- Internal Medicine 3, Department of Medicine, University of Padua, Padua, Italy
Obesity is the consequence of chronic positive energy balance and considered a leading risk factor for cardiovascular and metabolic diseases. Due to its epidemic trends among children and adults, there is an increasing interest in implementing new therapeutic interventions to tackle overweight and obesity. Activation of brown adipose tissue (BAT) represents today a promising strategy to enhance energy expenditure (EE) through heat production. More recently, “browning” of white adipose tissue (WAT) has gained increasing attention in research area as an alternative method in stimulating energy dissipation. This minireview aims to summarize the current knowledge of some dietary compounds that have been shown to promote BAT activation and WAT browning with subsequent beneficial health effects.
Introduction
Obesity is defined as an abnormal body fat accumulation due to chronic periods of imbalance between energy intake and expenditure. A major concern is the high risk of accompanying cluster of comorbidities, such as CVDs, metabolic syndrome and some forms of cancer (Andolfi and Fisichella, 2018).
The management of body weight is mainly based on lifestyle modifications and modulating the absorption of food. On the other hand, approaches aimed to enhance EE represent an alternative tool for counteracting obesity and related cardiometabolic disorders (Khera et al., 2016).
In addition to the well known WAT storing lipids and undergoing pathological structural and functional changes during obesity, mammals including adult humans are also equipped with BAT conferred with thermogenic capacity called adaptive or NST (Virtanen et al., 2009; Rosenwald and Wolfrum, 2014). BAT is a metabolically active tissue rich in mitochondria containing UCP1 that mediates the uncoupling of electron transport which leads to a decrease in the generation of ATP from adenosine diphosphate (ADP) with subsequent heat production (Bartelt and Heeren, 2014; Rossato et al., 2014). Today, the induction of BAT thermogenesis represents a promising protective strategy to combat obesity in humans by increasing EE (Rosenwald and Wolfrum, 2014).
However, NST is not restricted only to classical brown adipocytes since certain stimulations as cold exposure or ADRB3 activators can cause the so-called beige or brown-like adipocytes to emerge within WAT depots in a process termed “WAT browning” (Kiefer, 2017). The main physiological, biochemical and morphological characteristics of each adipose tissue subtype have been briefly summarized in Figure 1.
Research over the last decades has provided evidence to support the role of bioactive dietary components in the prevention and/or treatment of obesity and associated metabolic disorders (Martínez-González et al., 2015; Amiot et al., 2016). The current minireview aims to summarize the latest findings concerning the activation of BAT or browning of WAT induced by specific dietary components, including capsaicin, resveratrol, curcumin, green tea, menthol and fish-derived Omega-3 fatty acids.
In Figures 2 and 3 we have summarized the main physiologic mechanisms involved in BAT promotion through these dietary compounds.
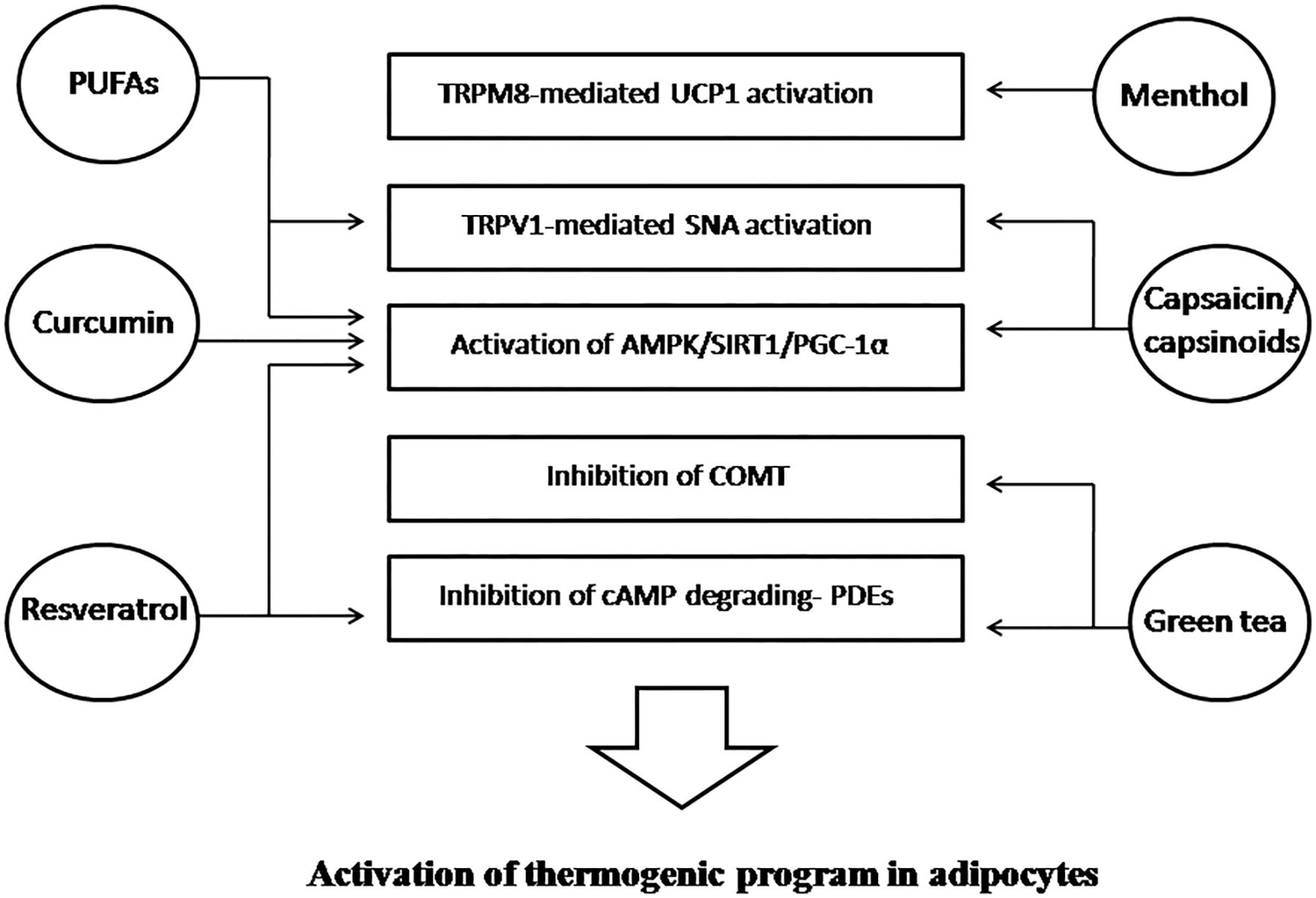
Figure 2. Food-derived components stimulating energy expenditure and their mechanisms of action involved in the activation of BAT or in the induction of WAT browning. BAT, brown adipose tissue; WAT, white adipose tissue; TRPM8, transient receptor potential cation channel melastatin 8; UCP1, uncoupling protein 1; TRPV1, transient receptor potential vanilloid 1; SNA, sympathetic nerve activity; AMPK 5′, adenosine monophosphate-activated protein kinase, SIRT1, sirtuin-1; PGC-1α, peroxisome proliferator-activated receptor gamma coactivator 1-alpha; COMT, catechol-O-methyl-transferase cAMP; cyclic adenosine monophosphate; PDEs, phosphodiesterases; PUFAs, polyunsaturated fatty acids.
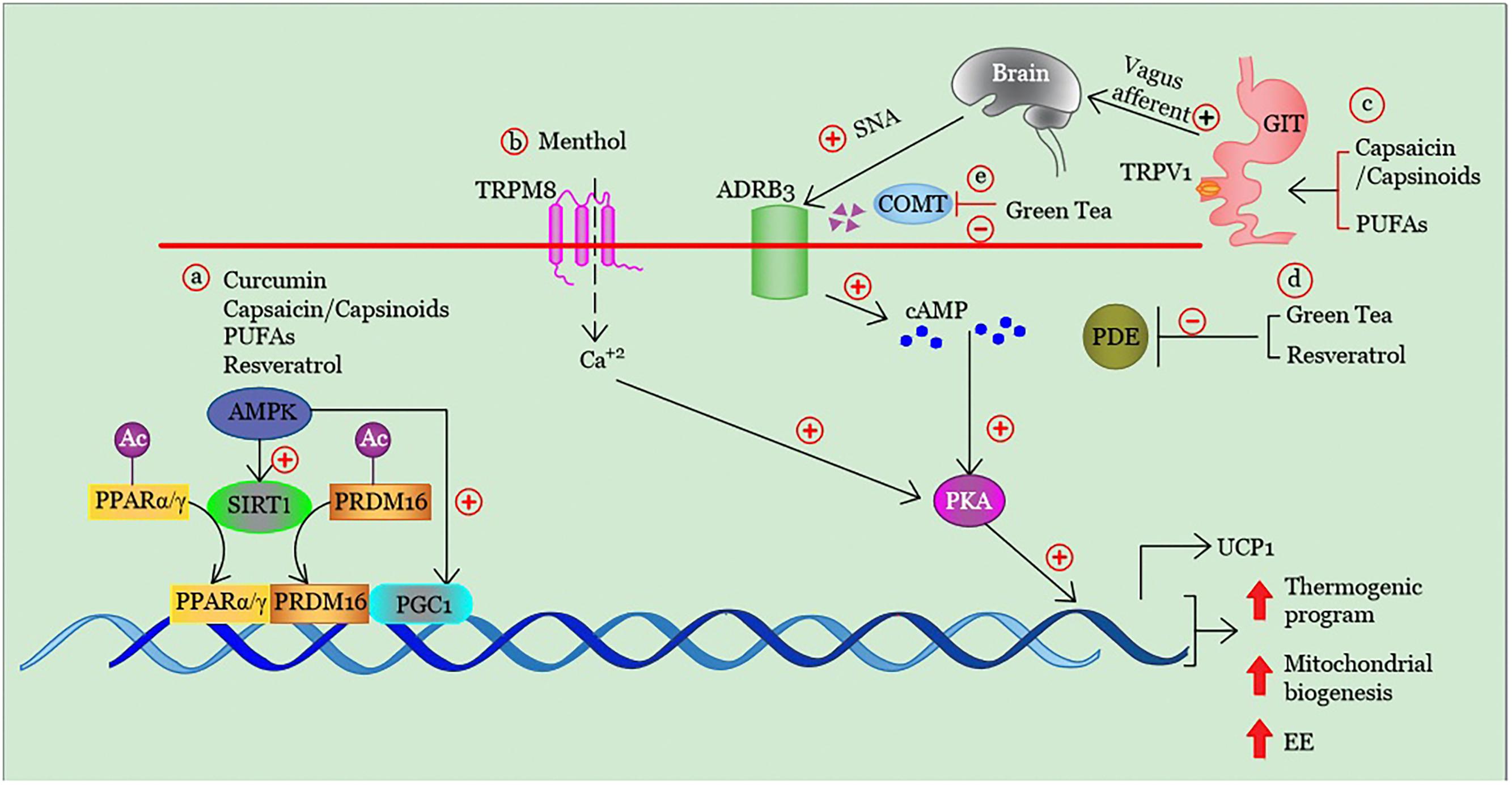
Figure 3. Summary of the mechanisms involved in the stimulation of brown adipogenesis, mitochondrial biogenesis and energy expenditure by some dietary molecules. (a) The direct and/ or indirect (via AMPK) activation of SIRT1 induces deacetylation and interaction of key transcription factors promoting brown and beige adipogenesis as PPARα/γ and PRDM16. The PPAR/PRDM16 complex is also able to bind and activate PGC1α, another cofactor specifically expressed in brown and beige adipocytes that stimulates the transcription of several genes involved in thermogenesis and mitochondrial biogenesis. Similarly, AMPK can also directly enhance PGC1α activity by phosphorylation, thus increasing mitochondrial biogenesis. (b) TRPM8 activation in brown adipocytes enhances the expression of thermogenic genes via Ca2+-dependent PKA signaling pathway. (c) Activation TRPV1 receptors in GIT, and consequent stimulation of the vagal afferent pathways leads to activation of neurons within the ventromedial hypothalamus. This mechanism of action induces a cold-independent adrenergic response that mediates brown adipogenesis. The adrenergic stimulation in brown adipocytes can be also promoted by the reduction of degradation of (d) cAMP and (e) norepinephrine through direct inhibition of PDEs and COMT activity, respectively. TRPM8, transient receptor potential cation channel melastatin 8; UCP1, uncoupling protein 1; TRPV1, transient receptor potential vanilloid 1; SNA, sympathetic nerve activity; AMPK, adenosine monophosphate-activated protein kinase, SIRT1, sirtuin-1; PGC-1α, peroxisome proliferator-activated receptor gamma coactivator 1-alpha; COMT, catechol-O-methyl-transferase cAMP; cyclic adenosine monophosphate; PDEs, phosphodiesterases; PUFAs, polyunsaturated fatty acids; Ac, acetyl group; cAMP, cyclic adenosine monophosphate; EE, energy expenditure; PPARα/γ peroxisome proliferator-activated receptor alpha/gamma; PKA, protein kinase A, PRDM16; PR-domain containing 16, (+), stimulation; (–), inhibition; ↑, increase.
Capsaicin and Capsinoids
Capsaicin (8-methyl-N-vanillyl-6-nonenamide) is an active component of hot pepper and belongs to the capsicum genus of plants. This alkaloid is responsible for the pungency and hotness sensation of chili peppers (Thiele et al., 2008). Capsinoids, which include dihydrocapsiate, nordihydrocapsiate, and capsiate, have the same chemical structure as capsaicin. They are active ingredients found in the non-pungent type of red chili (Kobata et al., 1999). Both capsaicin and capsinoids have elicited enormous interest due to their role in enhancing fat oxidation and EE (Ludy et al., 2012). The oral treatment with capsinoids (6 mg/kg for 12 weeks) in overweight or obese subjects was associated with abdominal fat loss and increase in fat oxidation compared with placebo group (Snitker et al., 2009). Moreover, Lejeune et al. (2003) showed that capsaicin treatment (135 mg per day) in overweight subjects induced elevation of the resting EE. In another randomized placebo-controlled study, capsinoids (10 mg/kg per day) were able to increase EE, VO2, and enhance fat burning (Inoue et al., 2007). The thermogenic and anti-obesity effects of capsaicin and capsinoids have been shown to be mediated, at least in part, by the promotion of BAT activity. Yoneshiro et al. (2012) assessed the short-term effects of dietary supplementation of capsinoids (9 mg) on EE and BAT activity in healthy males. BAT activity was assessed by [18F]- FDG-PET. The authors demonstrated a significant increase of EE (by 15.2 kJ/h) in subjects with active BAT. On the other hand, only a slight increase in EE (by 1.7 kJ/h) after oral administration of capsinoids was observed in the BAT-negative group (Yoneshiro et al., 2012).
The intragastric administration of capsaicin analog in rats induced an increase in SNA of BAT via activation of TRPV1 channels expressed along the gastrointestinal tract (Ono et al., 2011). These findings were supported by another study that showed a TRPV1-dependent increase in the temperature of the colon and intrascapular BAT after jejunal administration of capsinoids in mice. This effect was inhibited by an extrinsic denervation of the jejunal segment suggesting a role of the gastrointestinal vagus nerve in capsinoids-mediated thermogenesis (Kawabata et al., 2009). In a recent report, a combination of mild cold exposure and capsinoids in C57BL/6 mice has been shown to promote the development of both brown and beige adipocytes in inguinal WAT suggesting a centrally mediated effect of capsinoids. Capsinoids interact with TRPV1 receptors in gut, which in turn stimulate the vagal afferent pathways leading to activation of neurons within the ventromedial hypothalamus. This mechanism of action induces a cold-independent adrenergic response in WAT, leading to an increase in PRDM16 levels and stability. Therefore, the two β-adrenergic pathways mediate synergically the capsinoids-induced beige adipocyte biogenesis (Ohyama et al., 2016).
Moreover, Baskaran et al. (2016) showed that capsaicin triggered browning of WAT by promoting the expression of SIRT1, UCP1, BMP8B, and PPARγ, PGC-1α in white adipocytes. SIRT1 is a NAD+- dependent deacetylase of several transcription factors including PGC-1α, hence modulating oxidative mitochondrial metabolism (Cantó and Auwerx, 2009). In white adipocytes, SIRT1 also induces deacetylation and interaction of PPARγ and PR-domanin containing 16 (PRDM16) and thereby regulates key factors involved in BAT development (Qiang et al., 2012). Despite the fact that the direct mechanism by which capsaicin and its derivatives activate BAT has been demonstrated in animal models, much work is still to be done in humans.
Resveratrol
Resveratrol (trans-3,5,4′-trihydroxystilben) is a natural polyphenol that has attracted a lot of research interests mainly in the field of obesity management (Kim and Park, 2011). This compound was first found in the roots of white hellebore, and then in mulberries, red wine, grapes and peanuts (Burns et al., 2002).
The administration of resveratrol (400 mg/kg/day for 10 weeks) in mice fed with HFD has shown to reduce visceral fat-pad weights and suppress adipogenesis in epididymal white adipocytes (Kim et al., 2011). Furthermore, Rayalam et al. (2008) provided additional evidence for the inhibitory effects of resveratrol on adipogenesis. Resveratrol reduced adipocytes viability and downregulated the expression of adipogenic factors such as SREBP-1c, LPL, C/EBP-α, HSL, and PPARγ. In addition, resveratrol upregulated genes involved in mitochondrial biogenesis such as mitofusin (Mfn)-2 and UCP1 (Rayalam et al., 2008).
Low concentrations of resveratrol have been shown to promote the expression of brown adipogenic markers including UCP1, PRDM16, PGC1α and CIDEA in stromal vascular cells isolated from mouse inguinal WAT, suggesting a main role of resveratrol in WAT browning (Wang et al., 2015a). Similar findings were observed in stromal primary vascular cells separated from interscapular BAT after treatment by resveratrol in vitro (Wang et al., 2017). The browning effect of resveratrol has been suggested to be mediated by 5′ adenosine monophosphate-activated protein kinase (AMPK) α1 activation, since these changes wereabsent in cells lacking AMPKα1 (Wang et al., 2015a, 2017).
In C57BL/6J mice, the ingestion of HFD supplemented with resveratrol for 15 weeks led to an increase in adaptive thermogenesis in response to external cooling. Moreover, BAT of resveratol-treated mice showed larger mitochondria structures and DNA content, significant increase in gene expression of pathways characteristically related to energy homeostasis, including PPARα involved β-oxidation of fatty acids and UCP1. These effects were combined with increased gene expression of SIRT1 enhancing subsequently PGC-1α activity in brown adipocytes (Lagouge et al., 2006). In this report, the authors suggested a potential contribution of SIRT1-mediated deacytelation of PGC-1α in BAT activation (Lagouge et al., 2006; Cantó and Auwerx, 2009). Similarly, Andrade et al. (2014) showed that feeding mice a standard diet plus resveratrol induced an expression of SIRT1 and UCP1 genes in BAT. Also, resveratrol effects included an increased expression of other genes, such as PTEN, which promotes EE, and Bmp7, which is known to play a central role in brown fat development and differentiation (Tseng et al., 2008).
Taken together, these results suggest that resveratrol plays a crucial role in brown adipocytes formation and activation by enhancing the AMPK–SIRT1–PGC-1α signaling pathway. It has been hypothesized that resveratrol can exert a direct stimulatory effect on SIRT1 (Price et al., 2012), whereas other data indicate an indirect activation via AMP (Wang et al., 2015a). To this regard, it has been reported that resveratrol can directly inhibit cyclic AMP (cAMP)-specific PDEs in skeletal muscle and WAT of mice leading to elevated intracellular cAMP levels. The activation of EPAC, a cAMP effector, leads to increased intracellular calcium (Ca2+) concentration that activates AMPK pathway and subsequently increase SIRT1 activity. However, the effect of this resveratrol-mediated by cAMP signaling pathway on brown adipogenesis remains to be elucidated (Park et al., 2012). In contrast to animal studies, there is a lack of evidence whether resveratrol can affect WAT browning or BAT activation in humans.
Curcumin
Curcumin, also called diferuloylmethane, is a yellow-colored hydrophobic polyphenol found in extracts of Turmeric roots (a plant of the ginger family). Curcumin is commonly used as a spice in cooking and has been recognized for its potential value as an anti-obesity agent (Mantzorou et al., 2018). A recent clinical trial assessed the safety and effectiveness of 30 day treatment with curcumin combined with phosphatidylserine in overweight subjects undergoing weight loss by diet and lifestyle intervention. In this study, curcumin administration increased weight loss, enhanced the fat mass loss and induced a reduction in waist and hip circumference (Di Pierro et al., 2015).
Wang et al. (2015b) demonstrated that intra-gastric administration of curcumin (50 or 100 mg/kg daily) in mice for 50 days induced a decrease in fat mass and body weight without altering food intake. After cold challenge (exposure to 4°C), mice treated with curcumin exhibited an increase in adaptive thermogenesis, blood norepinephrine concentrations, and expression of characteristic BAT genes (e.g., ADRB3, CIDEA, PRDM 16, PGC-1α, and UCP1) in inguinal WAT (Wang et al., 2015b). In another study, Lone et al. (2016) added evidence on the effect of dietary curcumin (10–20 μM/day) in inducing a beige phenotype in white adipocytes from rats. These changes were accompanied by increased gene expression of brown fat markers such as FGF21, Tbx1, TMEM26, and CIDEA and some brown cell marker proteins including PRDM16, UCP1 and PGC-1α in a dose-dependent manner. These curcumin-induced browning effects have been shown to be mediated via the activation of AMPK-pathway (Lone et al., 2016).
In a recent study, mice fed with HFD in association with curcumin showed an increase in EE and adaptive thermogenesis following mild cold exposure. Within this study, curcumin treatment was associated with increased UCP1 expression in BAT, possibly involving PPAR-dependent and independent mechanisms (Song et al., 2018).
A common feature in these animal and human studies was the administration of high doses of curcumin. This was justified by the low systemic bioavailability of oral curcumin which also can be a reason of non-guaranteed positive results (Pan et al., 1999; Ireson et al., 2001). To this regard, Nishikawa et al. (2018) demonstrated that administration of low doses of curcumin formulations but having higher bioavailability compared to native curcumin, enhanced in vivo WAT-browning and increased EE in mice. These effects were induced via norepinephrine production by alternatively activated macrophages in WAT (Nishikawa et al., 2018).
Green Tea
Green tea is a widely consumed beverage extracted from leaves of Camellia Sinensis. Several reports indicated that green tea may induce weight loss by enhancing EE and fat oxidation in humans (Westerterp-Plantenga et al., 2006). These beneficial effects are, at least in part, attributable to tea catechins such as EGCG which is the most active catechin in green tea, epigallocatechin, and epicatechin gallate (Basu and Lucas, 2007). Interestingly, green tea extracts have substantial amounts of caffeine which is known for its thermogenic properties (Westerterp-Plantenga et al., 2006). Tea catechins intake in rats fed with normal-fat diet induced an increase in BAT UCP1 expression and a loss in WAT mass (Nomura et al., 2008). Chen et al. (2017) have shown that oral administration by gavage of green tea for 8 weeks improved obesity-related parameters and upregulated the expression of BAT markers (i.e., PPAR-γ, PRDM-16, and PGC-1α) in WAT of rats fed with a HFD-diet.
With regard to human studies, Dulloo et al. (1999) demonstrated that green tea enhances EE and fat oxidation. In this study, the administration of equivalent amounts of caffeine found in green tea extracts failed to induce similar metabolic effects (Dulloo et al., 1999). However, catechins and caffeine may synergically mediate an adrenergic-induced BAT thermogenesis by acting at different check-points of the norepinephrine-cAMP axis. It was suggested that green tea catechins may promote the SNA by reducing the degradation of norepinephrine through a direct inhibition of COMT. Interestingly, caffeine may synergically prolong the effects of norepinephrine by direct inhibition of PDEs activity (Dulloo et al., 1999, 2000). The synergistic thermogenic effect exerted by EGCG and caffeine was further confirmed after administration of encapsulated EGCG-caffeine mixtures (Bérubé-Parent et al., 2005).
However, the role of green tea in tackling obesity seemed controversial in several human trials (Huang et al., 2014). It was hypothesized that these findings might be influenced by the body composition, dietary habits and ethnicity of the studied populations (Huang et al., 2014). In addition, most studies aimed to assess the impact of green tea catechins on fat oxidation rather than thermogenesis (Rains et al., 2011), therefore more studies are warranted to elucidate the role of green tea in the activation and recruitment of BAT in humans.
Menthol
Menthol (2-isopropyl-5-methyl-cyclohexanol) also known as mint camphor, is a cyclic monoterpene alcohol produced synthetically or obtained from peppermint Mentha piperita (Patel et al., 2007; Pergolizzi et al., 2018). For centuries, menthol has found application in medical field due to its promising biological properties including antitussive, anti-inflammatory, antipruritic, antibacterial and analgesic effects (Patel et al., 2007). Menthol is also known to induce cooling sensation by activating the TRPM8 receptor, a Ca2+-permeable non-selective channel that detects cold stimuli in the thermosensory system (McKemy et al., 2002; Bautista et al., 2007). TRPM8 is also expressed in significant amounts in the lungs, male reproductive system and cancerous tissues where its function is yet not well understood (Tsavaler et al., 2001; Stein et al., 2004). Recent studies have demonstrated TRPM8 expression on the membrane of brown and white adipocytes (Ma et al., 2012; Rossato et al., 2014; Jiang et al., 2017).
Long-term administration of menthol in mice has been shown to enhance UCP1 expression and activity in BAT, increase EE, ameliorate insulin sensitivity and prevent HFD-induced weight gain. These effects have shown to be mediated by TRPM8 activation in brown adipocytes with consequent Ca2+-dependent PKA phosphorylation (Ma et al., 2012). Similarly, Jiang et al. (2017) reported that TRPM8 is also expressed on cultured white adipocytes from mice and its activation by menthol enhanced the expression of thermogenic genes (UCP1, PGC1α) via PKA signaling pathway in white adipocytes. Interestingly, menthol administration protected mice against HFD-obesity and enhanced beige adipocytes (Jiang et al., 2017).
In addition, TRPM8 activation in vitro by menthol in human white adipocytes induced a brown-like phenotype, stimulated UCP1 expression and adipocyte thermogenesis (Rossato et al., 2014). Moreover, topical application of menthol on skin has been shown to activate TRPM8 and induce parallel increase in NST and body temperature (Tajino et al., 2007; Vizin et al., 2018).
Taken together, these findings reinforce evidence about the prospective use of menthol as a promising approach in the management of obesity and associated comorbidities by regulating energy balance and metabolic homeostasis.
Fish-Derived Omega-3 Fatty Acids
Omega-3 PUFAs such as DHA and EPA are major polyunsaturated fats found fish oil supplements and in fatty fish such as salmon (Anderson and Ma, 2009). The supplementation of fish oil had shown to increase UCP1 expression (Takahashi and Ide, 2000) and protein levels in interscapular BAT of rats (Kawada et al., 1998). Recently, dietary n-3 PUFAs has shown to lower the amount of visceral WAT and increase the mass of interscapular BAT in rats (Crescenzo et al., 2017).
It has been shown that fish oil increases the expression of numerous thermogenic genes in BAT including β3 ADRB3, PRDM16, PGC1α and PPARs in BAT (Bargut et al., 2016; Pahlavani et al., 2017). In addition, in inguinal WAT fish oil exerts its browning effects by recruiting beige adipocytes. In another study, Kim et al. (2015) added evidence that fish oil supplementation in rats for 10 weeks induced a recruitment of beige adipocytes by increasing the expression of UCP1, PGC1α, CIDEA and PRDM16 in inguinal WAT. The authors suggested that fish oil contributes to brown adipogenesis by acting as ligand of TRPV1 in digestive tract that triggers, via the brain, a β2-adrenergical sympathetic response in adipose depots.
Furthermore, Laiglesia et al. (2016) have recently shown that EPA induces a switch from white to beige-like adipocytes in human subcutaneous adipocytes of overweight subjects by promoting the activation of AMPK/SIRT1/PGC1- α axis. However, despite promising data from murine studies, little is known about the thermogenic activity of n-3 PUFAs in humans.
Conclusion
The recent discovery of metabolically active BAT in adult humans has raised the expectations for the development of novel anti-obesity treatments that can regulate brown or beige fat development. In this review we focused on few dietary molecules that have shown to regulate BAT activation or beige fat development. Despite the promising data from animal models or cell lines, these findings need to be validated in humans by further large clinical trials with relatively long-term period of follow-up and taking in consideration factors such as ethnicity, genetics and lifestyles. Moreover, current knowledge deriving from cell culture and animal models suggests that polyphenols, mainly curcumin, and resveratrol, exert their thermogenic effect when supplemented at doses that are quite elevated. Therefore, further research is warranted to define the optimal preparation, doses as well as the bioavailability and safety of these molecules in humans.
Finally, the above discussed dietary components have been shown to share common molecular targets involved in the induction of brown adipogenesis. Then future studies should test the hypothesis whether combined supplementations may also operate synergistically to activate NST in human through activation of BAT and/or beige adipose tissue recruitment.
Author Contributions
HE wrote the manuscript. MR proofread the manuscript and provided guidance on the overall direction of the manuscript. All authors critically appraised the final version of the paper.
Funding
This project has received funding from the European Union’s Horizon 2020 research and innovation program under the Marie Skłodowska-Curie Grant Agreement No. 691061.
Abbreviations
ADRB3, β3-adrenergic receptor; AMPK, 5′adenosine monophosphate-activated protein kinase; ATP, adenosine triphosphate; BAT, brown adipose tissue; BMP8B, bone morphogenetic protein 8b; C/EBP-α, CCAAT-enhancer binding protein alpha; cAMP, cyclic adenosine monophosphate; CD137, member of tumor necrosis factor receptor; CIDEA, cell death-inducing DFFA-like effector a; COMT, catechol-O-methyl-transferase; CVDs, cardiovascular diseases; DHA, docosahexaenoic acid; EE, energy expenditure; EGCG, epigallocatechin gallate; EPA, eicosapentaenoic acid; EPAC, exchange protein directly activated by cAMP; FAS, Death receptor; FDG-PET [18F], fluorodeoxyglucose–positron emission tomography; FGF21, fibroblast growth factor 21; HFD, high-fat diet; HSL, hormone-sensitive lipase; LPL, lipoprotein lipase; Mfn2, mitofusin 2; mRNA, messenger RNA; NAD+, nicotinamide adenine dinucleotide; NST, non-shivering thermogenesis; PDEs, phosphodiesterases; PGC-1α, peroxisome proliferator-activated receptor gamma coactivator 1-alpha; PKA, protein kinase A; PPARγ, peroxisome proliferator-activated receptor gamma; PRDM16, PR-domain containing 16; PTEN, phosphatase and tensin homolog; PUFAs, polyunsaturated fatty acids; SIRT1, sirtuin-1; SNA, sympathetic nerve activity; SREBP-1c, sterol regulatory element binding protein-1c; Tbx1, T-box transcription factor 1; TMEM26, transmembrane protein 26; TRPM8, transient receptor potential cation channel melastatin 8; TRPV1, transient receptor potential vanilloid 1; UCP1, uncoupling protein 1; VO2, oxygen consumption; WAT, white adipose tissue.
Conflict of Interest Statement
The authors declare that the research was conducted in the absence of any commercial or financial relationships that could be construed as a potential conflict of interest.
References
Amiot, M. J., Riva, C., and Vinet, A. (2016). Effects of dietary polyphenols on metabolic syndrome features in humans: a systematic review. Obes. Rev. 17, 573–586. doi: 10.1111/obr.12409
Anderson, B. M., and Ma, D. W. (2009). Are all n-3 polyunsaturated fatty acids created equal? Lipids Health Dis. 8:33. doi: 10.1186/1476-511X-8-33
Andolfi, C., and Fisichella, P. M. (2018). Epidemiology of obesity and associated comorbidities. J. Laparoendosc. Adv. Surg. Tech. A 28, 919–924. doi: 10.1089/lap.2018.0380
Andrade, J. M., Frade, A. C., Guimarães, J. B., Freitas, K. M., Lopes, M. T., Guimarães, A. L., et al. (2014). Resveratrol increases brown adipose tissue thermogenesis markers by increasing SIRT1 and energy expenditure and decreasing fat accumulation in adipose tissue of mice fed a standard diet. Eur. J. Nutr. 53, 1503–1510. doi: 10.1007/s00394-014-0655-6
Bargut, T. C., Silva-e-Silva, A. C., Souza-Mello, V., Mandarim-de-Lacerda, C. A., and Aguila, M. B. (2016). Mice fed fish oil diet and upregulation of brown adipose tissue thermogenic markers. Eur. J. Nutr. 55, 159–169. doi: 10.1007/s00394-015-0834-0
Bartelt, A., and Heeren, J. (2014). Adipose tissue browning and metabolic health. Nat. Rev. Endocrinol. 10, 24–36. doi: 10.1038/nrendo.2013.204
Baskaran, P., Krishnan, V., Ren, J., and Thyagarajan, B. (2016). Capsaicin induces browning of white adipose tissue and counters obesity by activating TRPV1 channel-dependent mechanisms. Br. J. Pharmacol. 173, 2369–2389. doi: 10.1111/bph.13514
Basu, A., and Lucas, E. A. (2007). Mechanisms and effects of green tea on cardiovascular health. Nutr. Rev. 65, 361–375. doi: 10.1111/j.1753-4887.2007.tb00314.x
Bautista, D. M., Siemens, J., Glazer, J. M., Tsuruda, P. R., Basbaum, A. I., Stucky, C. L., et al. (2007). The menthol receptor TRPM8 is the principal detector of environmental cold. Nature 448, 204–208. doi: 10.1038/nature05910
Bérubé-Parent, S., Pelletier, C., Doré, J., and Tremblay, A. (2005). Effects of encapsulated green tea and guarana extracts containing a mixture of epigallocatechin-3-gallate and caffeine on 24 h energy expenditure and fat oxidation in men. Br. J. Nutr. 94, 432–436. doi: 10.1079/BJN20051502
Burns, J., Yokota, T., Ashihara, H., Lean, M. E., and Crozier, A. (2002). Plant foods and herbal sources of resveratrol. J. Agric. Food Chem. 50, 3337–3340. doi: 10.1021/jf0112973
Cantó, C., and Auwerx, J. (2009). PGC-1alpha, SIRT1 and AMPK, an energy sensing network that controls energy expenditure. Curr. Opin. Lipidol. 20, 98–105. doi: 10.1097/MOL.0b013e328328d0a4
Chen, L. H., Chien, Y. W., Liang, C. T., Chan, C. H., Fan, M. H., and Huang, H. Y. (2017). Green tea extract induces genes related to browning of white adipose tissue and limits weight-gain in high energy diet-fed rat. Food Nutr. Res. 61:1347480. doi: 10.1080/16546628.2017.1347480
Crescenzo, R., Mazzoli, A., Cancelliere, R., Bianco, F., Giacco, A., Liverini, G., et al. (2017). Polyunsaturated fatty acids stimulate de novo lipogenesis and improve glucose homeostasis during refeeding with high fat diet. Front. Physiol. 8:178. doi: 10.3389/fphys.2017.00178
Di Pierro, F., Bressan, A., Ranaldi, D., Rapacioli, G., Giacomelli, L., and Bertuccioli, A. (2015). Potential role of bioavailable curcumin in weight loss and omental adipose tissue decrease: preliminary data of a randomized, controlled trial in overweight people with metabolic syndrome. Preliminary study. Eur. Rev. Med. Pharmacol. Sci. 19, 4195–4202.
Dulloo, A. G., Duret, C., Rohrer, D., Girardier, L., Mensi, N., Fathi, M., et al. (1999). Efficacy of a green tea extract rich in catechin polyphenols and caffeine in increasing 24-h energy expenditure and fat oxidation in humans. Am. J. Clin. Nutr. 70, 1040–1045. doi: 10.1093/ajcn/70.6.1040
Dulloo, A. G., Seydoux, J., Girardier, L., Chantre, P., and Vandermander, J. (2000). Green tea and thermogenesis: interactions between catechin-polyphenols, caffeine and sympathetic activity. Int. J. Obes. Relat. Metab. Disord. 24, 252–258. doi: 10.1038/sj.ijo.0801101
Huang, J., Wang, Y., Xie, Z., Zhou, Y., Zhang, Y., and Wan, X. (2014). The anti-obesity effects of green tea in human intervention and basic molecular studies. Eur. J. Clin. Nutr. 68, 1075–1087. doi: 10.1038/ejcn.2014.143
Inoue, N., Matsunaga, Y., Satoh, H., and Takahashi, M. (2007). Enhanced energy expenditure and fat oxidation in humans with high BMI scores by the ingestion of novel and non-pungent capsaicin analogues (capsinoids). Biosci. Biotechnol. Biochem. 71, 380–389. doi: 10.1271/bbb.60341
Ireson, C., Orr, S., Jones, D. J., Verschoyle, R., Lim, C. K., Luo, J. L., et al. (2001). Characterization of metabolites of the chemopreventive agent curcumin in human and rat hepatocytes and in the rat in vivo, and evaluation of their ability to inhibit phorbol ester-induced prostaglandin E2 production. Cancer Res. 61, 1058–1064.
Jiang, C., Zhai, M., Yan, D., Li, D., Li, C., Zhang, Y., et al. (2017). Dietary menthol-induced TRPM8 activation enhances WAT “browning” and ameliorates diet-induced obesity. Oncotarget 8, 75114–75126. doi: 10.18632/oncotarget.20540
Kawabata, F., Inoue, N., Masamoto, Y., Matsumura, S., Kimura, W., Kadowaki, M., et al. (2009). Non-pungent capsaicin analogs (capsinoids) increase metabolic rate and enhance thermogenesis via gastrointestinal TRPV1 in mice. Biosci. Biotechnol. Biochem. 73, 2690–2697. doi: 10.1271/bbb.90555
Kawada, T., Kayahashi, S., Hida, Y., Koga, K., Nadachi, Y., and Fushiki, T. (1998). Fish (Bonito) oil supplementation enhances the expression of uncoupling protein in brown adipose tissue of rat. J. Agric. Food Chem. 46, 1225–1227. doi: 10.1021/jf9711000
Khera, R., Murad, M. H., Chandar, A. K., Dulai, P. S., Wang, Z., Prokop, L. J., et al. (2016). Association of pharmacological treatments for obesity with weight loss and adverse events: a systematic review and meta-analysis. JAMA 315, 2424–2434. doi: 10.1001/jama.2016.7602
Kiefer, F. W. (2017). The significance of beige and brown fat in humans. Endocr. Connect. 6, R70–R79. doi: 10.1530/EC-17-0037
Kim, K. H., and Park, Y. (2011). Food components with anti-obesity effect. Annu. Rev. Food Sci. Technol. 2, 237–257. doi: 10.1146/annurev-food-022510-133656
Kim, M., Goto, T., Yu, R., Uchida, K., Tominaga, M., Kano, Y., et al. (2015). Fish oil intake induces UCP1 upregulation in brown and white adipose tissue via the sympathetic nervous system. Sci. Rep. 5:18013. doi: 10.1038/srep18013
Kim, S., Jin, Y., Choi, Y., and Park, T. (2011). Resveratrol exerts anti-obesity effects via mechanisms involving down-regulation of adipogenic and inflammatory processes in mice. Biochem. Pharmacol. 81, 1343–1351. doi: 10.1016/j.bcp.2011.03.012
Kobata, K., Sutoh, K., Todo, T., Yazawa, S., Iwai, K., and Watanabe, T. (1999). Nordihydrocapsiate, a new capsinoid from the fruits of a nonpungent pepper, Capsicum annuum. J. Nat. Prod. 62, 335–336. doi: 10.1021/np9803373
Lagouge, M., Argmann, C., Gerhart-Hines, Z., Meziane, H., Lerin, C., Daussin, F., et al. (2006). Resveratrol improves mitochondrial function and protects against metabolic disease by activating SIRT1 and PGC-1alpha. Cell 15, 1109–1122. doi: 10.1016/j.cell.2006.11.013
Laiglesia, L. M., Lorente-Cebrián, S., Prieto-Hontoria, P. L., Fernández-Galilea, M., Ribeiro, S. M., Sáinz, N., et al. (2016). Eicosapentaenoic acid promotes mitochondrial biogenesis and beige-like features in subcutaneous adipocytes from overweight subjects. J. Nutr. Biochem. 37, 76–82. doi: 10.1016/j.jnutbio.2016.07.019
Lejeune, M. P., Kovacs, E. M., and Westerterp-Plantenga, M. S. (2003). Effect of capsaicin on substrate oxidation and weight maintenance after modest body-weight loss in human subjects. Br. J. Nutr. 90, 651–659. doi: 10.1079/BJN2003938
Lone, J., Choi, J. H., Kim, S. W., and Yun, J. W. (2016). Curcumin induces brown fat-like phenotype in 3T3-L1 and primary white adipocytes. J. Nutr. Biochem. 27, 193–202. doi: 10.1016/j.jnutbio.2015.09.006
Ludy, M. J., Moore, G. E., and Mattes, R. D. (2012). The effects of capsaicin and capsiate on energy balance: critical review and meta-analyses of studies in humans. Chem. Senses 37, 103–121. doi: 10.1093/chemse/bjr100
Ma, S., Yu, H., Zhao, Z., Luo, Z., Chen, J., Ni, Y., et al. (2012). Activation of the cold-sensing TRPM8 channel triggers UCP1-dependent thermogenesis and prevents obesity. J. Mol. Cell Biol. 4, 88–96. doi: 10.1093/jmcb/mjs001
Mantzorou, M., Pavlidou, E., Vasios, G., Tsagalioti, E., and Giaginis, C. (2018). Effects of curcumin consumption on human chronic diseases: a narrative review of the most recent clinical data. Phytother. Res. 32, 957–975. doi: 10.1002/ptr.6037
Martínez-González, M. A., Salas-Salvadó, J., Estruch, R., Corella, D., Fitó, M., Ros, E., et al. (2015). Benefits of the mediterranean diet: insights from the PREDIMED study. Prog. Cardiovasc. Dis. 58, 50–60. doi: 10.1016/j.pcad.2015.04.003
McKemy, D. D., Neuhausser, W. M., and Julius, D. (2002). Identification of a cold receptor reveals a general role for TRP channels in thermosensation. Nature 416, 52–58. doi: 10.1038/nature719
Nishikawa, S., Kamiya, M., Aoyama, H., Nomura, M., Hyodo, T., Ozeki, A., et al. (2018). Highlydispersible and bioavailable curcumin but not native curcumin induces brown-like adipocyte formation in mice. Mol. Nutr. Food Res. 62:1700131. doi: 10.1002/mnfr.201700731
Nomura, S., Ichinose, T., Jinde, M., Kawashima, Y., Tachiyashiki, K., and Imaizumi, K. (2008). Tea catechins enhance the mRNA expression of uncoupling protein 1 in rat brown adipose tissue. J. Nutr. Biochem. 19, 840–847. doi: 10.1016/j.jnutbio.2007.11.005
Ohyama, K., Nogusa, Y., Shinoda, K., Suzuki, K., Bannai, M., and Kajimura, S. (2016). A synergistic antiobesity effect by a combination of capsinoids and cold temperature through promoting beige adipocyte biogenesis. Diabetes Metab. Res. Rev. 65, 1410–1423. doi: 10.2337/db15-0662
Ono, K., Tsukamoto-Yasui, M., Hara-Kimura, Y., Inoue, N., Nogusa, Y., Okabe, Y., et al. (2011). Intragastric administration of capsiate, a transient receptor potential channel agonist, triggers thermogenic sympathetic responses. J. Appl. Physiol. 110, 789–798. doi: 10.1152/japplphysiol.00128.2010
Pahlavani, M., Razafimanjato, F., Ramalingam, L., Kalupahana, N. S., Moussa, H., Scoggin, S., et al. (2017). Eicosapentaenoic acid regulates brown adipose tissue metabolism in high-fat-fed mice and in clonal brown adipocytes. J. Nutr. Biochem. 39, 101–109. doi: 10.1016/j.jnutbio.2016.08.012
Pan, M. H., Huang, T. M., and Lin, J. K. (1999). Biotransformation of curcumin through reduction and glucuronidation in mice. Drug Metab. Dispos. 27, 486–494.
Park, S. J., Ahmad, F., Philp, A., Baar, K., Williams, T., Luo, H., et al. (2012). Resveratrol ameliorates aging-related metabolic phenotypes by inhibiting cAMP phosphodiesterases. Cell 3, 421–433. doi: 10.1016/j.cell.2012.01.017
Patel, T., Ishiuji, Y., and Yosipovitch, G. (2007). Menthol: a refreshing look at this ancient compound. J. Am. Acad. Dermatol. 57, 873–878. doi: 10.1016/j.jaad.2007.04.008
Pergolizzi, J. V. Jr., Taylor, R. Jr., LeQuang, J. A., Raffa, R. B., and Nema Research Group. (2018). The role and mechanism of action of menthol in topical analgesic products. J. Clin. Pharm. Ther. 43, 313–319. doi: 10.1111/jcpt.12679
Price, N. L., Gomes, A. P., Ling, A. J., Duarte, F. V., Martin-Montalvo, A., North, B. J., et al. (2012). SIRT1 is required for AMPK activation and the beneficial effects of resveratrol on mitochondrial function. Cell Metab. 15, 675–690. doi: 10.1016/j.cmet.2012.04.003
Qiang, L., Wang, L., Kon, N., Zhao, W., Lee, S., Zhang, Y., et al. (2012). Brown remodeling of white adipose tissue by SirT1-dependent deacetylation of Pparγ. Cell 150, 620–632. doi: 10.1016/j.cell.2012.06.027
Rains, T. M., Agarwal, S., and Maki, K. C. (2011). Antiobesity effects of green tea catechins: a mechanistic review. J. Nutr. Biochem. 22, 1–7. doi: 10.1016/j.jnutbio.2010.06.006
Rayalam, S., Yang, J. Y., Ambati, S., Della-Fera, M. A., and Baile, C. A. (2008). Resveratrol induces apoptosis and inhibits adipogenesis in 3T3-L1 adipocytes. Phytother. Res. 22, 1367–1371. doi: 10.1002/ptr.2503
Rosenwald, M., and Wolfrum, C. (2014). The origin and definition of brite versus white and classical brown adipocytes. Adipocyte 3, 4–9. doi: 10.4161/adip.26232
Rossato, M., Granzotto, M., Macchi, V., Porzionato, A., Petrelli, L., Calcagno, A., et al. (2014). Human white adipocytes express the cold receptor TRPM8 which activation induces UCP1 expression, mitochondrial activation and heat production. Mol. Cell. Endocrinol. 383, 137–146. doi: 10.1016/j.mce.2013.12.005
Snitker, S., Fujishima, Y., Shen, H., Ott, S., Pi-Sunyer, X., Furuhata, Y., et al. (2009). Effects of novel capsinoid treatment on fatness and energy metabolism in humans: possible pharmacogenetic implications. Am. J. Clin. Nutr. 89, 45–50. doi: 10.3945/ajcn.2008.26561
Song, Z., Revelo, X., Shao, W., Tian, L., Zeng, K., Lei, H., et al. (2018). Dietary curcumin intervention targets mouse white adipose tissue inflammation and brown adipose tissue UCP1 expression. Obesity 26, 547–558. doi: 10.1002/oby.22110
Stein, R. J., Santos, S., Nagatomi, J., Hayashi, Y., Minnery, B. S., Xavier, M., et al. (2004). Cool (TRPM8) and hot (TRPV1) receptors in the bladder and male genital tract. J. Urol. 172, 1175–1178. doi: 10.1097/01.ju.0000134880.55119.cf
Tajino, K., Matsumura, K., Kosada, K., Shibakusa, T., Inoue, K., Fushiki, T., et al. (2007). Application of menthol to the skin of whole trunk in mice induces autonomic and behavioral heat-gain responses. Am. J. Physiol. Regul. Integr. Comp. Physiol. 293, R2128–R2135. doi: 10.1152/ajpregu.00377.2007
Takahashi, Y., and Ide, T. (2000). Dietary n-3 fatty acids affect mRNA level of brown adipose tissue uncoupling protein 1, and white adipose tissue leptin and glucose transporter 4 in the rat. Br. J. Nutr. 84, 175–184.
Thiele, R., Mueller-Seitz, E., and Petz, M. (2008). Chili pepper fruits: presumed precursors of fatty acids characteristic for capsaicinoids. J. Agric. Food Chem. 56, 4219–4224. doi: 10.1021/jf073420h
Tsavaler, L., Shapero, M. H., Morkowski, S., and Laus, R. (2001). Trp-p8, a novel prostate-specific gene, is up-regulated in prostate cancer and other malignancies and shares high homology with transient receptor potential calcium channel proteins. Cancer Res. 61, 3760–3769.
Tseng, Y. H., Kokkotou, E., Schulz, T. J., Huang, T. L., Winnay, J. N., Taniguchi, C. M., et al. (2008). New role of bone morphogenetic protein 7 in brown adipogenesis and energy expenditure. Nature 454, 1000–1004. doi: 10.1038/nature07221
Virtanen, K. A., Lidell, M. E., Orava, J., Heglind, M., Westergren, R., Niemi, T., et al. (2009). Functional brown adipose tissue in healthy adults. N. Engl. J. Med. 360, 1518–1525. doi: 10.1056/NEJMoa0808949
Vizin, R. C. L., Motzko-Soares, A. C. P., Armentano, G. M., Ishikawa, D. T., Cruz-Neto, A. P., Carrettiero, D. C., et al. (2018). Short-term menthol treatment promotes persistent thermogenesis without induction of compensatory food consumption in wistar rats: implications for obesity control. J. Appl. Physiol. 124, 672–683. doi: 10.1152/japplphysiol.00770.2017
Wang, S., Liang, X., Yang, Q., Fu, X., Rogers, C. J., Zhu, M., et al. (2015a). Resveratrol induces brown-like adipocyte formation in white fat through activation of AMP-activated protein kinase (AMPK) α1. Int. J. Obes. 39, 967–976. doi: 10.1038/ijo.2015.23
Wang, S., Wang, X., Ye, Z., Xu, C., Zhang, M., Ruan, B., et al. (2015b). Curcumin promotes browning of white adipose tissue in a norepinephrine-dependent way. Biochem. Biophys. Res. Commun. 466, 247–253. doi: 10.1016/j.bbrc.2015.09.018
Wang, S., Liang, X., Yang, Q., Fu, X., Zhu, M., Rodgers, B. D., et al. (2017). Resveratrol enhances brown adipocyte formation and function by activating AMP-activated protein kinase (AMPK) α1 in mice fed high-fat diet. Mol. Nutr. Food. Res. 61:1600746. doi: 10.1002/mnfr.201600746
Westerterp-Plantenga, M., Diepvens, K., Joosen, A. M., Bérubé-Parent, S., and Tremblay, A. (2006). Metabolic effects of spices, teas, and caffeine. Physiol. Behav. 89, 85–91. doi: 10.1016/j.physbeh.2006.01.027
Keywords: brown adipose tissue, capsaicin, resveratrol, green tea, curcumin, menthol, Omega-3 polyunsaturated fatty acids
Citation: El Hadi H, Di Vincenzo A, Vettor R and Rossato M (2019) Food Ingredients Involved in White-to-Brown Adipose Tissue Conversion and in Calorie Burning. Front. Physiol. 9:1954. doi: 10.3389/fphys.2018.01954
Received: 04 November 2018; Accepted: 22 December 2018;
Published: 11 January 2019.
Edited by:
Anna Maria Giudetti, University of Salento, ItalyCopyright © 2019 El Hadi, Di Vincenzo, Vettor and Rossato. This is an open-access article distributed under the terms of the Creative Commons Attribution License (CC BY). The use, distribution or reproduction in other forums is permitted, provided the original author(s) and the copyright owner(s) are credited and that the original publication in this journal is cited, in accordance with accepted academic practice. No use, distribution or reproduction is permitted which does not comply with these terms.
*Correspondence: Marco Rossato, bWFyY28ucm9zc2F0b0B1bmlwZC5pdA==