- 1State Key Laboratory of Integrated Management of Pest Insects and Rodents, Institute of Zoology, Chinese Academy of Sciences, Beijing, China
- 2Centre for Behavioural and Physiological Ecology, Zoology, University of New England, Armidale, NSW, Australia
- 3Mammalian Ecology Laboratory, Institute of General and Experimental Biology, Mongolian Academy of Sciences, Ulaanbaatar, Mongolia
- 4University of the Chinese Academy of Sciences, Beijing, China
Most mammals and birds are altricial, small and naked at birth/hatching. They attain endothermic thermoregulation at a fraction of their adult size at a vulnerable stage with high heat loss when many could profit from using torpor for energy conservation. Nevertheless, detailed data on the interrelations between torpor expression and development of endothermic thermoregulation are currently restricted to <0.1% of extant endotherms. We investigated at what age and body mass (BM) desert hamsters (Phodopus roborovskii), wild-caught in Inner Mongolia and born in autumn/early winter when environmental temperatures in the wild begin to decrease, are able to defend their body temperature (Tb) at an ambient temperature (Ta) of ∼21°C and how soon thereafter they could express torpor. Measurements of surface temperatures via infrared thermometer and thermal camera show that although neonate hamsters (BM 0.9 ± 0.1 g) cooled rapidly to near Ta, already on day 15 (BM 5.5 ± 0.2 g) they could defend a high and constant Tb. As soon as day 16 (BM 5.8 ± 0.2 g), when their maximum activity metabolism (measured as oxygen consumption) approached maxima measured in vertebrates, animals were able to enter torpor for several hours with a reduction of metabolism by >90%, followed by endothermic arousal. Over the next weeks, torpor depth and duration decreased together with a reduction in resting metabolic rate at Ta 30–32°C. Our data show that development of endothermy and torpor expression in this altricial hamster is extremely fast. The results suggest that precocious torpor by juvenile hamsters in autumn and winter is an important survival tool in their vast and harsh Asian desert habitats, but likely also for many other small mammals and birds worldwide.
Introduction
Hibernation (multiday torpor) and daily torpor are used by many endothermic mammals and birds to reduce energy expenditure, often to deal with a shortage of food or adverse environmental conditions (Boyer and Barnes, 1999; Nowack et al., 2017). Torpor in these “heterothermic endotherms” is characterized by a controlled reduction of body temperature (Tb) from high normothermic values, typically ranging from about 36 to 41°C, to values usually between 0 and 29°C and a substantial decrease of metabolic rate (MR) by up to 99% (Boyer and Barnes, 1999; McKechnie and Mzilikazi, 2011; Ruf and Geiser, 2015; Dausmann and Warnecke, 2016).
Torpor expression is strongly affected by size. Torpor is more common in small than in large endotherms and, apparently to maximize energy savings during the torpid state, small species have on average lower minimum Tbs and their MR reductions are more pronounced (Ruf and Geiser, 2015). This seems to be to a large extent related to the fact that, when normothermic, small species have to compensate for high heat loss via a relative large surface area, have a limited capacity for fat storage and high costs for locomotion (Tucker, 1975; Calder, 1996). Moreover, because the lower critical temperature of the thermo-neutral zone (TNZ) of small species is often around 25 to 30°C and ambient temperature (Ta) experienced in the wild is generally below that, they typically have to produce heat endogenously because of the large Tb–Ta differential (Tattersall et al., 2012; Kronfeld-Schor and Dayan, 2013; Riek and Geiser, 2013; Withers et al., 2016; Mitchell et al., 2018).
Although almost all mammals and birds are competent endotherms as adults, the vast majority are altricial at birth or hatching. Altricial neonates or hatchlings are small, naked and uncoordinated and, when removed from the nest or away from their parents, cool rapidly to near ambient temperature (Ta) because they are only partially endothermic and unable to produce sufficient endogenous heat for maintenance of a high and constant Tb (Dawson and Evans, 1960; Morrison and Petajan, 1962; Holloway and Geiser, 2000); therefore they are often referred to as being poikilothermic (Greek “poikilo” = variable, “therme” = heat). As these animals grow to larger size and fur or feathers develop together with a maturation of the nervous and other organ systems, the ability for endogenous heat production and heat retention improves and eventually they are able to maintain a constant high Tb, at least during exposure to moderate Tas. These newly endothermic young, typically at about 20–50% of adult body mass, are highly vulnerable to heat and energy loss or even death by starvation when they maintain a high constant Tb at times the parents are unable to supply enough food and because of their inexperience in obtaining food on their own (Geiser, 2008).
Potentially, using torpor during periods of high heat loss and insufficient food supply would provide an avenue to enhance survival of young. However, although such energetic bottlenecks are likely faced occasionally or even frequently by the majority of small young mammals and bird species, as for example during bad weather, information on torpor during development is limited to only a few species (<15 species or <0.1% of the >15,000 mammals and bird species), including some small marsupial mammals, a few placental mammals, and a few birds (Nagel, 1977; Geiser, 2008; Eichhorn et al., 2011; Giroud et al., 2014; Wacker et al., 2017). For most of the studied species the rate of development is rather slow, and, especially in marsupials, for which most detailed data are available, first expression of torpor is observed only months after birth following a prolonged poikilothermic phase (Geiser et al., 2006, 2017; Wacker et al., 2017). The slow development in many species expressing torpor is supporting the view that heterothermic species often have a slow life history (Turbill et al., 2011), typically producing only 1 or 2 litters/young per year.
The purpose of our investigation was to provide the first data on the development of endothermy and torpor expression in small desert hamsters (Phodopus roborovskii; adult BM ∼25 g). This species is interesting with regard to its reproductive and developmental biology as it differs from most other species in which development of torpor has been investigated. It produces up to four litters of up to ten young per year, can breed within 2 months of birth, lives for only 1 or 2 years in the wild, and is clearly r-selected (Kolynchuk, 2015). Desert hamsters are distributed over the harsh deserts of continental Asia and, as adults, use daily torpor to aid survival in their environment (Chi et al., 2016). As they reproduce well into autumn, their young are potentially exposed to adverse environmental conditions and energy shortages in late autumn/early winter, and may require the use of energy savings mechanisms such as torpor to survive over winter into adulthood. We therefore tested the hypothesis that this fast-growing species is able to express torpor early during the growth phase, soon after endothermy is attained. Such data will improve the understanding of the functional significance of torpor especially in rapidly developing altricial mammals and the role of heterothermy during development in endotherms in general.
Materials and Methods
Animals
Litters of five female desert hamsters, captured in Inner Mongolia (Hunshandake sandy land, 43°11 ′N, 116°10 ′E) on the last week of August 2017, were used in our study. They were transported to the Chinese Academy of Sciences in Beijing on 1 September, where they were held individually in standard cages supplied with bedding (wood shavings and cotton wool), food (mouse pellets: crude protein ≥18%; crude fat ≥4%; coarse fiber ≤5%; ash ≤8%; moisture ≤10%, Beijing HFK Bio-Technology Co., Ltd., and Millet seeds) and water ad libitum under a LD 12:12 photoperiod and Ta 21 ± 1°C. This Ta was selected for maintenance and measurements because during the trapping month mean maximum Ta was 23.9 ± 3.1°C and the mean minimum Ta was 12.2 ± 3.2°C (China Meteorological Data Service Center) and because we wanted to ensure that the animals were not stressed, but nevertheless experienced a mild cold load during experiments. This study was carried out in accordance with the recommendations of Animal Care and Use Committee of Institute of Zoology, the Chinese Academy of Sciences. The protocol was approved by the Animal Care and Use Committee of Institute of Zoology, the Chinese Academy of Sciences.
Two females gave birth on 4 September (Pr1, 5 young; Pr2, 4 young). These individuals were used to determine rates of growth, rates of cooling from day 1 to day 15, torpor expression from day 16 to day 45, and resting metabolic rate (RMR) (Figure 1). Two females (Pr3 and 4) gave birth to litters of 5 young each on 7th September; 9 of these animals were used to measure RMR and torpor expression. An additional female (Pr 5) mated in captivity and gave birth to a litter of 5 young in December; 3 of these animals were also used to measure RMR and torpor expression. A total of 20 young were examined.
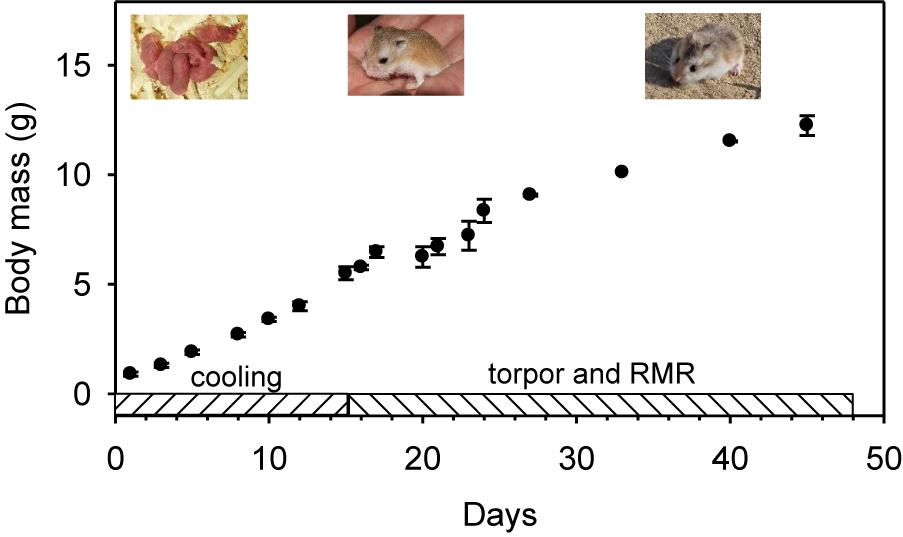
Figure 1. Experimental time-course and mean body mass with SD and pictures of developing desert hamsters at different ages.
Cooling Experiments
Rates of cooling in the two litters born on 4 September were measured from day 1. For these measurements, animals were removed from their nests and placed individually into cardboard beakers at the same Ta as in the holding cage (Ta 21 ± 1°C) and their surface temperature (Ts) measured non-invasively using two approaches. Maximum Ts was measured ventrally on naked skin early and on sparsely fur covered skin later during the development to the nearest 0.1°C with an infrared thermometer (Digitech, QM-7218) at the time of removal from the nest and then at 10 min intervals over the next 30 min, during which time the animals were not touched. Thermographs (Flir Model C2, resolution 0.1°C; pre-calibrated by manufacturer) were taken at the same time of the Ts readings. Emissivity was set at 0.95 for both infrared thermal devices throughout the study. Readings from thermometer and thermal camera used here were compared with axillary temperatures measured under the foreleg of the same young hamsters to the nearest 0.1°C using a calibrated thermocouple probe (TES-1310, TES Electric Electronic Corp.); these measurements were within 0.5°C of Ts reading. Animals were weighed to the nearest 0.1 g with an electronic balance at the end of the cooling experiments and were returned to their nest.
Torpor Induction
Metabolic Trials
From 1 day after animals were able to maintain a constant Tb at Ta 21 ± 1°C over 30 min, we tested whether they were able to enter a bout of torpor for energy conservation after food restriction. For these measurements, juveniles were removed from the nest in the late afternoon and placed individually into a respirometry chamber (volume 470 ml) to measure MR as the rate of oxygen consumption overnight (for up to 22 h) with an open flow respirometry system (Sable Systems, TurboFOX Complete Field System, including a mass-flow meter). An incubator (Yiheng Model LRH-250, Shanghai, China) was used to maintain constant Ta within the respirometry chamber during the measurement intervals at Ta 22.0 ± 0.5°C. Fresh air from outside was pumped through the chamber at ∼150 ml/min. Before entering the chamber, air passed through a copper coil to ensure that its temperature was adjusted to that inside the incubator. After passing through the respirometry chamber, the gas was subsampled and dried using a non-chemical gas drier (Sable systems, ND-2); approximately 70 ml/min at a stable flow rate was analyzed and recorded every 15 s. Baseline measurements of reference outside air were carried out every 3 h to compensate for potential drift of the oxygen sensor during long-term metabolic rate monitoring. Resting metabolic rate was taken as an average of 10-min the lowest, constant and stable readings during the first 3 h while hamsters were normothermic and in their resting phase.
Resting Metabolic Rate Measurements in the TNZ
From the age of 17 days (BM 6.0 g), resting metabolic rate of individuals was also measured at Ta 30–32°C (Ta 25–33°C, is the thermal neutral zone, TNZ, of adult desert hamsters; Zhan and Wang, 2004) for at least 3 h using the same respirometry equipment described above from day 17 until 45 days. In addition, after day 45, 9 adult hamsters from different litters that were 8–10 months old and were born in our laboratory colony with BM ranging from 19 to 26 g were examined. The lowest consecutive values over 10 min were assumed to be the RMR. Hamsters were weighed to the nearest 0.1 g before and after each respirometry measurement. The average of the two values of body mass was used to calculate the mass-specific metabolic rate.
Torpor Determination
In the morning when the MR of juvenile hamsters indicated that they were in a state of torpor (MR well below 75% of RMR measured in the same individual at the same Ta; Hudson and Scott, 1979; Chi et al., 2016) animals were removed from the chamber and their thermograph was taken and/or their Ts was measured immediately as described above. A Ts of ≤29.6°C was considered as an alternative definition of torpor because the mean resting Ts before measurements was 34.6 ± 0.6°C and a fall of Tb by 5°C or more from the resting Tb is often used for defining torpor (Ruf and Geiser, 2015). Animals were handled with gloves to ensure there was no heat transfer from the hand and then returned to the respirometry chamber within 1 min to determine whether they could rewarm from torpor; these measurements were repeated during the arousal process. Torpor duration was defined as the time with MR below 75% of RMR at the same Ta (Chi et al., 2016). Some young hamsters were placed individually in cages on sawdust into the incubator at Ta 22.0 ± 0.5°C overnight and it was determined via Ts and thermograph measurements whether they could enter and arouse from torpor. To minimize premature termination of torpor bouts measurements of Ts were conducted when obvious movements of a hamster were observed, which often happens before they arouse. Nevertheless, as some torpor bouts were disrupted, torpor bout duration was not further analyzed.
Statistics
Statistical test were performed using R (Pinheiro et al., 2018) and SPSS, Version 17.0. Linear mixed models were used with litter and animal ID as random factors to examine whether Ts in growing hamsters is related to BM and age because the same individuals were measured. Repeated Measures ANOVAs were conducted on Ts measurements both before and after the cooling experiments as well as on Ts and body mass among different ages together with LSD multiple comparisons. One sample t tests were used to examine the difference between Ts and Ta. Linear mixed models using litter as a random factor were used to analyze the relationship between RMR and BM. P-values of <0.05 were considered statistically significant. Numeric values are provided as means ± SD for the number of animals “n” measured.
Results
Body Mass in Young Hamsters During Cooling Experiments
The body mass (Figure 1 and Table 1) of neonate hamsters (litters of Pr1 and Pr2) ranged from 0.8 to 1.1 g (0.9 ± 0.1 g) and the animals were pink and naked. On day 5, mean body mass was 1.9 ± 0.1 g, increased to 2.7 ± 0.1 g on day 8, to 3.4 ± 0.1 g on day 10, to 4.0 ± 0.2 g on day 12 and to 5.5 ± 0.3 g on day 15 when hamsters looked like small adults with dorsal fur covering (Figure 1), but the ventral fur was still thin and sparse.
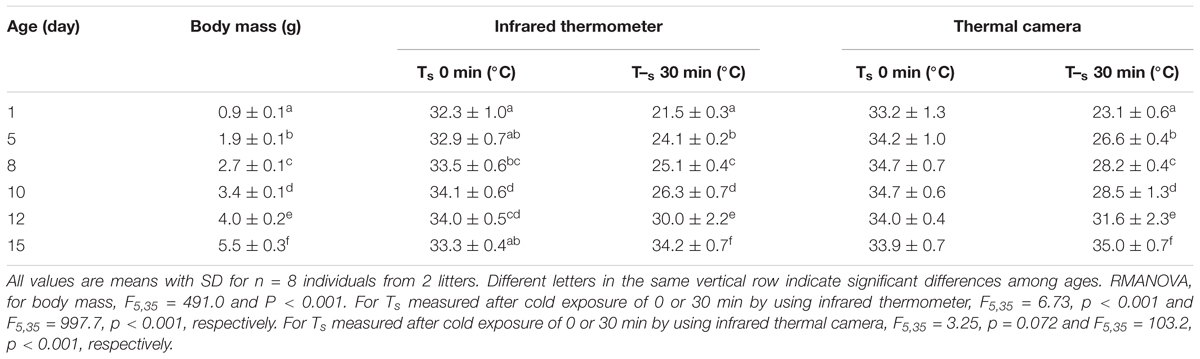
Table 1. Maximum Ts readings from infrared thermometer and thermographs in developing desert hamsters before (0 min) and after 30-min cold exposure at Ta 21°C.
Cooling Experiments
Rates of cooling in hamsters on day 1 were rapid (Figure 2 and Table 1). The Ts fell from 32.3 ± 1.0°C to ∼22°C within 10 min and after 30 min the Ts of 21.5 ± 0.3°C was barely distinguishable from the Ta of 21.2°C (t = 2.871, df = 7, p < 0.05). On day 5, initial Ts was slightly raised to 32.9 ± 0.7°C and cooling was slowed, with animals maintaining a Ts–Ta differential of 2.1 ± 0.2°C at 30 min and animals showed slight shivering (t = 25.31, df = 7, p < 0.01). Initial Ts further increased and cooling was further slowed on day 8, and 10 and 12 when animals showed good coordination and some shivering, but the eyes were still partially closed. On day 15 the initial Ts of 33.3 ± 0.4°C was actually lower, than the Ts of 34.2 ± 0.7°C measured after 30 min when the mean the Ts–Ta differential was 12.4°C; these animals showed strong shivering, digging and grooming, had stored millet seeds in their cheek pouches and tried to bite. The Ts at the beginning of cooling experiments were similar, but differed substantially after 30 min (Figure 2 and Table 1).
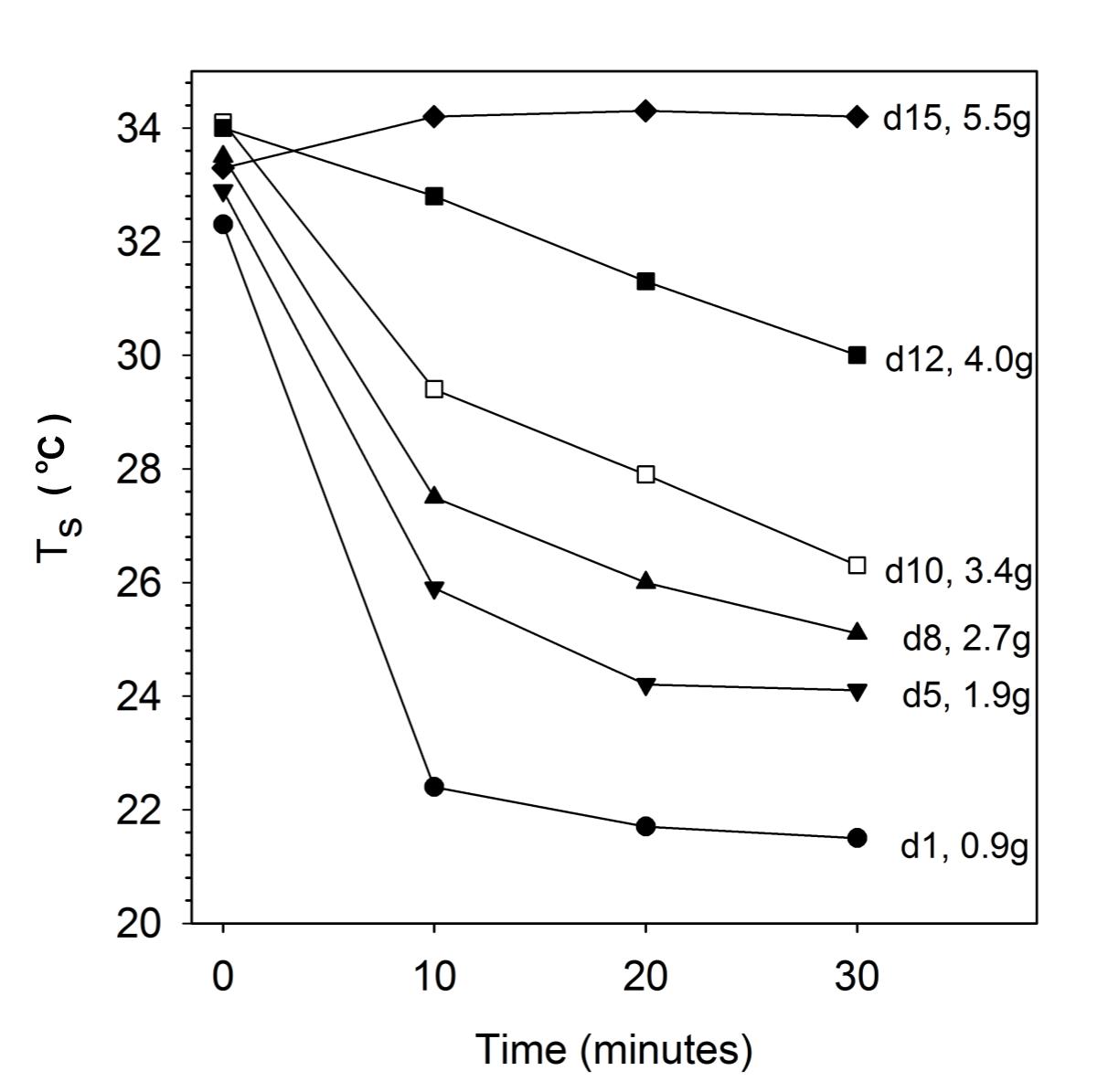
Figure 2. Cooling rates of desert hamsters at different ages from day 1 (d1) to day 15 (d15) at Ta 21 ± 1.0°C with corresponding body masses. Note the fast decline of surface temperature (Ts) measured with infrared thermometer on day 1 and the reduction of cooling with age. Only means are shown for clarity, SD are provided in Table 1.
Thermographs further emphasize the rapid developmental change in thermoregulatory ability (Figure 3 and Table 1). In 1 day old hamsters the maximum Ts was 35.6°C at 0 min (mean 33.2 ± 1.2°C), decreasing to 23.1 ± 0.6°C in 30 min. While the maximum Ts at 0 min seemed to change slightly because of the increasing fur cover it did not differ significantly with age (F5,35 = 3.25, p = 0.072). However, the rate of cooling slowed with growth as indicted by the thermograph color changes (Figure 3 and Table 1) and after 30 min exposure, Ts increased steadily to a maximum of 35.4°C on day 15. The maximum Ts after 30 min cold exposure was strongly affected by age and body mass (F5,35 = 103.2, p < 0.001, Ts after cold EXP = 0.80 age+22.0, R2 = 0.88, P < 0.001; Ts after cold EXP = 2.73 body mass+18.47, R2 = 0.92, P < 0.001). This supports the data from the infrared thermometer measurements that at the age of 15 days, desert hamsters are competent thermoregulators when exposed for short periods to a mild cold load.
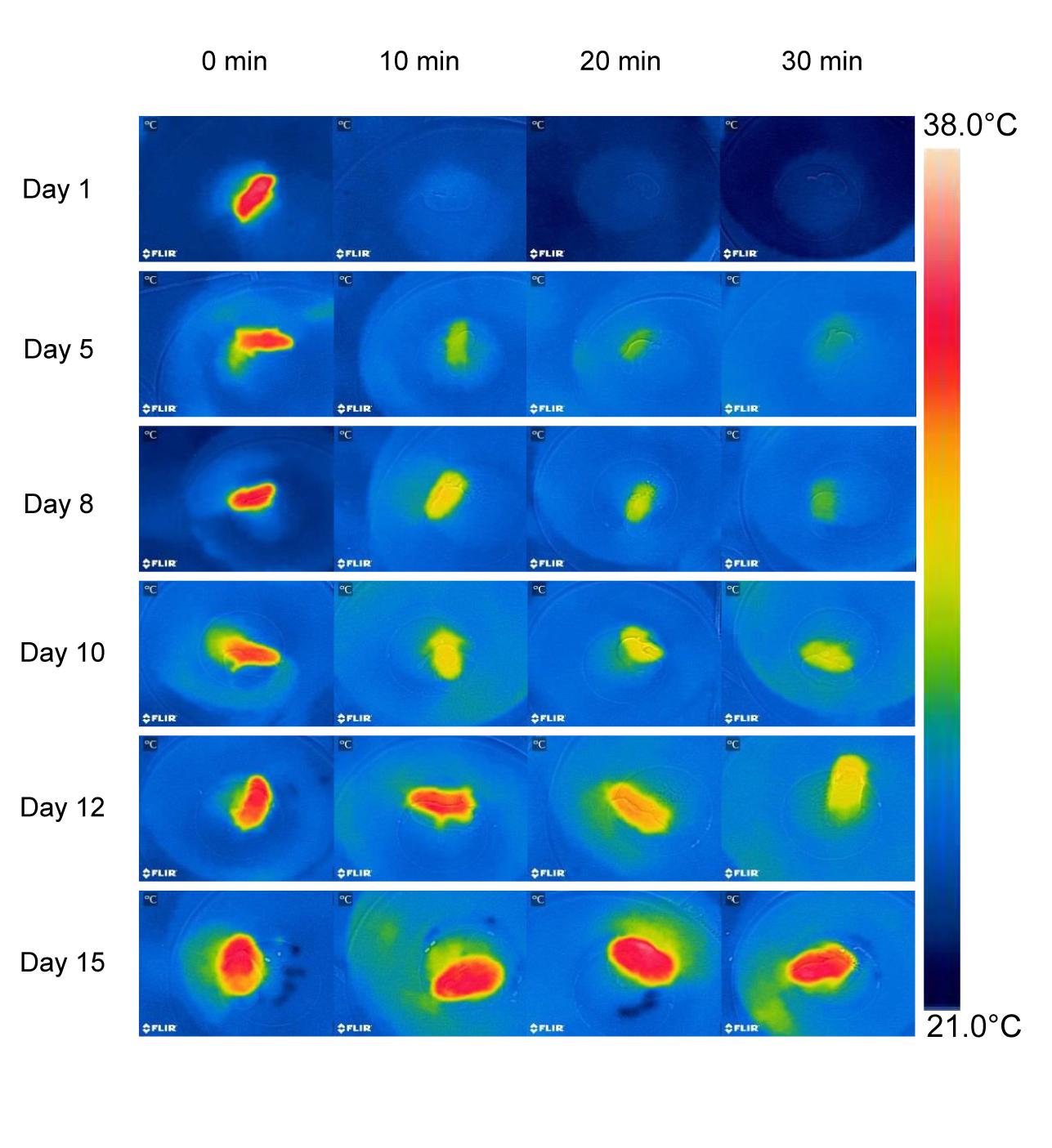
Figure 3. Thermographs of a representative desert hamster at each age experienced a cold exposure at Ta 21°C during development. Note the similar surface temperature (Ts) at 0 min, the different Ts after 30 min, the rapid fall of Ts on day 1, and the maintenance of a high Ts on day 15.
Metabolic Trials
When one of these animals (Pr2 #1) was exposed to Ta 22°C overnight on day 16 (mean BM 5.8 g), its MR initially ranged between ∼5 and 9 ml O2 g-1h-1, indicating resting and slight activity (Figure 4). Soon after the lights went off, MR increased to activity maxima approaching 16 ml O2 g-1h-1, interrupted by apparent resting phases. Both the maxima and minima declined with time in the dark phase until entry into torpor occurred at around 0400 h. Entry into torpor was characterized by a rapid fall of MR to a minimum MR during torpor of 1.14 ± 0.04 ml O2 g-1h-1 (∼30% of RMR at Ta 22°C, ∼7% of that during activity). When removed from the chamber after a torpor bout of ∼4 h at 0820 h, the Ts measured by infrared thermometer was 25.0°C, similar to that measured with the thermal camera.
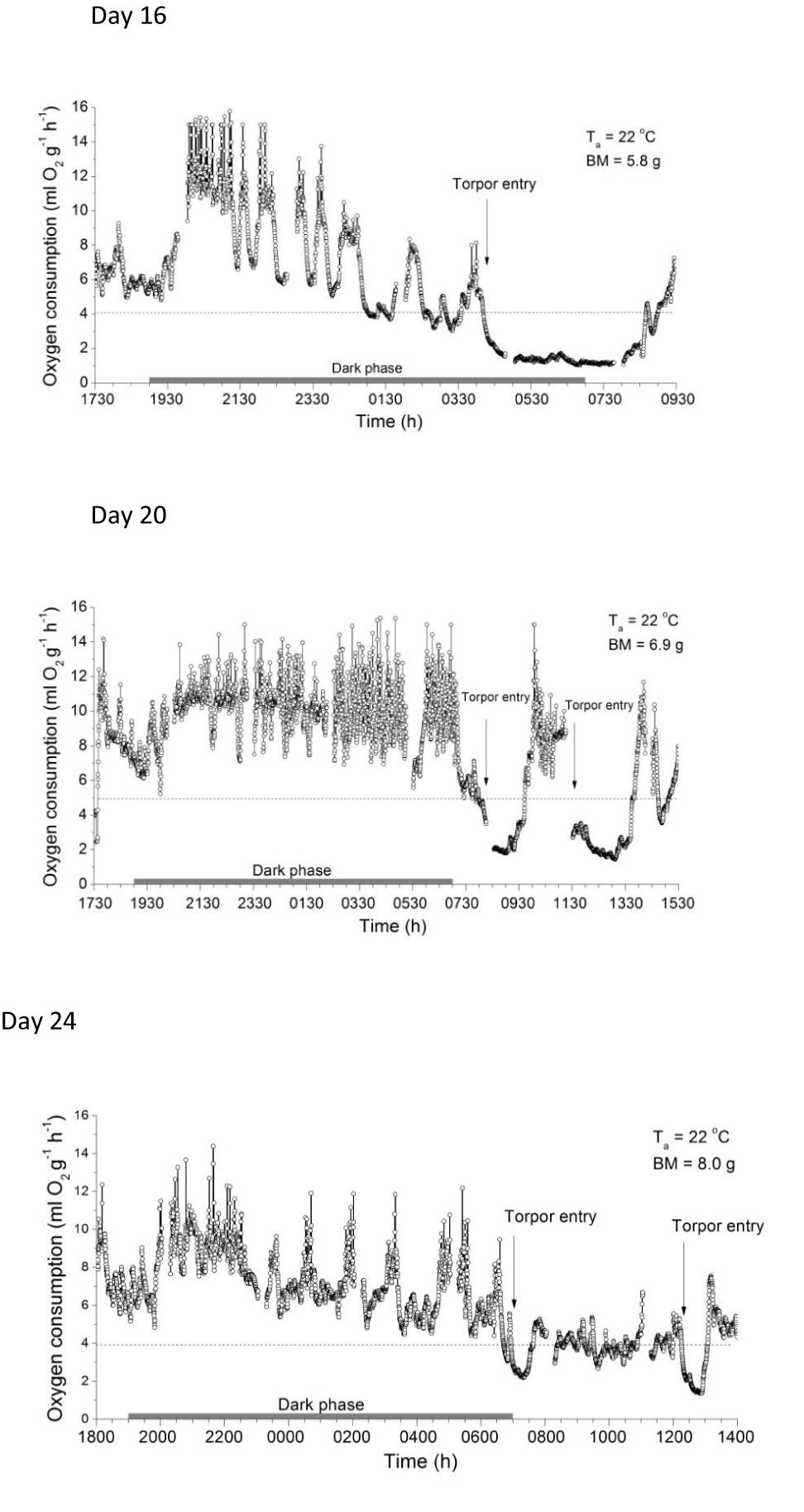
Figure 4. Metabolic rates (MR) of desert hamsters measured as oxygen consumption overnight. Note the high values during activity at night and the rapid fall of MR during torpor entry late at night or early in the morning. Torpor duration and depth decreased with age and increasing size. Gaps in the data points show baseline readings of the respirometry system every 3 h. Horizontal dotted lines show 75% of the resting metabolic rate measured at Ta 22°C.
Two other 16 day old individuals (Pr1 #2 and #3, BM = 5.8 ± 0.2 g) exposed in separate cages on wood shavings overnight without food and water, but without MR measurements, initially had a Ts of 34.4 ± 0.4°C, which fell to 24.6 and 25.8°C at 0820 h, and both were able to endogenously rewarm at Ta 22°C and increased Ts to ∼34°C.
When overnight MR measurements at Ta 22°C were repeated between 17 (BM = 6.5 ± 0.3 g) and 45 days (BM ∼12 g) other individuals also expressed torpor (Figure 4), but torpor duration and depth decreased with growth and age (Table 2). The Ts of all torpid individuals during or without metabolic rate measurements was 25.1 ± 0.6°C (16 days, BM = 5.8 ± 0.2 g, torpor use in 3/3), 28.8 ± 0.3°C (17 days, BM = 6.5 ± 0.3 g, torpor use 2/3), and 25.2 ± 3.9°C (20 days, BM = 6.3 ± 0.5 g, torpor use 3/3). From 21 days only shallow torpor was observed and usually only 1 out of three measured individuals expressed torpor.
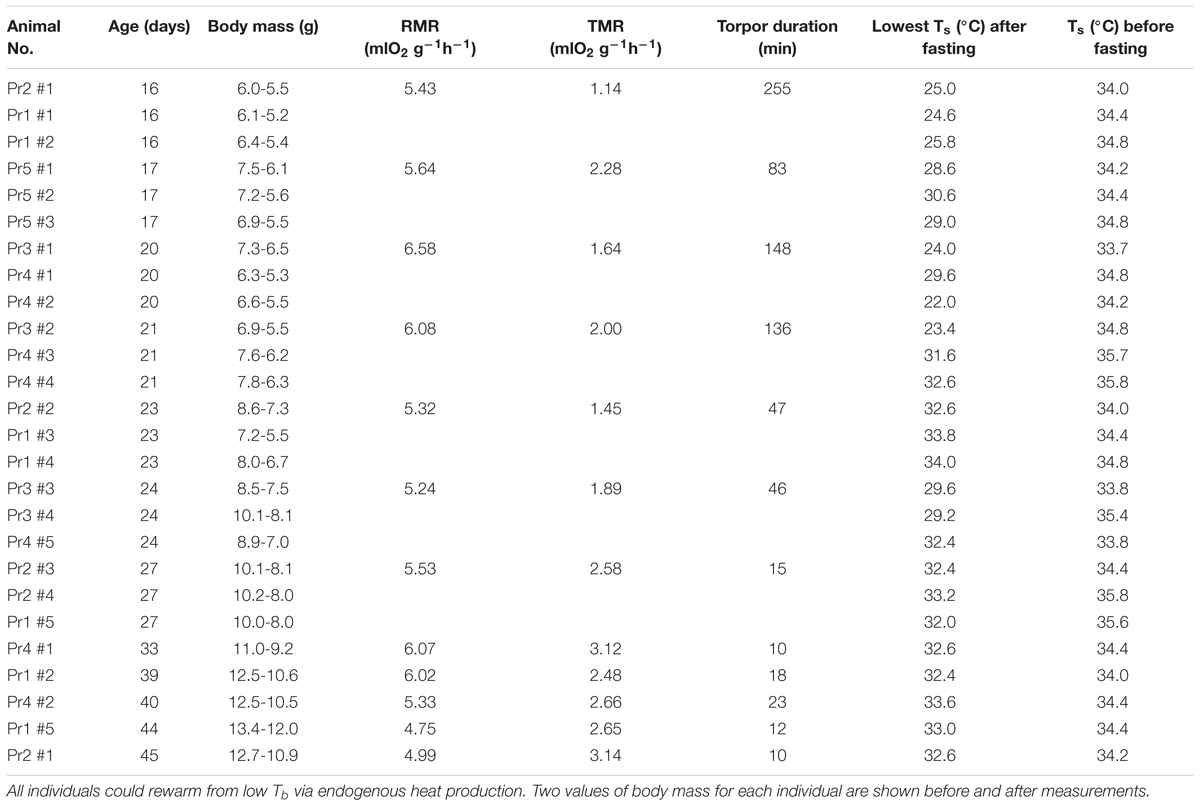
Table 2. Torpor metabolic rates (TMR), resting metabolic rates (RMR, maximum torpor duration) and Ts in desert hamsters fasted overnight at Ta of 22°C.
The mass-specific RMR measured in the TNZ at Ta 30–32°C ranged from 3.62 ml O2 g-1 h-1 (17 days, BM = 6 g) to 1.82 ml O2 g-1 h-1 (BM = 26.2 g adult). The log10 RMR was a negative function of log10 BM, with a slope of –0.33 (F1,22 = 20.89, P < 0.001; R2 = 0.59) (Figure 5).
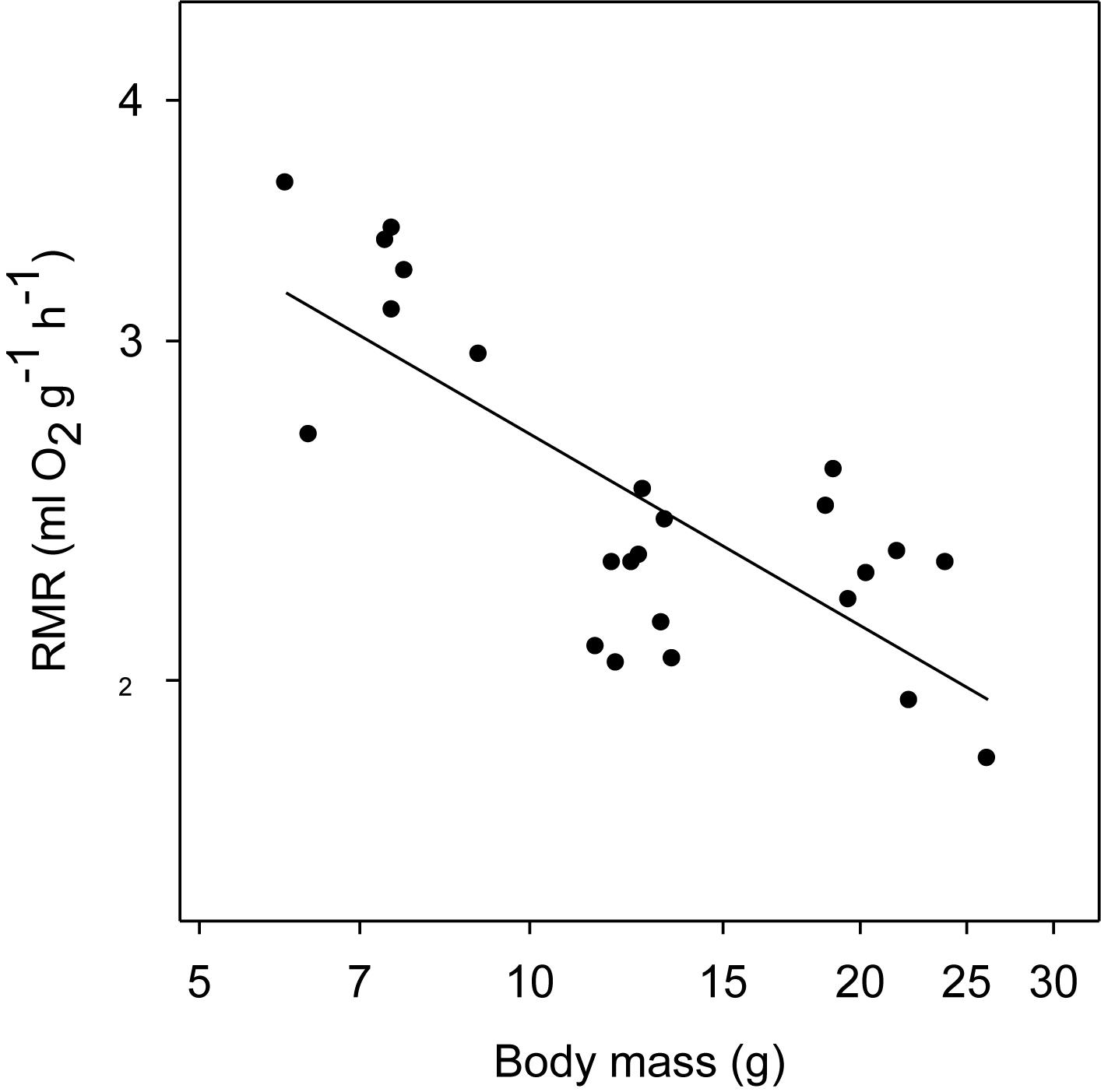
Figure 5. Resting metabolic rate (RMR) of desert hamsters in the TNZ as a function of body mass (BM) measured as rate of oxygen consumption at ambient temperatures (Ta) of 30 to 32°C. The RMR fell with the increase of BM (log10y = 0.757–0.33log10x; r2 = 0.59). RMR data were included for adult hamsters with body mass over 15 g (8–10 months age) raised under same laboratory conditions.
Discussion
The rate of development of desert hamsters is astonishing. Endothermy is reached as early as at 15 days of age at a body mass of only 5.5 g and already at 16 days (BM 5.8 g) the animals are able to express torpor with a rapid decline of MR at torpor entry and the ability of rewarming from low Tb at the end of the torpor bout.
The development of thermoregulation and morphology was qualitatively similar to what has been observed in other altricial birds and mammals (Morrison and Petajan, 1962; Geiser, 2008). Initially when the animals were small and naked, rates of cooling were high. Rates of cooling decreased with an increase in size, improvement of insulation, but likely also an improvement of non-shivering thermogenesis from brown adipose tissue (BAT) (Oelkrug et al., 2015). The difference between our and most other studies was the speed of development with competent endothermy in desert hamsters reached only after 15 days, much faster than in many other species, especially small marsupials that take several weeks to reach that state (Geiser et al., 2006; Wacker et al., 2017). However, the values were similar that what has been observed in the related and similar-sized Djungarian hamster (Phodopus sungorus) (Bae et al., 2003).
The fast development of thermoregulation of desert hamsters was followed by the ability to enter and arouse from torpor at an age as young as 16 days (BM 5.8 g). Although we quantified torpor at a rather mild Ta because of consideration of the animals’ wellbeing, lower Tas likely would have resulted in increased torpor use (see Chi et al., 2016) especially later during development. Torpor was characterized by a reduction of MR to <10% of the MR during activity, which was as high as that of bats and birds during flight, which are among the highest MR measured for vertebrates (Speakman and Racey, 1991). Rewarming from low Tb during torpor was achieved by juvenile desert hamsters although heat production is reduced at low Tb and although the high relative surface area at a small BM facilitates heat loss and makes endogenous rewarming difficult (Currie et al., 2015; Wacker et al., 2017). The fast development of torpor differs again from small marsupials, which reach that state only after several weeks (Wacker et al., 2017), but also from the related P. sungorus, which expressed fasting-induced torpor only from the age of 28 days (Bae et al., 2003). This difference may appear surprising considering the similar size, similar development of endothermy and similar general biology of the two congeners. However, the discrepancy is likely explained by the commencement of measurements of fasting-induced torpor in P. sungorus, which did not begin until day 21, soon after the animals had been implanted with transmitters. Therefore, it is possible that, if P. sungorus had been measured earlier, they would be rather similar to desert hamsters, an assumption that is supported by the observation of 2-deoxy-D-glucose (2-DG) induced Tb reduction in P. sungorus at the age of 16 days (Bae et al., 2003). Although it has been argued that the 2-DG induced reduction in Tb differs from natural torpor (Westman and Geiser, 2004, but see Chi et al., 2018) it nevertheless shows that the animals were able to rewarm from low Tb at a very early age.
What is the likely function of torpor during development? Constant endothermy in small species is extremely costly because of the high heat loss and requires a more or less uninterrupted supply of energy in the form of food. If adults are unable to supply the food or juveniles unable to obtain it, they are in danger of starvation or even death. This can be overcome by the expression of torpor, which reduces energy and therefore foraging requirements substantially (Nowack et al., 2017), and can prolong the time over which stored energy in the body can be extended, in extreme cases for up to several months (Ruf and Geiser, 2015). Of course huddling in nests also will reduce energy expenditure to some extent and is important during the development (Gilbert et al., 2010). However, it does not prevent occurrence of hypothermia in groups of young in the wild (Holloway and Geiser, 2000). Moreover, the limitation of huddling lies with its inability of reducing MR below basal levels, whereas during torpor MR values well below BMR can be reached and energy loss can be minimized (Namekata and Geiser, 2009). While survival is clearly an important function of torpor during development it also has the potential to be used for diverting nutrients to facilitate growth as growth rate on limited food is enhanced by torpor use (Giroud et al., 2014). Since the vast majority of mammals (for example, marsupials, bats and many rodents) and birds (for example passerines) are altricial at birth/hatching and will have to undergo similar morphological and functional changes described here, the expression of torpor during the development may be far more often employed that is currently appreciated, and may even include some small precocial species.
Past data suggested a different development sequence in torpor expression between birds and marsupial mammals on one hand and placental mammals on the other (Geiser, 2008). In birds and marsupials torpor expression seemed to develop immediately after competent endothermy was attained (i.e., heterothermic endothermy develops immediately after poikilothermy). In contrast, as outlined above, the data on the placental rodents suggested that the initial partial endothermic phase (poikilothermy) is followed by an intermediate homeothermic phase with constant high Tb lasting for about 2 weeks in hamsters (P. sungorus) with the first bout of torpor after food restriction observed only at the age of 28 days (Bae et al., 2003). More extreme, in juvenile ground squirrels (Spermophilus saturatus) this homeothermic phase is prolonged and torpor is expressed only ∼4 months after birth (Geiser and Kenagy, 1990). However, data from our present study with torpor expression in placental desert hamsters only 1 day after reaching endothermy suggest that there may be no generic difference between marsupial and placental mammals and that perhaps the pattern observed in ground squirrels is a reflection of their extreme seasonal thermal biology characterized by homeothermy in spring/summer and heterothermy only from late summer through autumn/winter. Thus, while our data do not support the view that there may be common developmental differences with regard to torpor expression among endothermic classes or sub-classes they nevertheless suggest that it is widely used by many small endotherms.
It is interesting that torpor expression is reduced with growth. Likely this is related to the reduction of mass-specific RMR in thermo-neutrality (Figure 5), which interestingly, despite the young were growing and should have a raised RMR, had a slope of –0.33 as that measured for BMR in fully grown adult mammals (White and Kearney, 2013). However, and perhaps more importantly with regard to our study, the reduced torpor expression with growth was likely caused by the exposure to only a mild cold load (Ta 22°C), a reduction on RMR during cold exposure, and a reduced cost of locomotion that decreases with size (Tucker, 1975). Especially the mild Ta differs substantially from those experienced by the animals in the wild in winter when they are exposed to very low Ta and food is probably scarce. Therefore individuals in the wild likely will express torpor even when they reach adulthood (Chi et al., 2016).
Clearly heterothermy during development provides juveniles with flexible thermo-energetics and consequently a better chance of survival, as is also the case even before birth during reproduction (Willis et al., 2006). Considering how few species have been studied in this regard, it is possible that torpor during development is used by many if not the majority of altricial species. This interpretation is supported by the fact that shallow torpor during development has been observed even in species considered to be homeothermic as adults such as in juvenile storm petrels (Oceanodroma furcata) and laboratory rats (Rattus norvegicus) (Boersma, 1986; Nuesslein-Hildesheim et al., 1995). It therefore seems an omission that the phenomenon of torpor use during development has not been more widely examined with the aim to improve the understanding of its diversity and function.
Ethics Statement
This study was carried out in accordance with the recommendations of Animal Care and Use Committee of Institute of Zoology, the Chinese Academy of Sciences. The protocol was approved by the Animal Care and Use Committee of Institute of Zoology, the Chinese Academy of Sciences.
Author Contributions
FG finished the main parts of the manuscript. Q-SC, JW, GS, and FG conducted the experiments. FG, Q-SC, and D-HW conceived this study.
Funding
Our study was financially supported by grants from the National Natural Science Foundation of China to Q-SC (31470473) and a Chinese Academy of Sciences Fellowship to FG.
Conflict of Interest Statement
The authors declare that the research was conducted in the absence of any commercial or financial relationships that could be construed as a potential conflict of interest.
References
Bae, H. H., Larkin, J. E., and Zucker, I. (2003). Juvenile Siberian hamsters display torpor and modified locomotor activity and body temperature rhythms in response to reduced food availability. Physiol. Biochem. Zool. 76, 858–867. doi: 10.1086/381462
Boersma, P. D. (1986). Body temperature, torpor, and growth in chicks of fork-tailed storm-petrels (Oceanodroma furcata). Physiol. Zool. 59, 10–19. doi: 10.1086/physzool.59.1.30156084
Boyer, B. B., and Barnes, B. M. (1999). Molecular and metabolic aspects of mammalian hibernation. Bioscience 49, 713–724. doi: 10.2307/1313595
Chi, Q. S., Li, X. J., and Wang, D. H. (2018). 2-Deoxyglucose, not marcaptoacetae, induces a reversible reduction of body temperature in males desert hamsters (Phodopus roborovskii). J. Therm. Biol. 71, 189–194. doi: 10.1016/j.jtherbio.2017.11.011
Chi, Q. S., Wan, X. R., Geiser, F., and Wang, D. H. (2016). Fasting-induced daily torpor in desert hamsters (Phodopus roborovskii). Comp. Biochem. Physiol. A 199, 71–77. doi: 10.1016/j.cbpa.2016.05.019
Currie, S. E., Noy, K., and Geiser, F. (2015). Passive rewarming from torpor in hibernating bats: minimizing metabolic costs and cardiac demands. Am. J. Physiol. 308, R34–R41. doi: 10.1152/ajpregu.00341.2014
Dausmann, K. H., and Warnecke, L. (2016). Primate torpor: ghost of the climatic past. Physiology 31, 398–408. doi: 10.1152/physiol.00050.2015
Dawson, W. R., and Evans, F. C. (1960). Relation of growth and development to temperature regulation in nestling vesper sparrows. Condor 62, 329–340. doi: 10.2307/1365163
Eichhorn, G., Groscolas, R., Le Glaunec, G., Parisel, C., Arnold, L., Medina, P., et al. (2011). Heterothermy in growing king penguins. Nature. Comm. 2:435. doi: 10.1038/ncomms1436
Geiser, F. (2008). Ontogeny and phylogeny of endothermy and torpor in mammals and birds. Comp. Biochem. Physiol. A 150, 176–180. doi: 10.1016/j.cbpa.2007.02.041
Geiser, F., and Kenagy, G. J. (1990). Development of thermoregulation and torpor in the golden-mantled ground squirrel Spermophilus saturatus. J. Mammal 71, 286–290. doi: 10.2307/1381938
Geiser, F., Stawski, C., Wacker, C. B., and Nowack, J. (2017). Phoenix from the ashes: fire, torpor and the evolution of mammalian endothermy. Front. Physiol. 8:842. doi: 10.3389/fphys.2017.00842
Geiser, F., Westman, W., McAllan, B. M., and Brigham, R. M. (2006). Development of thermoregulation and torpor in a marsupial: energetic and evolutionary implications. J. Comp. Physiol. B 176, 107–116. doi: 10.1007/s00360-005-0026-y
Gilbert, C., McCafferty, D., LeMaho, Y., Martrette, J. M., Giroud, S., Blanc, S., et al. (2010). One for all and all for one: the energetics benefits of huddling in endotherms. Biol. Rev. 85, 545–569. doi: 10.1111/j.1469-185X.2009.00115.x
Giroud, S., Zahn, S., Criscuolo, F., Chery, I., Blanc, S., Turbill, C., et al. (2014). Late-born intermittently fasted juvenile garden dormice use torpor to grow and fatten prior to hibernation: consequences for ageing processes. Proc. Biol. Sci. B 281:20141131. doi: 10.1098/rspb.2014.1131
Holloway, J. C., and Geiser, F. (2000). Development of thermoregulation in the sugar glider Petaurus breviceps (Marsupialia: Petauridae). J. Zool. 252, 389–397. doi: 10.1017/s0952836900000133
Hudson, J. W., and Scott, I. M. (1979). Daily torpor in the laboratory mouse, Mus musculus var. albino. Physiol. Zool. 52, 205–218. doi: 10.1086/physzool.52.2.30152564
Kolynchuk, A. (2015). Phodopus roborovskii Animal Diversity Web. Available at http:/animaldiversity.org/accounts/Phodopus_roborovskii/. (accessed December 12, 2017).
Kronfeld-Schor, N., and Dayan, T. (2013). Thermal ecology, environments, communities, and global change: energy intake and expenditure in endotherms. Annu. Rev. Ecol. Evol. Syst. 44, 461–480. doi: 10.1146/annurev-ecolsys-110512-135917
McKechnie, A. E., and Mzilikazi, N. (2011). Heterothermy in Afrotropical mammals and birds: a review. Int. Comp. Biol. 51, 349–363. doi: 10.1093/icb/icr035
Mitchell, D., Snelling, E. P., Hetem, R. S., Maloney, S. K., Strauss, W. M., and Fuller, A. (2018). Revisiting concepts of thermal physiology: predicting responses of mammals to climate change. J. Animal. Ecol. 87, 956–973. doi: 10.1111/1365-2656.12818
Morrison, P. R., and Petajan, J. H. (1962). The development of temperature regulation in the opossum, Didelphis marsupialis virginiana. Physiol. Zool. 35, 52–65. doi: 10.1086/physzool.35.1.30152713
Nagel, A. (1977). Torpor in the European white-toothed shrews. Experientia 33, 1455–1456. doi: 10.1007/bf01918804
Namekata, S., and Geiser, F. (2009). Effects of nest use, huddling, and torpor on thermal energetics of eastern pygmy-possums. Aust. Mammal 31, 31–34.
Nowack, J., Stawski, C., and Geiser, F. (2017). More functions of torpor and their roles in a changing world. J. Comp. Physiol. B 187, 889–897. doi: 10.1007/s00360-017-1100-y
Nuesslein-Hildesheim, B., Imai-Matsumura, K., Doering, H., and Schmidt, I. (1995). Pronounced juvenile circadian core temperature rhythms exist in several strains of rats but not in rabbits. J. Comp. Physiol. B 165, 13–18.
Oelkrug, R., Polymeropoulos, E. T., and Jastroch, M. (2015). Brown adipose tissue: physiological function and evolutionary significance. J. Comp. Physiol. B 185, 587–606. doi: 10.1007/s00360-015-0907-7
Pinheiro, J., Bates, D., DebRoy, S., Sarkar, D., and R Core Team (2018). nlme: Linear and Nonlinear Mixed Effects Models. R Package Version 3.1-137. Available at: https://CRAN.R-project.org/package=nlme
Riek, A., and Geiser, F. (2013). Allometry of thermal variables in mammals: consequences of body size and phylogeny. Biol. Rev. 88, 564–572. doi: 10.1111/brv.12016
Ruf, T., and Geiser, F. (2015). Daily torpor and hibernation in birds and mammals. Biol. Rev. 90, 891–926. doi: 10.1111/brv.12137
Speakman, J. R., and Racey, P. A. (1991). No cost of echolocation for bats in flight. Nature 350, 421–423. doi: 10.1038/350421a0
Tattersall, G. J., Sinclair, B. J., Withers, P. C., Field, P. A., Seebacher, F., Cooper, C. E., et al. (2012). Coping with thermal challenges: physiological adaptations to environmental temperature. Compr. Physiol. 2, 2151–2202.
Turbill, C., Bieber, C., and Ruf, T. (2011). Hibernation is associated with increased survival and the evolution of slow life histories among mammals. Proc. R Soc. B 278, 3355–3363. doi: 10.1098/rspb.2011.0190
Wacker, C. B., McAllan, B. M., Körtner, G., and Geiser, F. (2017). The role of basking in the development of endothermy and torpor in a marsupial. J. Comp. Physiol. B 187, 1029–1038. doi: 10.1007/s00360-017-1060-2
Westman, W., and Geiser, F. (2004). The effect of metabolic fuel availability on thermoregulation and torpor in a marsupial hibernator. J. Comp. Physiol. B 174, 49–57. doi: 10.1007/s00360-003-0388-y
White, C. R., and Kearney, M. R. (2013). Determinants of inter-specific variations in basal metabolic rate. J. Comp. Physiol. B 183, 1–26. doi: 10.1007/s00360-012-0676-5
Willis, C. K. R., Brigham, R. M., and Geiser, F. (2006). Deep, prolonged torpor by pregnant, free-ranging bats. Naturwissenschaften 93, 80–83. doi: 10.1007/s00114-005-0063-0
Withers, P. C., Cooper, C. E., Maloney, S. K., Bozinovic, F., and Cruz-Neto, A. P. (2016). Ecological and Environmental Physiology of Mammals. Oxford: Oxford University Press.
Keywords: Asia, desert hamsters (Phodopus roborovskii), heterothermy, altricial, body temperature, metabolic rate, torpor
Citation: Geiser F, Wen J, Sukhchuluun G, Chi Q-S and Wang D-H (2019) Precocious Torpor in an Altricial Mammal and the Functional Implications of Heterothermy During Development. Front. Physiol. 10:469. doi: 10.3389/fphys.2019.00469
Received: 18 November 2018; Accepted: 04 April 2019;
Published: 24 April 2019.
Edited by:
James Todd Pearson, National Cerebral and Cardiovascular Center, JapanReviewed by:
Chao Chen, University of California, Davis, United StatesFrank Seebacher, The University of Sydney, Australia
Copyright © 2019 Geiser, Wen, Sukhchuluun, Chi and Wang. This is an open-access article distributed under the terms of the Creative Commons Attribution License (CC BY). The use, distribution or reproduction in other forums is permitted, provided the original author(s) and the copyright owner(s) are credited and that the original publication in this journal is cited, in accordance with accepted academic practice. No use, distribution or reproduction is permitted which does not comply with these terms.
*Correspondence: Qing-Sheng Chi, Y2hpcXNjbkBob3RtYWlsLmNvbQ== De-Hua Wang, d2FuZ2RoQGlvei5hYy5jbg==