- 1Department of Intensive Care Medicine, Brugmann University Hospital, Brussels, Belgium
- 2Unit of Oxygen Study, Translational Research Laboratory, Université Libre de Bruxelles, Brussels, Belgium
- 3Laboratory of Integrative Physiology, Haute Ecole Bruxelles-Brabant, Brussels, Belgium
- 4Faculty of Medicine, Université catholique de Louvain, Brussels, Belgium
- 5Hypobaric Chamber, Queen Astrid Military Hospital, Brussels, Belgium
Objective: To observe the effects of a fast-acute ascent to high altitude on brain cognitive function and transcranial doppler parameters in order to understand the physiological countermeasures of hypoxia.
Methods: 17 high-altitude-naïve male subjects (mean age was 26.3 ± 8.1 years) participated in the study. We measured Critical Flicker Fusion Frequency (CFFF), blood oxygen saturation, Psychology Experiment Building (PEBL) including three tests (Modified Math Processing Task, Perceptual Vigilance Task, and Time Estimation Task), as well as Cerebral Blood Flow index (CBFi), mean cerebral artery Systolic and diastolic velocities, Cerebral Pulsatility index (CPi), and heart Rate. All were measured at sea level, at least 1 h after arrival at the hypobaric hypoxia equivalent of 3842 m and 1 h after return to sea level.
Results: Under acute exposure to hypobaric hypoxic conditions, significant decrease in CFFF [42.1 ± 1 vs. 43.5 ± 1.7 Hz at sea level (asl), p < 0.01], CBFi (611 ± 51 vs. 665 ± 71 asl, p < 0.01) and blood oxygen saturation (83 ± 4% vs. 98 ± 1% asl, p < 0.001) as compared to pre-ascent values were observed. Physiological countermeasures to hypoxia could be involved as there was no significant change in neuropsychometric tests, Systolic and Diastolic velocities and CPi. A significant increase in Heart Rate (81 ± 15 bpm vs. 66 ± 15 bpm asl, p < 0.001) was observed. All parameters returned to their basal values 1 h after regaining sea level.
Conclusion: Hypoxia results in a decrease in CFFF, CBFi and oxygen saturation and in an increase in heart rate. As it decreased, Cerebral Blood Flow index does not seem to be the physiological measurement of choice to hypoxia explaining the maintenance of cognitive performance after acute exposure to hypobaric hypoxia and requires further investigation. Cerebral oxygen delivery and extraction could be one of the underlying mechanisms.
Introduction
High altitude mountain environment causes important physiological stresses on the human body due to a decrease in atmospheric pressure with altitude inducing a low partial pressure of oxygen as well as low temperature (Imray et al., 2010).
Relationship between cerebral blood flow and partial arterial oxygen pressure (PaO2) is curvilinear whereas its relationship to arterial oxygen saturation (SpO2) is linear (Steinback and Poulin, 2016). Regional differences in cerebral artery blood flow exist. Anterior and mean cerebral arteries increase their flow with hypoxia whereas posterior arteries decrease their flows (Feddersen et al., 2015), which could have an incidence in cognitive functions (Lawley et al., 2017). Regulation of the cerebral blood flow is essentially linked to peripheral chemoreceptors (Iturriaga et al., 2016) and as the brain is highly sensitive to hypoxia (Lankford, 2014), physiological countermeasures are probably involved to compensate hypoxemia.
Literature shows mixed findings on cognition function under hyperoxic (Lafere et al., 2016; Brebeck et al., 2017; Germonpre et al., 2017) or hypobaric hypoxic conditions. This depends on duration of exposure, altitude level, individual susceptibility and/or education (Lefferts et al., 2019) and choice of battery testing. Decrease in reaction time (Li et al., 2000) or an increase in decisional errors (Davranche et al., 2016) has been reported. Furthermore prolonged exercise under hypoxic conditions is associated with a decrease in cerebral oxygenation partly associated with a decrease in cognitive functions (Dobashi et al., 2016). Working memory also seems altered under hypobaric hypoxic conditions (Malle et al., 2013). Cognitive performances are not only influenced by hypoxia but also through elements as age, gender and education (Lefferts et al., 2019).
Many studies have investigated cognitive performances after night acclimatization (Pun et al., 2018b) subacute or repeated exposure to high altitude (Pun et al., 2018a). Most showed a certain degree of cognitive impairment. Increasingly easy access to moderate or high altitude (cable car) has facilitated tourist to go there fast and without acclimatization. Certainty of potential danger in decision making has to our best knowledge never been explored in this setting.
Therefore, the objective of our study was to evaluate cognitive function during these first hours of acute exposure to high altitude. Previously, a pilot study from our group in the French ski resort of Chamonix showed surprising results in cognitive testing. Indeed, psychometric testing showed no statistical differences when done in the resort (mean SpO2: 98%) and at 3842 m (mean SpO2: 80%). This probably means that physiological countermeasures are present at least in the first hours of acute exposure to hypobaric hypoxia (high altitude). Here, we investigate transcranial Doppler variables and cognitive function after a rapid ascent (20 min) and a short stay (4 h) at 3842 m and 1 h after a return to sea level. This study aims to avoid climbing effort, to limit confounding factors and focusing on the “passive” physiological acute adaptations.
Materials and Methods
Population
All experimental procedures, conducted in accordance with the Declaration of Helsinki (World Medical Association, 2013), were approved by the Academic Ethical Committee of Brussels (Brussels Alliance for Research and Higher Education, B200-2014-045) and written informed signed consent was sought. Seventeen Caucasian, well educated (Lefferts et al., 2019) male subjects, naïve to high altitude were included in the study. Their mean age was 26.3 ± 8.1 years. Height and weight were, respectively, 179.6 ± 6.6 cm and 79.5 ± 8.6 kg. Subjects needed to be in good physical shape, non-smoking and not high-level athletes. Subjects suffering from arterial hypertension or another cardiovascular disease were excluded from the study, so were subjects on cardio-active drugs. No antioxidants (dark chocolate, red wine, green tea, …) were permitted 8 h preceding and during the study (Engan et al., 2012).
Protocol
Experiments were undertaken at two different dates (8 and 9 subjects) in the hypobaric chamber of Queen Astrid Military Hospital in Brussels, Belgium. The ascent scheme was the following to mimic the actual scheme present at Chamonix (France) to reach the top of the Aiguille du Midi pic: all subjects took the 20 min “cable car” ride from sea level to 3842 m; all two groups stayed 3 h 20 min at 3842 m before regaining sea level in 20 min. The purpose was to reproduce the scheme used in our pilot study in the French ski resort of Chamonix. All measurements were made before ascent, after at least 1 h stay at 3842 m and 1 h after return to sea level. Each experiment was made by the same investigator to decrease the risk of inter-experimenter variation. Measures were made in a thermoneutral space to exclude effects of cold. Exercise was restrained. Figure 1 shows the ascent scheme.
Measures
Oxygen Saturation and Heart Rate
Blood oxygen saturation (SpO2) and heart rate, in beats per minute (bpm). were measured at the finger through an oximeter (Hand-Held Pulse Oximeter – CMS60F – Contec Medical Systems Co., LTD., Qinhuangdao, China) including an infrared light captor placed on the subject’s finger. It was expressed in %. The measure was fast (a few seconds).
Transcranial Doppler
Transcranial Doppler (TCD) was a noninvasive practical way to assess blood flow in cerebral arteries Blood velocity in the middle cerebral artery (VMCA) was measured with a 25-MHz TCD probe (Mindray M7, Mindray Bio-Medical Electronics, Shenzhen, China) going through the temporal bone window at both sides of the skull, at a depth of 4–6 cm for 10 s (Aaslid et al., 1982). The values of the brain side with the highest mean VMCA were registered. We calculated the pulsatility index [PI = (velocity systolic-velocity diastolic)/mean velocity] and cerebral blood flow index (CBFi = MAP × 10/1.47xPI), where MAP stands for mean arterial pressure (Pierrakos et al., 2013). The pulsatility index was a result of the interaction between the cerebral perfusion pressure, the amplitude of pulse arterial pressure, cerebrovascular resistances and heart rate (de Riva et al., 2012).
Cognitive Functions
Critical flicker fusion frequency
The CFFF was assessed with a specific device (Human Breathing Technology, Trieste, Italy). The device consists on a rotating ring, surrounding a short cylindrical waterproof plastic housing of 8 cm diameter containing the numeric (digital) frequency indicator covered by an acrylic transparent window. Attached to this housing a flexible cable is connected to a single blue LED (Light Emitting Diode, color temperature 8000 Kelvin), inserted in a small cylindrical waterproof container (to protect it from straying light and reflections). During the test the subject was looking straight at the LED light. The distance was adapted individually and freely to his personal best visual perception (generally around 50 cm). The investigator started at 35 Hz and increased or decreased the flickering frequency of the LED by steps of 0.25 Hz with the use of appropriate button. Thanks to the design of the device the subjects were not able to know the start and the finish frequency. When the subject saw a change in LED light from flicker to fusion, the subject acknowledged it to the investigator (through a sign without leaving sight of the LED) and the test was stopped. This noninvasive test has been validated to measure awareness (Hemelryck et al., 2013; Balestra et al., 2018; Rocco et al., 2019), mental fatigue (Ma et al., 2014), or executive dysfunction (Mewborn et al., 2015). It has already been demonstrated that CFFF could follow the recovery of cognitive function after propofol sedation earlier than psychometric testing (Sharma et al., 2011). This kind of correlation between mental state, CFFF and electroencephalography (EEG) has also been proposed recently in a world-class chess player, who showed parallel increases in CFFF threshold and theta Fz/alpha Pz ratio (Fuentes et al., 2018). In another recent study CFFF and attentional performance were closely related, with a tight relationship between the CFFF and occipital gamma band activity both in frequency and power (Kahlbrock et al., 2012).
This measurement has been presented in percentage as a relative variation of his personal value everyone being his own control.
Psychology experiment building language tests (PEBL)
Psychology experiment building language or PEBL is an open access software1 widely used in cognitive psychology (Li et al., 2000). It gathers dozens of functional tests. The PEBL tests were executed on a laptop. Three modified tests have been run, using the PEBL software package which was installed on the laptop. When the test chain is launched, detailed instructions are given. The results were automatically stored for not displaying any results to the subjects. Modified Math Processing Task, Perceptual Vigilance Task and Time Estimation Task were the three tests. These tests measured efficiency of higher brain functions such as processing speed, processing efficiency, working memory, concentration difficulties, and spatiotemporal integration.
In the Modified Math Processing Task (MMPT), the subjects are asked to solve several three-term additions and subtractions to determinate if the answer is greater or less than five. Two possible responses are proposed: “greater than” or “less than.” “Less than” responses are given by pressing the left shift key of the keyboard whereas “greater than” responses were made by pressing the right key of the keyboard. No result could be equal to five. The volunteer tried to complete the task as quickly and accurately as possible and responded correctly to every problem in a maximum of 4 s. The task ran for 3 min and stopped automatically. Correct, incorrect and timed-out results were stored for further analyses. This test permitted to evaluate participants in their capabilities associated to working memory, usually partially directed by the prefrontal cortex (Lee and Goto, 2015).
The Perceptual Vigilance Task (PVT) was a test used to measure a simple reaction time. This version was commonly used to measure sleepiness and arousal. The subjects had to press the spacebar, as quickly as possible when a red circle stimulus appears randomly in a 2 to 12 s delay. This happened 16 times. The RT (reaction time) was stored for further analyses. The test was highly sensitive to sleep loss (decrease in diurnal vigilance) (Thomann et al., 2014). Sustained attention seemed linked to frontal cortex (Loo et al., 2009).
In the Time Estimation Task (Time Wall), a target always started at the top of the screen and descended at a constant rate toward the bottom. 20 targets passed at different, but constant, speed. When the target exceeded two-thirds of the way down, it passed behind a wall and became invisible. Subject’s task was to press a key from the keyboard at the exact moment the moving target would pass through the notch marked at the very bottom of the display. In making this judgment, subject could not count or use any other rhythm method to facilitate his or her judgment. Subject followed the target with eyes and imagine it continuing straight down behind the wall to the notch. The real time compared to the estimated time by the subject was stored for further analyses. A precision score was established and calculated as followed mean estimated time minus mean real time divided by mean estimated time. Decision making and attention seemed related to the frontal cortex (Piper et al., 2015).
Statistical Analysis
Statistical analysis was made with a Prism 6 statistical Software (Graphpad, La Jolla, CA, United States). Data are given as a percentage of pre-ascent values. The difference between the percentage of pre-ascent values and 100% (no change) was compared by a two-tailed one-sample t-test when normality of the sample was reached as assessed by the Kolmogorov-Smirnov test. Otherwise, the non-parametric Wilcoxon Rank Sum test was used. Statistically significant level was set at p < 0.05. Results are presented in the text as mean ± standard deviation.
Results
Oxygen Saturation and Heart Rate
Blood oxygen saturation significantly decreased at high altitude (83 ± 4%) as compared to pre-ascent (98 ± 1%, p < 0.001) and post-descent levels (98 ± 1%, p < 0.001). There was no difference between pre- and post-altitude values.
Heart rate significantly increased at high altitude (81 ± 15 bpm) as compared to pre-ascent (66 ± 15 bpm, p < 0.001) and post-descent levels (65 ± 12 bpm, p < 0.001). There was no difference between pre- and post-altitude values.
Transcranial Doppler
Cerebral blood flow index decreased during high altitude stay (611 ± 51 vs. 665 ± 71 asl, p < 0.01) and returned to baseline 1 h after regaining sea level (Figure 2A). Cerebral pulsatility index did not vary throughout the experiment (Figure 2B). There were no statistical differences between systolic, diastolic or mean (Figure 3). velocities of the mean cerebral artery at different time frames.
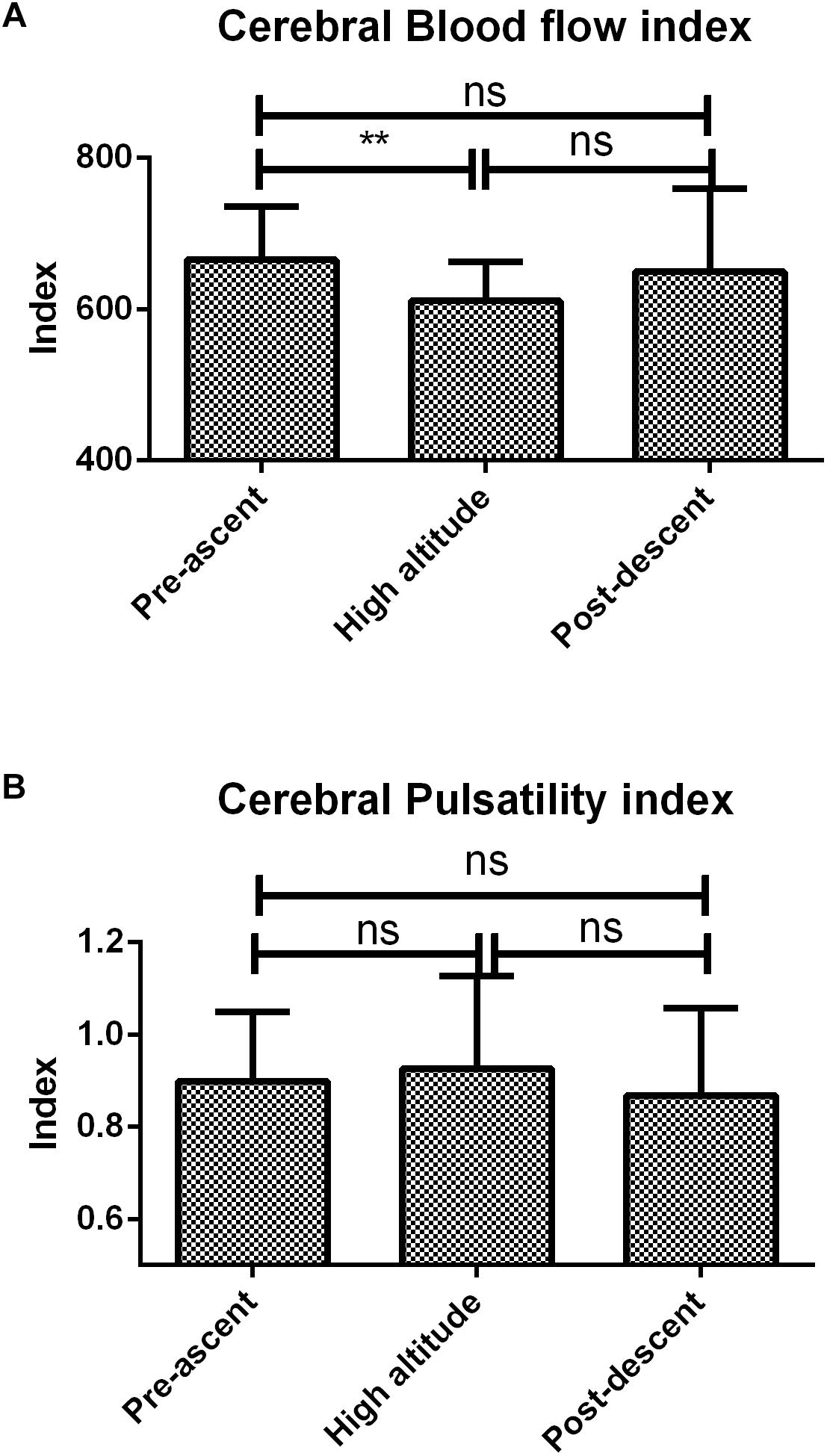
Figure 2. Mean cerebral artery velocity indexes. (A) Cerebral blood flow index, (B) Cerebral pulsatility index. Errors bars represent standard error of the mean (SEM). ∗∗p < 0.01.
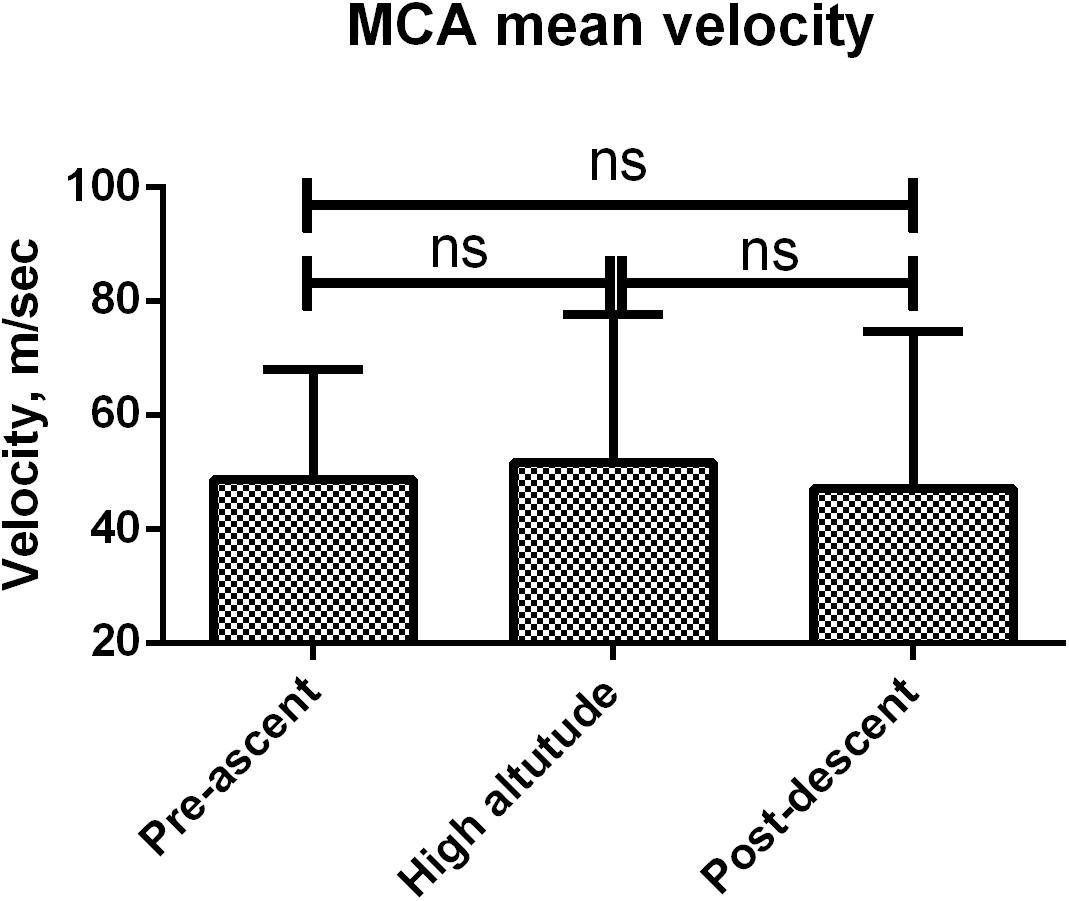
Figure 3. Mean cerebral artery mean velocity. Errors bars represent standard error of the mean (SEM).
Cognitive Functions
Critical Flicker Fusion Frequency
Critical Flicker Fusion Frequency significantly decreased when measured at high altitude (42.1 ± 1 Hz) as compared to pre-ascent (43.5 ± 1.7 Hz, p < 0.01) and post-descent measures (43.1 ± 1.8 Hz, p < 0.01). Figure 4 shows CFFF values at different time frames.
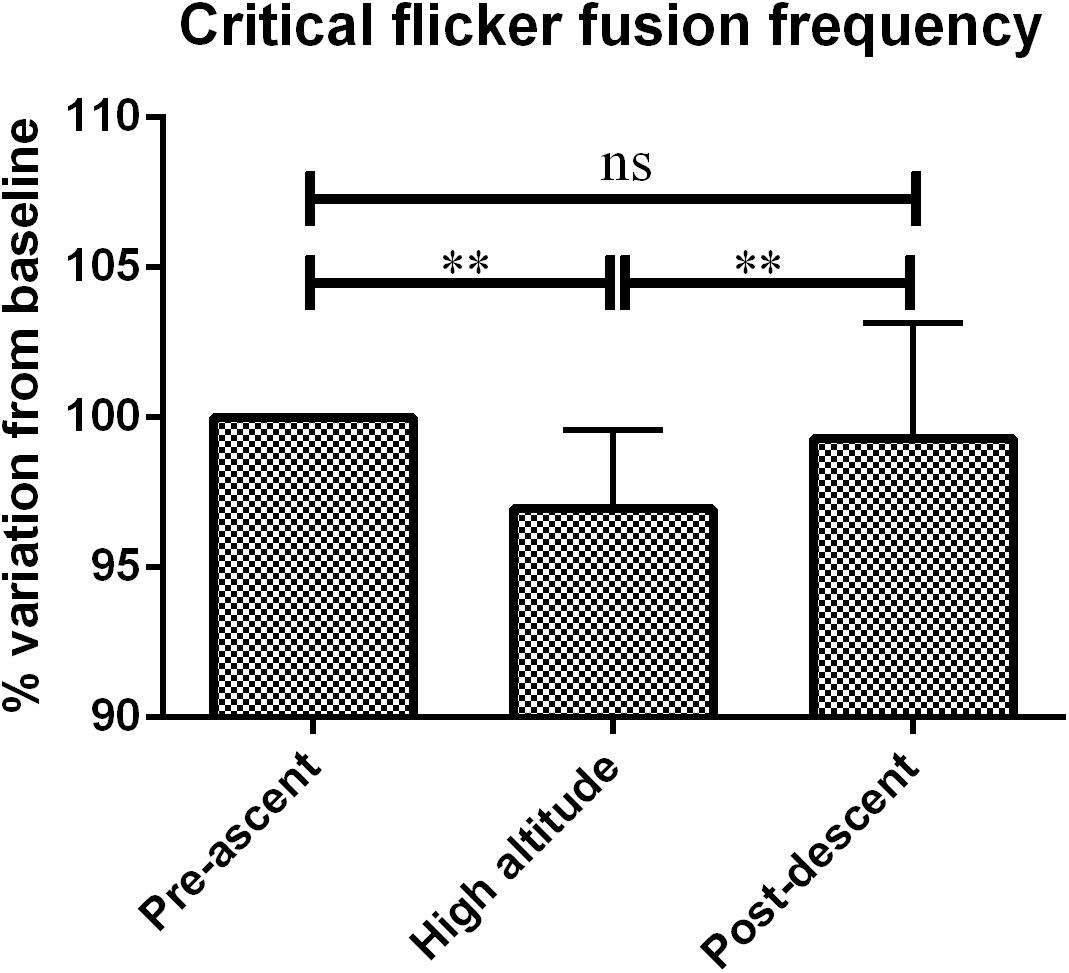
Figure 4. Critical flicker fusion frequency variation according to altitude expressed as percentage variation from baseline. Errors bars represent standard error of the mean (SEM). ∗∗p < 0.05.
PEBL
In the PEBL experiments, there was no statistical difference in all three tests between pre-ascent values, post-ascent values and high-altitude values as showed in Figure 5.
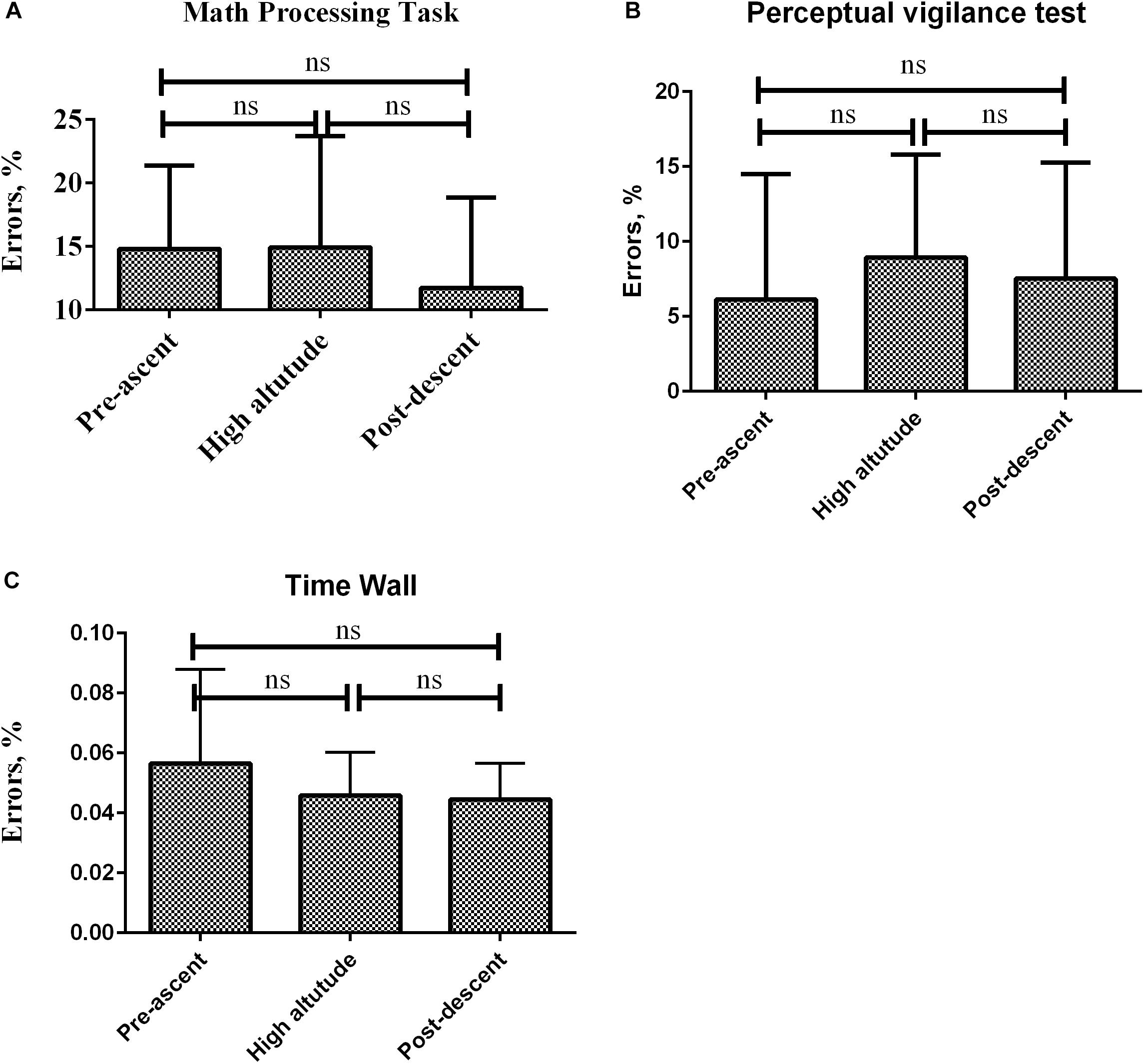
Figure 5. Psychology experiment building language tests variation according to altitude. (A) Math processing task. (B) Perceptual vigilance test. (C) Time wall. Errors bars represent standard error of the mean (SEM).
Discussion
Under acute exposure to hypobaric hypoxic conditions, pulsed oxygen saturation decreased due to a decrease in atmospheric pressure with altitude. This was associated to an increase in heart rate, a physiological countermeasure. Our group showed a decrease in the critical flicker fusion frequency possibly associated to a decrease in arousal. This was linked with an unexpected small but significant decrease in the cerebral blood flow index excluding cerebral blood flow increase as a physiological countermeasure. There was no change in the performances of the Modified Math Processing Task, the Perceptual Vigilance Task and the Time Estimation Task, all part of the Psychology experiment building language tests probably meaning that no cognitive changes were seen in this time frame.
Pulsed oxygen saturation (SpO2) reflected the organism’s hypoxic state. Mean SpO2 significantly decreased at high altitude. This decrease was mainly due to a decrease in oxygen partial pressure in the breathing air (Imray et al., 2010). Acute ascent to high altitude prevented any metabolic acclimatization by the organism to compensate for decreased oxygen, temperature and pressure at high altitude, so SpO2 is a good indicator of the organism’s hypoxic state (Bilo et al., 2012).
The effects of effort/exercise, were not present avoiding some other compensatory mechanisms such as an increased Bohr effect or muscular metabolites build up, known to interfere with respiration (Balestra et al., 2011), nevertheless the complete absence of such mechanisms is not possible, we consider reducing them as much as possible.
Heart rate was increased, possibly by orthosympathetic stimulation through peripheral chemoreceptors (Iturriaga et al., 2016) triggering adrenergic neurotransmitters (Richalet, 2016).
No significant change was seen in systolic, diastolic and mean velocities of the mean cerebral artery (MCA) suggesting that there was no change in vascular diameter. If heart rate increases in high altitude, the absence of changes in MCA mean velocities would suggest an increase in blood flow. This was not what we observed; indeed, we saw a decrease in cerebral blood flow index.
Nevertheless, our results also show a significant decrease in critical flicker fusion frequency reflecting a decrease in cerebral arousal. This has also been seen in hypobaric chamber pilot testing (Truszczynski et al., 2009). Differences in CFFF without changes in psychometric tests could be explained by CFFF interrogating much lesser complex physiological pathways than psychometric tests (Hemelryck et al., 2013). Arousal is triggered by the stimulation of the ascending reticular substance (Jang et al., 2016) and it could be interrogated by CFFF. Furthermore, the decrease in CFFF could be due to decreased cerebral blood flow index Some similar results have been reported during acute orthostatic stimulations altering cerebral blood flow (Balestra et al., 2018). Similar results have been shown when using Cerebral Near Infrared Spectroscopy to assess brain’s oxygenation (Sakudo, 2016). This decrease in cerebral arousal even very early after acute exposure to high altitude should draw caution to unexperienced tourists (Debevec et al., 2019).
A decrease in SpO2 could induce cognitive dysfunction but there were no changes in psychometric testing. This means that cognitive function remains the same in acute hypobaric conditions, at least in the first few hours. Literature shows an increase in visual perception (Benedek et al., 2002), paving the way to hypoxic adaptation of the retina. Cognitive dysfunction has been incriminated as a symptom of acute mountain sickness (Issa et al., 2016).
Oxygen facilitates nerve conduction and interacts with GABA neurotransmission (Rostain and Lavoute, 2016). Recent data obtained from pilot trainees using magnetic resonance spectroscopy demonstrated that higher striatal concentrations of GABA and glutamate/glutamine were related to superior performance in action control allowing differentiation between high and normal performers (Yildiz et al., 2014). High arousal has been reported to decrease proactive control and increase reactive control compared to low arousal (Cudo et al., 2018).
Three hypotheses could explain the fact that we found no differences in PEBL during acute exposure to high altitude. First, the tests in the PEBL were maybe not exploring the more sensitive areas of the brain concerned with hypoxia such as areas involved in memory, accuracy and motor speed (Virues-Ortega et al., 2004) even though Time wall results did not differ at high altitude in our study. Second, complex reaction times could not be altered below higher altitudes (Virues-Ortega et al., 2004). This could explain why CFFF decreased without contemporary decrease of PEBL. Finally, no changes in PEBL could also mean that physiological countermeasures are engaged to maintain cognitive functions. Whether these are due to an increase in oxygen extraction or other mechanisms remains to be seen.
Most of the literature focuses on acclimatization, mainly from very high-altitude mountain expeditions. Recent literature examined cognitive function after acute, subacute and repeated exposure to high altitude (Pun et al., 2018a, b). These studies showed: (1) that acute exposure decreased psychomotor vigilance whereas a 6-day acclimatization prevented impairments during subsequent re-exposure (Pun et al., 2018b); (2) that attention was impaired at high-altitude but improved after acclimatization (Pun et al., 2018a). Sleep disturbance could be an important factor expanding these results (Sforza et al., 2004; Kim et al., 2007). Acute exposure in these articles were very different from our protocol. Indeed, Pun’s groups slept one night at moderate altitude (2950 m) and climbed to 5050 m each day for 1 week. In contrast with the existing literature where cognitive functions were altered (Roach et al., 2014; Davranche et al., 2016; Griva et al., 2017), our protocol focused on very rapid exposure to high altitude before any acclimatization is possible without any night sleep.
Limitations in our study include a limited number of participants, the absence of physical effort and the rather moderate altitude. Strengths include a rigorously controlled environmental conditions, homogeneity of our group, rapid ascent in altitude and the battery of cognitive tests used. The limited number of participants permitted the group to be homogeneous. Physical activity was not considered in the study as the purpose was to explore how tourists using cable cars manage their cognitive function at high altitude.
In our measurement setting, going from velocity to blood flow remains somehow speculative but CBF is nearly impossible to measure non-invasively. To assess cerebral vascular velocity, we used trancranial Doppler. This easy to use, noninvasive technique was transported into the hypobaric chamber. We used the cerebral blood flow index to approximate cerebral blood flow which is validated in septic critically ill patients (Pierrakos et al., 2013). In the Pierrakos trial, measures were made in hemodynamically stable patients. Compensatory hyperventilation inducing cerebral vasoconstriction could also explain a decrease in Cerebral blood flow index. Even if respiratory rate has not been measured in our study, literature is unanimous on this compensatory mechanism (Naeije, 2010; Paralikar and Paralikar, 2010). Some studies have shown an increase in cerebral blood flow in acute hypoxic conditions (Harris et al., 2013; Steinback and Poulin, 2016) even if contradictory data exists (Lawley et al., 2017).
This study is also interesting in a clinical intensive care setting where rapid profound hypoxia can occur very fast.
Conclusion
Acute exposure to hypobaric hypoxia results in decreased oxygen saturation and increased heart rate. Critical flicker fusion frequency decreases at high altitude but Psychology experiment building language tests remain normal throughout the experiment. The decrease in the cerebral blood flow index does not explain the maintenance of cognitive performance and requires further investigation into other compensatory mechanisms such as increased oxygen extraction by brain tissue through near infrared spectroscopy. As all examined variables returned to normal 1 h after descent from high altitude, one could speculate that under acute conditions, critical flicker fusion frequency and cerebral blood flow index modifications may be transitory mechanisms.
Data Availability
The raw data supporting the conclusions of this manuscript will be made available by the authors, without undue reservation, to any qualified researcher.
Ethics Statement
All experimental procedures, conducted in accordance with the Declaration of Helsinki (World Medical Association, 2013), were approved by the Academic Ethical Committee of Brussels (Brussels Alliance for Research and Higher Education, B200-2013-127) and written informed signed consent, a total of seventeen healthy male subjects were included in the study.
Author Contributions
DDB, CP, AB, FR, QC, NM, GB, DV, CB, PH, and ST made a substantial contributions to the conception or design of the work, acquisition, analysis, and interpretation of data for the work, drafted the work, and revised it critically for the important intellectual content and approved the final version of the manuscript and agreed to be accountable for all aspects of the work in ensuring that questions related to the accuracy or integrity of any part of the work are appropriately investigated and resolved.
Conflict of Interest Statement
The authors declare that the research was conducted in the absence of any commercial or financial relationships that could be construed as a potential conflict of interest.
Acknowledgments
We gratefully acknowledge Emmanuel Cauchy2, M.D, Head of Ifremmont (French Institute for Mountain Research) who helped during our first experiment in Chamonix.
Footnotes
- ^ http://pebl.sourceforge.net
- ^ Deceased
References
Aaslid, R., Markwalder, T. M., and Nornes, H. (1982). Noninvasive transcranial Doppler ultrasound recording of flow velocity in basal cerebral arteries. J. Neurosurg. 57, 769–774.
Balestra, C., Levenez, M., Lafere, P., Dachy, B., Ezquer, M., and Germonpre, P. (2011). Respiratory rate can be modulated by long-loop muscular reflexes, a possible factor in involuntary cessation of apnea. Diving Hyperb. Med. 41, 3–8.
Balestra, C., Machado, M. L., Theunissen, S., Balestra, A., Cialoni, D., Clot, C., et al. (2018). Critical flicker fusion frequency: a marker of cerebral arousal during modified gravitational conditions related to parabolic flights. Front. Physiol. 9:1403. doi: 10.3389/fphys.2018.01403
Benedek, K., Keri, S., Grosz, A., Totka, Z., Toth, E., and Benedek, G. (2002). Short-term hypobaric hypoxia enhances visual contrast sensitivity. Neuroreport 13, 1063–1066.
Bilo, G., Revera, M., Bussotti, M., Bonacina, D., Styczkiewicz, K., Caldara, G., et al. (2012). Effects of slow deep breathing at high altitude on oxygen saturation, pulmonary and systemic hemodynamics. PLoS One 7:e49074. doi: 10.1371/journal.pone.0049074
Brebeck, A.-K., Deussen, A., Schmitz-Peiffer, H., Range, U., Balestra, C., Cleveland, S., et al. (2017). Effects of oxygen-enriched air on cognitive performance during SCUBA-diving – an open-water study. Res. Sports Med. 25, 1–12. doi: 10.1080/15438627.2017.1314289
Cudo, A., Francuz, P., Augustynowicz, P., and Strozak, P. (2018). The effects of arousal and approach motivated positive affect on cognitive control. An ERP Study. Front. Hum. Neurosci. 12:320. doi: 10.3389/fnhum.2018.00320
Davranche, K., Casini, L., Arnal, P. J., Rupp, T., Perrey, S., and Verges, S. (2016). Cognitive functions and cerebral oxygenation changes during acute and prolonged hypoxic exposure. Physiol. Behav. 164, 189–197. doi: 10.1016/j.physbeh.2016.06.001
de Riva, N., Budohoski, K. P., Smielewski, P., Kasprowicz, M., Zweifel, C., Steiner, L. A., et al. (2012). Transcranial Doppler pulsatility index: what it is and what it isn’t. Neurocrit. Care 17, 58–66. doi: 10.1007/s12028-012-9672-6
Debevec, T., Pialoux, V., Millet, G. P., Martin, A., Mramor, M., and Osredkar, D. (2019). Exercise overrides blunted hypoxic ventilatory response in prematurely born men. Front. Physiol. 10:437. doi: 10.3389/fphys.2019.00437
Dobashi, S., Horiuchi, M., Endo, J., Kiuchi, M., and Koyama, K. (2016). Cognitive function and cerebral oxygenation during prolonged exercise under hypoxia in healthy young males. High Alt. Med. Biol. 17, 214–221.
Engan, H. K., Jones, A. M., Ehrenberg, F., and Schagatay, E. (2012). Acute dietary nitrate supplementation improves dry static apnea performance. Respir. Physiol. Neurobiol. 182, 53–59. doi: 10.1016/j.resp.2012.05.007
Feddersen, B., Neupane, P., Thanbichler, F., Hadolt, I., Sattelmeyer, V., Pfefferkorn, T., et al. (2015). Regional differences in the cerebral blood flow velocity response to hypobaric hypoxia at high altitudes. J. Cereb. Blood Flow Metab. 35, 1846–1851. doi: 10.1038/jcbfm.2015.142
Fuentes, J. P., Villafaina, S., Collado-Mateo, D., De La Vega, R., Gusi, N., and Clemente-Suarez, V. J. (2018). Use of biotechnological devices in the quantification of psychophysiological workload of professional chess players. J. Med. Syst. 42:40. doi: 10.1007/s10916-018-0890-0
Germonpre, P., Balestra, C., Hemelryck, W., Buzzacott, P., and Lafere, P. (2017). Objective vs. subjective evaluation of cognitive performance during 0.4-MPa dives breathing air or nitrox. Aerosp. Med. Hum. Perform. 88, 469–475. doi: 10.3357/AMHP.4608.2017
Griva, K., Stygall, J., Wilson, M. H., Martin, D., Levett, D., Mitchell, K., et al. (2017). Caudwell xtreme everest: a prospective study of the effects of environmental hypoxia on cognitive functioning. PLoS One 12:e0174277. doi: 10.1371/journal.pone.0174277
Harris, A. D., Murphy, K., Diaz, C. M., Saxena, N., Hall, J. E., Liu, T. T., et al. (2013). Cerebral blood flow response to acute hypoxic hypoxia. NMR Biomed. 26, 1844–1852. doi: 10.1002/nbm.3026
Hemelryck, W., Rozloznik, M., Germonpre, P., Balestra, C., and Lafere, P. (2013). Functional comparison between critical flicker fusion frequency and simple cognitive tests in subjects breathing air or oxygen in normobaria. Diving Hyperb. Med. 43, 138–142.
Imray, C., Wright, A., Subudhi, A., and Roach, R. (2010). Acute mountain sickness: pathophysiology, prevention, and treatment. Prog. Cardiovasc. Dis. 52, 467–484. doi: 10.1016/j.pcad.2010.02.003
Issa, A. N., Herman, N. M., Wentz, R. J., Taylor, B. J., Summerfield, D. C., and Johnson, B. D. (2016). Association of cognitive performance with time at altitude, sleep quality, and acute mountain sickness symptoms. Wilderness Environ. Med. 27, 371–378. doi: 10.1016/j.wem.2016.04.008
Iturriaga, R., Del Rio, R., Idiaquez, J., and Somers, V. K. (2016). Carotid body chemoreceptors, sympathetic neural activation, and cardiometabolic disease. Biol. Res. 49:13. doi: 10.1186/s40659-016-0073-8
Jang, S. H., Chang, C. H., Jung, Y. J., and Seo, Y. S. (2016). Change of ascending reticular activating system with recovery from vegetative state to minimally conscious state in a stroke patient. Medicine 95:e5234.
Kahlbrock, N., Butz, M., May, E. S., Brenner, M., Kircheis, G., Haussinger, D., et al. (2012). Lowered frequency and impaired modulation of gamma band oscillations in a bimodal attention task are associated with reduced critical flicker frequency. Neuroimage 61, 216–227. doi: 10.1016/j.neuroimage.2012.02.063
Kim, H., Dinges, D. F., and Young, T. (2007). Sleep-disordered breathing and psychomotor vigilance in a community-based sample. Sleep 30, 1309–1316.
Lafere, P., Balestra, C., Hemelryck, W., Guerrero, F., and Germonpre, P. (2016). Do environmental conditions contribute to narcosis onset and symptom severity? Int. J. Sports Med. 37, 1124–1128.
Lankford, H. V. (2014). Extreme altitude: words from on high. Wilderness Environ. Med. 25, 346–351. doi: 10.1016/j.wem.2014.03.010
Lawley, J. S., Macdonald, J. H., Oliver, S. J., and Mullins, P. G. (2017). Unexpected reductions in regional cerebral perfusion during prolonged hypoxia. J. Physiol. 595, 935–947. doi: 10.1113/JP272557
Lee, Y. A., and Goto, Y. (2015). Chronic stress effects on working memory: association with prefrontal cortical tyrosine hydroxylase. Behav. Brain Res. 286, 122–127. doi: 10.1016/j.bbr.2015.03.007
Lefferts, W. K., Deblois, J. P., White, C. N., Day, T. A., Heffernan, K. S., and Brutsaert, T. D. (2019). Changes in cognitive function and latent processes of decision-making during incremental ascent to high altitude. Physiol. Behav. 201, 139–145. doi: 10.1016/j.physbeh.2019.01.002
Li, X. Y., Wu, X. Y., Fu, C., Shen, X. F., Yang, C. B., and Wu, Y. H. (2000). Effects of acute exposure to mild or moderate hypoxia on human psychomotor performance and visual-reaction time. Space Med. Med. Eng. 13, 235–239.
Loo, S. K., Hale, T. S., Macion, J., Hanada, G., Mcgough, J. J., Mccracken, J. T., et al. (2009). Cortical activity patterns in ADHD during arousal, activation and sustained attention. Neuropsychologia 47, 2114–2119. doi: 10.1016/j.neuropsychologia.2009.04.013
Ma, J., Ma, R. M., Liu, X. W., Bian, K., Wen, Z. H., Li, X. J., et al. (2014). Workload influence on fatigue related psychological and physiological performance changes of aviators. PLoS One 9:e87121. doi: 10.1371/journal.pone.0087121
Malle, C., Quinette, P., Laisney, M., Bourrilhon, C., Boissin, J., Desgranges, B., et al. (2013). Working memory impairment in pilots exposed to acute hypobaric hypoxia. Aviat. Space Environ. Med. 84, 773–779.
Mewborn, C., Renzi, L. M., Hammond, B. R., and Miller, L. S. (2015). Critical flicker fusion predicts executive function in younger and older adults. Arch. Clin. Neuropsychol. 30, 605–610. doi: 10.1093/arclin/acv054
Naeije, R. (2010). Physiological adaptation of the cardiovascular system to high altitude. Prog. Cardiovasc. Dis. 52, 456–466. doi: 10.1016/j.pcad.2010.03.004
Paralikar, S. J., and Paralikar, J. H. (2010). High-altitude medicine. Indian J. Occup. Environ. Med. 14, 6–12. doi: 10.4103/0019-5278.64608
Pierrakos, C., Antoine, A., Velissaris, D., Michaux, I., Bulpa, P., Evrard, P., et al. (2013). Transcranial doppler assessment of cerebral perfusion in critically ill septic patients: a pilot study. Ann. Intensive Care 3:28. doi: 10.1186/2110-5820-3-28
Piper, B. J., Mueller, S. T., Geerken, A. R., Dixon, K. L., Kroliczak, G., Olsen, R. H., et al. (2015). Reliability and validity of neurobehavioral function on the psychology experimental building language test battery in young adults. PeerJ. 3:e1460. doi: 10.7717/peerj.1460
Pun, M., Guadagni, V., Bettauer, K. M., Drogos, L. L., Aitken, J., Hartmann, S. E., et al. (2018a). Effects on cognitive functioning of acute, subacute and repeated exposures to high altitude. Front. Physiol. 9:1131. doi: 10.3389/fphys.2018.01131
Pun, M., Hartmann, S. E., Furian, M., Dyck, A. M., Muralt, L., Lichtblau, M., et al. (2018b). Effect of acute, subacute, and repeated exposure to high altitude (5050 m) on psychomotor vigilance. Front. Physiol. 9:677. doi: 10.3389/fphys.2018.00677
Richalet, J. P. (2016). Physiological and clinical implications of adrenergic pathways at high altitude. Adv. Exp. Med. Biol. 903, 343–356. doi: 10.1007/978-1-4899-7678-9_23
Roach, E. B., Bleiberg, J., Lathan, C. E., Wolpert, L., Tsao, J. W., and Roach, R. C. (2014). AltitudeOmics: decreased reaction time after high altitude cognitive testing is a sensitive metric of hypoxic impairment. Neuroreport 25, 814–818. doi: 10.1097/WNR.0000000000000169
Rocco, M., Pelaia, P., Di Benedetto, P., Conte, G., Maggi, L., Fiorelli, S., et al. (2019). Inert gas narcosis in scuba diving, different gases different reactions. Eur. J. Appl. Physiol. 119, 247–255. doi: 10.1007/s00421-018-4020-y
Rostain, J. C., and Lavoute, C. (2016). Dopamine, neurochemical processes, and oxygen toxicity at pressure. Compr. Physiol. 6, 1339–1344. doi: 10.1002/cphy.c140025
Sakudo, A. (2016). Near-infrared spectroscopy for medical applications: current status and future perspectives. Clin. Chim. Acta 455, 181–188. doi: 10.1016/j.cca.2016.02.009
Sforza, E., Haba-Rubio, J., De Bilbao, F., Rochat, T., and Ibanez, V. (2004). Performance vigilance task and sleepiness in patients with sleep-disordered breathing. Eur. Respir. J. 24, 279–285.
Sharma, P., Singh, S., Sharma, B. C., Kumar, M., Garg, H., Kumar, A., et al. (2011). Propofol sedation during endoscopy in patients with cirrhosis, and utility of psychometric tests and critical flicker frequency in assessment of recovery from sedation. Endoscopy 43, 400–405. doi: 10.1055/s-0030-1256182
Steinback, C. D., and Poulin, M. J. (2016). Influence of hypoxia on cerebral blood flow regulation in humans. Adv. Exp. Med. Biol. 903, 131–144. doi: 10.1007/978-1-4899-7678-9_9
Thomann, J., Baumann, C. R., Landolt, H. P., and Werth, E. (2014). Psychomotor vigilance task demonstrates impaired vigilance in disorders with excessive daytime sleepiness. J. Clin. Sleep Med. 10, 1019–1024. doi: 10.5664/jcsm.4042
Truszczynski, O., Wojtkowiak, M., Biernacki, M., and Kowalczuk, K. (2009). The effect of hypoxia on the critical flicker fusion threshold in pilots. Int. J. Occup. Med. Environ. Health 22, 13–18. doi: 10.2478/v10001-009-0002-y
Virues-Ortega, J., Buela-Casal, G., Garrido, E., and Alcazar, B. (2004). Neuropsychological functioning associated with high-altitude exposure. Neuropsychol. Rev. 14, 197–224.
World Medical Association (2013). World medical association declaration of Helsinki: ethical principles for medical research involving human subjects. JAMA 310, 2191–2194.
Keywords: acute hypoxia, cognitive function, PEBL, transcranial doppler, cerebral blood flow index
Citation: De Bels D, Pierrakos C, Bruneteau A, Reul F, Crevecoeur Q, Marrone N, Vissenaeken D, Borgers G, Balestra C, Honoré PM and Theunissen S (2019) Variation of Cognitive Function During a Short Stay at Hypobaric Hypoxia Chamber (Altitude: 3842 M). Front. Physiol. 10:806. doi: 10.3389/fphys.2019.00806
Received: 27 February 2019; Accepted: 06 June 2019;
Published: 26 June 2019.
Edited by:
Ginés Viscor, University of Barcelona, SpainReviewed by:
Vittore Verratti, G. d’Annunzio University of Chieti-Pescara, ItalyMatiram Pun, University of Calgary, Canada
Marcos Serrano-Dueñas, Pontificia Universidad Católica del Ecuador, Ecuador
Copyright © 2019 De Bels, Pierrakos, Bruneteau, Reul, Crevecoeur, Marrone, Vissenaeken, Borgers, Balestra, Honoré and Theunissen. This is an open-access article distributed under the terms of the Creative Commons Attribution License (CC BY). The use, distribution or reproduction in other forums is permitted, provided the original author(s) and the copyright owner(s) are credited and that the original publication in this journal is cited, in accordance with accepted academic practice. No use, distribution or reproduction is permitted which does not comply with these terms.
*Correspondence: D. De Bels, ZGF2aWQuZGViZWxzQGNodS1icnVnbWFubi5iZQ==