- 1Department of Nephrology, EMMS Nazareth Hospital, Galilee Faculty of Medicine, Bar-Ilan University, Ramat Gan, Israel
- 2Department of Obstetrics and Gynecology, EMMS Nazareth Hospital, Galilee Faculty of Medicine, Bar-Ilan University, Ramat Gan, Israel
- 3Laboratory Medicine, EMMS Nazareth Hospital, Galilee Faculty of Medicine, Bar-Ilan University, Ramat Gan, Israel
- 4Department of Physiology, The Ruth and Burce Rappaport Faculty of Medicine, Technion–IIT, Haifa, Israel
- 5Department of Laboratory Medicine, Rambam Health Campus, Haifa, Israel
Preeclampsia is a serious complication of pregnancy where it affects 5–8% of all pregnancies. It increases the morbidity and mortality of both the fetus and pregnant woman, especially in developing countries. It deleteriously affects several vital organs, including the kidneys, liver, brain, and lung. Although, the pathogenesis of preeclampsia has not yet been fully understood, growing evidence suggests that aberrations in the angiogenic factors levels and coagulopathy are responsible for the clinical manifestations of the disease. The common nominator of tissue damage of all these target organs is endothelial injury, which impedes their normal function. At the renal level, glomerular endothelial injury leads to the development of maternal proteinuria. Actually, peripheral vasoconstriction secondary to maternal systemic inflammation and endothelial cell activation is sufficient for the development of preeclampsia-induced hypertension. Similarly, preeclampsia can cause hepatic and neurologic dysfunction due to vascular damage and/or hypertension. Obviously, preeclampsia adversely affects various organs, however it is not yet clear whether pre-eclampsia per se adversely affects various organs or whether it exposes underlying genetic predispositions to cardiovascular disease that manifest in later life. The current review summarizes recent development in the pathogenesis of preeclampsia with special focus on novel diagnostic biomarkers and their relevance to potential therapeutic options for this disease state. Specifically, the review highlights the renal manifestations of the disease with emphasis on the involvement of angiogenic factors in vascular injury and on how restoration of the angiogenic balance affects renal and cardiovascular outcome of Preeclamptic women.
Introduction
Preeclampsia (PE) is a profound complication of pregnancy, where it affects 3–8% of all pregnancies and dramatically increases the risk of all-cause mortality, especially in women who experienced early, severe, preterm episode (Backes et al., 2011; Jim and Karumanchi, 2017). Preeclampsia negatively affects both the mother and fetus (Table 1). Concerning the latter, preeclampsia may cause intra-uterine fetal growth restriction (IUGR), placental abruption, preterm delivery and associated complications including, neonatal respiratory distress syndrome, cerebral palsy, necrotizing enterocolitis retinopathy of prematurity and even perinatal death (Table 1; Backes et al., 2011). Besides its deleterious impact on the fetus, preeclampsia also affects the pregnant woman, where it is associated with hypertension, kidney damage, liver injury/failure, central nervous system (CNS) damage, stroke, cardiomyopathy, pulmonary edema, adult respiratory distress syndrome, and even death (Table 1; Berg et al., 1996; Vikse et al., 2008; Ghulmiyyah and Sibai, 2012). Actually, preeclampsia is responsible for more than 60,000 maternal deaths annually worldwide, placing it as the third cause of maternal mortality after bleeding and embolism (Mongraw-Chaffin et al., 2010; Young et al., 2010). Higher mortality rate was observed when preeclampsia is associated with HELLP (hemolysis, elevated liver enzymes, low platelets), syndrome liver hemorrhage or rupture, acute kidney injury (AKI), oliguria, disseminated intravascular coagulation (DIC), and pulmonary edema (Ghulmiyyah and Sibai, 2012). Preeclampsia is of special relevance in the developing countries, where the maternal mortality is ∼15% compared with 0–1.8% in the developed countries (Ghulmiyyah and Sibai, 2012). This difference is largely attributed to inadequate perinatal care in poor regions of the world, and subsequently missing timely detection of hypertension, generalized or local edema, and proteinuria to detect preeclampsia at early stages.
In the last decade, the definition of preeclampsia was revisited as the mechanisms underlying the disease were dramatically evolved. Concerning the former, several leading groups have challenged the half century old classic definition of preeclampsia, namely, de novo hypertension, new onset of proteinuria and liver dysfunction after mid pregnancy, motivated by the discovery of additional biomarkers of preeclampsia (Tjoa et al., 2007; Staff et al., 2013; Palomaki et al., 2015; Baltajian et al., 2016). In this context, several studies have suggested to modernize the definition by incorporating key biomarkers of either placental or vascular origins, including placenta growth factor (PlGF) and antiangiogenic factors such as soluble fms-like tyrosine kinase-1 (sFLT1) or soluble endoglin (sENG) in the diagnosis of preeclampsia and the risk for developing the disease and even in predicting the outcome (Tjoa et al., 2007; Staff et al., 2013; March et al., 2015; Palomaki et al., 2015; Sircar et al., 2015; Baltajian et al., 2016). The suggested definition takes into account the impressive advancement in understanding the pathophysiology of preeclampsia and the mechanism-based novel diagnostics and therapeutic options.
In light of the rapid pace in the development of this issue and its clinical relevance, the current review concentrates on recent breakthroughs in diagnosing preeclampsia and the derived therapeutic options, which are currently been tested in advanced clinical trials. The initial results seem encouraging and may break down the old dogma claiming that no intervention has been proved to prevent or delay the onset of preeclampsia and the only effective treatment is delivery.
Risk Factors for Preeclampsia
Although the mechanisms of preeclampsia are poorly elucidated, there are several predisposing factors that increase the risk for the development of the disease (Table 2; Al-Jameil et al., 2014). Among the leading risk factors (yet uncommon) is antiphospholipid antibody syndrome (APLA-S). In addition, numerous epidemiological studies have demonstrated that chronic kidney disease (CKD) significantly increases the risk of preeclampsia, especially lupus (Roberts et al., 1989; Mostello et al., 2002; Clowse et al., 2008; Jim and Karumanchi, 2017). Risk factors for pre-eclampsia include also former preeclampsia, first pregnancy, obesity, pregestational hypertension, older age, and diabetes mellitus (Al-Jameil et al., 2014). It is also more frequent in multifetal pregnancy, where the incidence of preeclampsia is increased in twin compared to singleton pregnancies to 6–31% (McFarlane and Scott, 1976; Coonrod et al., 1995). Despite the association between these risk factors and preeclampsia, the mechanisms whereby these factors increase this risk are largely unknown. However, underlying diseases characterized by imbalance of angiogenetic factors and coagulation may explain why certain populations are at risk. Despite that, in most cases preeclampsia is unpredictable (Jim and Karumanchi, 2017).
Pathogenesis of Preeclampsia
In the last decade, our understanding of the pathogenesis of preeclampsia has progressively advanced (Phipps et al., 2016). Therefore, in this section we will focus on the most recent concepts in the pathogenesis of the disease, especially the involvement of angiogenic factors. It is obvious today that preeclampsia is a systemic disease characterized by generalized endothelial damage (Roberts et al., 1989), thus negatively affecting almost all organs of preeclamptic women, including the potential to affect future cardiovascular and renal diseases even decades after the disease occurrence (Figure 1; Berg et al., 1996; Vikse et al., 2008). In this context, a comprehensive prospective study revealed that preeclampsia was independently associated with cardiovascular disease death (mutually adjusted hazard ratio: 2.14 [95% CI: 1.29–3.57]) (Mongraw-Chaffin et al., 2010). The situation was even grimmer in women who experienced preeclampsia by 34 weeks of gestation (HR, 9.54; 95% CI, 4.50–20.25) (Mongraw-Chaffin et al., 2010). The high mortality rate could be explained by the findings that early-onset preeclampsia conferred a substantially higher risk of cardiovascular, respiratory, CNS, renal, hepatic, and other morbidity and was evident by end target damage (Lisonkova et al., 2014). Collectively, these findings suggest that the risk of morbidity/mortality among preeclamptic women is related to the severity the disease and gestational age at onset, namely early (<34 weeks) or late (>34 weeks). However, it should be emphasized that if the mother has a genetic predisposition to cardiovascular disease, then it is this rather than pre-eclampsia per se that causes the increased morbidity in later life as outlined above. Therefore, additional studies are needed to distinguish between the contribution of preeclampsia itself and the genetics to the high prevalence of cardiovascular morbidity and mortality among preeclamptic women.
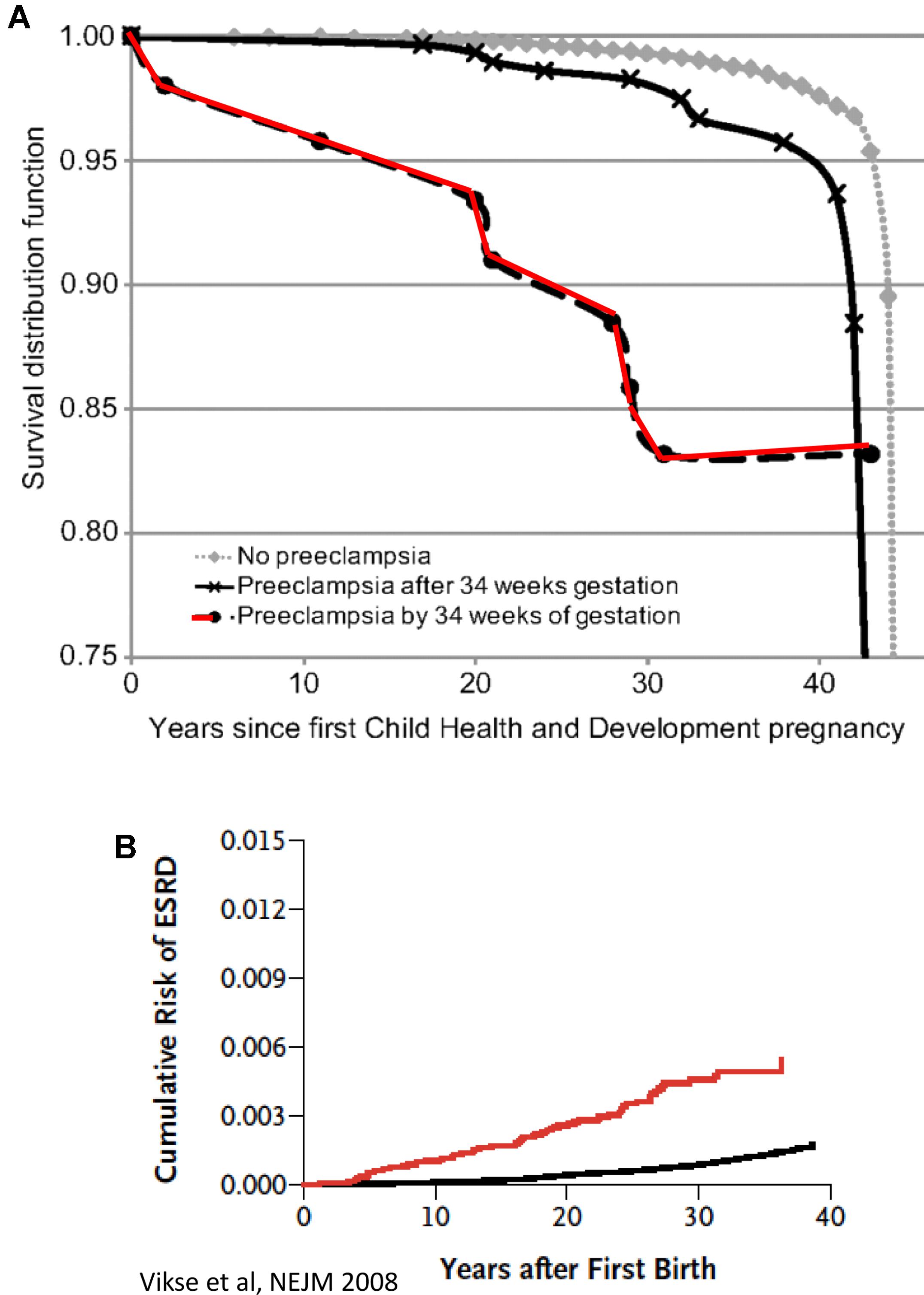
FIGURE 1. (A) CVD death Kaplan–Meier survival according to gestational timing of preeclampsia. Survival analysis is based on 14, 403 pregnant women. A total of 481 had observed preeclampsia, and 266 died from cardiovascular disease (adopted with permission from Mongraw-Chaffin et al., 2010). (B) Cumulative risk of end-stage renal disease (ESRD) after first preeclampsia (adopted with permission from Vikse et al., 2008).
It is now appreciated that early- and late-onset pre-eclampsia have different pathophysiologies, thus advancing our understanding of the syndrome. In early-onset, also referred to as placental pre-eclampsia, there is clear evidence of reduced maternal spiral artery conversion in early pregnancy. This is associated with placental malperfusion, and gross and molecular pathology of the placental tissues. Oxidative stress of the placenta causes increased secretion of sFLT-1 and reduced PlGF, reflecting the biomarker patterns. In late-onset pre-eclampsia, called also maternal preeclampsia, there is little evidence of reduced arterial conversion and placental perfusion is maintained or even increased (Sohlberg et al., 2014). Thus, there is only minimal placental stress (Yung et al., 2005) so that sFLT and placental growth factor (PlGF) secretion by the placenta are close to the normal range. These cases, which represent nearly 80% of pre-eclampsia, are now thought to be due to a genetic maternal pre-disposition to cardiovascular disease, which manifests as pre-eclampsia during the stress-test of pregnancy.
The pathology early-onset preeclampsia starts with abnormal formation of blood vessels in the maternal uterine spiral arteries. During normal pregnancy, major adaptive changes take place including spiral artery remodeling in the pregnant uterus aimed at decreasing maternal blood vessel resistance and subsequently increasing unteroplacental perfusion (Lyall, 2005). However, mathematical modeling shows that the remodeling has relatively little impact on uteroplacental perfusion, and is more concerned with reducing the velocity of inflow and ensuring constancy of blood flow (Burton et al., 2009).
These alterations in spiral arteries, namely high-capacitance low-pressure flow to the placenta, are essential for fetal nutrition. Spiral artery remodeling is achieved through invasion of trophoblasts and disappearance of the smooth muscle in the blood vessel wall (Kaufmann et al., 2003; Lyall, 2005; Osol and Mandala, 2009). Using mouse model revealed that this process involves the full circumference of the vessel in its segment entering the placenta from the mesometrial triangle, so called, the central canal. The deeper parts of the spiral artery within the mesometrial triangle and even beyond it, as deep as the mesometrium, are only partially remodeled and retain the muscular wall in part of their circumference (Geusens et al., 2008; Skarzinski et al., 2009; Figure 2). In order to achieve spiral remodeling during normal pregnancy, many molecules including vasoactive substances, growth factors, adhesion molecules and proteases are secreted by the placenta and the vasculature (Brosens et al., 1972; Norwitz et al., 2001; Kaufmann et al., 2003; Lyall, 2005; Pijnenborg et al., 2006). Among the most famous representative substances in this context are vascular endothelial growth factor (VEGF), sFlt1, PlGF, and endoglin (Takimoto et al., 1996; Maynard et al., 2003; Levine et al., 2004; Li et al., 2005; Venkatesha et al., 2006; Kanasaki et al., 2008; Zhou et al., 2008). Furthermore, interactions with the maternal immune cells, especially uterine natural killer cells and their corresponding human leukocyte antigen-C (HLA-C) ligands on the invading trophoblast, are important for release of proteases and remodeling (Moffett et al., 2015). Interference with their central role in creating efficient uteroplacental interface and cardiovascular and renal adaptations during pregnancy contributes to preeclampsia as elaborated below. It is widely accepted that abrupt remodeling of the uterine spiral arteries plays a key role in the pathogenesis of early onset preeclampsia (Brosens et al., 1972; Norwitz et al., 2001; Kaufmann et al., 2003; Red-Horse et al., 2004; Pijnenborg et al., 2006), yet there is no evidence that they are involved in arterial remodeling of the spiral arteries.
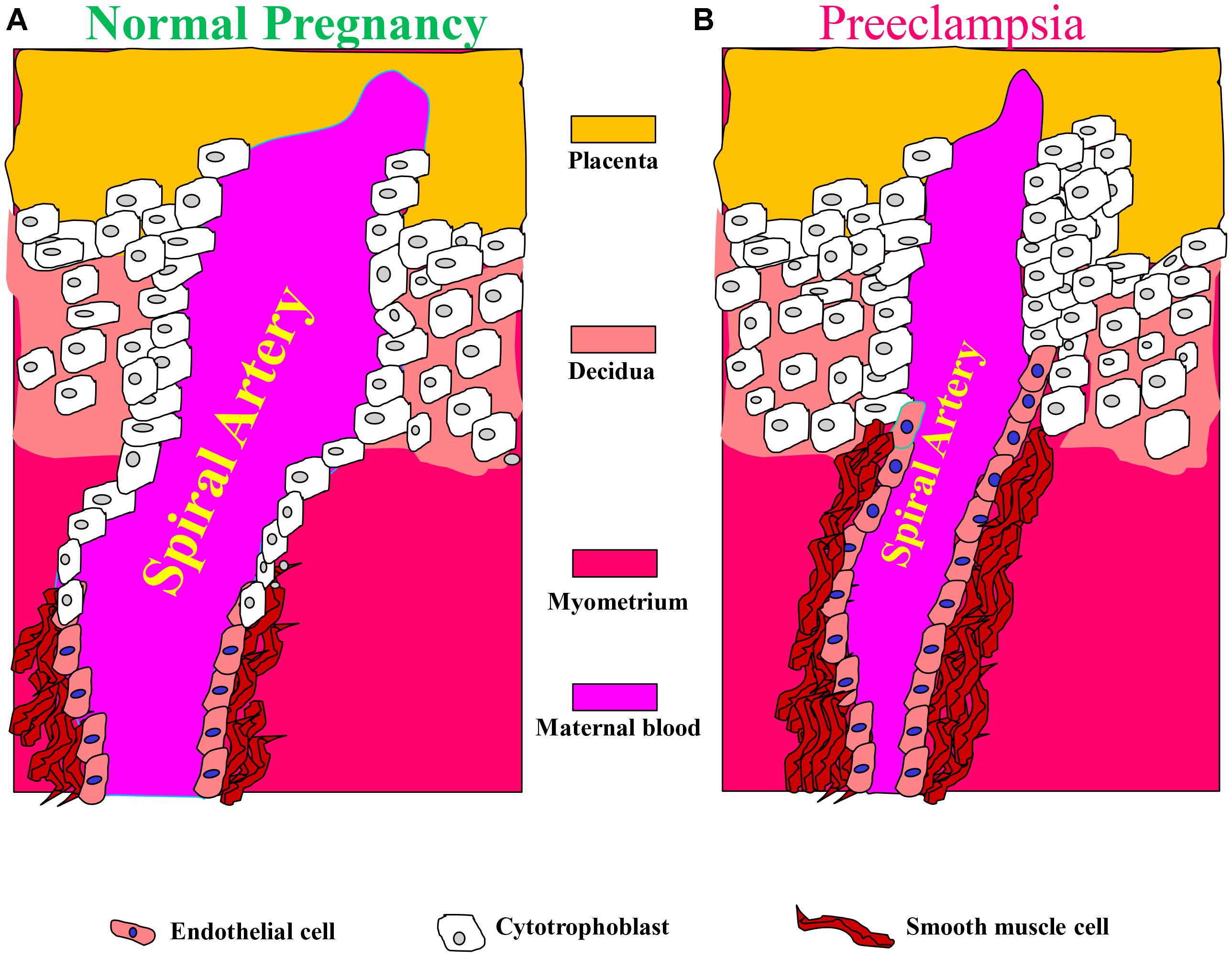
FIGURE 2. Aberrant placentation and angiogenesis in preeclampsia. In normal pregnancy, cytotrophoblasts of fetal origin invade the maternal spiral arteries and replace endothelial cell layer. This action converts the spiral arteries from narrow highly resistant vessels to high-caliber capacitance vessels, which are capable of providing sufficient blood and nutrition supply to the fetus. During the process of vascular invasion, the cytotrophoblasts differentiate from an epithelial to an endothelial phenotype, a process referred to as pseudovasculogenesis, or vascular mimicry (Right). In preeclampsia, cytotrophoblasts fails to acquire invasive endothelial phenotype features, thus the invasion of the spiral arteries is inadequate leaving them narrow and highly resistant (Left). Modified with permission from Lam et al. (2005) and Powe et al. (2011).
Angiogenic Factors
As mentioned above, insufficient spiral artery remodeling due to superficial invasion of trophoblasts is the basis for the development of early-, but not late-onset cases of preeclampsia (Brosens et al., 1972; Norwitz et al., 2001; Kaufmann et al., 2003; Red-Horse et al., 2004; Pijnenborg et al., 2006). Perturbations in the generation of normal uteroplacental interface results in ischemic placenta and oxidative stress which stimulates the release of prohypertensive and anti-angiogenic factors (such as sFlt-1) (Cindrova-Davies, 2009). Moreover, sFlt-1 sensitizes the endothelial cells of the maternal circulation to pro-inflammatory cytokines such as tumor necrosis factor-α (TNF-α) (Cindrova-Davies et al., 2011), causing generalized endothelial dysfunction and subsequently multisystem damage (Figure 3; Roberts et al., 1989; Llurba et al., 2015; Verdonk et al., 2015).
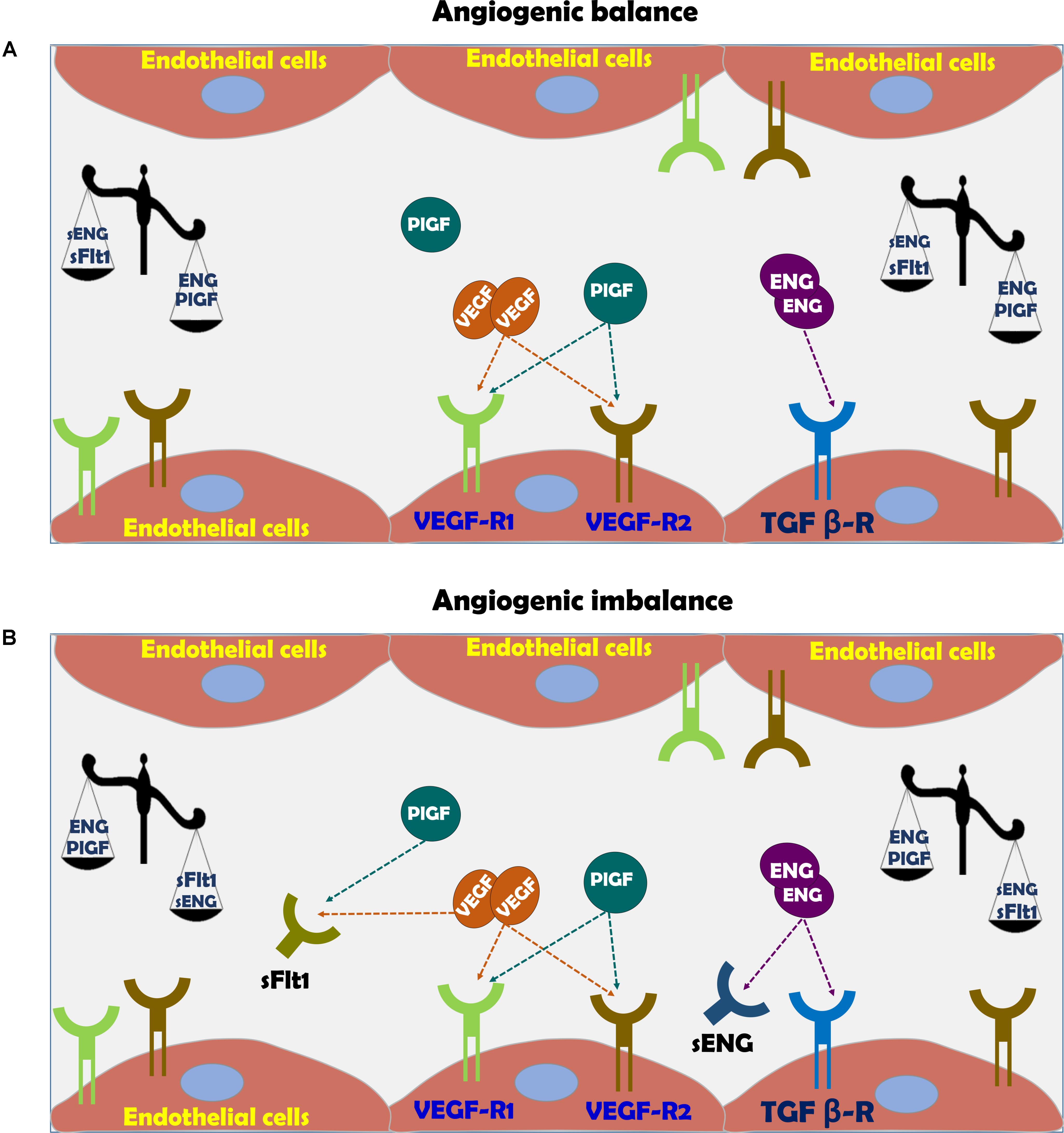
FIGURE 3. (A) Vascular function in normal pregnancy and (B) Vascular dysfunction in preeclampsia. In normal pregnancy there is angiogenic balance as evident by VEGF, PlGF, and TGF-β levels, which are necessary for normal blood vessel formation and endothelial function via eNOS activation and subsequent nitric oxide generation. The action of these factors is hampered in preeclampsia due to angiogenic imbalance, where soluble sFlt-1 and sEng act as scavengers for native VEGF, PlGF, and TGF-β, thus inducing endothelial dysfunction.
Placenta Growth Factor
Among these substances are VEGF sFlt1, PlGF, sENG, and endothelin (ET-1) (Figure 3; Llurba et al., 2015; Verdonk et al., 2015). Therefore, sFlt-1, PlGF and endoglin are extensively assessed as potential biomarkers for the diagnosis of preeclampsia (Venkatesha et al., 2006; Staff et al., 2013). While PlGF is proangiogenic, sFlt-1 is antiangiogenic factor (Ahmed, 2011). PlGF is expressed by the placenta, especially the syncytiotrophoblast (Maglione et al., 1991), but also by the endothelium (Staff et al., 2013). PlGF is a prominent angiogenic player in the development of the placental vascular system (Iwasaki et al., 2011; De Falco, 2012). During normal pregnancy, PlGF can be detected in the maternal circulation from 8 weeks gestation, reaching a maximal concentration toward the end of second trimester and declining thereafter until delivery (Taylor et al., 2003). In line with its proangiogenic function, reduced levels of PlGF were found in preeclampsia (George and Granger, 2010; Staff et al., 2013; Kar, 2014). It is noteworthy that infusion of recombinant human PlGF via intraperitoneal osmotic minipumps abolished the development of hypertension in experimental preeclampsia model (Spradley et al., 2016).
Soluble fms-Like Tyrosine Kinase-1
Vascular endothelial growth factor is critical for vascular homeostasis and activates both VEGF receptor-1 (VEGFR-1) and VEGFR-2 coupled to endothelial nitric oxide synthase (eNOS) required for angiogenesis (Figure 3; Ferrara, 2004; Ahmad et al., 2006; Sison et al., 2010; Bertuccio et al., 2011; Veron et al., 2012). The importance of VEGF for the maintenance of normal endothelial function and development of placental vasculature is derived from the consequences of impairment of VEGF activity due to certain drugs or elevation of sFlt-1 (Ahmed, 1997; Kabbinavar et al., 2003; Sison et al., 2010; Bertuccio et al., 2011; Veron et al., 2012). In this context, anti- VEGF therapy with Avastin display preeclampsia-like symptoms, namely hypertension and proteinuria (Kabbinavar et al., 2003; Eremina et al., 2008; Muller-Deile and Schiffer, 2011; Hayman et al., 2014). sFlt-1 is a splice variant of VEGF receptor fms-like tyrosine kinase 1 (Maynard et al., 2003). sFlt-1 acts as a potent scavenger of VEGF- and PlGF, thus preventing their interaction with endothelial receptors on the cell surface and subsequently induces endothelial dysfunction (Figure 3; Kendall and Thomas, 1993; Levine et al., 2004). The elevation of sFlt-1 is due to overexpression of sFlt-1 mRNA as was demonstrated in in vivo and in vitro models of human placental hypoxia mediated by hypoxia inducible factor 1 (HIF-1) (Nevo et al., 2006; Onda et al., 2017). Support for its pro preeclamptic role came from experimental studies, where administration of adenoviral enhanced overexpression of sFlt-1 into pregnant rats or mice, induced clinical manifestations of preeclampsia, including profound elevation of blood pressure (BP), albuminuria, and renal histologic changes such as endotheliosis and fibrin deposition within the enlarged glomeruli (Gartner et al., 1998; Maynard et al., 2003; Onda et al., 2017). At the mechanistic level, sFlt-1 indirectly prevents the production of VEGF-induced NO, resulting in enhanced generation of reactive oxygen species and exaggerated vasoconstriction (Ahmad and Ahmed, 2004; Burke et al., 2016).
In clinical setting, sFlt-1 levels were found to be elevated as early as 5 weeks before the diagnosis of preeclampsia and directly correlate with disease severity (Levine et al., 2004; Kar, 2014). Furthermore, support for sFlt-1 role in the pathogenesis of preeclampsia is derived from experimental and clinical studies involving sFlt-1 elimination (Ahmad and Ahmed, 2004; Thadhani et al., 2016; Jim and Karumanchi, 2017). Specifically, sFlt-1 removal by dextran sulfate apheresis in humans reduced proteinuria and prolonged pregnancy (Thadhani et al., 2016).
Endoglin
Endoglin (ENG) is a type I membrane glycoprotein localized to the cell membrane where it constitutes the transmembrane co-receptor for TGF beta receptor complex (TGF-β1 and TGF-β3) (Gregory et al., 2014). ENG is expressed by endothelial cells and monocytes, especially during neoangiogenesis and embryogenesis (Gregory et al., 2014). Concerning the latter, the human placenta, especially syncytiotrophoblast is an important source of ENG (Gougos and Letarte, 1990). The primary roles of ENG include angiogenesis, endothelial cell differentiation and regulation of vascular tone through eNOS (Ahmad and Ahmed, 2004). Proteolytic cleavage of the extracellular domain of endoglin, generates sEng that presumably functions as limiting factor for the activity of TGF-β and the coupled eNOS (Figure 3; Qu et al., 1998; Bourdeau et al., 1999). Since TGF-β acts as anti-inflammatory and vasodilator growth factor, its elimination by sEng leads to endothelial dysfunction characterized by vasoconstriction, overexpression of adhesion molecules and reduced T cells characterizing preeclamptic women (Matsubara et al., 2000; Ahmed, 2011). By using experimental model of preeclampsia, it was shown that sEng and sFlt-1 act synergistically to induce endothelial dysfunction especially the severe variant of the disease, namely HELLP syndrome (Santner-Nanan et al., 2009). Similarly, circulating sEng was found to be high in preeclamptic women even prior to the disease manifestations correlating with disease severity and falls after delivery (Levine et al., 2004; Venkatesha et al., 2006), making it a reliable predictor of patients destined to develop severe early-onset preeclampsia (Robinson and Johnson, 2007). The regulators of sEng release are largely unknown, however like sFlt-1, it was reported that both cytokines (Zhou et al., 2010), and autoantibodies to angiotensin II AT-1 receptors stimulate (Cudmore et al., 2007) and heme oxygenase-1 (HO-1) inhibits its release (see later) (Zhou et al., 2010).
Other Vasoactive Substances
One of the major features of preeclampsia is generalized vasoconstriction and reduced plasma volume, assumedly due to endothelial activation even weeks before clear evidence of the disease (Roberts et al., 1989; Roberts and Lain, 2002). Endothelial dysfunction is characterized by reduced blood flow to virtually all organs in preeclamptic women due to vasoconstriction. The latter is partially attributed to imbalance in neurohormonal systems, including activation of the sympathetic nervous system and renin angiotensin aldosterone system (RAAS) as well as endothelin (ET-1) (Gant et al., 1973; Roberts and Lain, 2002). On the other hand, endothelium-dependent vasodilation (PGs, VEGF, TGF-β, and NO system) is also attenuated in preeclamptic patients (Fischer et al., 2000; Yoshida et al., 2000), secondary to oxidative stress which is known to provoke endothelial dysfunction (Roberts et al., 1989; McKinney et al., 2000). Partial restoration of the balance (even for a short while) by water immersion of preeclamptic women increased cardiac output and reduced systemic vascular resistance (SVR), yet to a lower extent than normal pregnant women (Elvan-Taspinar et al., 2006). Yet, the therapeutic potential for water immersion in preeclampsia appears to be limited (Elvan-Taspinar et al., 2006). Several studies have reported elevated ET-1 levels in preeclampsia and some of them demonstrated a positive correlation between ET-1 and the severity of symptoms (Taylor et al., 1990; Mastrogiannis et al., 1991; Benigni et al., 1992; Granger et al., 2006; George and Granger, 2011, 2012; George et al., 2012). The cadence of ET-1 as mediator of many preeclampsia manifestations is appealing in light of its potent vasoconstrictory, inflammatory and proteinuric properties (Davenport et al., 2016; Saleh et al., 2016; Bakrania et al., 2017). Support for this notion is derived from animal models of preeclampsia, where it has been shown that endothelin receptor blockers prevent the development of the disease (Saleh et al., 2016; Bakrania et al., 2017).
Finally, HO-1 plays an anti-inflammatory and inhibitory role on sFlt-1 and sEng release via its metabolite carbon monoxide (CO) (Ahmed, 2011). In line with HO-1 involvement in the pathogenesis of preeclampsia, women with the disease exhale less CO than women with normal pregnancies and HO-1 expression decreases as the severity of preeclampsia increases (Ahmed, 2011). The downregulation of HO-1 aggravates the inflammatory aspect of preeclampsia, and deprives of the body from important anti stress and anti-oxidant defense mechanism (Ahmed, 2011).
Diagnosis of Preeclampsia
For more than half century, the clinical syndrome of preeclampsia is defined as de novo hypertension and new onset of proteinuria after mid pregnancy (ACOG practice bulletin, 2002). Hypertension is diagnosed when it is greater than 140 mmHg systolic or 90 mmHg diastolic at two separate times, more than 4 h apart in a woman after 20 weeks of gestation (Duley, 2003). In addition, proteinuria of >300 mg/day is milestone for the diagnosis of preeclampsia (Staff et al., 2013). However, in the last decade this concept has been challenged in light of the fact that the disease develops long time prior to its keen manifestations (Staff et al., 2013). Actually, early clinical signs of preeclampsia may be absent or unremarkable, and the reliability of these two hallmarks (hypertension and proteinuria) as gold standard is compromised, especially if the pregnant women suffer from predisposing conditions, such as chronic hypertension and CKD (Sibai and Stella, 2009). Therefore, the search for more sensitive and early biomarker of the disease continued all the time and is more zeal in the last decade. This issue is of great importance since early diagnosis of preeclampsia may be the first step in the journey for the development of effective treatment, especially if the biomarkers are of mechanistic relevance. In this context, new biomarkers were derived from the recent unprecedented advances in our understanding of the pathogenic mechanisms underlying preeclampsia (Ahmed, 2011; Staff et al., 2013; Phipps et al., 2016; Jim and Karumanchi, 2017). Specifically, it is now obvious that angiogenic imbalance, as reflected by elevated levels of sFlt-1, sEng, and ET-1 along decreased PlGF concentrations in the maternal circulation (Ahmed, 2011; Staff et al., 2013; Phipps et al., 2016; Saleh et al., 2016; Jim and Karumanchi, 2017), is the link between this syndrome and the malperfused placenta characterizing the early-onset pre-eclampsia, and the maternal genetic predisposition, as in the late-onset form. Therefore, sFlt-1, sEng, and PlGF are mounting biomarkers for the diagnosis of preeclampsia (Ahmed, 2011; Staff et al., 2013; Phipps et al., 2016; Jim and Karumanchi, 2017). Besides their diagnostic features, these biomarkers were found to possess prognostic features. For instance, Rana et al. (2013) showed that high ratio of sFlt to PlGF in preeclamptic women is associated with worse maternal and fetal outcomes compared with women with a lower ratio.
In a prospective multicenter observational study, Zeisler et al. (2016) examined whether ratio of serum sFlt-1 to PlGF predicts the absence or presence of preeclampsia in the short term in women with singleton pregnancies in whom preeclampsia was suspected (24 weeks 0 days to 36 weeks 6 days of gestation). These authors have shown that an sFlt-1-to-PlGF ratio of 38 or lower drawn at 24–37 weeks of gestation can reliably predict the absence of preeclampsia and fetal adverse outcomes within 1 week, with negative predictive values of 99.3 and 99.5%, respectively. Similarly, Sovio et al. (2017) who determined sFlt-1:PlGF ratio at 20, 28, and ≈36 weeks of gestational age in 4,099 women recruited to Pregnancy Outcome Prediction. At 28 gestational week, a sFlt-1:PlGF ratio >38 had a positive predictive value of 32% for preeclampsia and preterm birth. At 36 weeks, a sFlt-1:PlGF ratio >38 had a predictive value for severe preeclampsia of 20% in high-risk women and 6.4% in low-risk women. When sFlt-1:PlGF ratio was >110 it has predictive value of 30% for severe preeclampsia. Among low-risk women at 36 weeks, a sFlt-1:PlGF ratio ≤38 had a negative predictive value for severe preeclampsia of 99.2%. Collectively, the sFlt-1:PlGF ratio provided clinically useful prediction of the risk of the most important manifestations of preeclampsia, confirming the pioneer findings by Levine et al. (2004) and providing rational for the use of angiogenic biomarkers to stratify women at high risk for preeclampsia.
In similarity with sFlt-1, serum concentrations of sEng are elevated in preeclamptic women (Chen, 2009), as compared with stable levels throughout normal pregnancy. A positive correlation between the elevated serum levels of sEng and the severity of pre-eclampsia has been demonstrated (Chen, 2009). Noteworthy, serum sEng have been shown to be significantly increased before the onset of disease. Specifically, sEng levels increased as early as 9–11 weeks in pregnant women at risk for preeclampsia and by12–14 weeks in women with term preeclampsia (Levine et al., 2004). Thus, sEng could be used to predict preeclampsia at 11–13 week gestation (Akolekar et al., 2011) with precaution since high levels of sEng are detected also in other gestational disorders such as small gestational age, thus limiting its specificity. Therefore, the pattern of changes in the ratio of different combinations of PlGF/sEng; sflt-1 + sEng)/PlGF, at 13 weeks and around 20 weeks, is more informative than the individual biomarkers at single time-point screening (Levine et al., 2004; Romero et al., 2008; Rana et al., 2013).
Kidney Placenta Crosstalk
The kidney in normal pregnancy
There is an important crosstalk between the placenta and the kidney during normal pregnancy, as evident by adaptive anatomic and physiologic renal changes. The latter include an increase in renal size by 30% and length by 1–1.5 cm mainly due to the increased renal blood flow (RBF) as early as the first 4 weeks of pregnancy (Hussein and Lafayette, 2014). The collecting systems of both kidneys are normally dilated and are more pronounced on the right, therefore “Physiological hydronephrosis” can occur in late pregnancy. Moreover, reduction in BP secondary to generalized peripheral vasodilation due to the reduced SVR, is probably due to the increased resistance to angiotensin II (Gant et al., 1973). Likewise, imbalance between the vasodilatory prostacyclin and relaxin and vasoconstrictive thromboxane in favor of the first, and activation of nitric oxide (NO), a potent vasodilator that mediates endothelium dependent relaxation (Hussein and Lafayette, 2014), may contribute to this phenomenon. At the renal level, there is an increase in glomerular filtration rate (GFR) secondary to increased RBF by ∼35–50% (Hussein and Lafayette, 2014). For this reason, normal pregnancy is accompanied by low serum creatinine, urea, sodium, uric acid levels and increased urinary protein excretion up to 300 mg/d (Hussein and Lafayette, 2014). The renal vasodilation is responsible also for the activation of the RAAS. In fact, despite the elevated levels of renin and aldosterone in pregnant woman, both the BP and SVR are reduced (Gant et al., 1973).
The kidney in preeclampsia
The above-mentioned changes are partially realized in complicated pregnancy such as preeclampsia, where pathologic changes in both the placenta and the kidneys take place. One of the most vulnerable organ to miss adaptive changes during preeclampsia is the kidney, where glomerular endotheliosis and proteinuria develop (Spargo et al., 1959; Gartner et al., 1998; Craici et al., 2013). The hallmark characteristic renal pathologic lesion of preeclampsia “glomerular endotheliosis” is characterized by an enlarged bloodless glomerulus with obliteration of the capillary lumen, but usually not accompanied by prominent capillary thrombi (Figure 4; Spargo et al., 1959; Hussein and Lafayette, 2014). Initially, endothelial cell swelling and disruption of their fenestrae were thought to be the cause of proteinuria seen in preeclampsia (Hussein and Lafayette, 2014). However, there is increasing evidence that damage to the podocytes, the visceral epithelial glomerular cell, is largely responsible for the proteinuria (Figure 4). In this context podocyturia (loss of podocytes in the urine), along shedding of slit diaphragm proteins such as nephrin, podocin, synaptopodin and podocalyxin were noticed in preeclampsia, and even precede the typical clinical features of preeclampsia by several weeks (Garovic et al., 2007a,b, 2013; Aita et al., 2009; Zhao et al., 2009, 2011; Facca et al., 2012; Jim et al., 2012; Kelder et al., 2012; Wang et al., 2012; Chen et al., 2013; Son et al., 2013). It should be emphasized that these slit diaphragm proteins play a key role in maintaining the integrity of the glomerular barrier (Kestila et al., 1998).
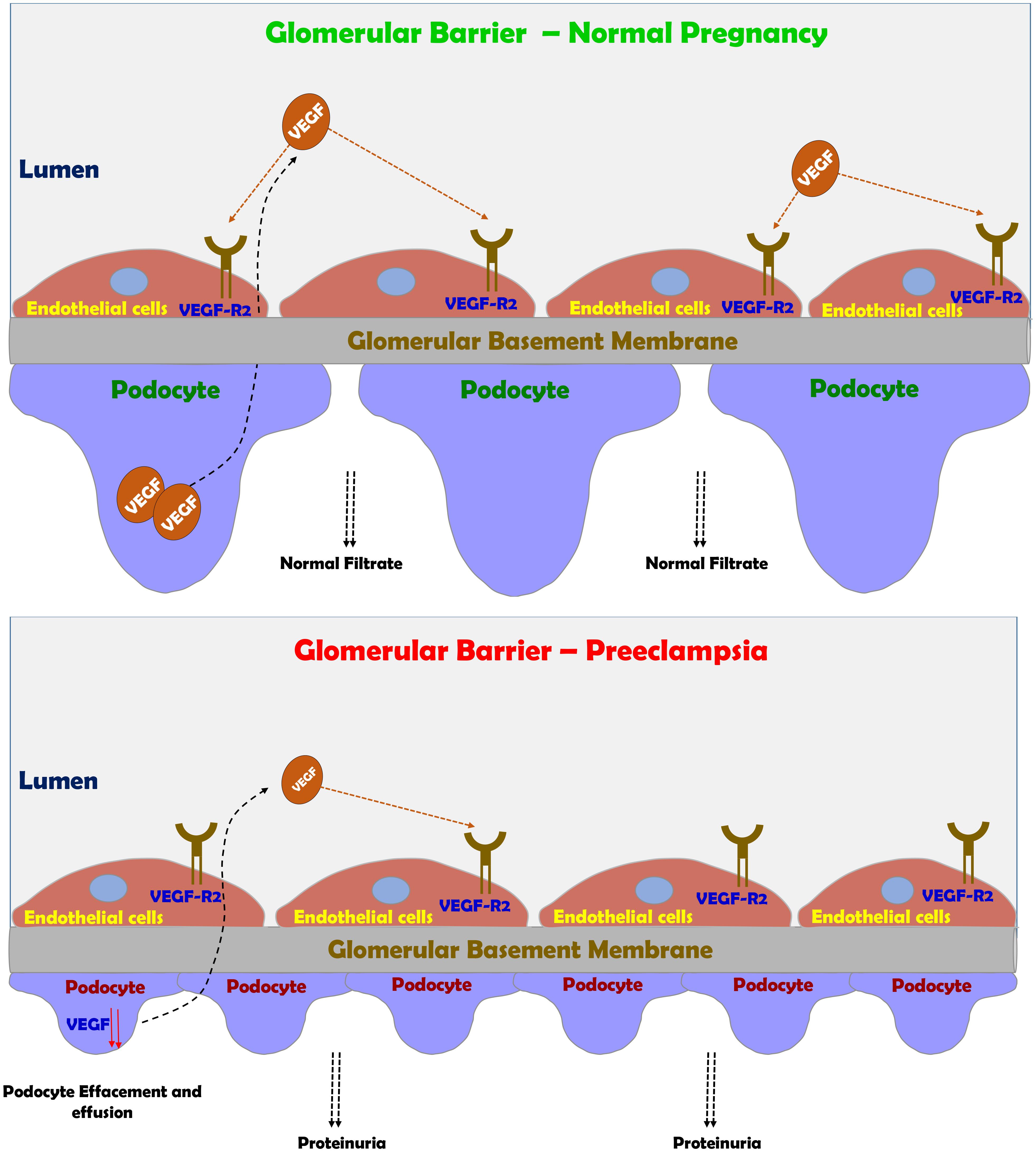
FIGURE 4. Preeclampsia-induced renal dysfunction. Under normal condition, VEGF plays a central role in maintaining the integrity of glomerular barrier as evident by healthy podocytes with normal foot process and slit diaphragm proteins. Under preeclamptic condition, angiogenic imbalance develops mainly due to sFlt-1 and sEng, leading to podocyte and endothelial damage in the glomerular filtration barrier and subsequently to the development of proteinuria.
The end result of this disruption of the glomerular filtration barrier and podocyte detachment is proteinuria (Figure 4; Spargo et al., 1959; Craici et al., 2013). In fact, there is a positive correlation between the grade of podocyturia and the severity of proteinuria. One of the main mediators of the adverse renal consequences of preeclampsia is ET-1 (Taylor et al., 1990; Mastrogiannis et al., 1991; Benigni et al., 1992; Granger et al., 2006; George and Granger, 2011, 2012; George et al., 2012; Verdonk et al., 2015; Davenport et al., 2016; Saleh et al., 2016; Bakrania et al., 2017). Support for this notion is derived from the observation that pre-eclamptic sera are not directly toxic to cultured podocytes, but if the glomerular capillary endothelium is exposed to sera from pre-eclamptic women it produces podocytes damage via ETA receptor subtype (Collino et al., 2008). Addition keen evidence for the involvement of ET-1 system in the pathogenesis of renal dysfunction characterizing preeclampsia came from the observation that podocyte damage and shedding can be prevented by ETA blockers (George and Granger, 2011; Verdonk et al., 2015; Bakrania et al., 2017). However, in the last decade, there is growing evidence that imbalance between the proangiogenic and anti angiogenic factors plays a key role in podocyte injury (Genbacev et al., 1997; Fulton et al., 1999; Robertson et al., 2003; Venkatesha et al., 2006; Baelde et al., 2007; Sison et al., 2010; Bertuccio et al., 2011; Veron et al., 2012). This concept is supported by the observation that bevacizumab, an anti-VEGF antibody used to treat patients with various types of cancer or diabetic proliferative retinopathy causes hypertension and proteinuria mimicking the effect of sFlt-1 (Eremina et al., 2008; Muller-Deile and Schiffer, 2011; Hayman et al., 2014). Interestingly, the renal findings in patients who were treated with bevacizumab including endotheliosis, thrombotic microangiopathy, and podocytes shedding, are similar to those found in preeclamptic state (Eremina et al., 2008; Muller-Deile and Schiffer, 2011; Hayman et al., 2014). Kidney damage during preeclampsia as evident by endothelial and podocytes’ loss contributes to the increased risk of later hypertension, CKD, ischemic heart disease, stroke, persistent proteinuria and finally ends stage renal disease (ESRD) (LaMarca B.D. et al., 2008; Vikse et al., 2008, 2012; McDonald et al., 2010; Kattah et al., 2013; Wang et al., 2013).
As mentioned above, superficial placental implantation due to abnormal angiogenesis is the early driving event for the development of preeclampsia. The imbalance between the pro-angiogenic VEGF and PlGF, and the antiangiogenic sFlt-1 and sEng plays a central role in the pathogenesis of placental hypoxia, as both VEGF and PlGF are essential for fetal and placental angiogenesiss (Lam et al., 2005; Sison et al., 2010; Bertuccio et al., 2011; Powe et al., 2011; Rana et al., 2012; Veron et al., 2012). Excessive production of antiangiogenic sFlt-1 and sEng reduces the bioavailability of free pro-angiogenic PlGF and VEGF, by binding and neutralizes VEGF and PlGF, thus reducing the availability of free VEGF for fetal and placental angiogenesis. In comparison, the sEng is implicated in neutralizing TGF- β, an anti-inflammatory growth factor (Roberts et al., 1989) that activates eNOS (Phipps et al., 2016). This imbalance leads to systemic endothelial dysfunction, including in the kidney (Kaufmann et al., 2003; Venkatesha et al., 2006), where disruption of slit diaphragm was reported (Garovic et al., 2007a; Henao et al., 2008; Zhao et al., 2009), as VEGF is essential for the maintenance glomerular barrier (Baelde et al., 2007). Support for the adverse effect of preeclampsia on glomerular barrier was reported by Henao et al. (2008). These authors demonstrated that when a human podocyte cell line was stimulated with serum from women with preeclampsia, disruption of CD2AP, podocin and actin were observed, but not when sera from normal pregnancy was added. Furthermore, the mean resistance value of podocytes cultured with serum from women with preeclampsia was significantly lower than podocytes cultured with serum from controls. This effect is mediated by ET-1 release by endothelial glomerular cells as preeclamptic sera induce nephrin shedding from podocytes (Romero et al., 2008). In this context, Elevated levels of ET-1, autoantibodies to the angiotensin II type I receptor, tumor necrosis factor α (TNFα) and interleukin-6 (IL-6) are also elevated in pre-eclampsia (Taylor et al., 1990; Mastrogiannis et al., 1991; Benigni et al., 1992; Granger et al., 2006; LaMarca B. et al., 2008; LaMarca B.D. et al., 2008; George and Granger, 2011, 2012; George et al., 2012).
Preeclampsia is commonly (but not always) accompanied by new onset proteinuria (>300 mg/d) or worsening proteinuria diagnosed after 20 weeks of pregnancy and generalized edema. The latter is mainly due to primary renal retention of salt and water despite the suppression of RAAS during preeclampsia due to vasoconstriction, in contrast to its upregulation in normal pregnancy, which is characterized by vasodilation (Schrier, 1988). Thus, the edema-accompanied preeclampsia resembles the “over-fill” edematous clinical settings. Another abnormal laboratory tests include elevated levels of creatinine, urea, uric acid levels along hypocalciuria, decreased urate excretion, and proteinuria.
Novel Mechanisms Based Therapeutics
Despite the rapid progress in understanding the mechanisms underlying the pathogenesis of preeclampsia, the treatment options remained very limited, except for early delivery. The current treatment options such as low Na+ diet, diuretics, Ca++ supplementation, Vitamin C and E were ineffective in most cases (Jim and Karumanchi, 2017). Aspirin moderately reduced the incidence of preterm preeclampsia in high-risk patients when given the drug at 11–14 weeks of gestation until 36 weeks (Rolnik et al., 2017). Therefore, there is unmet need for novel therapies to treat preeclampsia. Fortunately, as a token for unraveling the role of soluble vascular factors in preeclampsia, several new therapeutics have been developed that target implicated circulating angiogenic factors, including sFlt-1 (Figure 5; Sircar et al., 2015; Jim and Karumanchi, 2017). Specifically, these strategies rely on correcting the angiogenic balance, either by promoting proangiogenic factors or by blocking those of antiangiogenic properties. As outlined above, sFlt 1 is involved in the hemodynamic and pathophysiologic changes characterizing preeclampsia such as hypertension, renal dysfunction and shallow placentation (as the case in early-onset preeclampsia). Thus, elimination or reduction of the circulating levels of this deleterious anti-angiogenic factor below critical levels is supposed to ameliorate the angiogenic imbalance. Restoring angiogenic balance eventually improves the clinical signs of preeclampsia as has been confirmed in clinical and experimental models of the disease (Bergmann et al., 2010). In line with this assumption, an early study in five women with severe, early onset preeclampsia has demonstrated that negatively charged dextran sulfate cellulose column apheresis significantly decreased the plasma levels of sFlt-1 and attenuate the deleterious manifestations of the disease, including BP and proteinuria (Thadhani et al., 2011). Interestingly, pregnancy was prolonged by 15–23 days in these women without substantial side effects on the mother or fetus. In agreement with these results, a recent study has demonstrated that sFlt-1 removal by more efficient extracorporeal removal approach in 11 women who suffered from severe, early preeclampsia reduced proteinuria and prolonged pregnancy by 2–21 days depending on the number of courses underwent by the women, without causing major adverse maternal or fetal consequences (Figure 5; Thadhani et al., 2016). Additional approach for the reduction of sFlt-1 applied proton pump inhibitors (PPIs) in experimental model of the disease, namely placental sFlt-1 transgenic mice and in vitro human primary placental tissue and HUVEC (Onda et al., 2017). Proton pump inhibitors (PPIs) decrease sFlt-1 and sEng secretion, attenuate endothelial dysfunction, dilate blood vessels, decrease BP, and exert antioxidant and anti-inflammatory effects. The authors concluded that PPIs have therapeutic potential for preeclampsia and other diseases characterized by endothelial dysfunction (Onda et al., 2017). At the clinical level, PPI used by pregnant women (430 in number) was associated with decrease in sFlt-1 (Saleh et al., 2017). Moreover, their plasma endoglin and ET-1 levels were lower while sFlt-1 levels correlated positively with both. These findings suggest that PPI may bear therapeutic potential for preeclampsia, although prospective trials are still warranted.
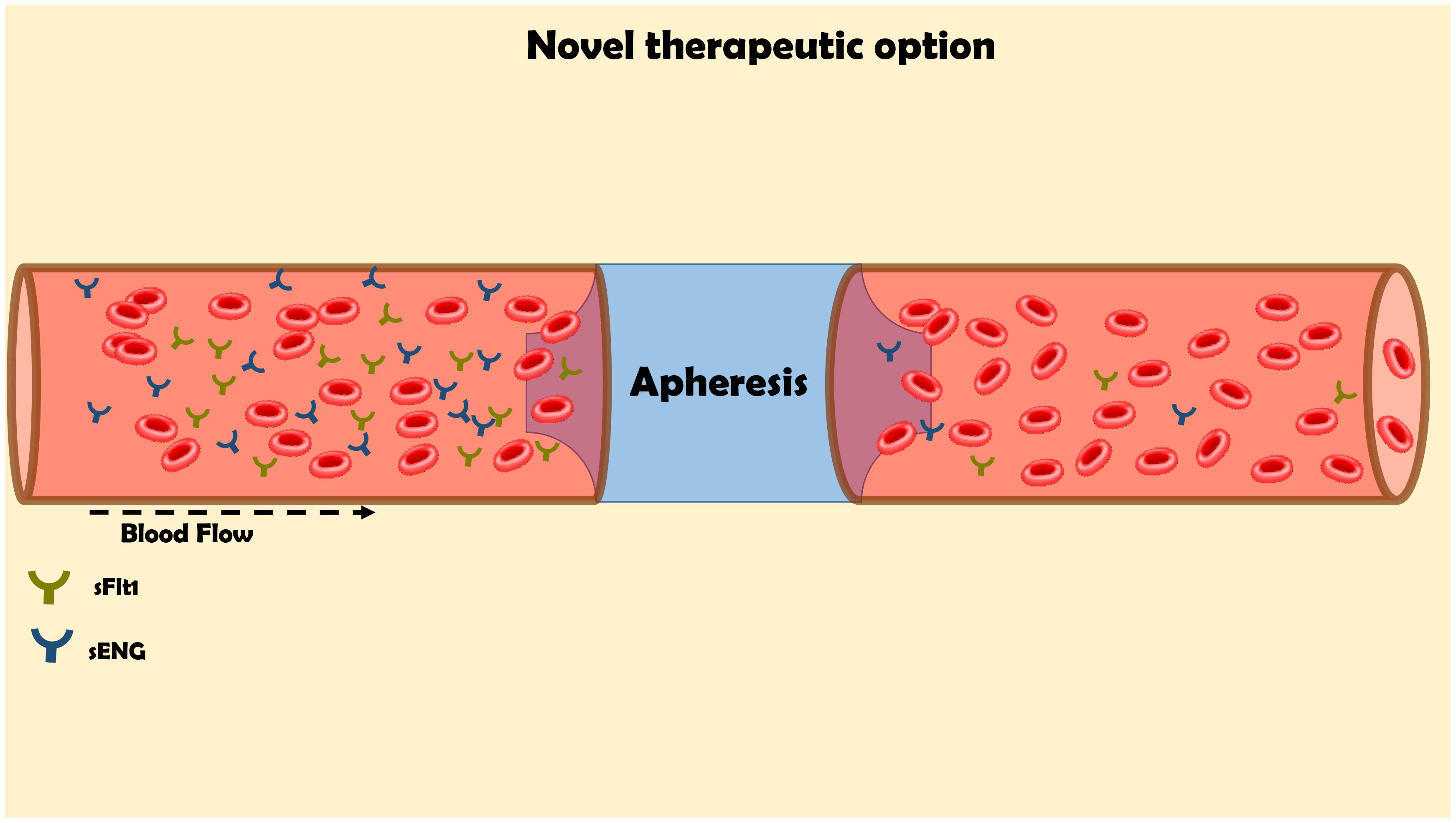
FIGURE 5. Innovative approach for the elimination of the elevated levels of sFlt-1 by apheresis for the management of preeclampsia. The maternal blood flow through columns, which contains antibodies against sFlt1 in order to remove it from the circulation.
In agreement with their physiological role, replenishing the circulatory levels of VEGF or PlGF exerted beneficial effects in experimental preeclampsia. Specifically, recombinant human PlGF supplementation restores the imbalance and abolished hypertension and GFR impairment in a rat preeclampsia model induced by reduced uterine perfusion pressure (RUPP) (Spradley et al., 2016). Since 3-hydroxy-3-methyl-glutaryl-coenzyme A reductase inhibitors (statins) have been shown to ameliorate the signs of experimental preeclampsia via upregulation of PlGF expression (Kumasawa et al., 2011), their efficacy and safety for prevention of preeclampsia are currently being tested in two clinical trials (Ahmed, 2011; Costantine and Cleary, 2013). Additional alternative to these approaches is administration of VEGF 121, which was shown to alleviate symptoms of preeclampsia including hypertension and renal injury (proteinuria, glomerular endotheliosis) in experimental model of the disease (Li et al., 2007). Co-administration of adenovirus and VEGF in an sFlt-1-induced model of preeclampsia rescued endothelial dysfunction along reduction of free circulatory sFlt-1 by ∼70% (Bergmann et al., 2010). Similarly, chronic infusion of VEGF121 via osmotic minipumps during late gestation reduced sFlt-1, restores GFR and endothelial function, and reduces high BP in experimental model of placental ischemia (Li et al., 2007). Interestingly, in all the above-mentioned studies administration of VEGF to sFlt-1 transgenic animals caused reduction of sFlt-1 along improvement of endothelial function (Bergmann et al., 2010). Although these results suggest that VEGF121 may be a candidate molecule for management of preeclampsia and its related complications, it should be emphasized that such approach may increase the fetal weight as the case in diabetic women, and may cause undesirable side effects such as edema due to its unselective binding to both VEGFR-1 and VEGFR-2.
Although, experimental studies in animal models of preeclampsia have shown that endothelin receptor blockers prevent the development of the disease (Saleh et al., 2016; Bakrania et al., 2017), clinical trials are still needed to validate these promising findings. Moreover, it should be emphasized that rebalancing the angiogenic ratios will improve the peripheral manifestations of the syndrome, but do not impact the underlying pathology of the early-onset cases, such as the acute atherotic changes in the spiral arteries. Hence, there a is danger of increasing stillbirths through prolonging a pregnancy in which uteroplacental perfusion is impaired.
Summary
Preeclampsia is a multifactorial clinical state that adversely affects almost all vital organs of pregnant women. After a half century of stumbling in understanding the molecular basis of the disease, the last decade has witnessed great advancement in the research of preeclampsia as evident by the discovery of wide battery of novel biomarkers that allow early diagnosis of the disease and prediction of the outcome.
In early-onset, pre-eclampsia there is clear evidence of reduced maternal spiral artery conversion in early pregnancy due to deficient trophoblast invasion and arterial remodeling, resulting in aberrant maternal–fetal interactions during early pregnancy and placental malperfusion. Oxidative stress of the placenta causes the increased secretion of sFLT-1 and reduced PlGF, and so explains the biomarker patterns. The abnormal angiogenic ratios are subsequent to the impaired placentation, and not the cause of it, as there is no evidence that sFLT affects trophoblast invasion. In contrast, in late-onset pre-eclampsia there is slight reduction in arterial conversion and the placental perfusion is maintained or even increased. Therefore, there is only minimal placental stress and sFLT and PLGF secretion by the placenta are close to normal range. These cases, which represent the overwhelming majority of pre-eclampsia, are now thought to be due to a genetic maternal pre-disposition to cardiovascular disease, which manifests as pre-eclampsia in response to pregnancy-induced stress.
Thus, sFlt-1 and sEng do not serve solely as biomarkers, rather they are responsible for the angiogenic imbalance and generalized endothelial dysfunction characterizing preeclampsia. The new insights into the pathogenesis of this clinical condition will provide great opportunity to improve the care of preeclamptic women before delivery and undoubtedly will lead 1 day to the development of novel strategies for prevention and treatment of the disease. Pipeline clinical trials based on elimination of serum sFlt-1 by means of apheresis yielded promising results indicating that the remedy for this prevalent dangerous entity is within reach (Nakakita et al., 2015; Easterling, 2016).
Author Contributions
ZA writing the section that deals with the renal aspect of preeclampsia and organizing all sections. JJ writing the introduction and organizing the MS. AJ writing the biochemical biomarkers of preeclampsia. ZAA writing the vascular aspects of preeclampsia and the molecular base underlying the pathogenesis of the diseases, editing the whole MS.
Conflict of Interest Statement
The authors declare that the research was conducted in the absence of any commercial or financial relationships that could be construed as a potential conflict of interest.
Acknowledgments
The authors are grateful for Safa Kinaneh, M.Sc. for her technical assistance.
References
ACOG practice bulletin (2002). Diagnosis and management of preeclampsia and eclampsia. Number 33, January. American College of Obstetricians and Gynecologists. Int. J. Gynaecol. Obstet. 77, 67–75.
Ahmad, S., and Ahmed, A. (2004). Elevated placental soluble vascular endothelial growth factor receptor-1 inhibits angiogenesis in preeclampsia. Circ. Res. 95, 884–891. doi: 10.1161/01.RES.0000147365.86159.f5
Ahmad, S., Hewett, P. W., Wang, P., Al-Ani, B., Cudmore, M., Fujisawa, T., et al. (2006). Direct evidence for endothelial vascular endothelial growth factor receptor-1 function in nitric oxide-mediated angiogenesis. Circ. Res. 99, 715–722. doi: 10.1161/01.RES.0000243989.46006.b9
Ahmed, A. (1997). Heparin-binding angiogenic growth factors in pregnancy: a review. Placenta 18, 215–258. doi: 10.1016/S0143-4004(97)80091-4
Ahmed, A. (2011). New insights into the etiology of preeclampsia: identification of key elusive factors for the vascular complications. Thromb. Res. 127(Suppl. 3), S72–S75. doi: 10.1016/S0049-3848(11)70020-2
Aita, K., Etoh, M., Hamada, H., Yokoyama, C., Takahashi, A., Suzuki, T., et al. (2009). Acute and transient podocyte loss and proteinuria in preeclampsia. Nephron Clin. Pract. 112, c65–c70. doi: 10.1159/000213083
Akolekar, R., Syngelaki, A., Sarquis, R., Zvanca, M., and Nicolaides, K. H. (2011). Prediction of early, intermediate and late pre-eclampsia from maternal factors, biophysical and biochemical markers at 11-13 weeks. Prenat. Diagn. 31, 832–832. doi: 10.1002/pd.2828
Al-Jameil, N., Aziz Khan, F., Fareed Khan, M., and Tabassum, H. (2014). A brief overview of preeclampsia. J. Clin. Med. Res. 6, 1–7.
Backes, C. H., Markham, K., Moorehead, P., Cordero, L., Nankervis, C. A., and Giannone, P. J. (2011). Maternal preeclampsia and neonatal outcomes. J. Pregnancy 2011:214365. doi: 10.1155/2011/214365
Baelde, H. J., Eikmans, M., Lappin, D. W. P., Doran, P. P., Hohenadel, D., Brinkkoetter, P. T., et al. (2007). Reduction of VEGF-A and CTGF expression in diabetic nephropathy is associated with podocyte loss. Kidney Int. 71, 637–645. doi: 10.1038/sj.ki.5002101
Bakrania, B., Duncan, J., Warrington, J. P., and Granger, J. P. (2017). The endothelin type a receptor as a potential therapeutic target in preeclampsia. Int. J. Mol. Sci. 18:E522. doi: 10.3390/ijms18030522
Baltajian, K., Bajracharya, S., Salahuddin, S., Berg, A. H., Geahchan, C., Wenger, J. B., et al. (2016). Sequential plasma angiogenic factors levels in women with suspected preeclampsia. Am. J. Obstet. Gynecol. 215, 89.e1–89.e10. doi: 10.1016/j.ajog.2016.01.168
Benigni, A., Orisio, S., Gaspari, F., Frusca, T., Amuso, G., and Remuzzi, G. (1992). Evidence against a pathogenetic role for endothelin in pre-eclampsia. Br. J. Obstet. Gynaecol. 99, 798–802. doi: 10.1111/j.1471-0528.1992.tb14409.x
Berg, C. J., Atrash, H. K., Koonin, L. M., Tucker M. (1996). Pregnancy-related mortality in the United States, 1987-1990. Obstet. Gynecol. 88, 161–167. doi: 10.1016/0029-7844(96)00135-4
Bergmann, A., Ahmad, S., Cudmore, M., Gruber, A. D., Wittschen, P., Lindenmaier, W., et al. (2010). Reduction of circulating soluble Flt-1 alleviates preeclampsia-like symptoms in a mouse model. J. Cell Mol. Med. 14, 1857–1867. doi: 10.1111/j.1582-4934.2009.00820.x
Bertuccio, C., Veron, D., Aggarwal, P. K., Holzman, L., and Tufro, A. (2011). Vascular endothelial growth factor receptor 2 direct interaction with nephrin links VEGF-A signals to actin in kidney podocytes. J. Biol. Chem. 286, 39933–39944. doi: 10.1074/jbc.M111.241620
Bourdeau, A., Dumont, D. J., and Letarte, M. (1999). A murine model of hereditary hemorrhagic telangiectasia. J. Clin. Invest. 104, 1343–1351. doi: 10.1172/JCI8088
Brosens, I. A., Robertson, W. B., and Dixon, H. G. (1972). The role of the spiral arteries in the pathogenesis of preeclampsia. Obstet. Gynecol. Annu. 1, 177–191.
Burke, S. D., Zsengeller, Z. K., Khankin, E. V., Lo, A. S., Rajakumar, A., DuPont, J. J., et al. (2016). Soluble fms-like tyrosine kinase 1 promotes angiotensin II sensitivity in preeclampsia. J. Clin. Invest. 126, 2561–2574. doi: 10.1172/JCI83918
Burton, G. J., Woods, A. W., Jauniaux, E., and Kingdom, J. C. (2009). Rheological and physiological consequences of conversion of the maternal spiral arteries for uteroplacental blood flow during human pregnancy. Placenta 30, 473–482. doi: 10.1016/j.placenta.2009.02.009
Chen, G., Zhang, L., Jin, X., Zhou, Y., Niu, J., Chen, J., et al. (2013). Effects of angiogenic factors, antagonists, and podocyte injury on development of proteinuria in preeclampsia. Reprod. Sci. 20, 579–588. doi: 10.1177/1933719112459227
Chen, Y. (2009). Novel angiogenic factors for predicting preeclampsia: sFlt-1, PlGF, and soluble endoglin. Open Clin. Chem. J. 2, 1–6. doi: 10.2174/1874241600902010001
Cindrova-Davies, T. (2009). Gabor than award lecture 2008: pre-eclampsia - from placental oxidative stress to maternal endothelial dysfunction. Placenta 30(Suppl. A), S55–S65. doi: 10.1016/j.placenta.2008.11.020
Cindrova-Davies, T., Sanders, D. A., Burton, G. J., and Charnock-Jones, D. S. (2011). Soluble FLT1 sensitizes endothelial cells to inflammatory cytokines by antagonizing VEGF receptor-mediated signalling. Cardiovasc. Res. 89, 671–679. doi: 10.1093/cvr/cvq346
Clowse, M. E. B., Jamison, M., Myers, E., and James, A. H. (2008). A national study of the complications of lupus in pregnancy. Am. J. Obstet. Gynecol. 199, 127.e1–127.e6. doi: 10.1016/j.ajog.2008.03.012
Collino, F., Bussolati, B., Gerbaudo, E., Marozio, L., Pelissetto, S., Benedetto, C., et al. (2008). Preeclamptic sera induce nephrin shedding from podocytes through endothelin-1 release by endothelial glomerular cells. Am. J. Physiol. Renal Physiol. 294, F1185–F1194. doi: 10.1152/ajprenal.00442.2007
Coonrod, D. V., Hickok, D. E., Zhu, K., Easterling, T. R., and Daling, J. R. (1995). Risk factors for preeclampsia in twin pregnancies: a population-based cohort study. Obstet. Gynecol. 85(5 Pt 1):645–650. doi: 10.1016/0029-7844(95)00049-W
Costantine, M. M., and Cleary, K. (2013). Pravastatin for the prevention of preeclampsia in high-risk pregnant women. Obstet. Gynecol. 121(2 Pt 1), 349–353. doi: 10.1097/AOG.0b013e31827d8ad5
Craici, I. M., Wagner, S. J., Bailey, K. R., Fitz-Gibbon, P. D., Wood-Wentz, C. M., Turner, S. T., et al. (2013). Podocyturia predates proteinuria and clinical features of preeclampsia: longitudinal prospective study. Hypertension 61, 1289–1296. doi: 10.1161/HYPERTENSIONAHA.113.01115
Cudmore, M., Ahmad, S., Al-Ani, B., Fujisawa, T., Coxall, H., Chudasama, K., et al. (2007). Negative regulation of soluble Flt-1 and soluble endoglin release by heme oxygenase-1. Circulation 115, 1789–1797. doi: 10.1161/CIRCULATIONAHA.106.660134
Davenport, A. P., Hyndman, K. A., Dhaun, N., Southan, C., Kohan, D. E., Pollock, J. S., et al. (2016). Endothelin. Pharmacol. Rev. 68, 357–418. doi: 10.1124/pr.115.011833
De Falco, S. (2012). The discovery of placenta growth factor and its biological activity. Exp. Mol. Med. 44, 1–9. doi: 10.3858/emm.2012.44.1.025
Duley, L. (2003). Pre-eclampsia and the hypertensive disorders of pregnancy. Br. Med. Bull. 67, 161–176. doi: 10.1093/bmb/ldg005
Easterling, T. R. (2016). Apheresis to treat preeclampsia: insights, opportunities and challenges. J. Am. Soc. Nephrol. 27, 663–665. doi: 10.1681/ASN.2015070794
Elvan-Taspinar, A., Franx, A., Delprat, C. C., Bruinse, H. W., and Koomans, H. A. (2006). Water immersion in preeclampsia. Am. J. Obstet. Gynecol. 195, 1590–1595. doi: 10.1016/j.ajog.2006.05.007
Eremina, V., Jefferson, J. A., Kowalewska, J., Hochster, H., Haas, M., Weisstuch, J., et al. (2008). VEGF inhibition and renal thrombotic microangiopathy. N. Engl. J. Med. 358, 1129–1136. doi: 10.1056/NEJMoa0707330
Facca, T. A., Kirsztajn, G. M., Pereira, A. R., Moreira, S. R., Teixeira, V. P., Nishida, S. K., et al. (2012). Renal evaluation in women with preeclampsia. Nephron Extra 2, 125–132. doi: 10.1159/000338271
Ferrara, N. (2004). Vascular endothelial growth factor: basic science and clinical progress. Endocr. Rev. 25, 581–611. doi: 10.1210/er.2003-0027
Fischer, T., Schneider, M. P., Schobel, H. P., Heusser, K., Langenfeld, M., and Schmieder, R. E. (2000). Vascular reactivity in patients with preeclampsia and HELLP (hemolysis, elevated liver enzymes, and low platelet count) syndrome. Am. J. Obstet. Gynecol. 183, 1489–1494. doi: 10.1067/mob.2000.107323
Fulton, D., Gratton, J. P., McCabe, T. J., Fontana, J., Fujio, Y., Walsh, K., et al. (1999). Regulation of endothelium-derived nitric oxide production by the protein kinase Akt. Nature 399, 597–601. doi: 10.1038/21218
Gant, N. F., Daley, G. L., Chand, S., Whalley, P. J., and MacDonald, P. C. (1973). A study of angiotensin II pressor response throughout primigravid pregnancy. J. Clin. Invest. 52, 2682–2689. doi: 10.1172/JCI107462
Garovic, V. D., Craici, I. M., Wagner, S. J., White, W. M., Brost, B. C., Rose, C. H., et al. (2013). Mass spectrometry as a novel method for detection of podocyturia in pre-eclampsia. Nephrol. Dial. Transplant. 28, 1555–1561. doi: 10.1093/ndt/gfs074
Garovic, V. D., Wagner, S. J., Petrovic, L. M., Gray, C. E., Hall, P., Sugimoto, H., et al. (2007a). Glomerular expression of nephrin and synaptopodin, but not podocin, is decreased in kidney sections from women with preeclampsia. Nephrol. Dial. Transplant. 22, 1136–1143. doi: 10.1093/ndt/gfl711
Garovic, V. D., Wagner, S. J., Turner, S. T., Rosenthal, D. W., Watson, W. J., Brost, B. C., et al. (2007b). Urinary podocyte excretion as a marker for preeclampsia. Am. J. Obstet. Gynecol. 196, 320.e1–320.e7. doi: 10.1016/j.ajog.2007.02.007
Gartner, H. V., Sammoun, A., Wehrmann, M., Grossmann, T., Junghans, R., and Weihing, C. (1998). Preeclamptic nephropathy – an endothelial lesion. A morphological study with a review of the literature. Eur. J. Obstet. Gynecol. Reprod. Biol. 77, 11–27. doi: 10.1016/S0301-2115(97)00219-4
Genbacev, O., Zhou, Y., Ludlow, J. W., and Fisher, S. J. (1997). Regulation of human placental development by oxygen tension. Science 277, 1669–1672. doi: 10.1126/science.277.5332.1669
George, E. M., and Granger, J. P. (2010). Recent insights into the pathophysiology of preeclampsia. Expert Rev. Obstet. Gynecol. 5, 557–566. doi: 10.1586/eog.10.45
George, E. M., and Granger, J. P. (2011). Endothelin: key mediator of hypertension in preeclampsia. Am. J. Hypertens. 24, 964–969. doi: 10.1038/ajh.2011.99
George, E. M., and Granger, J. P. (2012). Linking placental ischemia and hypertension in preeclampsia: role of endothelin 1. Hypertension 60, 507–511. doi: 10.1161/HYPERTENSIONAHA.112.194845
George, E. M., Palei, A. C., and Granger, J. P. (2012). Endothelin as a final common pathway in the pathophysiology of preeclampsia: therapeutic implications. Curr. Opin. Nephrol. Hypertens. 21, 157–162. doi: 10.1097/MNH.0b013e328350094b
Geusens, N., Verlohren, S., Luyten, C., Taube, M., Hering, L., Vercruysse, L., et al. (2008). Endovascular trophoblast invasion, spiral artery remodelling and uteroplacental haemodynamics in a transgenic rat model of pre-eclampsia. Placenta 29, 614–623. doi: 10.1016/j.placenta.2008.04.005
Ghulmiyyah, L., and Sibai, B. (2012). Maternal mortality from preeclampsia/eclampsia. Semin. Perinatol. 36, 56–59. doi: 10.1053/j.semperi.2011.09.011
Gougos, A., and Letarte, M. (1990). Primary structure of endoglin, an RGD-containing glycoprotein of human endothelial cells. J. Biol. Chem. 265, 8361–8364.
Granger, J. P., Abram, S., Stec, D., Chandler, D., Speed, J., and LaMarca, B. (2006). Endothelin, the kidney, and hypertension. Curr. Hypertens. Rep. 8, 298–303. doi: 10.1007/s11906-006-0068-x
Gregory, A. L., Xu, G., Sotov, V., and Letarte, M. (2014). Review: the enigmatic role of endoglin in the placenta. Placenta 35, S93–S99. doi: 10.1016/j.placenta.2013.10.020
Hayman, S. R., Calle, J. C., Jatoi, A., Craici, I. M., Wagner, S. J., Weaver, A. L., et al. (2014). Urinary podocyte excretion and proteinuria in patients treated with antivascular endothelial growth factor therapy for solid tumor malignancies. Oncology 86, 271–278. doi: 10.1159/000360180
Henao, D. E., Arias, L. F., Mathieson, P. W., Ni, L., Welsh, G. I., Bueno, J. C., et al. (2008). Preeclamptic sera directly induce slit-diaphragm protein redistribution and alter podocyte barrier-forming capacity. Nephron Exp. Nephrol. 110, e73–e81. doi: 10.1159/000166993
Hussein, W., and Lafayette, R. A. (2014). Renal function in normal and disordered pregnancy. Curr. Opin. Nephrol. Hypertens. 23, 46–53. doi: 10.1097/01.mnh.0000436545.94132.52
Iwasaki, H., Kawamoto, A., Tjwa, M., Horii, M., Hayashi, S., Oyamada, A., et al. (2011). PlGF repairs myocardial ischemia through mechanisms of angiogenesis, cardioprotection and recruitment of myo-angiogenic competent marrow progenitors. PLoS One 6:e24872. doi: 10.1371/journal.pone.0024872
Jim, B., Jean-Louis, P., Qipo, A., Garry, D Mian, S., and Matos, T. (2012). Podocyturia as a diagnostic marker for preeclampsia amongst high-risk pregnant patients. J. Pregnancy 2012:984630. doi: 10.1155/2012/984630
Jim, B., and Karumanchi, S. A. (2017). Preeclampsia: pathogenesis, prevention, and long-term complications. Semin. Nephrol. 37, 386–397. doi: 10.1016/j.semnephrol.2017.05.011
Kabbinavar, F., Hurwitz, H. I., Fehrenbacher, L., Meropol, N. J., Novotny, W. F., Lieberman, G., et al. (2003). Phase II, randomized trial comparing bevacizumab plus fluorouracil (FU)/leucovorin (LV) with FU/LV alone in patients with metastatic colorectal cancer. J. Clin. Oncol. 21, 60–65. doi: 10.1200/JCO.2003.10.066
Kanasaki, K., Palmsten, K., Sugimoto, H., Ahmad, S., Hamano, Y., Xie, L., et al. (2008). Deficiency in catechol-O-methyltransferase and 2-methoxyoestradiol is associated with pre-eclampsia. Nature 453, 1117–1121. doi: 10.1038/nature06951
Kar, M. (2014). Role of biomarkers in early detection of preeclampsia. J. Clin. Diagn. Res. 8, BE01–BE04. doi: 10.7860/JCDR/2014/7969.4261
Kattah, A. G., Asad, R., Scantlebury, D. C., Bailey, K. R., Wiste, H. J., Hunt, S. C., et al. (2013). Hypertension in pregnancy is a risk factor for microalbuminuria later in life. J. Clin. Hypertens. 15, 617–623. doi: 10.1111/jch.12116
Kaufmann, P., Black, S., and Huppertz, B. (2003). Endovascular trophoblast invasion: implications for the pathogenesis of intrauterine growth retardation and preeclampsia. Biol. Reprod. 69, 1–7. doi: 10.1095/biolreprod.102.014977
Kelder, T. P., Penning, M. E., Uh, H. W., Cohen, D., Bloemenkamp, K. W. M., Bruijn, J. A., et al. (2012). Quantitative polymerase chain reaction-based analysis of podocyturia is a feasible diagnostic tool in preeclampsia. Hypertension 60, 1538–1544. doi: 10.1161/HYPERTENSIONAHA.112.201681
Kendall, R. L., and Thomas, K. A. (1993). Inhibition of vascular endothelial cell growth factor activity by an endogenously encoded soluble receptor. Proc. Natl. Acad. Sci. U.S.A. 90, 10705–10709. doi: 10.1073/pnas.90.22.10705
Kestila, M., Lenkkeri, U., Mannikko, M., Lamerdin, J., McCready, P., Putaala, H., et al. (1998). Positionally cloned gene for a novel glomerular protein - nephrin - is mutated in congenital nephrotic syndrome. Mol. Cell 1, 575–582. doi: 10.1016/S1097-2765(00)80057-X
Kumasawa, K., Ikawa, M., Kidoya, H., Hasuwa, H., Saito-Fujita, T., Morioka, Y., et al. (2011). Pravastatin induces placental growth factor (PGF) and ameliorates preeclampsia in a mouse model. Proc. Natl. Acad. Sci. U.S.A. 108, 1451–1455. doi: 10.1073/pnas.1011293108
Lam, C., Lim, K. H., and Karumanchi, S. A. (2005). Circulating angiogenic factors in the pathogenesis and prediction of preeclampsia. Hypertension 46, 1077–1085. doi: 10.1161/01.HYP.0000187899.34379.b0
LaMarca, B., Wallukat, G., Llinas, M., Herse, F., Dechend, R., and Granger, J. P. (2008). Autoantibodies to the angiotensin type I receptor in response to placental ischemia and tumor necrosis factor alpha in pregnant rats. Hypertension 52, 1168–1172. doi: 10.1161/HYPERTENSIONAHA.108.120576
LaMarca, B. D., Gilbert, J., and Granger, J. P. (2008). Recent progress toward the understanding of the pathophysiology of hypertension during preeclampsia. Hypertension 51, 982–988. doi: 10.1161/HYPERTENSIONAHA.107.108837
Levine, R. J., Maynard, S. E., Qian, C., Lim, K. H., England, L. J., Yu, K. F., et al. (2004). Circulating angiogenic factors and the risk of preeclampsia. N. Engl. J. Med. 350, 672–683. doi: 10.1056/NEJMoa031884
Li, H., Gu, B., Zhang, Y., Lewis, D. F., and Wang, Y. (2005). Hypoxia-induced increase in soluble Flt-1 production correlates with enhanced oxidative stress in trophoblast cells from the human placenta. Placenta 26, 210–217. doi: 10.1016/j.placenta.2004.05.004
Li, Z., Zhang, Y., Ying Ma, J., Kapoun, A. M., Shao, Q., Kerr, I., et al. (2007). Recombinant vascular endothelial growth factor 121 attenuates hypertension and improves kidney damage in a rat model of preeclampsia. Hypertension 50, 686–692. doi: 10.1161/HYPERTENSIONAHA.107.092098
Lisonkova, S., Sabr, Y., Mayer, C., Young, C., Skoll, A., and Joseph, K. S. (2014). Maternal morbidity associated with early-onset and late-onset preeclampsia. Obstet. Gynecol. 124, 771–781. doi: 10.1097/AOG.0000000000000472
Llurba, E., Crispi, F., and Verlohren, S. (2015). Update on the pathophysiological implications and clinical role of angiogenic factors in pregnancy. Fetal Diagn. Ther. 37, 81–92. doi: 10.1159/000368605
Lyall, F. (2005). Priming and remodelling of human placental bed spiral arteries during pregnancy–a review. Placenta 26(Suppl. A), S31–S36. doi: 10.1016/j.placenta.2005.02.010
Maglione, D., Guerriero, V., Viglietto, G., Delli-Bovi, P., and Persico, M. G. (1991). Isolation of a human placenta cDNA coding for a protein related to the vascular permeability factor. Proc. Natl. Acad. Sci. U.S.A. 88, 9267–9271. doi: 10.1073/pnas.88.20.9267
March, M. I., Geahchan, C., Wenger, J., Raghuraman, N., Berg, A., Haddow, H., et al. (2015). Circulating angiogenic factors and the risk of adverse outcomes among haitian women with preeclampsia. PLoS One 10:e0126815. doi: 10.1371/journal.pone.0126815
Mastrogiannis, D. S., O’Brien, W. F., Krammer, J., and Benoit, R. (1991). Potential role of endothelin-1 in normal and hypertensive pregnancies. Am. J. Obstet. Gynecol. 165(6 Pt 1), 1711–1716. doi: 10.1016/0002-9378(91)90020-R
Matsubara, S., Bourdeau, A., terBrugge, K. G., Wallace, C., and Letarte, M. (2000). Analysis of endoglin expression in normal brain tissue and in cerebral arteriovenous malformations. Stroke 31, 2653–2660. doi: 10.1161/01.STR.31.11.2653
Maynard, S. E., Min, J. Y., Merchan, J., Lim, K. H., Li, J., Mondal, S., et al. (2003). Excess placental soluble fms-like tyrosine kinase 1 (sFlt1) may contribute to endothelial dysfunction, hypertension, and proteinuria in preeclampsia. J. Clin. Invest. 111, 649–658. doi: 10.1172/JCI17189
McDonald, S. D., Han, Z., Walsh, M. W., Gerstein, H. C., and Devereaux, P. J. (2010). Kidney disease after preeclampsia: a systematic review and meta-analysis. Am. J. Kidney Dis. 55, 1026–1039. doi: 10.1053/j.ajkd.2009.12.036
McFarlane, A., and Scott, J. S. (1976). Pre-eclampsia/eclampsia in twin pregnancies. J. Med. Genet. 13, 208–211. doi: 10.1136/jmg.13.3.208
McKinney, E. T., Shouri, R., Hunt, R. S., Ahokas, R. A., and Sibai, B. M. (2000). Plasma, urinary, and salivary 8-epi-prostaglandin f2alpha levels in normotensive and preeclamptic pregnancies. Am. J. Obstet. Gynecol. 183, 874–877. doi: 10.1067/mob.2000.108877
Moffett, A., Hiby, S. E., and Sharkey, A. M. (2015). The role of the maternal immune system in the regulation of human birthweight. Philos. Trans. R. Soc. Lond. B Biol. Sci. 370:20140071. doi: 10.1098/rstb.2014.0071
Mongraw-Chaffin, M. L., Cirillo, P. M., and Cohn, B. A. (2010). Preeclampsia and cardiovascular disease death: prospective evidence from the child health and development studies cohort. Hypertension 56, 166–171. doi: 10.1161/HYPERTENSIONAHA.110.150078
Mostello, D., Catlin, T. K., Roman, L., Holcomb, W. L., and Leet, T. (2002). Preeclampsia in the parous woman: Who is at risk? Am. J. Obstet. Gynecol. 187, 425–429. doi: 10.1067/mob.2002.123608
Muller-Deile, J., and Schiffer, M. (2011). Renal involvement in preeclampsia: similarities to VEGF ablation therapy. J. Pregnancy 2011:176973. doi: 10.1155/2011/176973
Nakakita, B., Mogami, H., Kondoh, E., Tsukamoto, T., Yanagita, M., and Konishi, I. (2015). Case of soluble fms-like tyrosine kinase 1 apheresis in severe pre-eclampsia developed at 15 weeks’ gestation. J. Obstet. Gynaecol. Res. 41, 1661–1663. doi: 10.1111/jog.12760
Nevo, O., Soleymanlou, N., Wu, Y., Xu, J., Kingdom, J., Many, A., et al. (2006). Increased expression of sFlt-1 in in vivo and in vitro models of human placental hypoxia is mediated by HIF-1. Am. J. Physiol. Regul. Integr. Comp. Physiol. 291, R1085-R1093. doi: 10.1152/ajpregu.00794.2005
Norwitz, E. R., Schust, D. J., and Fisher, S. J. (2001) Implantation and the survival of early pregnancy. N. Engl. J. Med. 345, 1400–1408. doi: 10.1056/NEJMra000763
Onda, K., Tong, S., Beard, S., Binder, N., Muto, M., Senadheera, S. N., et al. (2017). Proton pump inhibitors decrease soluble fms-like tyrosine kinase-1 and soluble endoglin secretion, decrease hypertension, and rescue endothelial dysfunction. Hypertension, 69, 457–468. doi: 10.1161/HYPERTENSIONAHA.116.08408
Osol, G., and Mandala, M. (2009). Maternal uterine vascular remodeling during pregnancy. Physiology 24, 58–71. doi: 10.1152/physiol.00033.2008
Palomaki, G. E., Haddow, J. E., Haddow, H. R., Salahuddin, S., Geahchan, C., Cerdeira, A. S., et al. (2015). Modeling risk for severe adverse outcomes using angiogenic factor measurements in women with suspected preterm preeclampsia. Prenat. Diagn. 35, 386–393. doi: 10.1002/pd.4554
Phipps, E., Prasanna, D., Brima, W., and Jim, B. (2016). Preeclampsia: updates in pathogenesis, definitions, and guidelines. Clin. J. Am. Soc. Nephrol. 11, 1102–1113. doi: 10.2215/CJN.12081115
Pijnenborg, R., Vercruysse, L., and Hanssens, M. (2006). The uterine spiral arteries in human pregnancy: facts and controversies. Placenta 27, 939–958. doi: 10.1016/j.placenta.2005.12.006
Powe, C. E., Levine, R. J., and Karumanchi, S. A. (2011). Preeclampsia, a disease of the maternal endothelium: the role of antiangiogenic factors and implications for later cardiovascular disease. Circulation 123, 2856–2869. doi: 10.1161/CIRCULATIONAHA.109.853127
Qu, R., Silver, M. M., and Letarte, M. (1998). Distribution of endoglin in early human development reveals high levels on endocardial cushion tissue mesenchyme during valve formation. Cell Tissue Res. 292, 333–343. doi: 10.1007/s004410051064
Rana, S., Powe, C. E., Salahuddin, S., Verlohren, S., Perschel, F. H., Levine, R. J., et al. (2012). Angiogenic factors and the risk of adverse outcomes in women with suspected preeclampsia. Circulation 125, 911–919. doi: 10.1161/CIRCULATIONAHA.111.054361
Rana, S., Schnettler, W. T., Powe, C., Wenger, J., Salahuddin, S., Cerdeira, A. S., et al. (2013). Clinical characterization and outcomes of preeclampsia with normal angiogenic profile. Hypertens. Pregnancy 32, 189–201. doi: 10.3109/10641955.2013.784788
Red-Horse, K., Zhou, Y., Genbacev, O., Prakobphol, A., Foulk, R., McMaster, M., et al. (2004). Trophoblast differentiation during embryo implantation and formation of the maternal-fetal interface. J. Clin. Invest. 114, 744–754. doi: 10.1172/JCI200422991
Roberts, J. M., and Lain, K. Y. (2002). Recent Insights into the pathogenesis of pre-eclampsia. Placenta 23, 359–372. doi: 10.1053/plac.2002.0819
Roberts, J. M., Taylor, R. N., Musci, T. J., Rodgers, G. M., Hubel, C. A., and McLaughlin, M. K. (1989). Preeclampsia: an endothelial cell disorder. Am. J. Obstet. Gynecol. 161, 1200–1204. doi: 10.1016/0002-9378(89)90665-0
Robertson, A. K., Rudling, M., Zhou, X., Gorelik, L., and Flavell, R. A., Hansson, G. K. (2003). Disruption of TGF-beta signaling in T cells accelerates atherosclerosis. J. Clin. Invest. 112, 1342–1350. doi: 10.1172/JCI18607
Robinson, C. J., and Johnson, D. D. (2007). Soluble endoglin as a second-trimester marker for preeclampsia. Am. J. Obstet. Gynecol. 197, 174.e1–174.e5. doi: 10.1016/j.ajog.2007.03.058
Rolnik, D. L., Wright, D., Poon, L. C., O’Gorman, N., Syngelaki, A., Matallana, C. D., et al. (2017). Aspirin versus placebo in pregnancies at high risk for preterm preeclampsia. N. Engl. J. Med. 377, 613–622. doi: 10.1056/NEJMoa1704559
Romero, R., Nien, J. K., Espinoza, J., Todem, D., Fu, W., Chung, H., et al. (2008). A longitudinal study of angiogenic (placental growth factor) and anti-angiogenic (soluble endoglin and soluble vascular endothelial growth factor receptor-1) factors in normal pregnancy and patients destined to develop preeclampsia and deliver a small for gestational age neonate. J. Matern. Fetal Neonatal Med. 21, 9–23. doi: 10.1080/14767050701830480
Saleh, L., Samantar, R., Garrelds, I. M., van den Meiracker, A. H., Visser, W., and Danser, A. H. J. (2017). Low soluble fms-like tyrosine kinase-1, endoglin, and endothelin-1 levels in women with confirmed or suspected preeclampsia using proton pump inhibitors. Hypertension 70, 594–600. doi: 10.1161/HYPERTENSIONAHA.117.09741
Saleh, L., Verdonk, K., Visser, W., van den Meiracker, A. H., and Danser A H. (2016). The emerging role of endothelin-1 in the pathogenesis of pre-eclampsia. Ther. Adv. Cardiovasc. Dis. 10, 282–293. doi: 10.1177/1753944715624853
Santner-Nanan, B., Peek, M. J., Khanam, R., Richarts, L., Zhu, E., Fazekas de St Groth, B., and Nanan R. (2009). Systemic increase in the ratio between Foxp3+ and IL-17-producing CD4+ T cells in healthy pregnancy but not in preeclampsia. J. Immunol. 183, 7023–7030. doi: 10.4049/jimmunol.0901154
Schrier, R. W. (1988). Pathogenesis of sodium and water retention in high-output and low-output cardiac failure, nephrotic syndrome, cirrhosis, and pregnancy (2). N. Engl. J. Med. 319, 1127–1134. doi: 10.1056/NEJM198810273191705
Sibai, B. M., and Stella, C. L. (2009). Diagnosis and management of atypical preeclampsia-eclampsia. Am. J. Obstet. Gynecol. 200, 481.e1–481.e7. doi: 10.1016/j.ajog.2008.07.048
Sircar, M., Thadhani, R., and Karumanchi, S. A. (2015). Pathogenesis of preeclampsia. Curr. Opin. Nephrol. Hypertens. 24, 131–138. doi: 10.1097/MNH.0000000000000105
Sison, K., Eremina, V., Baelde, H., Min, W., Hirashima, M., Fantus, I. G., et al. (2010). Glomerular structure and function require paracrine, not autocrine, VEGF-VEGFR-2 signaling. J. Am. Soc. Nephrol. 21, 1691–1701. doi: 10.1681/ASN.2010030295
Skarzinski, G., Khamaisi, M., Bursztyn, M., Mekler, J., Lan, D., Evdokimov, P., et al. (2009). Intrauterine growth restriction and shallower implantation site in rats with maternal hyperinsulinemia are associated with altered NOS expression. Placenta 30, 898–906. doi: 10.1016/j.placenta.2009.07.014
Sohlberg, S., Mulic-Lutvica, A., Lindgren, P., Ortiz-Nieto, F., Wikstrom, A. K., and Wikstrom J. (2014). Placental perfusion in normal pregnancy and early and late preeclampsia: a magnetic resonance imaging study. Placenta 35, 202–206. doi: 10.1016/j.placenta.2014.01.008
Son, G. H., Kwon, J. Y., Lee, S., Park, J., Kim, Y. J., Yun, B., et al. (2013). Comparison of serum and urinary nephrin levels between normal pregnancies and severe preeclampsia. Eur. J. Obstet. Gynecol. Reprod. Biol. 166, 139–144. doi: 10.1016/j.ejogrb.2012.10.011
Sovio, U., Gaccioli, F., Cook, E., Hund, M., Charnock-Jones, D. S., and Smith, G. C. S. (2017). Prediction of preeclampsia using the soluble fms-like tyrosine kinase 1 to placental growth factor ratio: a prospective cohort study of unselected nulliparous women. Hypertension 69, 731–738. doi: 10.1161/HYPERTENSIONAHA.116.08620
Spargo, B., Mc, C. C., and Winemiller, R. (1959). Glomerular capillary endotheliosis in toxemia of pregnancy. Arch. Pathol. 68, 593–599.
Spradley, F. T., Tan, A. Y., Joo, W. S., Daniels, G., Kussie, P., Karumanchi, S. A., et al. (2016). Placental growth factor administration abolishes placental ischemia-induced hypertension. Hypertension 67, 740–747. doi: 10.1161/HYPERTENSIONAHA.115.06783
Staff, A. C., Benton, S. J., von Dadelszen, P., Roberts, J. M., Taylor, R. N., Powers, R. W., et al. (2013). Redefining preeclampsia using placenta-derived biomarkers. Hypertension 61, 932–942. doi: 10.1161/HYPERTENSIONAHA.111.00250
Takimoto, E., Ishida, J., Sugiyama, F., Horiguchi, H., Murakami, K., and Fukamizu, A. (1996). Hypertension induced in pregnant mice by placental renin and maternal angiotensinogen. Science 274, 995–998. doi: 10.1126/science.274.5289.995
Taylor, R. N., Grimwood, J., Taylor, R. S., McMaster, M. T., Fisher, S. J., and North, R. A. (2003). Longitudinal serum concentrations of placental growth factor: evidence for abnormal placental angiogenesis in pathologic pregnancies. Am. J. Obstet. Gynecol. 188, 177–182. doi: 10.1067/mob.2003.111
Taylor, R. N., Varma, M., Teng, N. N. H., and Roberts, J. M. (1990). Women with preeclampsia have higher plasma endothelin levels than women with normal pregnancies. J. Clin. Endocrinol. Metab. 71, 1675–1677. doi: 10.1210/jcem-71-6-1675
Thadhani, R., Hagmann, H., Schaarschmidt, W., Roth, B., Cingoez, T., Karunnanchi, S. A., et al. (2016). Removal of soluble fms-like tyrosine kinase-1 by dextran sulfate apheresis in preeclampsia. J. Am. Soc. Nephrol. 27, 903–913. doi: 10.1681/ASN.2015020157
Thadhani, R., Kisner, T., Hagmann, H., Bossung, V., Noack, S., Schaarschmidt, W., et al. (2011). Pilot study of extracorporeal removal of soluble fms-like tyrosine kinase 1 in preeclampsia. Circulation 124, 940–950. doi: 10.1161/CIRCULATIONAHA.111.034793
Tjoa, M. L., Levine, R. J., and Karumanchi, S. A. (2007). Angiogenic factors and preeclampsia. Front. Biosci. 12, 2395–2402. doi: 10.2741/2241
Venkatesha, S., Toporsian, M., Lam, C., Hanai, J., Mammoto, T., Kim, Y. M., et al. (2006). Soluble endoglin contributes to the pathogenesis of preeclampsia. Nat. Med. 12, 642–649. doi: 10.1038/nm1429
Verdonk, K., Saleh, L., Lankhorst, S., Smilde, J. E., van Ingen, M. M., Garrelds, I. M., et al. (2015). Association studies suggest a key role for endothelin-1 in the pathogenesis of preeclampsia and the accompanying renin-angiotensin-aldosterone system suppression. Hypertension 65, 1316–1323. doi: 10.1161/HYPERTENSIONAHA.115.05267
Veron, D., Villegas, G., Aggarwal, P. K., Bertuccio, C., Jimenez, J., Velazquez, H., et al. (2012). Acute podocyte vascular endothelial growth factor (VEGF-A) knockdown disrupts alphaVbeta3 integrin signaling in the glomerulus. PLoS One 7:e40589. doi: 10.1371/journal.pone.0040589
Vikse, B. E., Irgens, L. M., Karumanchi, S. A., Thadhani, R., Reisaeter, A. V., and Skjaerven, R. (2012). Familial factors in the association between preeclampsia and later ESRD. Clin. J. Am. Soc. Nephrol. 7, 1819–1826. doi: 10.2215/CJN.01820212
Vikse, B. E., Irgens, L. M., Leivestad, T., Skjaerven, R., and Iversen, B. M. (2008). Preeclampsia and the risk of end-stage renal disease. N. Engl. J. Med. 359, 800–809. doi: 10.1056/NEJMoa0706790
Wang, I. K., Muo, C. H., Chang, Y. C., Liang, C. C., Chang, C. T., Lin, S. Y., et al. (2013). Association between hypertensive disorders during pregnancy and end-stage renal disease: a population-based study. Can. Med. Assoc. J. 185, 207–213. doi: 10.1503/cmaj.120230
Wang, Y., Zhao, S., Loyd, S., and Groome, L. J. (2012). Increased urinary excretion of nephrin, podocalyxin, and betaig-h3 in women with preeclampsia. Am. J. Physiol. Renal Physiol. 302, F1084–F1089. doi: 10.1152/ajprenal.00597.2011
Yoshida, A., Nakao, S., Kobayashi, M., and Kobayashi, H. (2000). Flow-mediated vasodilation and plasma fibronectin levels in preeclampsia. Hypertension 36, 400–404. doi: 10.1161/01.HYP.36.3.400
Young, B. C., Levine, R. J., and Karumanchi, S. A. (2010). Pathogenesis of preeclampsia. Annu. Rev. Pathol. 5, 173–192. doi: 10.1146/annurev-pathol-121808-102149
Yung, H. W., Atkinson, D., Campion-Smith, T., Olovsson, M., Charnock-Jones, D. S., and Burton, G. J. (2005). Differential activation of placental unfolded protein response pathways implies heterogeneity in causation of early- and late-onset pre-eclampsia. J. Pathol. 234, 262–276.
Zeisler, H., Llurba, E., Chantraine, F., Vatish, M., Staff, A. C., Sennstrom, M., et al. (2016). Predictive value of the sFlt-1:PlGF ratio in women with suspected preeclampsia. N. Engl. J. Med. 374, 13–22. doi: 10.1056/NEJMoa1414838
Zhao, S., Gu, X., Groome, L. J., and Wang, Y. (2009). Decreased nephrin and GLEPP-1, but increased VEGF, Flt-1, and nitrotyrosine, expressions in kidney tissue sections from women with preeclampsia. Reprod. Sci. 16, 970–979. doi: 10.1177/1933719109338630
Zhao, S., Gu, Y., Coates, G., Groome, L. J., Saleem, M. A., Mathieson, P. W., et al. (2011). Altered nephrin and podoplanin distribution is associated with disturbed polarity protein PARD-3 and PARD-6 expressions in podocytes from preeclampsia. Reprod. Sci. 18, 772–780. doi: 10.1177/1933719111398145
Zhou, C. C., Irani, R. A., Zhang, Y., Blackwell, S. C., Mi, T., Wen, J., et al. (2010). Angiotensin receptor agonistic autoantibody-mediated tumor necrosis factor-alpha induction contributes to increased soluble endoglin production in preeclampsia. Circulation 121, 436–444. doi: 10.1161/CIRCULATIONAHA.109.902890
Keywords: preeclampsia, maternity, fetus, endothelium, kidney, placental growth factor (PlGF), soluble growth factor receptor-sFlt, endoglin
Citation: Armaly Z, Jadaon JE, Jabbour A and Abassi ZA (2018) Preeclampsia: Novel Mechanisms and Potential Therapeutic Approaches. Front. Physiol. 9:973. doi: 10.3389/fphys.2018.00973
Received: 05 March 2018; Accepted: 02 July 2018;
Published: 25 July 2018.
Edited by:
Elke Winterhager, Universität Duisburg-Essen, GermanyReviewed by:
Reecha Sharma, Saint Joseph’s University, United StatesGraham Burton, University of Cambridge, United Kingdom
Copyright © 2018 Armaly, Jadaon, Jabbour and Abassi. This is an open-access article distributed under the terms of the Creative Commons Attribution License (CC BY). The use, distribution or reproduction in other forums is permitted, provided the original author(s) and the copyright owner(s) are credited and that the original publication in this journal is cited, in accordance with accepted academic practice. No use, distribution or reproduction is permitted which does not comply with these terms.
*Correspondence: Zaher Armaly, emFoZXJhcm1hbHlAbmF6aG9zcC5jb20= Zaid A. Abassi, YWJhc3NpQHR4LnRlY2huaW9uLmFjLmls