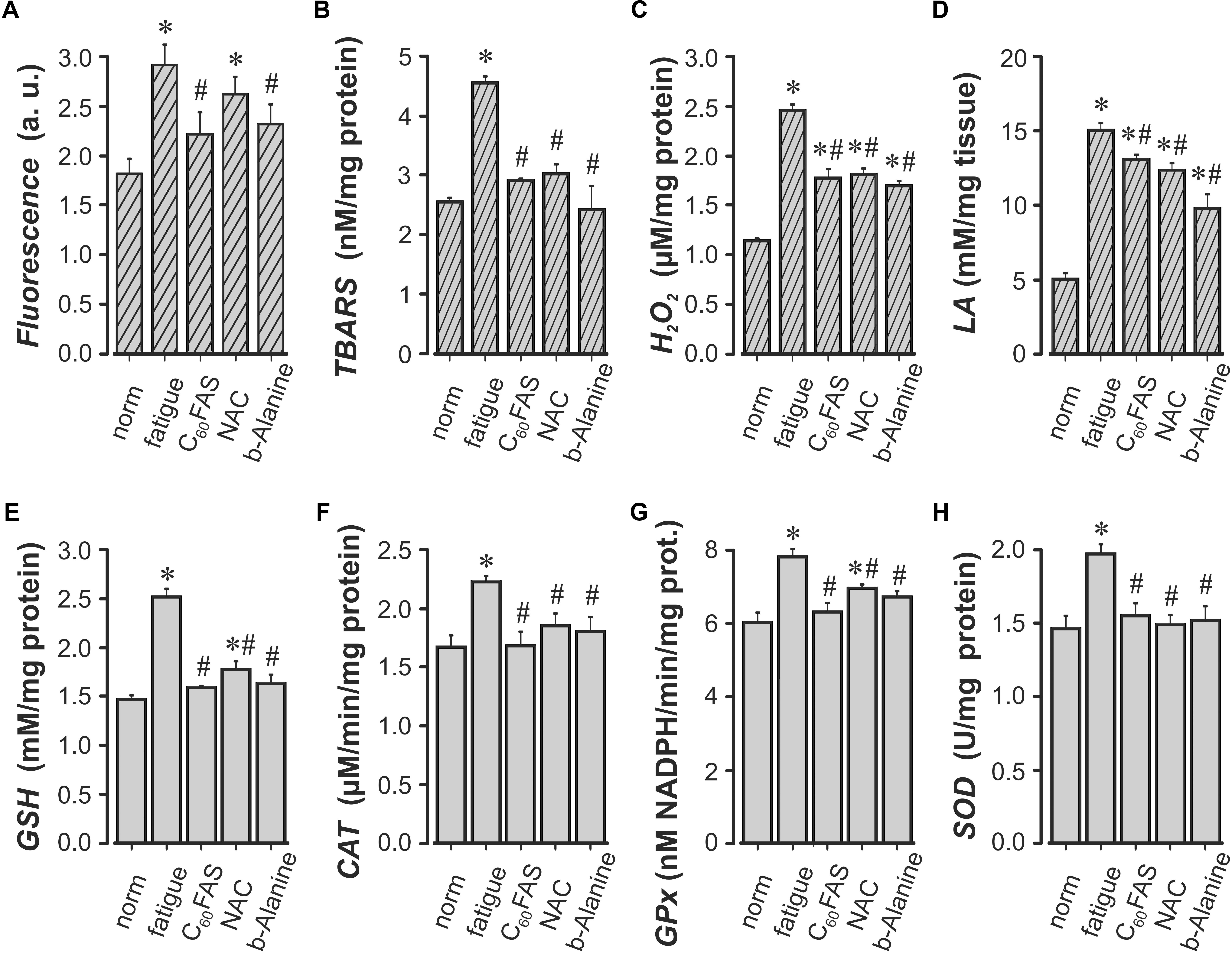
FIGURE 6. Indicators of the prooxidant-antioxidant balance in the m. triceps surae (TS) of rats. ROS formation (A), the concentration of thiobarbituric acid reactive substances (TBARS) (B), hydrogen peroxide (H2O2) (C), lactic acid (LA) (D), reduced glutathione (GSH) (E), catalase (CAT) (F), glutathione peroxidase (GPx) (G) and superoxide dismutase (SOD) (H) are in intact animal muscles (norm), TS fatigue-induced animals (fatigue) and TS fatigue-induced animals after C60FAS oral administration (C60FAS), NAC-injection (NAC) and β-Al-injection (β-Alanine), respectively. Values are the means ± SEM, n = 6. ∗P < 0.05 vs. “norm”; #P < 0.05 vs. “fatigue.”
In animals that drank C60FAS, ROS formation and concentrations of TBARS, H2O2 and LA were significantly lower at 24.1, 36.1, 27.8, and 13.1% (P < 0.05), respectively, compared to the “fatigue” group. Similar changes in the level of these marker concentrations were also observed in the NAC-injection and β-Al-injection groups. A similar dynamic has been revealed in changes in the activity of antioxidant enzymes. Under conditions of fatigue development, the level of GSH, CAT, GPx, and SOD activity significantly increased by 71.4, 18.6, 28.5, and 34.2% relatively to the “norm,” respectively. The animal groups F-drinking, NAC-injection and β-Al-injection showed a decrease in endogenous antioxidants activity relative to the “fatigue” group (P < 0.05). Furthermore, in rats that drank C60FAS, the activity levels of GSH, CAT, and GPx were smaller.
Discussion
In this study, we compared the effects of an aqueous colloid solution of pristine C60 fullerene with the action of the NAC and β-Alanine on exercise tolerance and contractile properties of rat TS during development muscle fatigue. This experimental approach allowed us to analyze and compare the characteristics of the force parameter changes of muscle contraction under conditions with the same fatigue stimulation pattern applied after oral introduction of C60FAS or separate i.p. injections of C60FAS, NAC and β-Alanine. The results showed a statistically significant reduction in muscle force in all animals without prior administration of C60FAS, NAC, and β-Alanine (Figure 2). To maintain the normal functional and physiological state of the muscle during the dynamic work performance, the duration of its recovery and active rest are very important factors (Harris and Sale, 2012). An insignificant decrease in the muscle contraction force compared to the control occurred in animals that drank C60FAS and in both NAC- or β-Al-injected rats (Figure 5). This outcome supported our previous finding that fullerene can lower the effects of fatigue development and promote force maintenance at a constant level (Prylutskyy et al., 2017). At the same time, in most animals of the F-drinking group, there was a recovery of the force level after some decrease, whereas in the NAC- and β-Al-injected groups, only an insignificant decline occurred (Figures 4B,D,F). A definite recovery of the contractile ability was noticed in only two animals from the NAC-injected group (Figure 4D). These data on the effects of C60FAS as a potent antioxidant under fatigue development conditions are in accordance with the data that were obtained earlier (Prylutskyy et al., 2017). In this case, one can speak about the predominant influence of C60 fullerene on muscle strength characteristics during fatigue development compared to the action of other investigated exogenous antioxidants, such as NAC or β-Alanine.
In our study, we showed that fatigue stimulation in the working muscle led to an increase in the metabolic products (LA) and the intensification of the oxidative processes, a namely a significant increase in ROS formation and lipid peroxidation, which occurs simultaneously with an increase in CAT and GSH activity in both fast and slow twitch muscles fibers relative to the intact muscle (Figure 6). The increased LA level further reduced the pH, which could induce various biochemical and physiological effects during muscular contractions, including glycolysis, phosphofructokinase, and calcium release (Wang et al., 2012). Therefore, LA is an important marker for evaluate the degree of fatigue of a living organism. In the group of animals that drank C60FAS, attenuation of oxidative stress was observed (i.e., a decrease in ROS generation and TBARS concentration). This outcome was confirmed by the data obtained earlier on the effects of C60 fullerenes for the prooxidant–antioxidant homeostasis of rat muscle tissue (Prylutskyy et al., 2017). Similar changes in the number of metabolites and peroxide oxidation markers were observed in the NAC-injected and β-Alanine-injected groups. It has been supported that β-Alanine supplementation increases carnosine levels and decreases lactate responses after high-intensity exercise in rat muscles (Culbertson et al., 2010). In skeletal muscles, carnosine acts as a pH buffer and functions as an antioxidant (Boldyrev et al., 1993), which suggests that there is a potential role for carnosine for reversing or limiting the effects of oxidative stress and cellular senescence. Therefore, it cannot be excluded that the β-Alanine intake results in decrease of lactate and H+-ion production. The skeletal muscles from NAC-injected rats showed a low content of TBARS, suggesting a reduced muscle fiber disruption due to cell membrane lipid peroxidation as compared with non-injected rats. This protective effect of NAC is due to direct scavenging of ROS and GSH synthesis enhancement (Aruoma et al., 1989; Supinski et al., 1997; Sen and Packer, 2000).
The important role of GSH in protecting against exercise-induced oxidative stress has been demonstrated in several studies (Sen et al., 1994; Leeuwenburgh et al., 1997). The increased amount of GSH in the stimulated muscle (without the C60FAS, NAC, or β-Alanine administration and after their application) indicates compensatory activation of the endogenous GSH antioxidant system on the action of the stimulus (Figure 6E). In some studies, it has been shown that during intense loads, there is a significant decrease in GSH content in m. soleus and an increase in GSH content in m. deep vastus lateralis (DVL), but did not alter GSH status in the liver or plasma (Leeuwenburgh et al., 1997). Although other studies indicate an increase in the concentrations of GSH and GSSG in m. soleus, less in DVL and m. superficial vastus lateralis (SVL). However, the GSSG/GSH ratio does not change significantly because GSSG can be reduced to GSH by glutathione reductase. Furthermore, exercising skeletal muscles appear to increase GSH import from plasma (Ji et al., 1992) as well as the synthesis of GSH in the liver from endo- or exogenous amino acids, which adds most of the circulating GSH (Meister and Anderson, 1983), that ensures plasma GSH homeostasis despite enhanced tissue GSH use. Our experiments showed an increase in GSH activity during fatigue and a decrease in GSH under the actions of C60FAS, NAC, or β-Alanine. This outcome is consistent with the data of other authors who have shown that the use of NAC, in particular, decreases exercise-induced GSSG, and blood lipid peroxidation in rats (Sen et al., 1994) improves muscle contractile functions as well as reduces low frequency fatigue in the diaphragm muscle (Shindoh et al., 1990) and human leg muscles (Reid et al., 1994).
An increase in the level of H2O2 activity during muscle contractions led to an increase in the CAT enzyme concentration (Figure 6F). These data also support studies performed earlier in rats (Ji and Fu, 1992; Hollander et al., 1999). It was shown that CAT activities were significantly elevated after exhaustive exercise with or without hydroperoxide injection in muscle and not in liver (Ji and Fu, 1992). Previously, it was reported that after the acute stage of the exercise, CAT was significantly higher in m. soleus than in DVL and SVL. Furthermore, the exercise at moderate intensities elicited significant increases in CAT activity in DVL (Ji et al., 1992). However, some studies have reported no changes in muscle CAT with training (Powers et al., 1994a; Radák et al., 1995), and a few studies even reported a decrease in CAT activities in m. soleus of the adult and old rats (Leeuwenburgh et al., 1994). In our study, during fatigue under NAC or β-Alanine treatment, the effects of CAT activity decreased with respect to “fatigue,” but exceeded the control values. However, in animals that drank C60FAS, CAT activity remained at the control level, which indicated that there was a greater compensatory effect of C60 fullerenes.
An increase in the concentration of oxidative process markers led to an increase in the activity of GPX in the working muscle (Figure 6G). However, with using of NAC and β-Alanine, the activity of this enzyme decreased relatively to “fatigue,” and in the group of F-drinking, it was practically restored to normal. Data about GPX activity remain controversial. Powers et al. (1994a) showed an increase in GPX activity in red m. gastrocnemius after endurance training in rats, whereas m. soleus and white m. gastrocnemius revealed no training effect. The magnitude of the GPX increase was directly related to exercise duration but independent of intensity. Thus, the GPX activity has demonstrated variable responses to acute exercise for various types of skeletal muscles. For example, GPX activity increased the next day after running on the treadmill until exhaustion in the m. soleus of rats, but not in m. tibialis (Radák et al., 1995). In our study, the activity level of GPX under fatigue conditions in the F-drinking group may indicate that C60FAS, by affecting endogenous antioxidants, prevents fatigue in an actively contracting muscle better than NAC and β-Alanine.
It has been proved that C60 fullerenes normalize cellular metabolism and nervous processes by increasing resistance to stress, increase the activity of enzymes and regenerative capacity of tissues, and also exhibit pronounced anti-inflammatory and antiallergenic effects (Cataldo and Da Ros, 2008) and effectively regulate the ATPase activity of actomyosin (Andreichenko et al., 2013). It has been experimentally found that C60 fullerenes and their derivatives may be auxiliary agents in complex therapy due to their ability to intensify the protective functions of the immune and antioxidant systems of the body (Ashcroft et al., 2006; Didenko et al., 2013; Halenova et al., 2016). In this regard, the above electrophysiological and biochemical results suggest a real perspective for the use of water-soluble pristine C60 fullerenes as potential agents for improving the efficiency of human skeletal muscle functioning by modifying ROS-dependent mechanisms that play an important role in the development of muscle fatigue.
It was shown that under intense loads in skeletal muscles, the activity of SOD increases (Ji and Fu, 1992; Ji et al., 1992; Lawler et al., 1993). We investigated the isoenzyme located in the mitochondria and Mn2- contained in the active center (Weisiger and Fridovich, 1973). Under the conditions of the fatiguing tests, the activity of Mn-SOD increased, and after application of the test substances, it decreased approximately to same level, insignificantly exceeding the control value. These results were confirmed by the data of Radák et al. (1995), where it was shown that in rats after the exhausted treadmill run, the immunoreactive content and activity of both isoenzymes of SOD (Mn-SOD and Cu, Zn-SOD are found in the cytoplasm, in erythrocytes and liver (McCord and Fridovich, 1969) increased in the m. soleus and m. tibialis immediately after the run. In animals that had been previously injected by the antioxidant, attenuation of oxidative stress was observed (i.e., a decrease in TBARS concentration). An increase in Mn-SOD was noted even in 1 day after the load in hepatic tissue (Radák et al., 1996). Additionally, it was shown that during intense swimming training, myocardial and diaphragmatic SOD were induced in rats (Powers et al., 1993, 1994b). Ohishi et al. (1997) showed that an adequate physical endurance load increases both the activity and the content of Mn-SOD and that untrained rats are very sensitive to oxidative stress during exercise. Mn-SOD is a reliable indicator of physical condition. It is concluded that the muscles can react to the load in such way to reduce the damage that results from the accumulation of free oxygen radicals due to increased metabolic activity.
Conclusion
It should be noted that there are studies in humans and animals demonstrating both various exogenous antioxidants and submaximal long-term training. The training increases the activity of endogenous antioxidants (Powers et al., 1993, 1994a) and the antioxidant defense system of glutathione (Banerjee et al., 2003) in skeletal muscles, which reduces the risk of cell damage, improving performance, and slowing down the muscle fatigue. Recent studies show that the addition of some antioxidant nutrients is necessary for physically active people (Sen, 1995; Powers et al., 1999; Banerjee et al., 2003).
The results obtained in this study confirm previous data on the mechanism of action of C60FAS intramuscular injections (Prylutskyy et al., 2017). A comparative analysis was produced using a non-invasive method for C60FAS administration, demonstrating a similarity of action of C60FAS with effects of already known exogenous antioxidants NAC and β-Alanine. But due to its membranotropic activity and the lack of acute in vivo toxicity (at least at low physiological concentrations), C60 fullerene has a greater effective influence on the homeostasis of muscle tissue in rats, which maintains the normal physiological state of the muscle and increases the duration of its active work.
Author Contributions
IV, NB, and AM designed and performed the experiments, and the in vitro assays were performed by OG. UR and YP were responsible for C60FAS creation and characterization. WM and TT helped with preparation of the manuscript and provided funding support. DN and IM helped to collect and analyzed the data. YP and AK provided supervision and guidance throughout this study. All authors revised the manuscript for important intellectual content and approved the final version of the article.
Conflict of Interest Statement
The authors declare that the research was conducted in the absence of any commercial or financial relationships that could be construed as a potential conflict of interest.
Abbreviations
C60FAS, pristine C60 fullerene aqueous colloid solution; CAT, catalase; GPx, glutathione peroxidase; GSH, reduced glutathione; H2O2, hydrogen peroxide; NAC, antioxidants N-acetylcysteine; SOD, superoxide dismutase; TBARS, thiobarbituric acid reactive substances; TS, m. triceps surae.
References
Aebi, H. (1983). “Catalase,” in Methods of Enzymatic Analysis, ed. H. U. Bergmeyer (New York, NY: Academic Press), 276–286.
Allen, D. G., Lamb, G. D., and Westerblad, H. (2008). Skeletal muscle fatigue: cellular mechanisms. Physiol. Rev. 88, 287–332. doi: 10.1152/physrev.00015.2007
Andreichenko, K. S., Prylutska, S. V., Medynska, K. O., Bogutska, K. I., Nurishchenko, N. E., Prylutskyy, Y. I., et al. (2013). Effect of fullerene C60 on ATPase activity and superprecipitation of skeletal muscle actomyosin. Ukr. Biochem. J. 85, 20–26. doi: 10.15407/ubj85.02.020
Anilkumar, P., Lu, F., Cao, L., Luo, P. G., Liu, J. H., Sahu, S., et al. (2011). Fullerenes for applications in biology and medicine. Curr. Med. Chem. 18, 2045–2059. doi: 10.2174/092986711795656225
Aruoma, O. I., Halliwell, B., Hoey, B. M., and Butler, J. (1989). The antioxidant action of N-acetylcysteine: its reaction with hydrogen peroxide, hydroxyl radical, superoxide, and hypochlorous acid. Free Radic. Biol. Med. 6, 593–597. doi: 10.1016/0891-5849(89)90066-X
Ashcroft, J. M., Tsyboulski, D. A., Hartman, K. B., Zakharian, T. Y., Marks, J. W., Weisman, R. B., et al. (2006). Fullerene (C60) immunoconjugates: interaction of water-soluble C60 derivatives with the murine anti-gp240 melanoma antibody. Chem. Commun. 28, 3004–3006. doi: 10.1039/b601717g
Bakry, R., Vallant, R. M., Najam-ul-Haq, M., Rainer, M., Szabo, Z., Huck, C. W., et al. (2007). Medicinal applications of fullerenes. Int. J. Nanomed. 2, 639–649.
Banerjee, A. K., Mandal, A., Chanda, D., and Chakraborti, S. (2003). Oxidant, antioxidant and physical exercise. Mol. Cell. Biochem. 253, 307–312. doi: 10.1023/A:1026032404
Boldyrev, A. A., Koldobski, A., Kurella, E., Maltseva, V., and Stvolinski, S. (1993). Natural histidine-containing dipeptide carnosine as a potent hydrophilic antioxidant with membrane stabilizing function. Mol. Chem. Neuropathol. 19, 185–192. doi: 10.1007/BF03160178
Bosi, S., Da Ros, T., Spalluto, G., and Prato, M. (2003). Fullerene derivatives: an attractive tool for biological applications. Eur. J. Med. Chem. 38, 913–923. doi: 10.1016/j.ejmech.2003.09.005
Brady, P. S., Brady, L. J., and Ullrey, D. E. (1979). Selenium, vitamin E and the response to swimming stress in the rat. J. Nutr. 109, 1103–1109. doi: 10.1093/jn/109.6.1103
Buege, J. A., and Aust, S. D. (1978). Microsomal lipid peroxidation. Methods Enzymol. 52, 302–310. doi: 10.1016/S0076-6879(78)52032-6
Castro, E., Hernandez Garcia, A., Zavala, G., and Echegoyen, L. (2017). Fullerenes in biology and medicine. J. Mater. Chem. B 5, 6523–6535. doi: 10.1039/C7TB00855D
Cataldo, F., and Da Ros, T. (2008). Medicinal Chemistry and Pharmacological Potential of Fullerenes and Carbon Nanotubes. Berlin: Springer. doi: 10.1007/978-1-4020-6845-4
Culbertson, J. Y., Kreider, R. B., Greenwood, M., and Cook, M. (2010). Effects of beta-alanine on muscle carnosine and exercise performance: a review of the literature. Nutrients 2, 75–98. doi: 10.3390/nu2010075
Dellinger, A., Zhou, Z., Connor, J., Madhankumar, A. B., Pamujula, S., Sayes, C. M., et al. (2013). Application of fullerenes in nanomedicine: an update. Nanomedicine 8, 1191–1208. doi: 10.2217/nnm.13.99
Didenko, G., Prylutska, S., Kichmarenko, Y., Potebnya, G., Prylutskyy, Y., Slobodyanik, N., et al. (2013). Evaluation of the antitumor immune response to C60 fullerene. Mat Wiss Werkst. 44, 124–128. doi: 10.1002/mawe.201300082
Flohé, L., and Günzler, W. A. (1984). Assay of glutathione peroxidase. Methods Enzymol. 105, 114–120. doi: 10.1016/S0076-6879(84)05015-1
Foley, S., Crowley, C., Smaihi, M., Bonfils, C., Erlanger, B. F., Seta, P., et al. (2002). Cellular localisation of a water-soluble fullerene derivative. Biochem. Biophys. Res. Commun. 294, 116–119. doi: 10.1016/S0006-291X(02)00445-X
Franskevych, D., Palyvoda, K., Petukhov, D., Prylutska, S., Grynyuk, I., Schuetze, C., et al. (2017). Fullerene C60 penetration into leukemic cells and its photoinduced cytotoxic effects. Nanoscale Res. Lett. 12:40. doi: 10.1186/s11671-016-1819-5
Gharbi, N., Pressac, M., Hadchouel, M., Szwarc, H., Wilson, S. R., and Moussa, F. (2005). [60]fullerene is a powerful antioxidant in vivo with no acute or subacute toxicity. Nano Lett. 5, 2578–2585. doi: 10.1021/nl051866b
Ghiasvand, R., Askari, G., Malekzadeh, J., Hajishafiee, M., Daneshvar, P., Akbari, F., et al. (2012). Effects of six weeks of beta-alanine administration on VO(2) max, time to exhaustion and lactate concentrations in physical education students. Int. J. Prev. Med. 3, 559–563.
Graham, T., Sjogaard, G., Lollgen, H., and Saltin, B. (1978). NAD in muscle of man at rest during exercise. Pflugers Arch. 376, 35–39. doi: 10.1007/BF00585245
Halenova, T. I., Vareniuk, I. M., Roslova, N. M., Dzerzhynsky, M. E., Savchuk, O. M., Ostapchenko, L. I., et al. (2016). Hepatoprotective effect of orally applied water-soluble pristine C60 fullerene against CCl4-induced acute liver injury in rats. RSC Adv. 6, 100046–100055. doi: 10.1039/C6RA20291H
Halliwell, B., and Gutteridge, J. M. C. (1989). Free Radicals in Biology and Medicine, 2nd Edn. Oxford: Clarendon Press.
Harris, R. C., and Sale, C. (2012). Beta-alanine supplementation in high-intensity exercise. Med. Sport Sci. 59, 1–17. doi: 10.1159/000342372
Hohorst, H. J. (1970). “a-(+)-Lactate: Bestimmung mit Lactataehydrogenase und NAD,” in Methoden der Enzymatischen Analyse, ed. H. U. Bergmeyer (Weinheim: Verleg Chemie), 1425–1429.
Hollander, J., Fiebig, R., Gore, M., Bejma, J., Ookawara, T., Ohno, H., et al. (1999). Superoxide dismutase gene expression in skeletal muscle: fiber-specific adaptation to endurance training. Am. J. Physiol. 277(3 Pt 2), R856–R862. doi: 10.1152/ajpregu.1999.277.3.R856
Janero, D. R., and Hreniuk, D. (1996). Suppression of TCA cycle activity in the cardiac muscle cell by hydroperoxide–induced oxidant stress. Am. Physiol. 270, C1735–C1742. doi: 10.1152/ajpcell.1996.270.6.C1735
Ji, L. L., and Fu, R. (1992). Responses of glutathione system and antioxidant enzymes to exhaustive exercise and hydroperoxide. J. Appl. Physiol. 72, 549–554. doi: 10.1152/jappl.1992.72.2.549
Ji, L. L., Fu, R. G., and Mitchell, E. (1992). Glutathione and antioxidant enzymes in skeletal muscle: effects of fiber type and exercise intensity. J. Appl. Physiol. 73, 1854–1859. doi: 10.1152/jappl.1992.73.5.1854
Kamat, J. P., Devasagayam, T. P., Priyadarsini, K. I., and Mohan, H. (2000). Reactive oxygen species mediated membrane damage induced by fullerene derivatives and its possible biological implications. Toxicology 155, 55–61. doi: 10.1016/S0300-483X(00)00277-8
Kostyukov, A. I., Hellström, F., Korchak, O. E., Radovanovic, S., Ljubisavljevic, M., Windhorst, U., et al. (2000). Fatigue effects in the cat gastrocnemius during frequency-modulated efferent stimulation. Neuroscience 92, 789–799. doi: 10.1016/S0306-4522(00)00066-X
Lalwani, G., and Sitharaman, B. (2013). Multifunctional fullerene- and metallofullerene-based nanobiomaterials. Nano LIFE 3:1342003. doi: 10.1142/S1793984413420038
Lawler, J. M., Powers, S. K., Visser, T., Van Dijk, H., Korthuis, M. J., and Ji, L. L. (1993). Acute exercise and skeletal muscle antioxidant and metabolic enzymes: Effect of fiber type and age. Am. J. Physiol. 265, R1344–R1350. doi: 10.1152/ajpregu.1993.265.6.R1344
Leeuwenburgh, C., Fiebig, R., Chandwaney, R., and Ji, L. L. (1994). Aging and exercise training in skeletal muscle: responses of glutathione and antioxidant enzyme systems. Am. J. Physiol. 267(2 Pt 2), R439–R445. doi: 10.1152/ajpregu.1994.267.2.R439
Leeuwenburgh, C., Hollander, J., Leichtweis, S., Griffith, M., Core, M., and Ji, L. L. (1997). Adaptation of glutathion antioxidant system to endurance training are tissue and fiber specific. Am. J. Physiol. 272, R363–R369. doi: 10.1152/ajpregu.1997.272.1.R363
Lyon, D. Y., Adams, L. K., Falkner, J. C., and Alvarez, P. J. (2006). Antibacterial activity of fullerene water suspensions: effects of preparation method and particle size. J. Environ. Sci. Technol. 40, 4360–4366. doi: 10.1021/es0603655
Mashino, T., Shimotohno, K., Ikegami, N., Nishikawa, D., Okuda, K., Takahashi, K., et al. (2005). Human immunodeficiency virus-reverse transcriptase inhibition and hepatitis C virus RNA-dependent RNA polymerase inhibition activities of fullerene derivatives. Bioorg. Med. Chem. Lett. 15, 1107–1109. doi: 10.1016/j.bmcl.2004.12.030
McCord, J. M., and Fridovich, I. (1969). Superoxide dismutase: an enzymic function for erythrocuprein (hemocuprein). J. Biol. Chem. 244, 6049–6055.
Meister, A., and Anderson, M. E. (1983). Glutatione. Annu. Rev. Biochem. 52, 711–760. doi: 10.1146/annurev.bi.52.070183.003431
Misra, H., and Fridovich, I. (1972). The role of superoxide anion in the autoxidation of epinephrine and a simple assay superoxide dismutase. J. Biol. Chem. 247, 3170–3175.
Mori, T., Takada, H., Ito, S., Matsubayashi, K., Miwa, N., and Sawaguchi, T. (2006). Preclinical studies on safety of fullerene upon acute oral administration and evaluation for no mutagenesis. Toxicology 225, 48–54. doi: 10.1016/j.tox.2006.05.001
Nakamura, S., and Mashino, T. (2012). Water-soluble fullerene derivatives for drug discovery. J. Nippon Med. Sch. 79, 248–254. doi: 10.1272/jnms.79.248
Ohishi, S., Kizaki, T., Nagasawa, J., Izawa, T., Komabayashi, T., Nagata, N., et al. (1997). Effects of endurance training on superoxide dismutase activity, content and mRNA expression in rat muscle. Clin. Exp. Pharmacol. Physiol. 24, 326–332. doi: 10.1111/j.1440-1681.1997.tb01196.x
Powers, S. K., Criswell, D., Lawler, J., Ji, L. L., Martin, D., Herb, R. A., et al. (1994a). Influence of exercise and fiber type on antioxidant enzyme activity in rat skeletal muscle. Am. J. Physiol. 266, R375–R380. doi: 10.1152/ajpregu.1994.266.2.R375
Powers, S. K., Criswell, D., Lawler, J., Martin, D., Ji, L. L., Herb, R. A., et al. (1994b). Regional training-induced alterations in diaphragmatic oxidative and antioxidant enzymes. Respir. Physiol. 95, 227–237. doi: 10.1016/0034-5687(94)90118-X
Powers, S. K., Criswell, D., Lawler, J., Martin, D., Lien, F. K., Ji, L. L., et al. (1993). Rigorous exercise training increases superoxide dismutase activity in the ventricular myocardium. Am. J. Physiol. 265, H2094–H2098. doi: 10.1152/ajpheart.1993.265.6.H2094
Powers, S. K., and Jackson, M. J. (2008). Exercise-induced oxidative stress: cellular mechanisms and impact on muscle force production. Physiol. Rev. 88, 1243–1276. doi: 10.1152/physrev.00031.2007
Powers, S. K., Ji, L. L., and Leeuwenburgh, C. (1999). Exercise training-induced alterations in skeletal muscle antioxidant capacity: a brief review. Med. Sci. Sports Exerc. 31, 987–997. doi: 10.1097/00005768-199907000-00011
Prylutska, S., Grynyuk, I., Matyshevska, O., Prylutskyy, Y., Evstigneev, M., Scharff, P., et al. (2014). C60 fullerene as synergistic agent in tumor-inhibitory doxorubicin treatment. Drugs R D 14, 333–340. doi: 10.1007/s40268-014-0074-4
Prylutska, S. V., Grynyuk, I. I., Grebinyk, S. M., Matyshevska, O. P., Prylutskyy, Y. I., Ritter, U., et al. (2009). Comparative study of biological action of fullerenes C60 and carbon nanotubes in thymus cells. Mat Wiss Werkst. 40, 238–241. doi: 10.1002/mawe.200900433
Prylutska, S. V., Grynyuk, I. I., Matyshevska, O. P., Prylutskyy, Y. I., Ritter, U., and Scharff, P. (2008). Anti-oxidant properties of C60 fullerenes in vitro. Fuller. Nanotubes Carbon Nanostruct. 16, 698–705. doi: 10.1080/15363830802317148
Prylutska, S. V., Politenkova, S. V., Afanasieva, K. S., Korolovych, V. F., Bogutska, K. I., Sivolob, A. V., et al. (2017). A nanocomplex of C60 fullerene with cisplatin: design, characterization and toxicity. Beilstein J. Nanotechnol. 8, 1494–1501. doi: 10.3762/bjnano.8.149
Prylutskyy, Y. I., Vereschaka, I. V., Maznychenko, A. V., Bulgakovva, N. V., Gonchar, O. O., Kyzyma, O. A., et al. (2017). C60 fullerene as therapeutic agent for correcting and preventing skeletal muscle fatigue. J. Nanobiotechnol. 15:8. doi: 10.1186/s12951-016-0246-1
Radák, Z., Asano, K., Inoue, M., Kizaki, T., Oh-Ishi, S., Suzuki, K., et al. (1995). Superoxide dismutase derivative reduces oxidative damage in skeletal muscle of rats during exhaustive exercise. J. Appl. Physiol. 79, 129–135. doi: 10.1152/jappl.1995.79.1.129
Radák, Z., Asano, K., Inoue, M., Kizaki, T., Oh-Ishi, S., Suzuki, K., et al. (1996). Superoxide dismutase derivative prevents oxidative damage in liver and kidney of rats induced by exhausting exercise. Eur. J. Appl. Physiol. Occup. Physiol. 72, 189–194. doi: 10.1007/BF00838637
Reid, M. B., Stokić, D. S., Koch, S. M., Khawli, F. A., and Lois, A. A. (1994). N-acetylcysteine inhibits muscle fatigue in humans. J. Clin. Invest. 94, 2468–2474. doi: 10.1172/JCI117615
Ritter, U., Prylutskyy, Y. I., Evstigneev, M. P., Davidenko, N. A., Cherepanov, V. V., Senenko, A. I., et al. (2015). Structural features of highly stable reproducible C60 fullerene aqueous colloid solution probed by various techniques. Fuller. Nanotubes Carbon Nanostruct. 23, 530–534. doi: 10.1080/1536383X.2013.870900
Sahlin, K. (1985). NADH in human skeletal muscle during short-term intense exercise. Pflugers Arch. 403, 193–196. doi: 10.1007/BF00584099
Sahlin, K., Katz, A., and Henriksson, J. (1987). Redox state and lactate accumulation in human skeletal muscle during dynamic exercise. Biochem. J. 245, 551–556. doi: 10.1042/bj2450551
Sandstrom, M. E., Zhang, S. J., Bruton, J., Silva, J. P., Reid, M. B., Westerblad, H., et al. (2006). Role of reactive oxigen species in contraction-mediated glucose transport in mouse skeletal muscle. J. Physiol. 575(Part 1), 251–262. doi: 10.1113/jphysiol.2006.110601
Scharff, P., Carta-Abelmann, L., Siegmund, C., Matyshevska, O. P., Prylutska, S. V., Kova, T. V., et al. (2004). Effect of X-ray and UV irradiation of the C60 fullerene aqueous solution on biological samples. Carbon 42, 1199–1201. doi: 10.1016/j.carbon.2003.12.055
Schnuck, J. K., Sunderland, K. L., Kuennen, M. R., and Vaughan, R. A. (2016). Characterization of the metabolic effect of β-Alanine on markers of oxidative metabolism and mitochondrial biogenesis in skeletal muscles. J Exerc. Nutrition Biochem. 20, 034–041. doi: 10.20463/jenb.2016.o6.20.2.5
Schuetze, C., Ritter, U., Scharff, P., Bychko, A., Prylutska, S., Rybalchenko, V., et al. (2011). Interaction of N-fluorescein-5-isothiocyanate pyrrolidine-C60 compound with a model bimolecular lipid membrane. Mater. Sci. Engineer. C 31, 1148–1150. doi: 10.1016/j.msec.2011.02.026
Sedlak, J., and Lindsay, R. H. (1968). Estimation of total, protein-bound, and nonprotein sulfhydryl groups in tissue with Ellman’s reagent. Anal. Biochem. 25, 192–205. doi: 10.1016/0003-2697(68)90092-4
Sen, C. K. (1995). Oxidants and antioxidants in exercise. J. Appl. Physiol. 79, 675–686. doi: 10.1152/jappl.1995.79.3.675
Sen, C. K., and Packer, L. (2000). Thiol homeostasis and supplements in physical exercise. Am. J. Clin. Nutr. 72, 653–669. doi: 10.1093/ajcn/72.2.653S
Sen, C. K., Rankinen, T., Vaisanen, S., and Rauramaa, R. (1994). Oxidative stress after human exercise: effect of N-acetylcysteine supplementation. J. Appl. Physiol. 76, 2570–2577. doi: 10.1152/jappl.1994.76.6.2570
Shindoh, C., DiMarco, A., Thomas, A., Manubay, P., and Supinski, G. (1990). Effect of N-acetylcysteine on diaphragm fatigue. J. Appl. Physiol. 68, 2107–2113. doi: 10.1152/jappl.1990.68.5.2107
Skamrova, G. B., Laponogov, I. V., Buchelnikov, A. S., Shckorbatov, Y. G., Prylutska, S. V., Ritter, U., et al. (2014). Interceptor effect of C60 fullerene on the in vitro action of aromatic drug molecules. Eur. Biophys. J. 43, 265–276. doi: 10.1007/s00249-014-0960-2
Song, M., Liu, S., Yin, J., and Wang, H. (2011). Interaction of human serum album and C60 aggregates in solution. Int. J. Mol. Sci. 12, 4964–4974. doi: 10.3390/ijms12084964
Stout, J. R., Cramer, J. T., Zoeller, R. F., Torok, D., Costa, P., Hoffman, J. R., et al. (2007). Effects of beta-alanine supplementation on the onset of neuromuscular fatigue and ventilatory threshold in women. Amino Acid 32, 381–386. doi: 10.1007/s00726-006-0474-z
Summermatter, S., and Handschin, C. (2012). PGC-1 alpha and exercise in control of body weight. Int. J. Obest. 36, 1428–1435. doi: 10.1038/ijo.2012.12
Supinski, G. S., Stofan, D., Ciufo, R., and DiMarco, A. (1997). N-acetylcysteine administration alters the response to inspiratory loading in oxygen-supplemented rats. J. Appl. Physiol. 82, 1119–1125. doi: 10.1152/jappl.1997.82.4.1119
Świdwińska-Gajewska, A. M., and Czerczak, S. (2016). Fullerenes: characteristics of the substance, biological effects and occupational exposure levels. Med. Pr. 67, 397–410. doi: 10.13075/mp.5893.00352
Tolkachov, M., Sokolova, V., Korolovych, V., Prylutskyy, Y., Epple, M., Ritter, U., et al. (2016). Study of biocompatibility effect of nanocarbon particles on various cell types in vitro. Mat Wiss Werkst. 47, 216–221. doi: 10.1002/mawe.201600486
Venditti, P., and Di Meo, S. (1997). Effect of training on antioxidant capacity, tissue damage, and endurance of adult male rats. Int. J. Sports Med. 18, 497–502. doi: 10.1055/s-2007-972671
Wang, S. Y., Huang, W. C., Liu, C. C., Wang, M. F., Ho, C. S., Huang, W. P., et al. (2012). Pumpkin (Cucurbita moschata) fruit extract improves physical fatigue and exercise performance in mice. Molecules 17, 11864–11876. doi: 10.3390/molecules171011864
Weisiger, R. A., and Fridovich, I. (1973). Superoxide dismutase: organelle specificity. J. Biol. Chem. 248, 3582–3592.
Wolff, S. P. (1994). Ferrous ion oxidation in presence of ferric ion indicator xylenol orange for measurement of hydroperoxides. Methods Enzymol. 233, 182–189. doi: 10.1016/S0076-6879(94)33021-2
Youle, R. J., and Karbowski, M. (2005). Mitochondrial fission in apoptosis. Nat. Rev. Mol. Cell Biol. 6, 657–663. doi: 10.1038/nrm1697
Keywords: C60 fullerene, skeletal muscles fatigue, electrical stimulation, oxidative stress markers, antioxidant system
Citation: Vereshchaka IV, Bulgakova NV, Maznychenko AV, Gonchar OO, Prylutskyy YI, Ritter U, Moska W, Tomiak T, Nozdrenko DM, Mishchenko IV and Kostyukov AI (2018) C60 Fullerenes Diminish Muscle Fatigue in Rats Comparable to N-acetylcysteine or β-Alanine. Front. Physiol. 9:517. doi: 10.3389/fphys.2018.00517
Received: 05 February 2018; Accepted: 20 April 2018;
Published: 15 May 2018.
Edited by:
Gareth Davison, Ulster University, United KingdomReviewed by:
Michalis G. Nikolaidis, Aristotle University of Thessaloniki, GreeceMutay Aslan, Akdeniz University, Turkey
Copyright © 2018 Vereshchaka, Bulgakova, Maznychenko, Gonchar, Prylutskyy, Ritter, Moska, Tomiak, Nozdrenko, Mishchenko and Kostyukov. This is an open-access article distributed under the terms of the Creative Commons Attribution License (CC BY). The use, distribution or reproduction in other forums is permitted, provided the original author(s) and the copyright owner are credited and that the original publication in this journal is cited, in accordance with accepted academic practice. No use, distribution or reproduction is permitted which does not comply with these terms.
*Correspondence: Inna V. Vereshchaka, aW5uYS52QGJpcGgua2lldi51YQ==