- 1Institute of Health and Biological Science, Federal University of Mato Grosso, Barra do Garças, Brazil
- 2Institute of Biological Science, Federal University of Goias, Goiânia, Brazil
- 3Biomedical Research Center, School of Medicine, Universidad de Valparaíso, Valparaíso, Chile
Successful placentation is a key event for fetal development, which commences following embryo implantation into the uterine wall, eliciting decidualization, placentation, and remodeling of blood vessels to provide physiological exchange between embryo-fetus and mother. Several signaling pathways are recruited to modulate such important processes and specific proteins that regulate placental function are a target for the glycosylation with O-linked β-N-acetylglucosamine (O-GlcNAc), or O-GlcNAcylation. This is a reversible post-translational modification on nuclear and cytoplasmic proteins, mainly controlled by O-GlcNAc transferase (OGT) and O-GlcNAcase (OGA). O-GlcNAcylation has been implicated as a modulator of proteins, both in physiological and pathological conditions and, more recently, O-GlcNAc has also been shown to be an important modulator in placental tissue. In this mini-review, the interplay between O-GlcNAcylation of proteins and placental function will be addressed, discussing the possible implications of this post-translational modification through placental development and pregnancy.
Introduction
The hemochorial placenta allows blood coming from the maternal circulation to directly contact the fetal chorion, favoring nutrient exchange to the embryo and fetus (Croy et al., 2009). In addition to providing a complete environment for embryo-fetal development, this non-innervated organ also has important implications in endocrine and physiological control during pregnancy. These features display a tightly regulated placentation process requiring precise mechanisms to modulate embryo implantation, decidualization of the endometrium and uterine blood vessel remodeling to generate a functional placenta (Maltepe et al., 2010).
The placenta displays crucial functions during pregnancy, and its performance is associated with morphological integrity, providing the desirable environment for fetal development. Several maternal factors may impact placental function, including co-existence of diabetes, hypertension, and other conditions. Metabolic homeostasis, together with the development of gestational immune tolerance (Elliot and Crespi, 2006; Croy et al., 2009), favor an environment without stressors, and are requirements for successful placental development.
O-GlcNAcylation is a reversible and dynamic post-translational modification with O-linked β-N-acetylglucosamine (O-GlcNAc), targeting cytoplasmic and nuclear proteins at serine, threonine and tyrosine (Ser-Thr-Tyr) residues. This process occurs in several proteins in eukaryotic cells, and is analogous to protein phosphorylation (Lima et al., 2012; van der Laarse et al., 2018). Unlike other post-translational modifications, O-GlcNAc is regulated exclusively by two enzymes: O-GlcNAc transferase (OGT), which catalyzes the β -attachment of O-GlcNAc to the hydroxy groups of Ser-Thr-Tyr residues; and β-N-acetylglucosaminidase (OGA, or O-GlcNAcase), which catalyzes the hydrolytic cleavage of O-GlcNAc from proteins (Laczy et al., 2009; Lima et al., 2011). Interestingly, OGA and OGT are extensively expressed in placentas (Lubas et al., 1997; Gao et al., 2001) and several proteins that play important roles in placental function are targets for O-GlcNAcylation.
The most important source for O-GlcNAc formation is the hexosamine biosynthetic pathway (HBP). After glucose uptake, glucose can either be used in the glycolytic pathway or the HBP. The HBP uses fructose 6-phosphate to form glucosamine 6-phosphate, with glutamine serving as the donor of the amino group, whereas this reaction is catalyzed via glutamine: fructose 6-phosphate aminotransferase (GFAT), which is rapidly acetylated through the action of acetyl-CoA:d-glucosamine-6-phosphate N-acetyltransferase (GAT) and isomerized to N-acetylglucosamine-1-phosphate (GlcNAc-1-P). UDP-GlcNAc pyrophosphorylase then converts GlcNAc-1-P to UDP-GlcNAc, which serves as the donor for O-GlcNAc when OGT is activated. Glucosamine can also enter the cell through glucose transporters and is rapidly phosphorylated by hexokinase yielding glucosamine 6-phosphate, thereby passing the rate-limiting first step of the HBP (Figure 1; Lima et al., 2012).
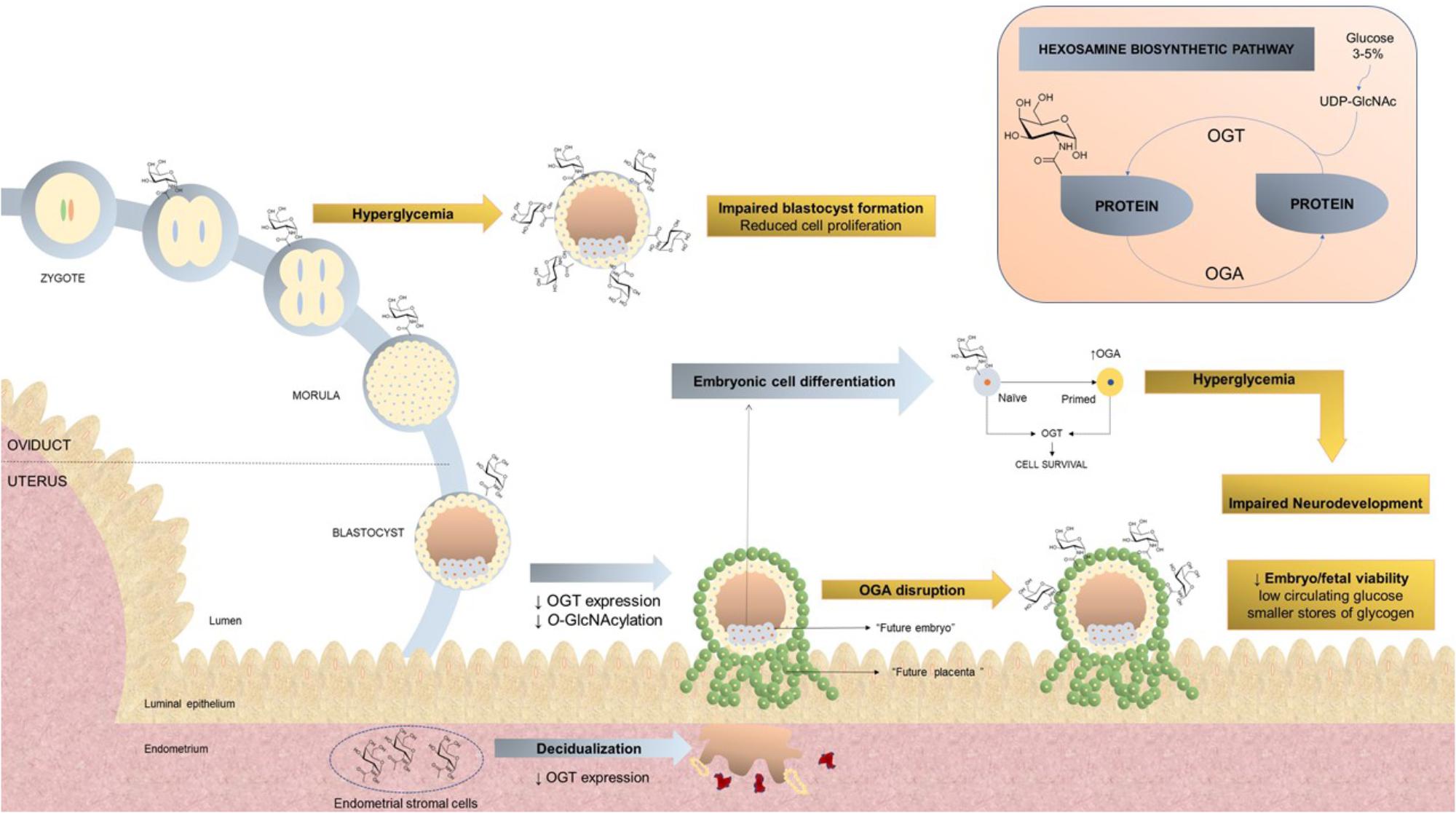
FIGURE 1. Hexosamine biosynthetic pathway and O-glycosylation with O-linked β-N-acetylglucosamine during pregnancy. (Pink rectangle) Around 3–5% of intracellular glucose is converted through the HBP to substrate for enzymes involved in the synthesis of UDP-GlcNAc, consequently leading to protein modification through O-GlcNAc. OGT catalyzes the addition of O-GlcNAc to proteins, whereas OGA removes O-GlcNAc from proteins. (Bigger figure) Under physiological conditions, O-GlcNAc modifications to intracellular proteins are essential for embryogenesis, promoting the function and viability of various cell types. This process may be disrupted under specific conditions, including hyperglycemia or OGA disruption, where an increase in O-GlcNAc is observed, with a consequent reduction in cell proliferation, impaired blastocyst formation and decreased embryo viability. Abbreviations: HBP, Hexosamine biosynthetic pathway; O-GlcNAc, glycosylation with O-linked β-N-acetylglucosamine; OGA, β-N-acetylglucosaminidase; OGT, O-GlcNAc transferase.
In this review, we will present evidence of how this post-translational modification interacts with proteins that are important for placental function, discussing possible implications in pregnancy.
O-GlcNAc During Pregnancy
O-GlcNAc Role During Pre-implantation, Implantation, and Embryo Development
Several stages of pregnancy may be affected by O-GlcNAc (Figure 1). Fast trophoblast proliferation, along with the development of the chorionic sac and chorionic villi marks the earliest stage of placental formation (Rossant and Cross, 2001). Maternal hyperglycemia may negatively impact pre-implantation by reducing the embryo’s ability for glucose uptake, favoring miscarriage and congenital anomalies (Jungheim and Moley, 2008; Damasceno et al., 2017). In hyperglycemia and glucosamine incubation increased O-GlcNAcylation occurs, resulting in reduced cell proliferation and, therefore, impaired blastocyst formation, as demonstrated in an experimental model of mouse zygote cultures. Inhibition of OGT was able to partially restore cell proliferation and blastocyst formation under hyperglycemic conditions, suggesting that dysregulation of both HBP and O-GlcNAcylation may favor embryotoxic effects during pre-implantation development (Pantaleon et al., 2010). O-GlcNAc displays an important role not only in trophoblast proliferation, but also in embryonic cell differentiation. Naïve mouse embryonic stem cells, derived from pre-implantation embryos, maintain an undifferentiated state via augmented O-GlcNAc expression (Shi et al., 2013). The differentiation process from naïve to a primed state, as observed in cells from post-implantation embryos, requires OGA expression, whereas OGT expression is observed in maintenance of cell survival (Jang et al., 2012; Shi et al., 2013; Speakman et al., 2014). Interestingly, OGT also contributes to cell survival of primed embryo cells (O’Donnell et al., 2004) and expression of both OGT and OGA is required to revert primed to naïve cells (Miura and Nishihara, 2016). Later, it was demonstrated that the histone variant H2A is a target for O-GlcNAc at Ser40, and this post-translational modification is required for H2A to contribute to the trophoblast stem cell differentiation process, being correlated with the evolution of placental tissue (Hirosawa et al., 2016). Indeed, H2A is required during mouse embryonic stem cell differentiation, allowing these cells to change gene expression. H2A is also highly expressed in early placental development (Kafer et al., 2015).
Post-translational modifications of histones represent an important mechanism of DNA damage repair. An example is the phosphorylation of the histone H2AX, of itself an effective repair mechanism. This process is, however, restrained to small compartments, and must occur within a limited time frame. O-GlcNAcylation also occurs in H2AX, limiting the expansion of DNA damage-induced phosphorylation of chromatin (Chen and Yu, 2016). Embryos from diabetic mothers display exacerbated DNA damage, evidenced by the co-localization of H2AX. O-GlcNAcylation was observed in diabetic blastocyst-stage embryos, favoring impairment of pre-implantation embryo development (Brown et al., 2018). That precursors coming from the metabolic flux have the ability to modulate nuclear function has been denominated metaboloepigenetics (Donohoe and Bultman, 2012); this mechanism provides evidence for an epigenetic contribution to the vertical transmission of diabetes.
OGA expression is also related to embryo/fetal viability since Oga gene disruption results in augmented levels of global O-GlcNAcylation. These genetically modified animals display high perinatal mortality, associated with low circulating glucose levels and smaller stores of glycogen in the liver. In this experimental model, other metabolic alterations were identified in heterozygous mice, including fat accumulation, reduced insulin sensitivity, glucose intolerance and hyperleptinemia. Oga disruption generated defective metabolic homeostasis, contributing to obesity and insulin resistance (Keembiyehetty et al., 2015).
Successful placentation involves decidual cells that encapsulate the implanting embryo, providing nutrition and favoring an immunological environment that allows trophoblast invasion and, later, placentation. It is mandatory for decidual cells to develop mechanisms to block stressor signals, favoring the integrity of this initial stage of the fetal-maternal interface (Weimar et al., 2012; Erlebacher, 2013). Decidualization of primary endometrial stromal cells results in reduced global O-GlcNAcylation, mediated by decreased OGT expression, without changes in OGA expression. Cell differentiation occurs simultaneously with enhanced expression of epidermal growth factor domain–specific O-linked GlcNAc transferase (EOGT), involving mechanisms of energy homeostasis and glucose and fatty acid metabolism, which explains, at least in part, adverse pregnancy outcomes observed in metabolic disorders (Muter et al., 2018).
O-GlcNAcylation impacts embryogenesis and alterations in the maternal glucose metabolism may disrupt neurodevelopment. Neural stem cells submitted to an in vitro hyperglycemic environment resulted in augmented global O-GlcNAcylation via OGT enhanced activity, and displayed neural tube defects, suggesting that inhibition of altered OGT might be beneficial in preventing birth defects in hyperglycemic pregnancies (Kim et al., 2017). Given the importance of this pathway on neurodevelopment, human embryonic stem cells were used to demonstrate that augmented global O-GlcNAcylation is associated with reduced neural progenitor proliferation and premature differentiation of cortical neurons. Therefore, O-GlcNAc regulation may represent an important mechanism observed in metabolically compromised pregnancies, contributing to neuronal impairment in the offspring (Parween et al., 2017).
O-GlcNAc and Immune System During Pregnancy
The embryo is recognized as non-self by the maternal immune system, and therefore, several adaptations are required to prevent rejection during implantation (Robertson and Moldenhauer, 2014). One important role of natural killer (NK) cells is to destroy cells that fail to express major histocompatibility complex (MHC) class I molecules; during pregnancy, in contrast to their primary function, placental NK cells tolerate cells from fetal tissue, which do not express maternal MHC I molecules (Ljunggren and Karre, 1990; King and Loke, 1991). A possible explanation for the tissue-specific behavior of placental NK cells is that non-classical MHC I molecules, including human leukocyte antigen-G (HLA-G), are expressed in fetal extra villous trophoblasts and may inhibit NK cell cytotoxicity during pregnancy (Carosella et al., 1999; Kumpel and Manoussaka, 2012). Trophoblast cells present in the maternofetal interface secrete a soluble HLA-G1 (sHLA-G1) isoform in the amniotic fluid, and are released into the maternal circulation, favoring systemic immunoinhibitory activity (McMaster et al., 1998). Indeed, sHLA-G1 secreted by syncytiotrophoblast specifically induces the apoptosis of CD8+ T cells (Solier et al., 2002). Interestingly, NK cell cytotoxicity occurs simultaneously with reduced O-GlcNAcylation and seems to be inhibited by the sHLA-G1 α chain via an O-GlcNAc dependent-mechanism. When NK92 cells, an NK cell line, were submitted to a cytotoxicity assay using K562 cells as target cells, O-GlcNAc levels decreased inversely to cytotoxic activity (Yao et al., 2004). Preincubation with GST-HLA-Glα chain prevented the decrease of O-GlcNAc levels, reverting NK92 cytotoxicity.
Cytokine production is also modulated by O-GlcNAcylation. Augmented O-GlcNAc levels in the placenta during hyperglycemia coincided with augmented placental levels of interleukin (IL)-6 and tumor necrosis factor alpha (TNF-α). Interestingly, both cytokines positively correlated with placental weight, and negatively correlated with fetal weight and placental efficiency in hyperglycemic conditions (Dela Justina et al., 2017). Reduced embryo implantation and impaired blastocyst development was observed in an experimental mouse model of diabetes. These findings were related to an inflammatory imbalance, elicited by increased expression of pro-inflammatory cytokines in the uterus, including IL-1α, TNF-α, and interferon gamma (IFNγ), and a pronounced reduction of anti-inflammatory regulatory T-cells within the uterus-draining lymph nodes (Brown et al., 2018).
O-GlcNAC Regulation of Transcriptional Factors in Placental Tissue
Post-translational modifications are important key regulators of transcription factors. Considering the high occurrence of O-GlcNAcylation of nuclear proteins, O-GlcNAc has been implicated to be a major modulator of transcriptional activity (Ozcan et al., 2010). The first transcription factor described to be a target for O-GlcNAc modulation was specific protein 1 (Sp-1), a member of the Sp factors; removal of O-GlcNAc favored protein association, as demonstrated in HeLa cells (Roos et al., 1997).
Later, it was demonstrated that transcription factors from the placenta are also targets of O-GlcNAc modulation. The oncofetal protein gene (Pem) is expressed in a stage-specific manner during murine embryogenesis in placental, but not adult, tissues (Wilkinson et al., 1990). Murine placenta and embryonic expression of Pem is highly regulated, involving E74 like Ets transcription factor 1 (Elf-1) and Sp-1 transcription factors (Rao et al., 2002). Efl-1, which belongs to the Ets family, and Sp-1 are, similarly, modulated by O-GlcNAc (Jackson and Tjian, 1988; Juang et al., 2002; Chu and Ferro, 2005). Sp-1 and Elf-1 activate Pem promoter elements, favoring its transcription (Rao et al., 2002). In vitro, DNA binding and competition assays have demonstrated that O-GlcNAcylation of Sp-1 does not affect DNA binding itself, but reduces the ability of Sp-1 to interact with Elf-1. As a result, the activation of the Pem promoter element is reduced, and, thus, Pem expression (Lim and Chang, 2009). This was the first work to provide evidence that O-GlcNAc modulates transcription factors that regulate genes specifically expressed during embryogenesis, displaying a placental distribution.
The hypoxia-inducible factor-1alpha (HIF-1α), is a subunit of a heterodimeric transcription factor, hypoxia-inducible factor 1 (HIF-1), which is stabilized at the protein level in response to hypoxia. This transcription factor plays an essential role during vascular development of the placenta and OGA favors HIF-1α stabilization. OGA deficient mice display elevated O-GlcNAcylation along with defective placental vasculogenesis, characterized by reduced vasculature in the labyrinth region, directly contributing to fetal growth restriction. Interestingly, in this model OGA deletion reduced OGT activity, which resulted in HIF-1α suppression, reducing transcriptional activation of target genes (Yang et al., 2015). This evidences an important role of O-GlcNAcylation in cellular stress conditions. During proliferation, OGT deletion, which reduces O-GlcNAc levels, modulates HIF-1α favoring ER stress-mediated apoptosis, as observed in cancer cells (Ferrer et al., 2014).
Under hyperglycemic conditions, O-GlcNAc modifications also occur in nuclear factor kappa-light-chain-enhancer of activated B cells (NF-κB), found in rat placental tissue (Dela Justina et al., 2017). This represents non-canonical activation of NF-κB, also described in other cells (Yang et al., 2008), in which augmented levels of O-GlcNAc bind to NF-κB, favoring its translocation to the nucleus, augmenting production of pro-inflammatory cytokines (Dela Justina et al., 2017).
O-GlcNAc and Maternal Stress
In several cell types, O-GlcNAcylation provides an important tool for cell survival in conditions of elevated stressors, and several forms of cellular injury result in dynamic changes to O-GlcNAcylation (Martinez et al., 2017). During pregnancy, exposure to stress contributes to maternal and fetal metabolic alterations and reduces placental growth. Both maternal stress and growth restriction have been consistently associated with metabolic dysfunction observed in offspring (Figure 2). High glucose levels, observed in mothers submitted to experimental models of stress, impact the gene expression profile of offspring. OGT is one of the genes affected and, in conditions of maternal stress, offspring display reduced OGT expression in the labyrinth region (Briffa et al., 2017).
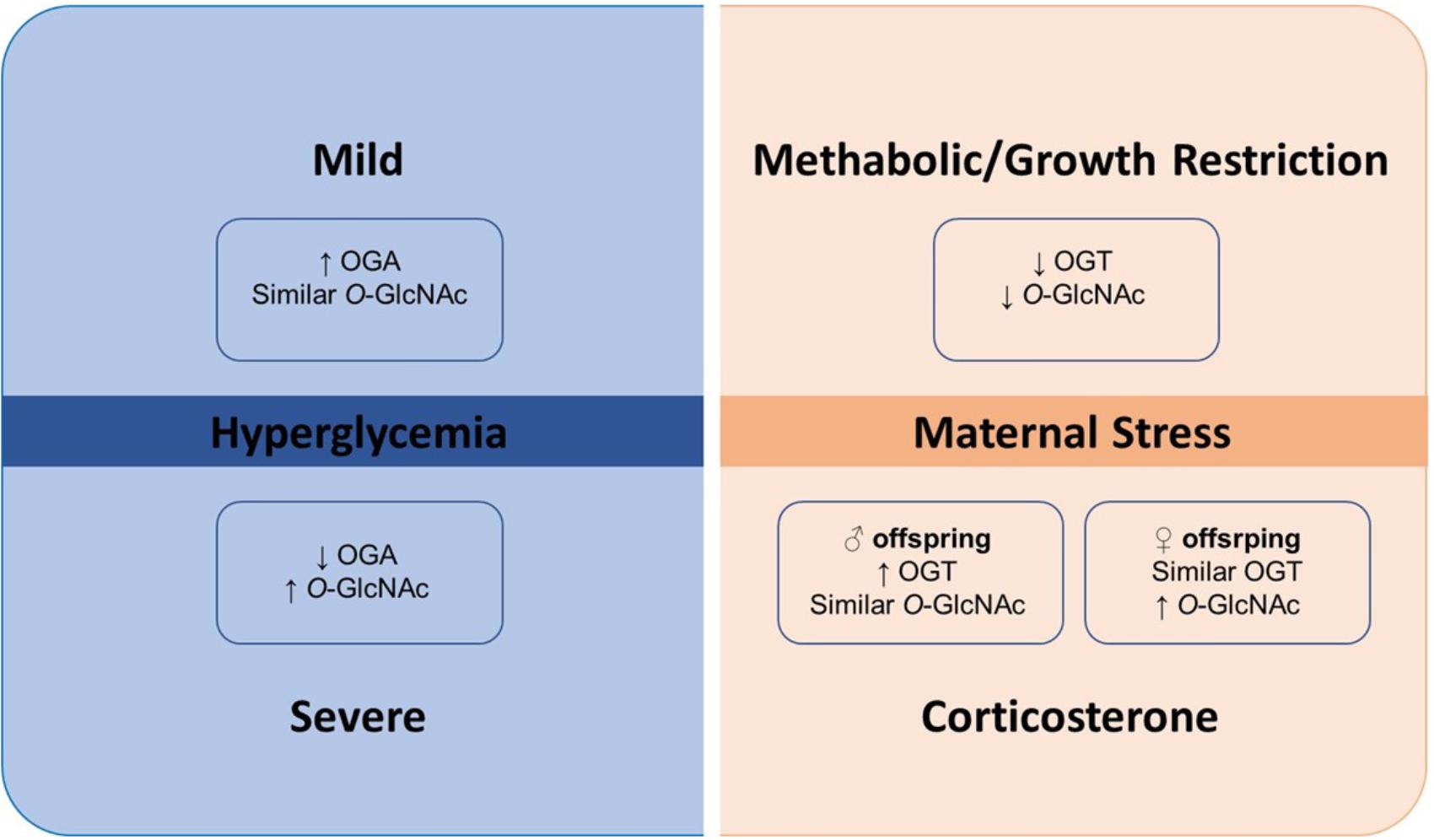
FIGURE 2. Metabolic maternal stress and O-GlcNAc. O-GlcNAcylation appears to act as a stress sensor since it exerts its fundamental effects in response to stress. OGT has also been identified as a placental biomarker of cellular stress. During metabolic maternal stress and growth restriction, both OGT and O-GlcNAcylation were significantly lower. Placentas of female mice offspring had higher basal OGT expression compared to placentas of male offspring. However, following exposure to corticosterone, OGT expression raised in male placentas and remained the same in female placentas, simultaneously with increased global O-GlcNAcylation in female placentas, which was unmodified in male placentas. Abbreviations: O-GlcNAc, glycosylation with O-linked β-N-acetylglucosamine; OGA, β-N-acetylglucosaminidase; OGT, O-GlcNAc transferase.
A genome-wide array approach identified OGT as a cellular stress marker during pregnancy. Both OGT and O-GlcNAcylation were significantly lower when mothers were submitted to prenatal stress. In addition to being a biomarker of stress, OGT was also shown to be crucial for neurodevelopment, and its expression was affected to a greater extent in male, compared to female offspring (Howerton et al., 2013). Physiologically, O-GlcNAcylation is greatly decreased during early development and the shortest isoform of OGT (sOGT) is essentially undetectable during early development, but increases consistently after birth (Liu et al., 2012). To address the direct impact of placental OGT in programming the developing brain, an elegant study was conducted in a transgenic mouse model with targeted placental disruption of OGT (PI-OGT). Offspring from this model were compared to those of a mouse model of early prenatal stress (Howerton and Bale, 2014). These experiments confirmed that OGT is an important placental biomarker of maternal stress, resulting in a long-term harmful effect on offspring, which is suitable for metabolic and neurodevelopmental programing under these conditions.
It appears that OGT expression may change according to the kind and intensity of stress experienced by the mother, and affect offspring in a sexually dimorphic manner. Physiologically, the placenta of female mice offspring had higher basal OGT expression, compared to placentas of males. Following corticosterone exposure, OGT expression increased in placentas of males, but global O-GlcNAcylation was not modified, whereas, in placentas of females, OGT expression remained the same, and increased global O-GlcNAcylation was observed. These findings show that placentas from female offspring have a greater capacity to rapidly respond to maternal stress and suggests that offspring are affected by maternal stress in a sexually dimorphic pattern. This may impact future life, where males may be more suitable for diseases that are influenced by intra-uterus environment (Pantaleon et al., 2017). A deleterious consequence of maternal stress on offspring includes depression. Adult female rats displayed sex-specific depressive-like behavior when submitted to an intrauterine stress environment as offspring, while males did not display these symptoms (Liu et al., 2018). Interestingly, when these animals were subjected to a swimming exercise, depression symptoms were ameliorated, and this improvement was associated with OGT-related mitochondrial motility.
Metabolic maternal stress, as observed in placentas during severe hyperglycemia, resulted in increased O-GlcNAc levels compared to placentas from control and mildly hyperglycemic rats. OGA expression was reduced in placentas from the severely hyperglycemic rats, whereas augmented OGA was found in placentas from the mild hyperglycemic group, compared to control. No changes in OGT were observed during severe or mild hyperglycemia. O-GlcNAc overexpression in hyperglycemic conditions co-exist with placental dysfunction, which was characterized by morphometric alterations along with reduced placental index (Dela Justina et al., 2018).
Perspectives
The evidence relating O-GlcNAc to placental function is at present still limited, mainly due to the limited number of studies conducted so far. OGA and OGT expression and O-GlcNAc modification represent important modulator mechanisms involved in placental development. Several pathological conditions result in augmented O-GlcNAc levels and may impact these mechanisms, leading to impaired placental development and adversely affect fetal growth. Most of the work conducted has been performed in cell culture or in experimental models of hyperglycemia; future work would be served by evaluating O-GlcNAcylation in human placentas. In addition to hyperglycemia, high O-GlcNAc levels co-exist in other conditions, including hypertension (Zachara, 2012), kidney injury (Hu et al., 2017), high-fat diet (Lima et al., 2016), obesity (da Costa et al., 2018), cancer (Tiainen et al., 2016), among others. Therefore, a next step would be to verify how the altered O-GlcNAc levels observed in various pathological conditions might impact placental development.
The current knowledge on this topic also reveals a potential area for exploration in sexual dimorphism during placentation. This is particularly important at the time of the placental collection in animal studies, with respect to correct sex identification. Hence, it will be exciting to describe whether O-GlcNAcylation occurs in a similar pattern at different stages of placentation for male and female offspring.
Several conventional pharmacological and non-pharmacological treatments, including medicinal plants, exercise, among others, are used to improve pregnancy outcomes and fetal growth. It will be interesting to evaluate whether these strategies impact O-GlcNAcylation of placental proteins or improve gestational success in pathological conditions.
Members of the RIVA-TREM
Victor Vitorino Lima, Sebastian San Martin, Paula Cristina S. Souto, Fernanda Regina Giachini (www.rivatrem.org).
Author Contributions
FG and VL have proposed the topic of this revision and designed the figures. All the authors have contributed to information recruitment, revision design, to write and revise the present version. FG has proposed the topic of this revision and designed the figures. All the authors have contributed to design, write and revise the present version.
Funding
This study was financed in part by the Coordenação de Aperfeiçoamento de Pessoal de Nível Superior – Brazil (CAPES) – Finance Code 001.
Conflict of Interest Statement
The authors declare that the research was conducted in the absence of any commercial or financial relationships that could be construed as a potential conflict of interest.
Acknowledgments
We thank Jana Stojanova for her valuable assistance in the linguistic revision.
References
Briffa, J. F., Hosseini, S. S., Tran, M., Moritz, K. M., Cuffe, J. S. M., and Wlodek, M. E. (2017). Maternal growth restriction and stress exposure in rats differentially alters expression of components of the placental glucocorticoid barrier and nutrient transporters. Placenta 59, 30–38. doi: 10.1016/j.placenta.2017.09.006
Brown, H. M., Green, E. S., Tan, T. C. Y., Gonzalez, M. B., Rumbold, A. R., Hull, M. L., et al. (2018). Periconception onset diabetes is associated with embryopathy and fetal growth retardation, reproductive tract hyperglycosylation and impaired immune adaptation to pregnancy. Sci. Rep. 8:2114. doi: 10.1038/s41598-018-19263-8
Carosella, E. D., Rouas-Freiss, N., Paul, P., and Dausset, J. (1999). HLA-G: a tolerance molecule from the major histocompatibility complex. Immunol. Today 20, 60–62.
Chen, Q., and Yu, X. (2016). OGT restrains the expansion of DNA damage signaling. Nucleic Acids Res. 44, 9266–9278. doi: 10.1093/nar/gkw663
Chu, S., and Ferro, T. J. (2005). Sp1: regulation of gene expression by phosphorylation. Gene 348, 1–11. doi: 10.1016/j.gene.2005.01.013
Croy, B. A., Wessels, J., Linton, N., and Tayade, C. (2009). Comparison of immune cell recruitment and function in endometrium during development of epitheliochorial (pig) and hemochorial (mouse and human) placentas. Placenta 30(Suppl. A), S26–S31. doi: 10.1016/j.placenta.2008.09.019S0143-4004(08)00334-2
da Costa, R. M., da Silva, J. F., Alves, J. V., Dias, T. B., Rassi, D. M., Garcia, L. V., et al. (2018). Increased O-GlcNAcylation of endothelial nitric oxide synthase compromises the anti-contractile properties of perivascular adipose tissue in metabolic syndrome. Front. Physiol. 9:341. doi: 10.3389/fphys.2018.00341
Damasceno, D. C., Leal-Silva, T., Soares, T. S., Moraes-Souza, R. Q., and Volpato, G. T. (2017). Medicinal Plants for Diabetes Treatment During Pregnancy. Curr. Med. Chem. 24, 404–410. doi: 10.2174/0929867323666161003122914
Dela Justina, V., Dos Passos, R. R., Bressan, A. F., Tostes, R. C., Carneiro, F. S., Soares, T. S., et al. (2018). O-linked N-acetyl-glucosamine deposition in placental proteins varies according to maternal glycemic levels. Life Sci. 15, 18–25. doi: 10.1016/j.lfs.2018.05.013
Dela Justina, V., Goncalves, J. S., de Freitas, R. A., Fonseca, A. D., Volpato, G. T., Tostes, R. C., et al. (2017). Increased O-Linked N-acetylglucosamine modification of NF-KappaB and augmented cytokine production in the placentas from Hyperglycemic Rats. Inflammation 40, 1773–1781. doi: 10.1007/s10753-017-0620-7
Donohoe, D. R., and Bultman, S. J. (2012). Metaboloepigenetics: interrelationships between energy metabolism and epigenetic control of gene expression. J. Cell Physiol. 227, 3169–3177. doi: 10.1002/jcp.24054
Elliot, M. G., and Crespi, B. J. (2006). Placental invasiveness mediates the evolution of hybrid inviability in mammals. Am. Nat. 168, 114–120. doi: 10.1086/505162
Erlebacher, A. (2013). Immunology of the maternal-fetal interface. Annu. Rev. Immunol. 31, 387–411. doi: 10.1146/annurev-immunol-032712-100003
Ferrer, C. M., Lynch, T. P., Sodi, V. L., Falcone, J. N., Schwab, L. P., Peacock, D. L., et al. (2014). O-GlcNAcylation regulates cancer metabolism and survival stress signaling via regulation of the HIF-1 pathway. Mol. Cell 54, 820–831. doi: 10.1016/j.molcel.2014.04.026S1097-2765(14)00360-8
Gao, Y., Wells, L., Comer, F. I., Parker, G. J., and Hart, G. W. (2001). Dynamic O-glycosylation of nuclear and cytosolic proteins: cloning and characterization of a neutral, cytosolic beta-N-acetylglucosaminidase from human brain. J. Biol. Chem. 276, 9838–9845. doi: 10.1074/jbc.M010420200
Hirosawa, M., Hayakawa, K., Yoneda, C., Arai, D., Shiota, H., Suzuki, T., et al. (2016). Novel O-GlcNAcylation on Ser(40) of canonical H2A isoforms specific to viviparity. Sci. Rep. 6:31785. doi: 10.1038/srep31785
Howerton, C. L., and Bale, T. L. (2014). Targeted placental deletion of OGT recapitulates the prenatal stress phenotype including hypothalamic mitochondrial dysfunction. Proc. Natl. Acad. Sci. U.S.A. 111, 9639–9644. doi: 10.1073/pnas.1401203111
Howerton, C. L., Morgan, C. P., Fischer, D. B., and Bale, T. L. (2013). O-GlcNAc transferase (OGT) as a placental biomarker of maternal stress and reprogramming of CNS gene transcription in development. Proc. Natl. Acad. Sci. U.S.A. 110, 5169–5174. doi: 10.1073/pnas.1300065110
Hu, J., Chen, R., Jia, P., Fang, Y., Liu, T., Song, N., et al. (2017). Augmented O-GlcNAc signaling via glucosamine attenuates oxidative stress and apoptosis following contrast-induced acute kidney injury in rats. Free Radic. Biol. Med. 103, 121–132. doi: 10.1016/j.freeradbiomed.2016.12.032
Jackson, S. P., and Tjian, R. (1988). O-glycosylation of eukaryotic transcription factors: implications for mechanisms of transcriptional regulation. Cell 55, 125–133.
Jang, H., Kim, T. W., Yoon, S., Choi, S. Y., Kang, T. W., Kim, S. Y., et al. (2012). O-GlcNAc regulates pluripotency and reprogramming by directly acting on core components of the pluripotency network. Cell Stem Cell 11, 62–74. doi: 10.1016/j.stem.2012.03.001
Juang, Y. T., Solomou, E. E., Rellahan, B., and Tsokos, G. C. (2002). Phosphorylation and O-linked glycosylation of Elf-1 leads to its translocation to the nucleus and binding to the promoter of the TCR zeta-chain. J. Immunol. 168, 2865–2871.
Jungheim, E. S., and Moley, K. H. (2008). The impact of type 1 and type 2 diabetes mellitus on the oocyte and the preimplantation embryo. Semin. Reprod. Med. 26, 186–195. doi: 10.1055/s-2008-1042957
Kafer, G. R., Carlton, P. M., and Lehnert, S. A. (2015). The histone variant H2A.Z is dynamically expressed in the developing mouse placenta and in differentiating trophoblast stem cells. Placenta 36, 1325–1328. doi: 10.1016/j.placenta.2015.08.018S0143-4004(15)30045-X
Keembiyehetty, C., Love, D. C., Harwood, K. R., Gavrilova, O., Comly, M. E., and Hanover, J. A. (2015). Conditional knock-out reveals a requirement for O-linked N-Acetylglucosaminase (O-GlcNAcase) in metabolic homeostasis. J. Biol. Chem. 290, 7097–7113. doi: 10.1074/jbc.M114.617779
Kim, G., Cao, L., Reece, E. A., and Zhao, Z. (2017). Impact of protein O-GlcNAcylation on neural tube malformation in diabetic embryopathy. Sci. Rep. 7:11107. doi: 10.1038/s41598-017-11655-6
King, A., and Loke, Y. W. (1991). On the nature and function of human uterine granular lymphocytes. Immunol. Today 12, 432–435. doi: 10.1016/0167-5699(91)90014-K
Kumpel, B. M., and Manoussaka, M. S. (2012). Placental immunology and maternal alloimmune responses. Vox Sang 102, 2–12. doi: 10.1111/j.1423-0410.2011.01533.x
Laczy, B., Hill, B. G., Wang, K., Paterson, A. J., White, C. R., Xing, D., et al. (2009). Protein O-GlcNAcylation: a new signaling paradigm for the cardiovascular system. Am. J. Physiol. Heart Circ. Physiol. 296, H13–H28. doi: 10.1152/ajpheart.01056.2008
Lim, K., and Chang, H. I. (2009). O-GlcNAc inhibits interaction between Sp1 and Elf-1 transcription factors. Biochem. Biophys. Res. Commun. 380, 569–574. doi: 10.1016/j.bbrc.2009.01.121
Lima, V. V., Giachini, F. R., Hardy, D. M., Webb, R. C., and Tostes, R. C. (2011). O-GlcNAcylation: a novel pathway contributing to the effects of endothelin in the vasculature. Am. J. Physiol. Regul. Integr. Comp. Physiol. 300, R236–R250. doi: 10.1152/ajpregu.00230.2010ajpregu.00230.2010
Lima, V. V., Giachini, F. R., Matsumoto, T., Li, W., Bressan, A. F., Chawla, D., et al. (2016). High-fat diet increases O-GlcNAc levels in cerebral arteries: a link to vascular dysfunction associated with hyperlipidaemia/obesity? Clin. Sci. 130, 871–880. doi: 10.1042/CS20150777CS20150777
Lima, V. V., Spitler, K., Choi, H., Webb, R. C., and Tostes, R. C. (2012). O-GlcNAcylation and oxidation of proteins: is signalling in the cardiovascular system becoming sweeter? Clin. Sci. 123, 473–486. doi: 10.1042/CS20110638
Liu, W., Wang, H., Xue, X., Xia, J., Liu, J., Qi, Z., et al. (2018). OGT-related mitochondrial motility is associated with sex differences and exercise effects in depression induced by prenatal exposure to glucocorticoids. J. Affect. Disord. 226, 203–215. doi: 10.1016/j.jad.2017.09.053
Liu, Y., Li, X., Yu, Y., Shi, J., Liang, Z., Run, X., et al. (2012). Developmental regulation of protein O-GlcNAcylation, O-GlcNAc transferase, and O-GlcNAcase in mammalian brain. PLoS One 7:e43724. doi: 10.1371/journal.pone.0043724
Ljunggren, H. G., and Karre, K. (1990). In search of the ’missing self’: MHC molecules and NK cell recognition. Immunol. Today 11, 237–244.
Lubas, W. A., Frank, D. W., Krause, M., and Hanover, J. A. (1997). O-Linked GlcNAc transferase is a conserved nucleocytoplasmic protein containing tetratricopeptide repeats. J. Biol. Chem. 272, 9316–9324.
Maltepe, E., Bakardjiev, A. I., and Fisher, S. J. (2010). The placenta: transcriptional, epigenetic, and physiological integration during development. J. Clin. Invest. 120, 1016–1025. doi: 10.1172/JCI4121141211
Martinez, M. R., Dias, T. B., Natov, P. S., and Zachara, N. E. (2017). Stress-induced O-GlcNAcylation: an adaptive process of injured cells. Biochem. Soc. Trans. 45, 237–249. doi: 10.1042/BST20160153BST20160153
McMaster, M., Zhou, Y., Shorter, S., Kapasi, K., Geraghty, D., Lim, K. H., et al. (1998). HLA-G isoforms produced by placental cytotrophoblasts and found in amniotic fluid are due to unusual glycosylation. J. Immunol. 160, 5922–5928.
Miura, T., and Nishihara, S. (2016). O-GlcNAc is required for the survival of primed pluripotentstem cells and their reversion to the naive state. Biochem. Biophys. Res. Commun. 480, 655–661. doi: 10.1016/j.bbrc.2016.10.111
Muter, J., Alam, M. T., Vrljicak, P., Barros, F. S. V., Ruane, P. T., Ewington, L. J., et al. (2018). The Glycosyltransferase EOGT Regulates Adropin Expression in Decidualizing Human Endometrium. Endocrinology 159, 994–1004. doi: 10.1210/en.2017-030644736308
O’Donnell, N., Zachara, N. E., Hart, G. W., and Marth, J. D. (2004). Ogt-dependent X-chromosome-linked protein glycosylation is a requisite modification in somatic cell function and embryo viability. Mol. Cell. Biol. 24, 1680–1690.
Ozcan, S., Andrali, S. S., and Cantrell, J. E. (2010). Modulation of transcription factor function by O-GlcNAc modification. Biochim. Biophys. Acta 1799, 353–364. doi: 10.1016/j.bbagrm.2010.02.005S1874-9399(10)00047-7
Pantaleon, M., Steane, S. E., McMahon, K., Cuffe, J. S. M., and Moritz, K. M. (2017). Placental O-GlcNAc-transferase expression and interactions with the glucocorticoid receptor are sex specific and regulated by maternal corticosterone exposure in mice. Sci. Rep. 7:2017. doi: 10.1038/s41598-017-01666-810.1038/s41598-017-01666-8
Pantaleon, M., Tan, H. Y., Kafer, G. R., and Kaye, P. L. (2010). Toxic effects of hyperglycemia are mediated by the hexosamine signaling pathway and o-linked glycosylation in early mouse embryos. Biol. Reprod. 82, 751–758. doi: 10.1095/biolreprod.109.076661
Parween, S., Varghese, D. S., Ardah, M. T., Prabakaran, A. D., Mensah-Brown, E., Emerald, B. S., et al. (2017). Higher O-GlcNAc levels are associated with defects in progenitor proliferation and premature neuronal differentiation during in-vitro human embryonic cortical neurogenesis. Front. Cell. Neurosci. 11:415. doi: 10.3389/fncel.2017.00415
Rao, M. K., Maiti, S., Ananthaswamy, H. N., and Wilkinson, M. F. (2002). A highly active homeobox gene promoter regulated by Ets and Sp1 family members in normal granulosa cells and diverse tumor cell types. J. Biol. Chem. 277, 26036–26045. doi: 10.1074/jbc.M203374200
Robertson, S. A., and Moldenhauer, L. M. (2014). Immunological determinants of implantation success. Int J Dev Biol. 58, 205–217. doi: 10.1387/ijdb.140096sr
Roos, M. D., Su, K., Baker, J. R., and Kudlow, J. E. (1997). O glycosylation of an Sp1-derived peptide blocks known Sp1 protein interactions. Mol. Cell Biol. 17, 6472–6480.
Rossant, J., and Cross, J. C. (2001). Placental development: lessons from mouse mutants. Nat. Rev. Genet. 2, 538–548. doi: 10.1038/35080570
Shi, F. T., Kim, H., Lu, W., He, Q., Liu, D., Goodell, M. A., et al. (2013). Ten-eleven translocation 1 (Tet1) is regulated by O-linked N-acetylglucosamine transferase (Ogt) for target gene repression in mouse embryonic stem cells. J. Biol. Chem. 288, 20776–20784. doi: 10.1074/jbc.M113.460386
Solier, C., Aguerre-Girr, M., Lenfant, F., Campan, A., Berrebi, A., Rebmann, V., et al. (2002). Secretion of pro-apoptotic intron 4-retaining soluble HLA-G1 by human villous trophoblast. Eur. J. Immunol. 32, 3576–3586.
Speakman, C. M., Domke, T. C., Wongpaiboonwattana, W., Sanders, K., Mudaliar, M., van Aalten, D. M., et al. (2014). Elevated O-GlcNAc levels activate epigenetically repressed genes and delay mouse ESC differentiation without affecting naive to primed cell transition. Stem Cells 32, 2605–2615. doi: 10.1002/stem.1761
Tiainen, S., Oikari, S., Tammi, M., Rilla, K., Hamalainen, K., Tammi, R., et al. (2016). High extent of O-GlcNAcylation in breast cancer cells correlates with the levels of HAS enzymes, accumulation of hyaluronan, and poor outcome. Breast Cancer Res. Treat. 160, 237–247. doi: 10.1007/s10549-016-3996-4
van der Laarse, S. A. M., Leney, A. C., and Heck, A. J. R. (2018). Crosstalk between phosphorylation and O-GlcNAcylation: friend or foe. FEBS J. doi: 10.1111/febs.14491 [Epub ahead of print].
Weimar, C. H., Kavelaars, A., Brosens, J. J., Gellersen, B., de Vreeden-Elbertse, J. M., Heijnen, C. J., et al. (2012). Endometrial stromal cells of women with recurrent miscarriage fail to discriminate between high- and low-quality human embryos. PLoS One 7:e41424. doi: 10.1371/journal.pone.0041424PONE-D-12-06894
Wilkinson, M. F., Kleeman, J., Richards, J., and MacLeod, C. L. (1990). A novel oncofetal gene is expressed in a stage-specific manner in murine embryonic development. Dev. Biol. 141, 451–455.
Yang, W. H., Park, S. Y., Nam, H. W., Kim, D. H., Kang, J. G., Kang, E. S., et al. (2008). NFkappaB activation is associated with its O-GlcNAcylation state under hyperglycemic conditions. Proc. Natl. Acad. Sci. U.S.A. 105, 17345–17350. doi: 10.1073/pnas.0806198105
Yang, Y. R., Jang, H. J., Lee, Y. H., Kim, I. S., Lee, H., Ryu, S. H., et al. (2015). O-GlcNAc cycling enzymes control vascular development of the placenta by modulating the levels of HIF-1alpha. Placenta 36, 1063–1068. doi: 10.1016/j.placenta.2015.08.001S0143-4004(15)30028-X
Yao, A. Y., Tang, H. Y., Wang, Y., Feng, M. F., and Zhou, R. L. (2004). Inhibition of the activating signals in NK92 cells by recombinant GST-sHLA-G1a chain. Cell Res. 14, 155–160. doi: 10.1038/sj.cr.7290215
Keywords: placental dysfunction, O-GlcNAc, post-translational modification, pregnancy, placentation
Citation: Lima VV, Dela Justina V, dos Passos RR Jr, Volpato GT, Souto PCS, San Martin S and Giachini FR (2018) O-GlcNAc Modification During Pregnancy: Focus on Placental Environment. Front. Physiol. 9:1263. doi: 10.3389/fphys.2018.01263
Received: 06 June 2018; Accepted: 21 August 2018;
Published: 12 September 2018.
Edited by:
Pasquale Pagliaro, Università degli Studi di Torino, ItalyReviewed by:
Brett M. Mitchell, Texas A&M Health Science Center, United StatesKeshari Thakali, University of Arkansas for Medical Sciences, United States
Copyright © 2018 Lima, Dela Justina, dos Passos, Volpato, Souto, San Martin and Giachini. This is an open-access article distributed under the terms of the Creative Commons Attribution License (CC BY). The use, distribution or reproduction in other forums is permitted, provided the original author(s) and the copyright owner(s) are credited and that the original publication in this journal is cited, in accordance with accepted academic practice. No use, distribution or reproduction is permitted which does not comply with these terms.
*Correspondence: Fernanda Regina Giachini, fernandagiachini@hotmail.com