- 1Key Laboratory of Exploration and Utilization of Aquatic Genetic Resources, College of Fisheries and Life Science, Shanghai Ocean University, Shanghai, China
- 2National Demonstration Center for Experimental Fisheries Science Education, Shanghai Ocean University, Shanghai, China
- 3Co-Innovation Center of Jiangsu Marine Bio-Industry Technology, Jiangsu Ocean University, Lianyungang, China
- 4Shanghai Engineering Research Center of Aquaculture, Shanghai, China
To facilitate transplanting razor clam (Sinonovacula constricta) populations to inland saline-alkaline waters (ISWs), we evaluated the tolerance of juvenile S. constricta (JSC) to Ca2+ and Mg2+ concentrations, and determined the effects of these ions on JSC growth and physiological parameters. After 30 days stress, the tolerable ranges of JSC to Ca2+ and Mg2+ were determined to be 0.19 mmol⋅L-1–19.46 mmol⋅L-1 and 0 mmol⋅L-1–29.54 mmol⋅L-1, respectively. The concentrations of Ca2+ (less than 0.65 mmol⋅L-1 or more than 3.24 mmol⋅L-1) and Mg2+ (less than 0.37 mmol⋅L-1 or more than 14.17 mmol⋅L-1) significantly inhibit JSC growth. Physiological enzyme activity no significant response when the concentrations range of Ca2+ and Mg2+ are 0.93 mmol⋅L-1–6.49 mmol⋅L-1 and 0.37 mmol⋅L-1–14.77 mmol⋅L-1, respectively. For transplantation practice, these data indicate that only high concentrations of Ca2+ (3.24–6.825 mmol⋅L-1) and Mg2+ (14.77–33.69 mmol⋅L-1) in target inland saline-alkaline water had significantly impact on growth and physiological response. In addition, present study suggests that the increase in Ca2+ and Mg2+ ion concentrations caused by ocean acidification will not affect the survival, growth and physiology of S. constricta. Current research suggests that S. constricta can adapt to extreme changes in the marine environment (Ca2+ and Mg2+) and may be an excellent candidate for inland saline-alkaline water transplantation practice.
Introduction
Saline-alkali soil and inland saline-alkaline waters (ISWs) exist in more than 100 countries (Shi, 2009). In China, there are approximately 1 million km2 of saline-alkaline soil and approximately 0.3 million km2 of ISW (He et al., 2010). Saline water resources have long been used for agriculture, but traditional terrestrial crops are not salt-tolerant and cannot be irrigated with salt water. Therefore, ISWs are often low-yielding land (Qadir et al., 2001; Wang et al., 2011). The use of ISWs to culture economically important marine organisms represents an effective means to use inland saline-alkali resources, such as the most successful species Litopenaeus vannamei (Roy et al., 2007, 2010). Inland aquaculture of economically important marine organisms worldwide is mainly concentrated in ISW areas, and is practiced in several countries. However, there are few reports on marine mollusc transplanting in ISWs; these reports only describe aquaculture of Mytilus edulis (Dinh and Fotedar, 2016), Ruditapes philippinarum (Hiele et al., 2014), Haliotis laevigata (Doupé et al., 2003), Trochus niloticus (Lee, 1997), and Crassostrea gigas and Saccostrea glomerata (Ingram et al., 2015).
In China, some ISWs have been used for aquaculture, but most of them have historically been in a state of disuse (Lv et al., 2012). According to incomplete statistics, more than 33 economically important organisms are cultured in ISWs in China (mainly freshwater fish) (Zhang and Zhang, 1999). However, the practice of aquaculture of economically important marine mollusc in ISW has only been attempted with Cyclaina sinensis (Lin et al., 2012) in China; this venture ultimately did not succeed.
The ion composition of ISW is quite different from that of seawater. Monovalent and divalent cations and their interactions have an important influence on the physiology of organisms (Duchen, 2000). All of the main ionic components in ISW (Ca2+, Mg2+, Na+, K+, SO42-, HCO3-, OH-, Cl-, Ca2+: Mg2+, Na+: K+, SO42-: Cl-) are factors that affect the survival and growth of aquatic animals (Hadfield et al., 2012; Hiele et al., 2014; Raizada et al., 2015). Ca2+ is a crucial element of the outer shell of marine crustaceans and mollusc, is involved in muscle activity, shell formation, neurotransmission, and osmotic regulation, and plays an important role in the growth of marine invertebrates such as crustaceans and mollusc (Wilbur and Yonge, 2013; Yang, 2011). Mg2+ is found in the active region of many enzymes, it is a metabolic cofactor, and is an important participant in the active transport of substances involved in maintenance of the cell membrane. Studies have shown that Mg2+ has an important effect on the survival, growth, and osmotic pressure of marine crustaceans (Mantel and Farmer, 1983). The imbalance of ionic components in ISW tends to affect aquaculture practices more than salinity, as demonstrated in L. vannamei by Saoud et al. (2003). In addition, it has been reported that the high content of Mg2+, Ca2+, and K+, and low Ca2+/Mg2+ and Na+/K+ ratios in ISW affect the survival of cultured L. vannamei.
Many marine invertebrates are osmotic conformers: the osmotic concentration (and usually the ionic composition) of the extracellular fluid is similar to that of the ambient seawater (Larsen et al., 2014). Thus, in aquatic mollusc can be found the stenohaline, euryhaline or oligohaline osmotic conformers, and, also the freshwater bivalves (Deaton, 2008). Most of the intertidal zone bivalve belongs to the euryhaline osmotic conformers, and usually their conformational floor are reported to be between 0.7 ppt and 3.4 ppt (Otto and Pierce, 1981; Deaton, 1992, 2008). There is also volume regulation in marine mollusc, the process that returns the cytoplasmic and extracellular fluid compartments to osmotic equilibrium with the ambient medium (Smith and Pierce, 1987; Deaton, 1992; Larsen et al., 2014). The capacity for volume regulation in marine osmotic conformers varies from limited to high (some molluscs) (Virkar, 1966; Pierce, 1971; Gainey, 1978). Although the study of osmotic and ionic regulation in bivalve is not sufficient, Ca2+ and Mg2+ play an important role in this system.
The Ca2+ and Mg2+ ion contents vary widely in ISWs in different regions of China. For example, the Ca2+ content of ISWs in Haolebaojinao (Wu Shen banner, Ordos City, the Inner Mongolia Autonomous Region, China) is only 0.199 mmol⋅L-1 of seawater (Tian, 2000). In contrast, the Ca2+ content of Bosten Lake is 5.61 mmol⋅L-1 that of seawater with the same salinity (Shi, 1989). Shi (2009) found that the Mg2+ content in ISW in the middle reaches of the Yellow River in China was 2.33–8.15 mmol⋅L-1. Therefore, studying the effects of Ca2+ and Mg2+ on marine invertebrates can help facilitate transplanting marine invertebrates in China ISW.
Sinonovacula constricta is a eurythermal and euryhaline marine mollusc that is distributed in estuary and intertidal zones (Lin and Wu, 1990). S. constricta feeds on microalgae, and fixes calcium ions in its aquatic environment during the formation of its shell, which can contribute to the improvement of an ISW environment. Peng et al. (2018) reported that S. constricta tolerate high pH and carbonate alkalinity under long-term stress, and suggested that S. constricta is a potentially good species for culture in ISW. However, the effects of different concentrations of Ca2+ and Mg2+ on the survival, growth, and physiological responses of S. constricta have not been reported. In addition, ocean acidification and climate warming also directly affect the concentration of Ca2+ and Mg2+ ions in the ocean (Orr et al., 2005). Therefore, understanding the critical tolerances and physiological responses of S. constricta to Ca2+ and Mg2+ stress is crucial for the development of optimal ISW culture conditions and ocean environments changing. Thus, this study investigated the effects of Ca2+ and Mg2+ on the survival, growth, and physiological responses of juvenile S. constricta (JSC), and the possibility of this species as a potential candidate for transplantation to ISWs, providing a theoretical basis for this process.
Materials and Methods
Experimental animals were treated according to the guidelines of the Institutional Animal Care and Use Committee of Shanghai Ocean University (IACUC-SHOU), Shanghai, China, under examination and approval number SHOU-DW-2018-014. In this tests the animals were artificially propagated as juveniles.
Healthy JSCs (20 days old) were obtained commercially (Zhejiang Sanmen Donghang). Before experimentation, all JSCs were kept in at 8 ppt seawater for 3 days, then were kept at 6 ppt artificial seawater (Ca2+: 1.71 mmol⋅L-1, Mg2+: 9.23 mmol⋅L-1) for 4 days. In these conditions the total salinity of water is set at 6 ppt, since the salinity of ISW (carbonate type) in China is around 6 ppt (Yao et al., 2010). Artificial seawater was formulated using chemical reagents (AR degree, Sangon Biotech) and distilled water according to the Artificial Seawater Recipe (Chu) in Table 1A (Harvey, 1957; Yao et al., 2010). Approximately 10,000 specimens of JSCs were obtained before experiments, and then 270 JSCs were randomly selected to measure shell length (0.2102 ± 0.058 cm) and body weight (0.000892 ± 0.00015 g). Water temperature in experiments was maintained between 20 to 22°C.
Long-Term Stress Study
Juvenile S. constrictas were divided into 29 groups for long-term stress testing (Table 1B), consisting of a control group (CK), 14 groups with different concentrations of Mg2+ (DCMg: G1-G14), and 14 groups with different concentrations of Ca2+ (DCCa: G1–G14). Each of the 29 groups had 3 parallel trials. For each parallel stress trial, 90 JSCs were cultured in an 11 cm diameter round plate for a month. 150 mL test water (with the appropriate salinity and Ca2+ or Mg2+ concentration) was added to the round plate, and the test water was changed once every single day. Feed-water was prepared as follows: test water was used to replace the culture water of Chaetoceros calcitrans by centrifugation, and the concentration of C. calcitrans was adjusted to 400–480 cells μL-1 in test water. For every single feeding, 15 mL feed-water was used to replace 15 mL of test water. JSCs were fed thrice a day.
Live JSCs were counted at day 0 (0-day) and at day 30 (30-day). At 0-day, 36 JSCs (6 repeats: 6 JSCs per repeat) were selected to determine the activities of the following enzymes: Na+/K+-ATPase (NKA), aspartate aminotransferase (AST), superoxide dismutase (SOD), acetylcholinesterase (AChE), and lysozyme (LZM). At 30-day, live JSCs were used to measure shell length, body weight, and enzymes activity (NKA, SOD, AST, AChE, and LZM). Nine JSCs (representing three separate tests with three JSCs in each group) were used to measure enzyme activities. Briefly, after shell removal, JSC tissue was homogenized in normal saline water in a ratio of 1 mg: 9 μl. After homogenization, the total protein content of the sample was measured by Coomassie Brilliant Blue Total Protein Assay kit (Nanjing Jiancheng Bioengineering Institute, China), according to the manufacturer’s instructions. NKA, AST, SOD, AChE, and LZM enzyme activities were measured by corresponding kits from Nanjing Jiancheng Bioengineering Institute Ltd., as previously described (Peng et al., 2018).
Na+/K+-ATPase activity was measured by Pi released from ATP, in the incubation solution containing: 100 mM NaCl, 20 mM KCl, 4 mM MgCl2, 1 mM EGTA, 0.2 mM 2-methyl-8-(phenylmethoxy)imidazol(1,2-α) pyridine-3-acetonitrile 40 mM Tris–HCl (pH 7.4) and 3 mM Na2ATP. The incubation time with substrate was 10 min. Pi concentration was calculated from the regression line based on standard Pi solutions. The units of NKA activity are expressed as U (μmol Pi mg prot-1 h-1). AST can transfer amino and keto groups between alpha ketoglutarate and aspartic acid, then generate glutamic acid, and oxaloacetate. Oxaloacetate can be decarboxylated to pyruvic acid by itself during the reaction. Pyruvic acid reacts with 2,4-Dinitrophenylhydrazine to form 2,4-Dinitrophenylhydrazone, which is reddish brown in an alkaline solution. After measuring the OD, the standard curve was checked to obtain AST activity, which is expressed as U (μmol Pyruvic acid mg prot-1 min-1). SOD activity was tested using SOD assay kit by WST-1 method. The WST-1 method depends on produces a water-soluble formazan dye upon reduction with the superoxide anion. The rate of the reduction of WST-1 with O2- are linearly related to the xanthine oxidase activity, and this reduction is inhibited by SOD. Therefore, the 50% inhibition activity of SOD can be determined by the colorimetric method. The SOD activity is expressed as units per mg of protein (U mg prot-1), where the one U represents the amount of enzyme required to achieve a SOD inhibition rate of 50%. AChE hydrolyzes acetylcholine to produce choline and acetic acid. Choline can react with sulfhydryl chromogenic reagent to form Sym-Trinitrobenzene (yellow compound), after measuring the OD, the standard curve was checked to obtain AChE activity, which is expressed as units per mg of protein (U mg prot-1), where the one U represents at 37°C for 6 min of reaction conditions, the amount of enzyme required to hydrolyze 1 mmol of acetylcholine. The activity of LZM was detected using a turbidimetric method, which light transmittance of the bacterial turbid solution. Lysozyme can lysis bacteria by hydrolyze peptidoglycan on the bacterial wall to increase the light transmission of the bacterial turbid solution. After measuring the light transmission, the standard curve was checked to obtain LZM activity, which is expressed as units per mg of protein (U mg prot-1), where the one U equivalent to the activity capacity of standard LZM. All data of enzyme activities are ultimately represented as a ratio of 30-day to 0-day.
Acute Stress Study
Within 3 days of the start of the long-term stress study, we found that all JSCs died in the following groups: DCMg-G10, DCMg-G11, DCMg-G12, DCMg-G13, DCMg-G14, DCCa-G13, and DCCa-G14. To more fully explain the physiological effects of Ca2+ and Mg2+ on JSCs, we designed and conducted an acute stress study. The CK, DCMg-G1, DCMg-G10, DCCa-G1, and DCCa-G13 were used in the acute stress experiment. In this experiment, the animals were exposed to the same conditions used in the long-term stress tests, except that the clams were not fed algae. At 0-h, 12-h, 24-h, and 48-h, ten JSCs from each group were used to measure oxygen consumption, then the hemolymph of JSCs was collected, as previously reported (Peng et al., 2017), to separate haemocytes and to measure metabolic and phagocytic activities.
The method used for measuring oxygen consumption rate was previously reported (Peng et al., 2019). After hemolymph collection, 20 μL of JSC haemocyte suspension solution was diluted with TBS to 100 μL. Then 10 μL of 1/10 diluted carboxylate-modified microspheres (diameter 1 μm, yellow-green fluorescent, FluoSpheres®, Invitrogen) was added, and samples were incubated in the dark at room temperature for 1 h (Wang et al., 2014). A BD C6Plus (BD Biosciences, United States) flow cytometer was used to measure the phagocytosis of haemocytes. The same haemocyte concentration without fluorescent microspheres in each group was analyzed by flow cytometry to obtain the cell concentration, and we set the threshold to be Gate A (cell only). The relative size of haemocytes (FSC value) and FITC fluorescence intensity (FITC value) were selected to limit the data. Finally, the data was extracted for analysis and figure mapping.
The metabolic activity of haemocytes was measured with a Cell Counting Kit-8 (CCK-8, Dojindo, Japan) assay. In every parallel group, 20 μL haemocyte suspension solution was diluted with TBS to 200 μL. Then, 20 μL of CCK-8 reagent was added and incubated at 28°C for 3 h. For the CK, 20 μL serum was obtained from the haemocyte suspension solution, and was diluted with TBS to 200 μL; then 0 μL of CCK-8 was added and incubated at 28°C for 3 h. After the OD value was measured, the relative metabolic activity was calculated using OD value and the total number of haemocytes (obtained from the phagocytosis test).
Calculation and Data Analytical Methods
Survival rate (SR):
N30 and N0 are the numbers of surviving individuals in the 30-day and 0-day groups, respectively.
Shell length growth rate (SGR):
L30 and L0 are the average lengths of the individuals in the 30-day and 0-day groups, respectively. T is 30 days.
Weight gain rate (WG):
W30 and W0 are the average wet body weights of the individuals in the 30-day and 0-day groups, respectively. T is 30 days.
Oxygen consumption rate:
Phagocytosis rate and relative metabolic activity:
Ht and Hu are the total haemocyte and un-phagocytic haemocyte numbers, respectively. ODG, ODGB, and HtG are the OD of test sample, the blank OD of test sample, and the total haemocyte counts of the test sample, respectively. ODCK, ODCKB, and HtCK are the OD of control sample, the blank OD of control sample and the total haemocyte count of the control sample, respectively.
Statistical Analysis
Sigmaplot 12.3 software was used to identify significant differences analysis and to plot results. Significant differences were analyzed by using single factor analysis of variance (one-way ANOVA analysis) and the LSD method.
Results
Survival and Growth of JSC Under Long-Term Ca2+ or Mg2+ Stress
During the long-term stress, we found that the concentrations of Mg2+ (higher than 73.86 mmol L-1) and Ca2+ (higher than 207.55 mmol L-1) cause JSCs to die rapidly (all JSC death groups are listed in Table 2). The concentrations of Ca2+ (lower than 0.093 mmol L-1) causes JSCs to die over an extended period of time. Meanwhile, we observed that the bodies of JSCs were lacked calcium carbonate shells in groups stressed with Ca2+ concentrations lower than 0.093 mmol L-1. However, the JSCs stressed with low concentrations of Mg2+ remained viable (Figure 1). In the range of Mg2+ concentration from 0 mmol L-1 to 29.54 mmol L-1, there is a trend of JSC survival ratio show as first rise and then fall. Similarly, in the range of Ca2+ concentration from 0.19 mmol L-1 to19.46 mmol L-1, there is a trend for JSC survival to first rise and then fall. The Mg2+ concentration from 0.18 mmol L-1 to 3.69 mmol L-1 and Ca2+ concentration from 0.93 mmol L-1 to 2.32 mmol L-1 showed no significant differences in survival compared to the CK.
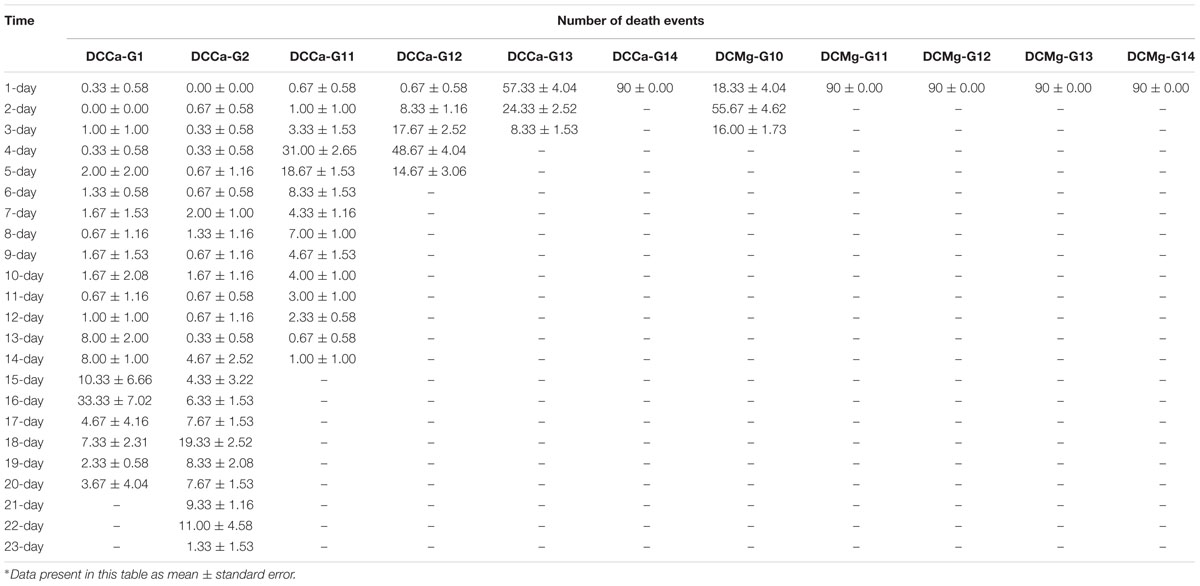
Table 2. Detailed juvenile Sinonovacula constricta mortality information in the test groups which cannot survive under long-term stress with different Ca2+ and Mg2+ concentrations.
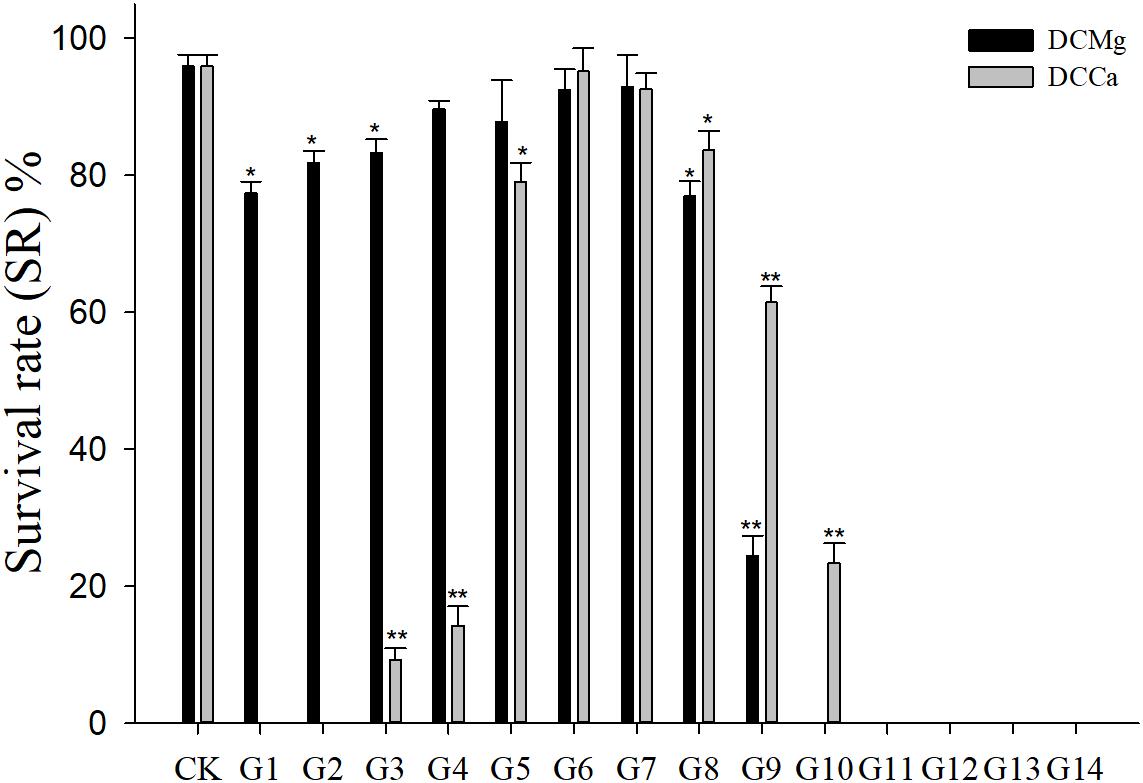
Figure 1. Survival rates of JSCs under long-term stress with different Ca2+ and Mg2+ concentrations. Bars (mean ± SE, n = 3) with an asterisk denote a significant difference (∗P < 0.05, ∗∗P < 0.001) between the test groups (G1–G14) and control group (CK).
The shell growth rate was significantly inhibited in the Mg2+ concentration from 0 mmol L-1 to 0.37 mmol L-1 (P < 0.05), and from 14.77 mmol L-1 to 29.54 mmol L-1 (P < 0.001), and was significantly inhibited in the Ca2+ concentration 0.19–0.65 mmol⋅L-1 (P < 0.05) and 6.49–19.46 mmol⋅L-1 (P < 0.05) (Figure 2). The body weight gain rate was significantly inhibited in the Mg2+ concentration 0–0.37 mmol⋅L-1 (P < 0.05) and 14.77–29.54 mmol⋅L-1 (P < 0.001), and was significantly inhibited in the Ca2+ concentration 0.19–0.65 mmol⋅L-1 (P < 0.05) and 3.24–19.46 mmol⋅L-1 (P < 0.05) (Figure 3).
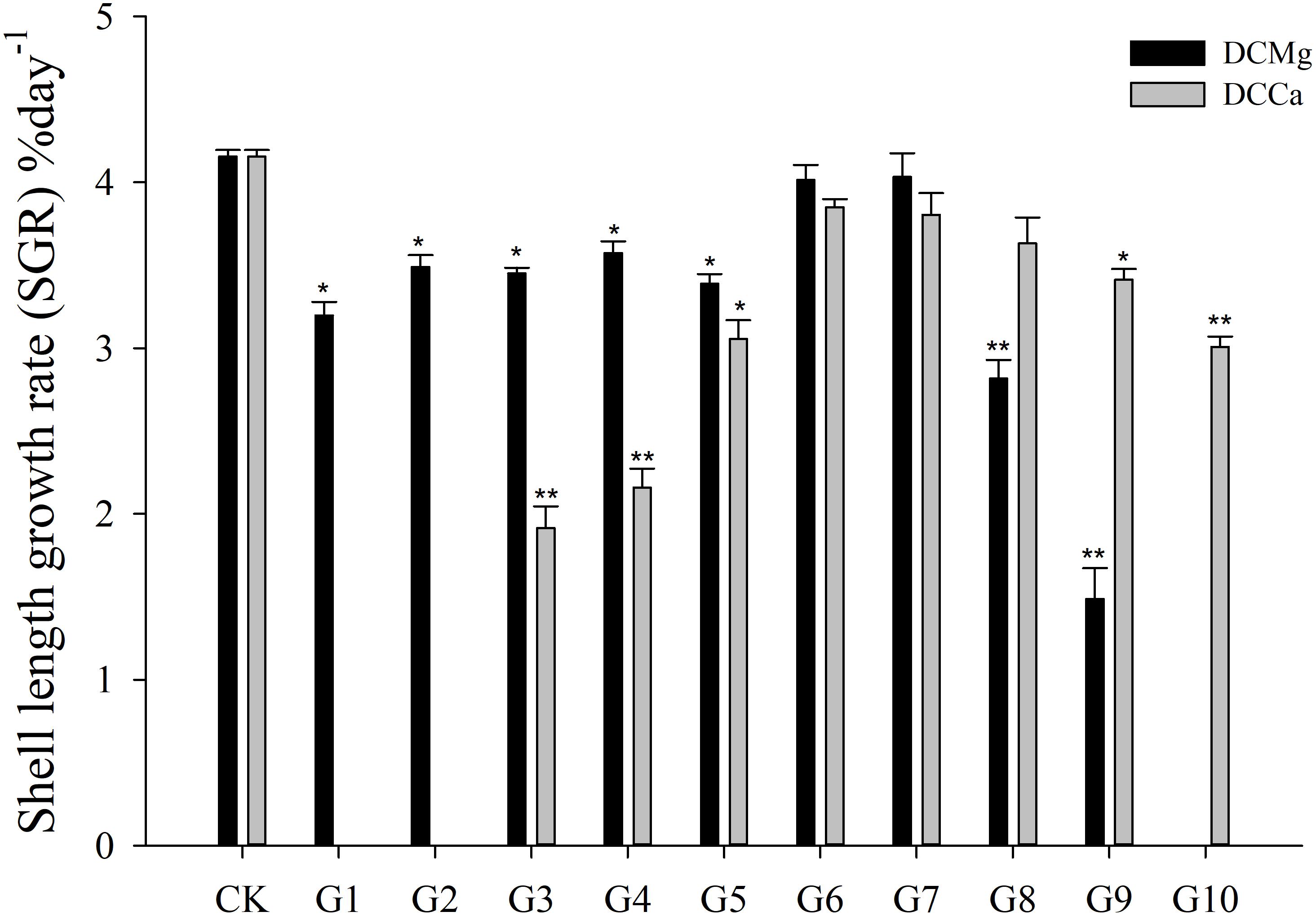
Figure 2. Daily shell length growth rate of JSCs under long-term stress with different Ca2+ and Mg2+ concentrations. Bars (mean ± SE, n = 3) with an asterisk denote a significant difference (∗P < 0.05, ∗∗P < 0.001) between the test groups (G1–G10) and CK.
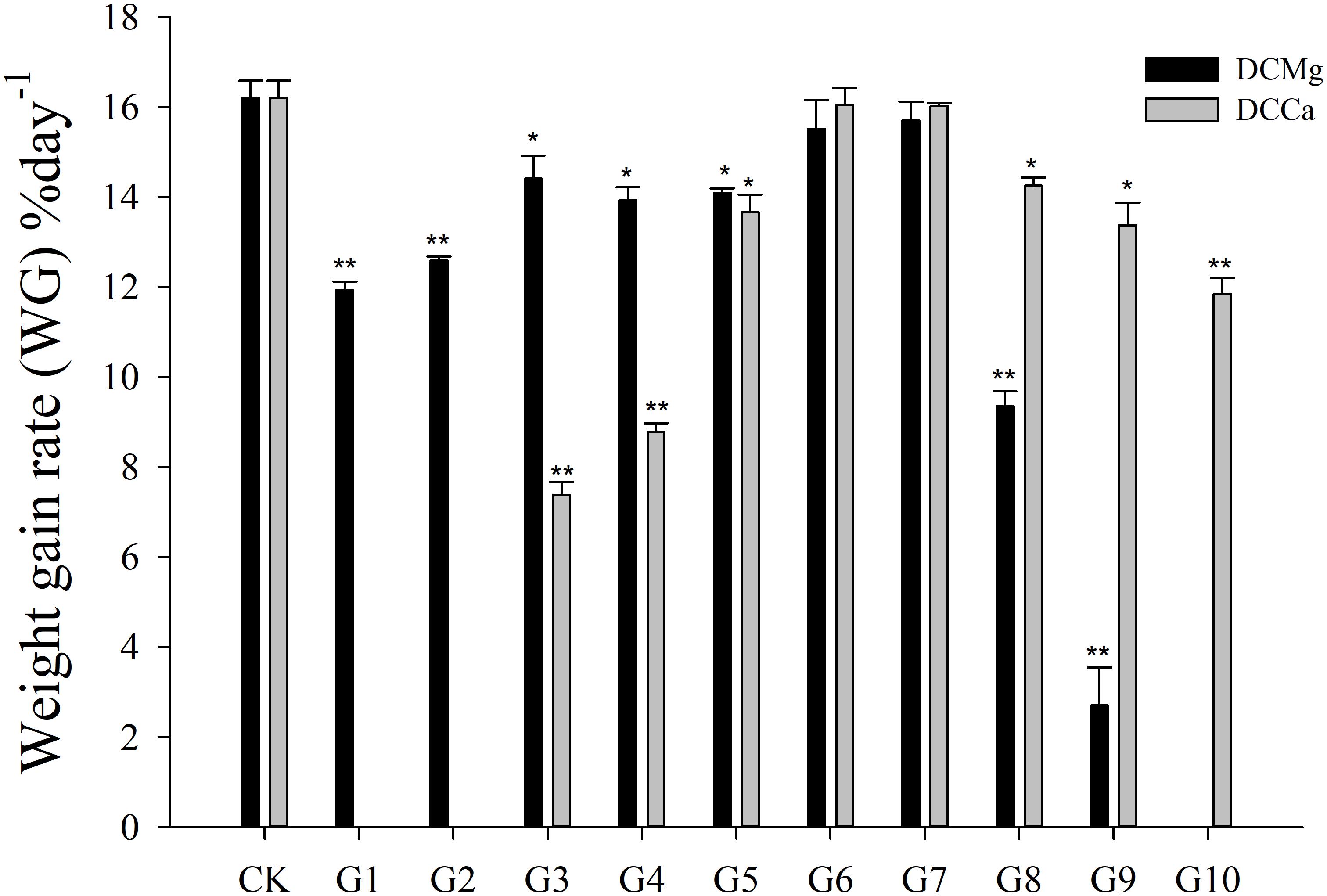
Figure 3. Daily weight gain rate of JSCs under long-term stress with different Ca2+ and Mg2+ concentrations. Bars (mean ± SE, n = 3) with an asterisk denote a significant difference (∗P < 0.05, ∗∗P < 0.001) between the test groups (G1–G10) and CK.
Enzyme Activity of JSC Under Long-Term Ca2+ or Mg2+ Stress
Na+/K+-ATPase activity was significantly decreased in JSCs stressed with low concentrations range (0–0.046 mmol⋅L-1) of Mg2+ (Figure 4). However, 14.77 mmol⋅L-1 of Mg2+ significantly increased NKA activity in JSCs (P < 0.05). Low concentrations and high concentrations of Ca2+ affected JSC NKA activity. NKA activity was increased significantly in the Ca2+ concentration of 0.19 mmol⋅L-1 (P < 0.001), 0.28 mmol⋅L-1 (P < 0.001), and 19.46 mmol⋅L-1 (P < 0.05). NKA and SOD activity patterns are totally consistent when Ca2+ varying. In contrast with NKA, SOD activity is not affected by low Mg2+ (Figure 5). AST activity (Figure 6) was significantly reduced in low concentrations (0–0.37 mmol⋅L-1) of Mg2+ (P < 0.05), but was significantly increased in the high Mg2+ concentration, 14.77 mmol⋅L-1 (P < 0.05) and 29.54 mmol⋅L-1 (P < 0.001). However, both 0.19–0.65 mmol⋅L-1 (P < 0.05) and 6.49–19.46 mmol⋅L-1 (P < 0.05) concentrations of Ca2+ significantly reduced AST activity in JSCs. AChE activity increased as the concentration of Mg2+ increased (Figure 7). AChE activity was inhibited significantly in the Mg2+ concentration of 0 mmol⋅L-1 and 0.046 mmol⋅L-1 (P < 0.05), but was increased significantly in the of 14.77 mmol⋅L-1 and 29.54 mmol⋅L-1 (P < 0.001). The same trend was observed in the effects of Ca2+ concentration on AChE activity. AChE activity was inhibited significantly in the Ca2+ concentration of 0.19 mmol⋅L-1 and 0.28 mmol⋅L-1 (P < 0.001), but was increased significantly in the 6.49 mmol⋅L-1 and 19.46 mmol⋅L-1 (P < 0.001). Only the Mg2+ – 1.85 mmol⋅L-1, Mg2+ – 3.69 mmol⋅L-1, Ca2+ – 2.32 mmol⋅L-1, and Ca2+ – 3.24 mmol⋅L-1 showed no significant inhibition of LZM activity in JSCs (Figure 8).
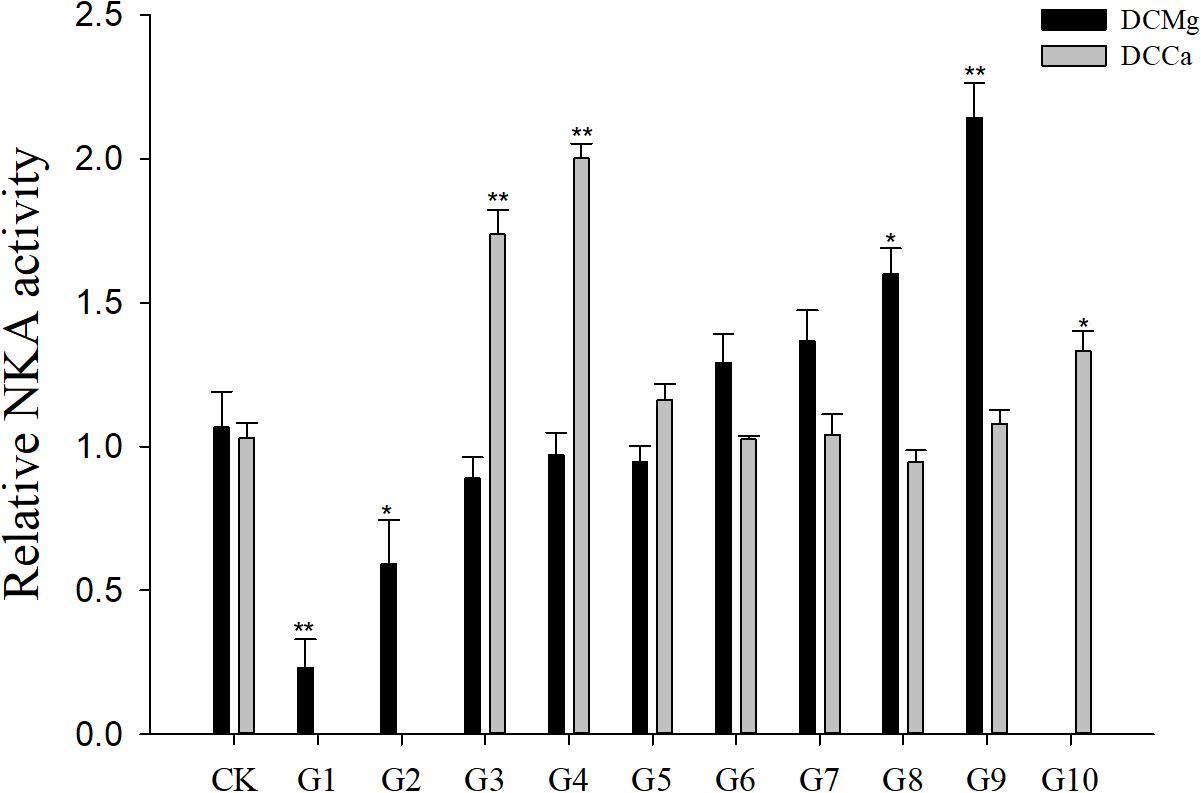
Figure 4. Relative NKA activity of JSCs under long-term stress with different Ca2+ and Mg2+ concentrations. Results show NKA activity at 30-days normalized to the 0-day. Bars (mean ± SE, n = 3) with an asterisk denote a significant difference (∗P < 0.05, ∗∗P < 0.001) between the test groups (G1–G10) and CK.
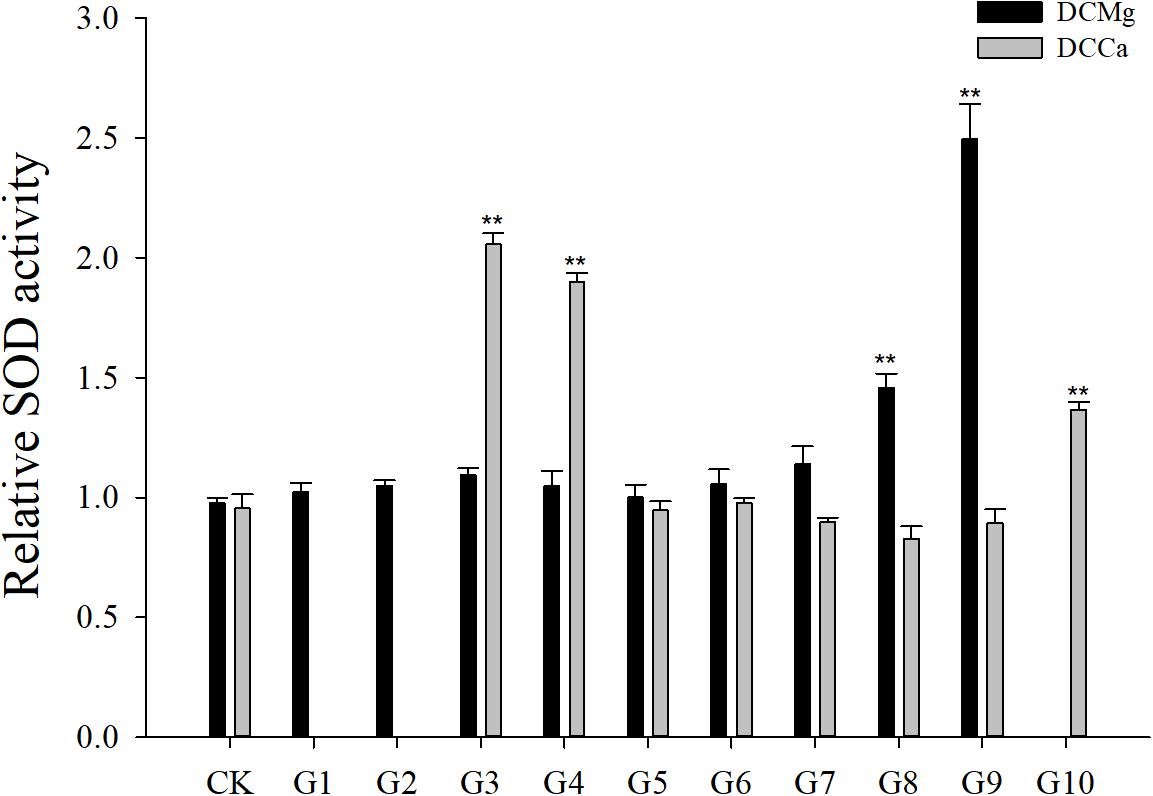
Figure 5. Relative SOD activity of JSCs under long-term stress with different Ca2+ and Mg2+ concentrations. Results show SOD activity at 30-days normalized to the 0-day. Bars (mean ± SE, n = 3) with an asterisk denote a significant difference (∗P < 0.05, ∗∗P < 0.001) between the test groups (G1–G10) and CK.
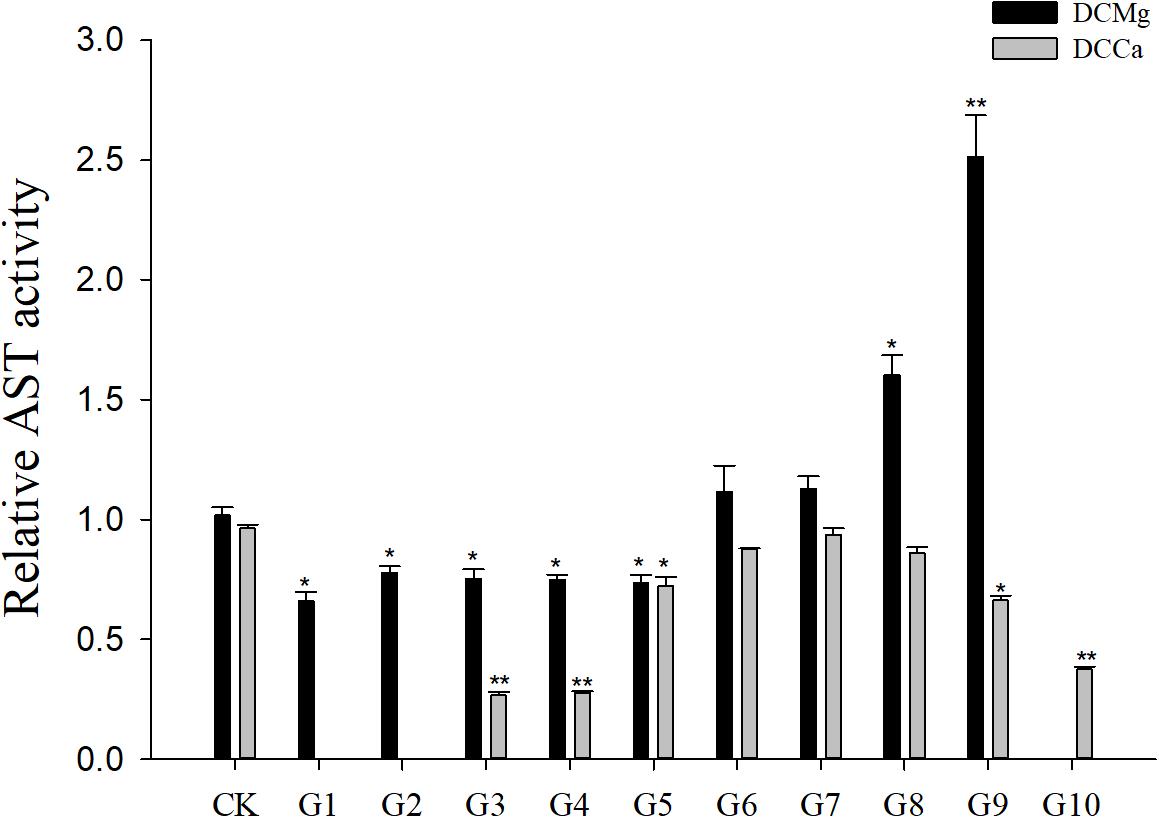
Figure 6. Relative AST activity of JSCs under long-term stress with different Ca2+ and Mg2+ concentrations. Results show AST activity at 30-days normalized to the 0-day. Bars (mean ± SE, n = 3) with an asterisk denote a significant difference (∗P < 0.05, ∗∗P < 0.001) between the test groups (G1–G10) and CK.
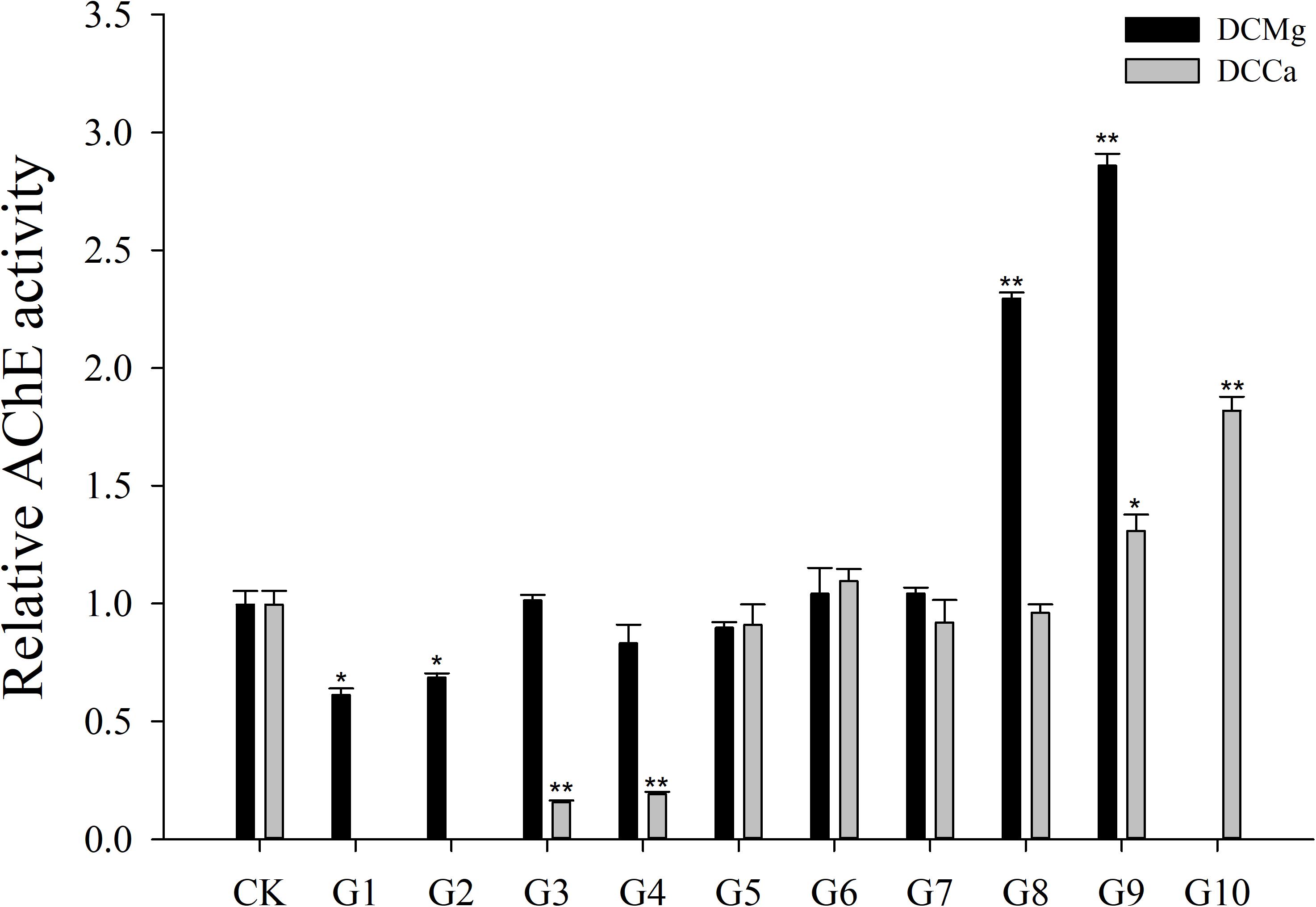
Figure 7. Relative AChE activity of JSCs under long-term stress with different Ca2+ and Mg2+ concentrations. Results show AChE activity at 30-days normalized to the 0-day. Bars (mean ± SE, n = 3) with an asterisk denote a significant difference (∗P < 0.05, ∗∗P < 0.001) between the test groups (G1–G10) and CK.
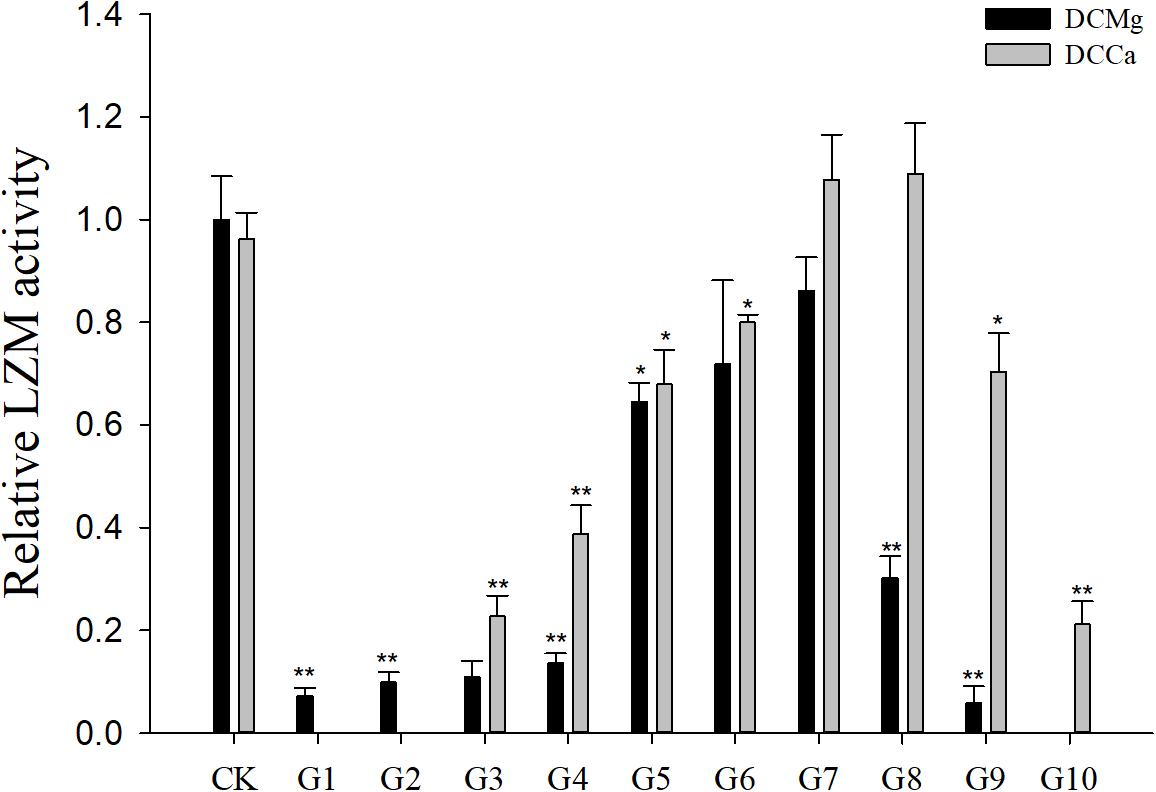
Figure 8. Relative LZM activity of JSCs under long-term stress with different Ca2+ and Mg2+ concentrations. Results show LZM activity at 30-days normalized to the 0-day. Bars (mean ± SE, n = 3) with an asterisk denote a significant difference (∗P < 0.05, ∗∗P < 0.001) between the test groups (G1–G10) and CK.
Oxygen Consumption Rate, Phagocytic Rate, and Metabolism Activity of JSC Under Acute Ca2+ or Mg2+ Stress
The oxygen consumption rate of JSCs changed under acute stress due to different concentrations of Ca2+ and Mg2+ (Figure 9). Within 48 h, the oxygen consumption rate at 0 mmol⋅L-1 of Mg2+ did not show any significant difference. However, the oxygen consumption rate in the Mg2+ concentration 73.86 mmol⋅L-1 was significantly increased at 24 h, and then was significantly decreased at 48 h. The oxygen consumption rate of the Ca2+ concentration 0 mmol⋅L-1 was significantly increased at 48 h. The oxygen consumption rate of the Ca2+ concentration 207.55 mmol⋅L-1 was significantly increased at 12 and 24 h, and then was significantly decreased at 48 h. The phagocytosis rate of all groups decreased over time (Figure 10). Among them, the Mg2+ concentration 0 mmol⋅L-1 showed a significant decreased at 48 h (P < 0.05), the Mg2+ concentration 73.86 mmol⋅L-1 showed a decrease at 24 h (P < 0.05) and 48 h (P < 0.001), and the Ca2+ concentration 0 mmol⋅L-1 and 207.55 mmol⋅L-1 began to decrease significantly at 12 h. The Mg2+ concentration 0 mmol⋅L-1 showed no significant change in metabolic activity (Figure 11). Metabolic activity was only significantly increased at 48 h in the Ca2+ concentration 0 mmol⋅L-1. However, metabolic activity of the Mg2+ concentration 73.86 mmol⋅L-1 and Ca2+ concentration 207.55 mmol⋅L-1 began to increase significantly at 12 h.
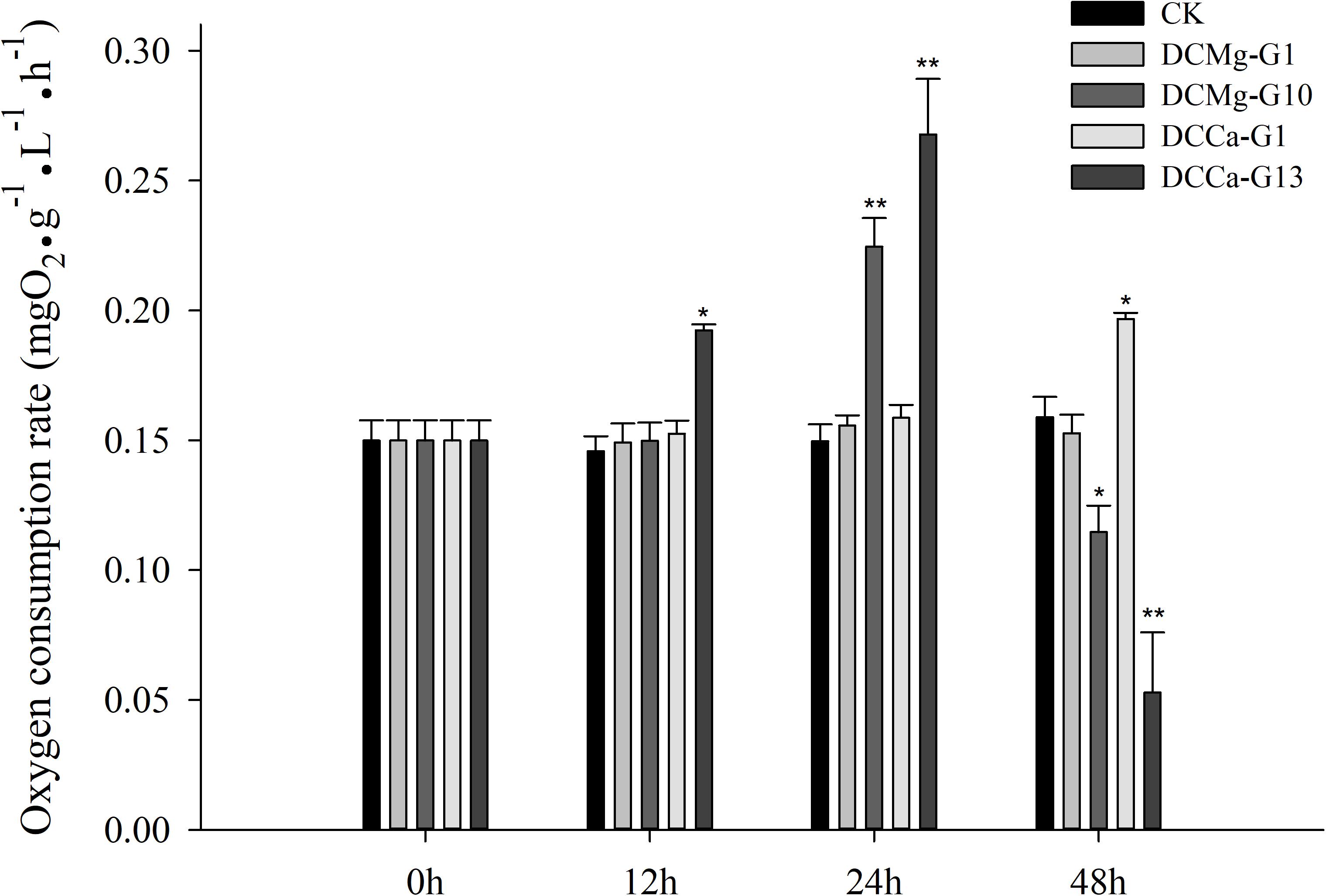
Figure 9. Acute oxygen consumption rate of JSCs under extreme Ca2+ and Mg2+ concentrations. Bars (mean ± SE, n = 3) with an asterisk denote a significant difference (∗P < 0.05, ∗∗P < 0.001) between the test groups and CK.
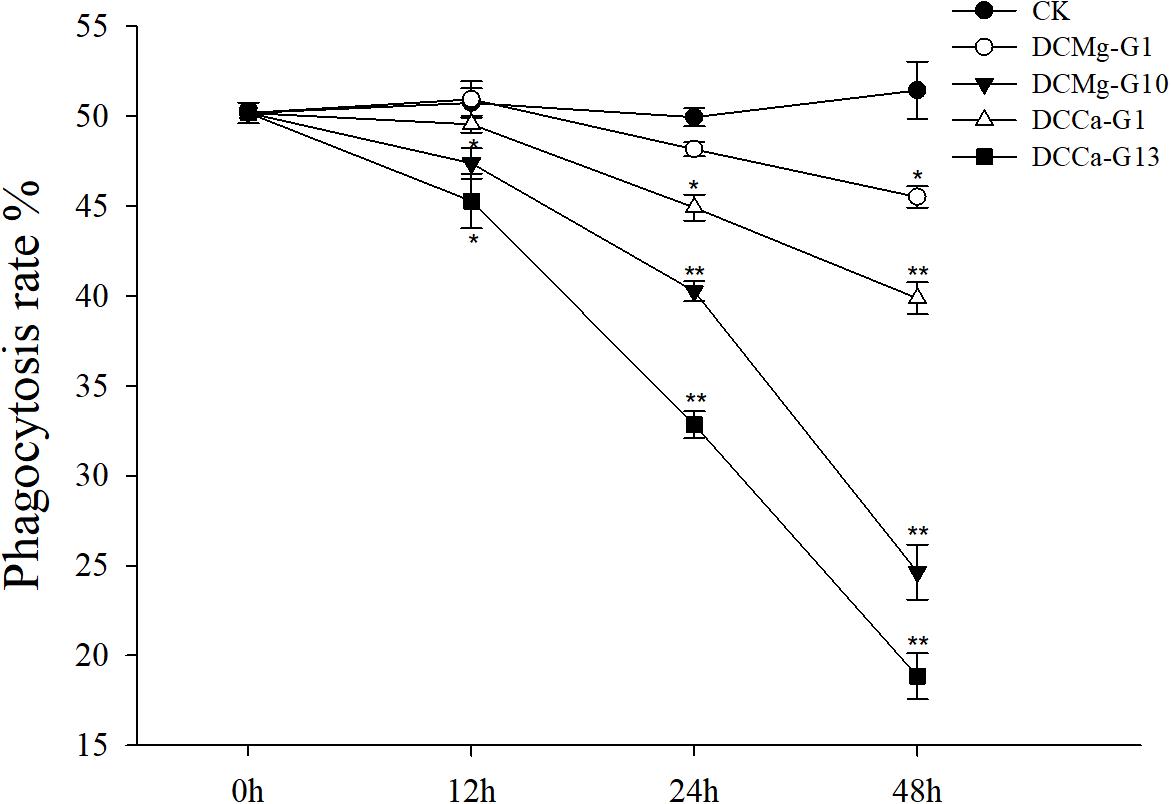
Figure 10. Acute phagocytosis rate of JSCs under extreme Ca2+ and Mg2+ concentrations. Bars (mean ± SE, n = 3) with an asterisk denote a significant difference (∗P < 0.05, ∗∗P < 0.001) between the test groups and CK.
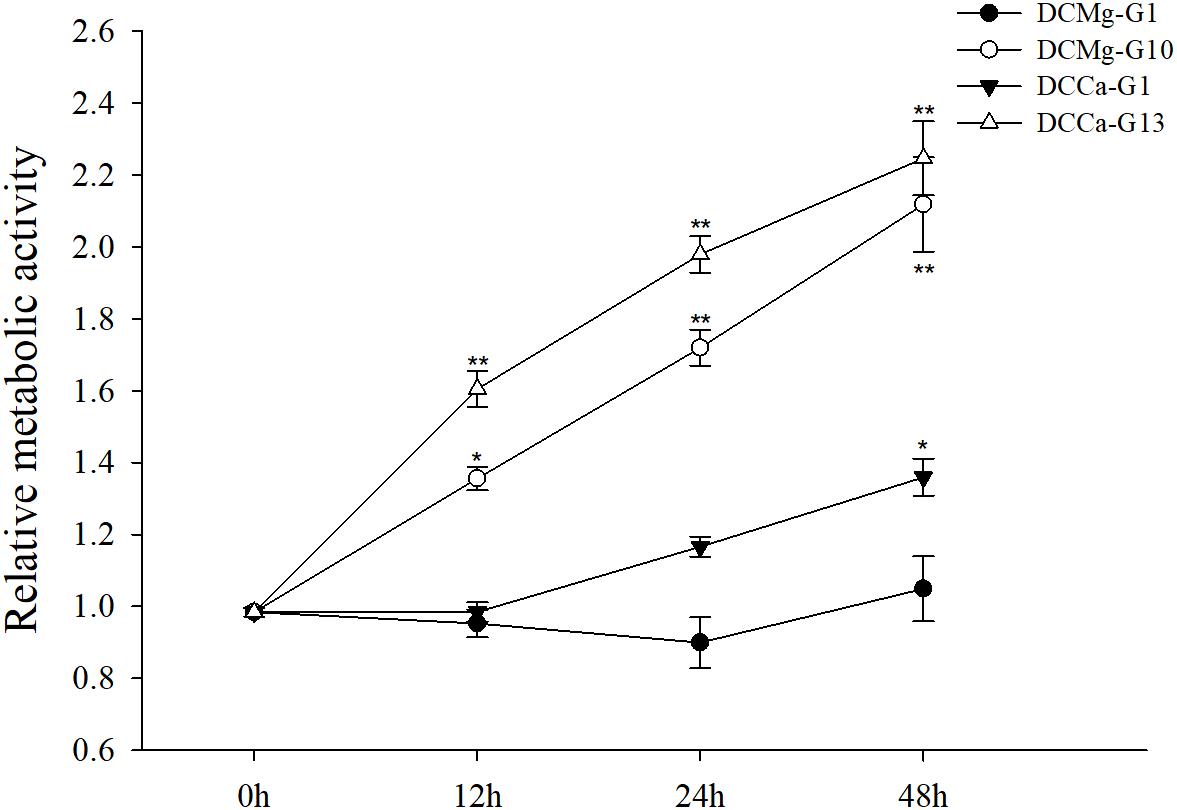
Figure 11. Acute metabolism of JSCs under extreme Ca2+ and Mg2+ concentrations. Results show metabolism activity of test groups normalized to the 0 h group. Bars (mean ± SE, n = 3) with an asterisk denote a significant difference (∗P < 0.05, ∗∗P < 0.001) between the test groups and CK.
Discussion
Changes of Ca2+ and Mg2+ content in water not only affect the survival and growth of bivalves, but also affect their physiological responses. ISW in northwest China, especially the Gansu area (a target area for the transplantation of S. constricta), is characterized by broad variation in pH, carbonate alkalinity, and Ca2+ (0.775 mmol⋅L-1–6.825 mmol⋅L-1) and Mg2+ (3.07 mmol⋅L-1–33.69 mmol⋅L-1) content. Studying the ion tolerance range of S. constricta is the first and crucial step toward transplanting S. constricta cultures to ISW.
There is a significant difference in the tolerance of Ca2+ and Mg2+ between different invertebrate species. Zhou et al. (2007) reported that the optimal concentrations of Ca2+ and Mg2+ for the survival of L. vannamei larvae were 0.76–8.78 mmol⋅L-1 and 0.36–35.51 mmol⋅L-1, respectively. Roy et al. (2007) found that Mg2+ concentrations are very important for shrimp. The concentration of Mg2+ in inland saline-alkali water is at least 25% higher than that of seawater at the same salinity. Sprung (1987) reported that if environmental Ca2+ concentrations are less than 0.3 mmol⋅L-1, the veliger larvae of the zebra mussel (Dreissena polymorpha) cannot survive. Furthermore, the New Zealand mud snail (Potamopyrgus antipodarum) is reported to have a minimum Ca2+ concentration tolerance of 0.125 mmol⋅L-1 (Herbst et al., 2008). Lin et al. (2012) studied the effects of acute Ca2+ and Mg2+ concentrations on the survival of C. sinensis to assess the possibility of cultivating C. sinensis as a cultured species in ISW. It was found that when Ca2+ concentrations were between 0.11 mmol⋅L-1 and 115 mmol⋅L-1, and Mg2+ concentrations were between 0 mmol⋅L-1 and 126.67 mmol⋅L-1, the C. sinensis survival rates were 66 and 91.5%, respectively. It was determined that Mg2+ concentration may not be a primary limiting factor for transplanting C. sinensis in ISW. Our findings are similar to those of Lin et al. (2012) namely that JSC can maintain a high survival rate for at least a month when under Mg2+ (0 mmol L-1) stress. However, the tolerance of JSC to high concentrations of Mg2+ is far less than that of C. sinensis. It is worth noting that the maximum tolerated concentration of JSC for Mg2+ is also slightly lower than the maximum measured value of the target-ISW. This suggests that perhaps Mg2+ be one of the limiting factors to the S. constricta transplantation. Thus special attention have to be paid to whether the concentration of Mg2+ ions too high when practicing transplantation. Mg2+-free medium have little effect on the survival rates of JSC and C. sinensis; this may be caused by the presence of dietary Mg2+ ions, or may indicate that the effect of free Mg2+ on survival is a long-term process. Because Dietz et al. (1994) have reported that D. polymorpha depleted of Mg2+ did not survive beyond 51 days. JSC can survival in a wide range of concentrations of Ca2+ (0.19–19.46 mmol⋅L-1), suggesting that transplantation of JSC to target-ISW may without effect of Ca2+, and could be performed in a broad range of Ca2+ concentrations.
The shell of bivalves serves as the first barrier to resist external threats, and the main component of the shell is calcium carbonate. The growth of shells is directly related to the growth of the bivalve. Since Ca2+ is the main cation involved in the shell formation of bivalves, it was chosen in previous growth models (Hincks and Mackie, 1997). Most of the environmental calcium (approximately 80%) that is actively taken up from the water is deposited in the shell (Van Der Borght and Van Puymbroeck, 1966). However, these reactions are reversible, and under condition of low Ca2+ concentrations, calcium is removed from the shell (Van Der Borght and Van Puymbroeck, 1966). Accordingly, in this test we observed that JSCs died from calcium carbonate shell loss when Ca2+ was less than 0.19 mmol⋅L-1. Such a situation may stem from the results of Ca2+ regulation. On the one hand, it is the release of Ca2+ in the shell caused by the efflux of free Ca2+ from the hemolymph and shell-mantle fluid. On the other hand, the efflux of Ca2+ also causes an increase in free amino acids and acidic metabolites in the body. These acidic end products are buffered by the mobilization of Ca2+ from the shell (Akberali et al., 1977). In addition, Wu et al. (2003) studied the effect of Ca2+ on the activity of digestive enzymes in S. constricta. It was found that an imbalance of Ca2+ concentration significantly decreased the activities of amylase and protease, thereby inhibiting growth. The higher Ca2+ concentration range of target-ISW 6.49–6.825 mmol⋅L-1 and 3.24–6.825 mmol⋅L-1 may significantly inhibit the shell length and body weight growth of JSC, respectively. This suggest that transplanting S. constricta in the target-ISW may not have growth inhibition caused by Ca2+ deficiency, and there may be only a physiological response by excessive Ca2+. Other hand suggest that the effect of Ca2+ on the growth of JSC may be more than one aspect, which weight is more likely to be affected than shell.
Mg2+ is the most abundant divalent ion within living cells. It is a cofactor in many enzymes, and its free ionic concentration regulates metabolic and shell formation processes (Bentov and Erez, 2006; Roy et al., 2010; Marin et al., 2012; Suzuki and Nagasawa, 2013; Wilbur and Yonge, 2013; González-Vera and Brown, 2017). However, Wu et al. (2003) found that the concentration of Mg2+ in the water did not affect the digestive enzyme activity of S. constricta, indicating that the effects of Mg2+ on JSC growth likely involves the osmotic and ionic regulation caused by Mg2+ imbalance and its role as the activity center of metabolic and mineral enzymes. From the Mg2+ concentration distribution of the target-ISW, the higher Mg2+ concentration range 14.77–33.69 mmol⋅L-1 will significantly inhibit the shell length and body weight growth of JSC. This suggest that transplanting S. constricta in the target-ISW will not have growth inhibition caused by Mg2+ deficiency, and there may be only a response caused by excessive Mg2+.
Most evidences in bivalves is based on observations that on simulative conditions, oxygen consumption rapidly rises to levels above normal (Moon and Pritchard, 1970; Akberali et al., 1977; Almada-Villela, 1984). Both cell volume regulation and taurine efflux are inhibited in Noetia ponderosa erythrocytes and Glycera dibranchiata coelomocytes exposed to Ca2+-free hypoosmotic artificial seawater, and both are potentiated in high Ca2+ hypoosmotic artificial seawater (Smith and Pierce, 1987). Divalent cations also stabilize the cell membrane. The increased membrane permeability to taurine caused by Ca2+-free isosmotic media may be due to a lack of divalent cations that bind to and stabilize membranes (Swinehart et al., 1980). Not difficult to imagine the volume regulation of JSC haemocytes is extremely active, it may be the main reason sustained increase in the metabolism. Meanwhile, we found that the oxygen consumption increases within 24 h but it decreases sharply in 48 h. This suggest that high Ca2+ and Mg2+ stresses affects ventilatory activity causing internal hypoxia at 48 h. This may be the main reason for the rapid death of JSCs. On the contrary, Lee et al. (2005) noted that in Mg2+-free artificial seawater, the respiration rate of hard clams is decreased. No significant changes in oxygen consumption were observed in the DCMg-G1 group (Mg2+-free group), and it is likely that this inhibitory effect will take longer in JSCs.
When osmotic concentration and the ionic composition of the extracellular fluid be changed, the cells release cytoplasmic ions and organic osmolytes into the extracellular fluid to eliminate the osmotic gradient across the plasma membrane (Deaton, 2008; Larsen et al., 2014). Changes in environmental Ca2+ and Mg2+ have important regulation for both inorganic ion and organic osmotic regulation. In research of G. dibranchiata, it was found that the lack of Ca2+ ions in the environment did not lead to the efflux of Na+ and Cl-, but it caused the efflux of K+ (Costa and Pierce, 1983). Comparing our results, it suggesting that the effect of extracellular fluid Ca2+ concentration on NKA activity may be directly related to the flux of K+. We believe that the ionic regulation caused by Ca2+ may be much more than that. A decrease in the concentration of Mg2+ ions in water tends to inactivate NKA in shrimp (Roy et al., 2010). Similar results were also seen in our study, but Watts and Pierce (1978) reported that the ATPase in Modiolus demissus ventricles requires a minimum of 5 mM Mg2+ in body to maintain constant activity. These suggest that S. constricta may existence very high regulation ability. In addition, when cultured in Mg2+-free water, the NKA activity in JSC is not completely lost, as Mg2+ may be obtained through dietary Mg2+ ions in food (algae). Mollusc farming does not utilize compound feed directly, like shrimp farming, so the impact of dietary Mg2+ is difficult to eliminate. Of course, the target-ISW does not have the Mg2+-free type of water, and the target-ISW does not significantly inhibit the NKA activity when the Mg2+ content is the lowest. But the higher Mg2+ concentration range 14.77–29.54 mmol⋅L-1 may significantly increase the NKA activity of JSC. In addition, the Ca2+ concentration range of target-ISW may not significant impact on NKA activity of JSC. This suggest that when transplanting S. constricta in the target-ISW, Mg2+ may be one of the main factors to induce individuals to produce intense osmotic and ionic regulation.
Mg2+ operates synergistically to facilitate glutamate dehydrogenase activity and interactions between this glutamate dehydrogenase and aminotransferase (Fahien et al., 1985). In bivalve, inorganic ions are the first efflux components that respond to ambient medium cation ions (Na+, K+, Ca2+, and Mg2+) balance changes (Akberali et al., 1977; Shumway, 1977; Smith and Pierce, 1987). The efflux of inorganic ions induces the osmotic regulation from the organic components (free amino acids). The regulation of divalent cations in JSC may affect the concentration of free amino acids and may be directly related to changes in AST activity. Decreases and increases in Mg2+ concentration directly affect AST activity, and these results were recapitulated in our study. However, decreases and increases of Ca2+ concentration inhibit AST activity in JSC. So from the Ca2+ and Mg2+ concentration distribution of the target-ISW, we can imagine that both the higher Ca2+ (6.49–6.825 mmol⋅L-1) and Mg2+ (14.77–33.69 mmol⋅L-1) concentrations range may significantly affect AST activity. This indicating that changes of Ca2+ concentration in water not only affect digestive enzyme activity but also affect enzyme activity related to protein metabolism, thereby inhibiting growth (Wu et al., 2003).
The imbalance between Ca2+ and Mg2+ in water leads to increased oxygen consumption in aquatic animals, resulting in the production of more oxygen free radicals (Lin and Kao, 2000; Culotta, 2001). The increase in oxygen free radicals induces an increase in SOD activity to scavenge these radicals. In the present study, SOD activity is only augmented at moderately low calcium concentrations, which are coincident with high NKA activity and partial restoring of shell growth. SOD is also increased at the highest concentrations of both ions, which are very stressful conditions. But in target-ISW may be only the higher Mg2+ concentration range (14.77–33.69 mmol⋅L-1) may significantly increase the SOD activity of JSC. This suggest that Mg2+ may cause excessive metabolism and produce a large amount of oxygen free radicals when transplanting S. constricta in the target-ISW.
The AChE is a hydrolase for the signal termination at cholinergic synapses by rapid hydrolysis of the neurotransmitter acetylcholine (Quinn, 1987). AChE paly as a important role in neurotransmission process also in fish and invertebrates (Fulton and Key, 2001). The inhibition of AChE activity has been used widely as a biomarker of exposure to organotoxic substance and heavy metal ions in mollusk (Rickwood and Galloway, 2004; Devin et al., 2017). In addition, Pfeifer et al. (2005) reported that the activity of AChE in Mytilus sp. is sensitive to environmental salinity. Although the effect of the concentration of Ca2+ and Mg2+ on AChE activity in mollusc has not been clearly reported, in fish, Tomlinson et al. (1981) reported that AChE activity reached maximum saturation when both Ca2+ and Mg2+ concentrations were on the order of magnitude of 10 mmol, and the activity level were maintained at more higher Ca2+ and Mg2+ concentrations. The same situation be found in our study, AChE activity was continuously increased with increasing concentrations of Ca2+ and Mg2+ in water. Although there are some differences in the physiological functions of fish and bivalve, the physiological roles and functional mechanisms of AChE (the perspective of heavy metal ion binding) are relatively conservative. This indicating that the molecular mechanism of bivalve and fish AChE are similar, and the difference between the them may occur in the magnitude of the cation strength affecting the enzyme activity. In our study, indicating that the neurotransmission process of JSC be significant inhibition when the concentration of Ca2+ higher than 6.49 mmol L-1 and concentration of Mg2+ higher than 14.77 mmol L-1. Then the corresponding physiological processes of neuromodulation are inhibited. Obviously, this effect may be existed in both the higher Ca2+ (6.49–6.825 mmol⋅L-1) and Mg2+ concentration range (14.77–33.69 mmol⋅L-1) of target-ISW.
Lysozyme is a typical immunoenzyme that specifically lyses bacteria (Bachali et al., 2002; Xue et al., 2010). Usually LZM is divided into two categories, based on whether it can be combined with Ca2+ (Jielian et al., 2017). In mollusc, there is a class of LZM that binds to Ca2+ and interacts with calcium carbonate to participate in the shell formation process (Jimenez-Lopez et al., 2003). However, in mollusc is reported that LZM activity has a low tolerance to Ca2+ and Mg2+ concentrations (Nilsen et al., 1999; Olsen et al., 2003). In addition, the results of SOD and phagocytosis in this study also reflect the immune response of JSCs. So, we propose that the effects of Ca2+ and Mg2+ on the immune capacity of JSC are comprehensive. We suggest that (1) Ca2+ concentration (lower than 0.28 mmol L-1, higher than 19.46 mmol L-1) and Mg2+ concentration (higher than 14.77 mmol L-1) affect metabolic immune enzymes; (2) function of haemocyte phagocytosis will be inhibited when the concentration of Ca2+ and Mg2+ in hemolymph sharply rise or fall in short period of time. Because adhesion and aggregation function be limited (Chen and Bayne, 1995); (3) Ca2+ concentration (lower than 0.93 mmol L-1, higher than 6.49 mmol L-1) and Mg2+ concentration (lower than 0.37 mmol L-1, higher than 14.77 mmol L-1) affect antibacterial enzyme activity. Therefore, this means that when the S. constricta is transplanted to the target-ISW, both the higher Ca2+ (6.49–6.825 mmol⋅L-1) and Mg2+ (14.77–33.69 mmol⋅L-1) concentration range may significantly enhances the metabolic immunity, but the cellular immunity (haemocyte) and bacterial immunity are weakened.
The Ca2+/Mg2+ ratio is often reported in shrimp and has important effects on survival, growth and physiology (Pan et al., 2006; González-Vera and Brown, 2017). The Ca2+/Mg2+ ratio not only has strong species specificity, but also has been widely researched in shrimp species. Tavabe et al. (2013) found that a Ca2+/Mg2+ ratio of 0.8 was optimal for larval development, while Latif (1992) found that a 1:1 Ca2+/Mg2+ ratio was desirable for juveniles of Macrobrachium rosenbergii. This suggests that the Ca2+/Mg2+ ratio is an important indicator for shrimp, but further study is needed to elucidate its importance in other aquatic species. The Ca2+/Mg2+ ratio in mollusc has not been reported on. We do not have definitive evidence to obtain the significant correlation between Ca2+/Mg2+ ratio and the survival, growth, and physiology of JSC, but this does not mean that Ca2+/Mg2+ ratio has no effect, so this requires future validation with independent test.
Over the past 250 years, atmospheric carbon dioxide (CO2) levels increased by nearly 40% (Solomon et al., 2007). Ocean CO2 uptake, causes pH reductions and alterations in fundamental chemical balances that together are commonly referred to as ocean acidification (Millero et al., 2009). Orr et al. (2005) predicted that ocean acidification will cause a pH drop of 0.3–0.4 for the 21st century, is equivalent to approximately a 150% increase in H+ and 50% decrease in CO32- concentrations. Marine carbonates are mainly in the form of CaCO3 and MgCO3, which means that the concentration of Ca2+ and Mg2+ in the 21st century will increase within 50% than it is now. Comparing our findings, we can see that such an increase may not have a negative impact on the survival, growth and physiology of JSC. However, the negative impact of ocean acidification on the survival and growth of corals and molluscs is a proven fact (Hoegh-Guldberg et al., 2007; Fabry et al., 2008). Ocean acidification is a complex environmental change process involving not only changes in the concentration of metal cations, but also pH, pCO2, CO32-, temperature and marine food chains (Fabry et al., 2008). Therefore, we believe that other factors or comprehensive factors other than Ca2+ and Mg2+ may eventually lead to the negative impact of ocean acidification on the survival and growth of molluscs.
Conclusion
Stress caused by changes in the concentration of Ca2+ and Mg2+ in the environment may cause efflux and reflux of Ca2+ and Mg2+ in JSC. Metabolism and oxygen consumption are rapidly increased but haemocyte phagocytosis are decreased in short time. At this time, a large number of JSCs died when Ca2+ and Mg2+ exceed physiological limits. In the long-term stress, the imbalance both Ca2+ and Mg2+ may cause a comprehensive physiological response including ionic regulation, metabolic levels, neurotransmission process, and immunity. Then ultimately affect growth and even survival. When using such a “standard ruler” to measure the effect of Ca2+ and Mg2+ concentrations in the target-ISW on S. constricta, we found that only high concentrations of Ca2+ (3.24–6.825 mmol⋅L-1) and Mg2+ (14.77–33.69 mmol⋅L-1) had significantly impact on transplantation practice. In physiology, such effects include accelerated ionic regulation, accelerated metabolism, increased oxygen free radical production, increased metabolic immunity, decreased bacterial and cellular immunity, and inhibit neurotransmission process. The concentrations of Ca2+ and Mg2+ in the target-ISW does not cause all S. constricta to die in the short term, but it inhibits growth and causes a part of individual death during long-term aquaculture. Those implies that we have to choose the right concentration of Ca2+ and Mg2+ or to improve the water quality in target-ISW. Fortunately, the Ca2+ and Mg2+ content of most area target-ISWs is suitable for long-term transplantation of S. constricta. Our study also suggests that the increase in Ca2+ and Mg2+ ion concentrations caused by ocean acidification will not affect the survival, growth and physiology of S. constricta.
Data Availability
All datasets generated for this study are included in the manuscript and/or the supplementary files.
Ethics Statement
In this study, the animals were artificially propagated as juvenile clams.
Author Contributions
MP was responsible for experimental design, test operations, data processing, and manuscript writing. ZL was responsible for data processing and manuscript modification. XL was responsible for experimental design. BY was responsible for test operations. TL was responsible for experimental data collection. JL, DN, and ZD were responsible for experimental program guidance.
Funding
This study was supported by grants from the National Natural Science Foundation of China (Grant No. 31101897), the “863” Hi-tech Research and Development Program of China (Grant No. 2012AA10A400-3), and the Shanghai Universities Knowledge Service Platform (Grant No. ZF1206).
Conflict of Interest Statement
The authors declare that the research was conducted in the absence of any commercial or financial relationships that could be construed as a potential conflict of interest.
References
Akberali, H. B., Marriott, K. R. M., and Trueman, E. R. (1977). Calcium utilisation during anaerobiosis induced by osmotic shock in a bivalve mollusc. Nature 266, 852–853. doi: 10.1038/266852a0
Almada-Villela, P. C. (1984). The effects of reduced salinity on the shell growth of small Mytilus edulis. J. Mar. Biol. Assoc. U.K. 64, 171–182. doi: 10.1017/s0025315400059713
Bachali, S., Jager, M., Hassanin, A., Schoentgen, F., Jolles, P., Fiala-Medioni, A., et al. (2002). Phylogenetic analysis of invertebrate lysozymes and the evolution of lysozyme function. J. Mol. Evol. 54, 652–664. doi: 10.1007/s00239-001-0061-6
Bentov, S., and Erez, J. (2006). Impact of biomineralization processes on the Mg content of foraminiferal shells: a biological perspective. Geochem. Geophys. Geosyst. 7, 1–11. doi: 10.1007/978-94-009-7944-4_1
Chen, J., and Bayne, C. J. (1995). Bivalve mollusc haemocyte behaviors: characterization of haemocyte aggregation and adhesion and their inhibition in the California mussel (Mytilus californianus). Biol. Bull. 188, 255–266. doi: 10.2307/1542303
Costa, C. J., and Pierce, S. K. (1983). Volume regulation in the red coelomocytes of Glycera dibranchiata: an interaction of amino acid and K+ effluxes. J. Comp. Physiol. 151, 133–144. doi: 10.1007/bf00689911
Culotta, V. C. (2001). Superoxide dismutase, oxidative stress, and cell metabolism, current topics in cellular regulation. Elsevier 36, 117–132. doi: 10.1016/s0070-2137(01)80005-4
Deaton, L. E. (1992). Osmoregulation and epithelial permeability in two euryhaline bivalve molluscs: Mya arenaria and Geukensia demissa. J. Exp. Mar. Biol Ecol. 158, 167–177. doi: 10.1016/0022-0981(92)90224-x
Deaton, L. E. (2008). “Osmotic and ionic regulation in molluscs,” in Osmotic and Ionic Regulation: Cells and Animals, ed. D. H. Evans (New York, NY: CRC Press), 107–133. doi: 10.1201/9780849380525.ch4
Devin, S., Buffet, P. E., Châtel, A., Perrein-Ettajani, H., Valsami-Jones, E., and Mouneyrac, C. (2017). The integrated biomarker response: a suitable tool to evaluate toxicity of metal-based nanoparticles. Nanotoxicology 11, 1–6. doi: 10.1080/17435390.2016.1269374
Dietz, T. H., Lessard, D., Silverman, H., and Lynn, J. W. (1994). Osmoregulation in Dreissena polymorpha: the importance of Na, Cl, K, and particularly Mg. Biol. Bull. 187, 76–83. doi: 10.2307/1542167
Dinh, H. Q., and Fotedar, R. (2016). Early development of the blue mussel Mytilus edulis (Linnaeus, 1758) cultured in potassium-fortified inland saline water. Aquaculture 452, 373–379. doi: 10.1016/j.aquaculture.2015.11.025
Doupé, R., Lymbery, A., and Starcevich, M. (2003). Rethinking the land: the development of inland saline aquaculture in Western Australia. Int. J. Agric. Sustain. 1, 30–37. doi: 10.3763/ijas.2003.0104
Duchen, M. (2000). Mitochondria and Ca2+ in cell physiology and pathophysiology. Cell Calcium. 28, 339–348. doi: 10.1054/ceca.2000.0170
Fabry, V. J., Seibel, B. A., Feely, R. A., and Orr, J. C. (2008). Impacts of ocean acidification on marine fauna and ecosystem processes. ICES. J. Mar. Sci. 65, 414–432. doi: 10.1093/icesjms/fsn048
Fahien, L., Kmiotek, E., Woldegiorgis, G., Evenson, M., Shrago, E., and Marshall, M. (1985). Regulation of aminotransferase-glutamate dehydrogenase interactions by carbamyl phosphate synthase-I, Mg2+ plus leucine versus citrate and malate. J. Biol. Chem. 260, 6069–6079.
Fulton, M. H., and Key, P. B. (2001). Acetylcholinesterase inhibition in estuarine fish and invertebrates as an indicator of organophosphorus insecticide exposure and effects. Environ. Toxicol. Chem. 20, 37–45. doi: 10.1002/etc.5620200104
Gainey, L. F. (1978). The response of the corbiculidae (Mollusca: Bivalvia) to osmotic stress: the organismal response. Physiol. Zool. 51, 68–78. doi: 10.1086/physzool.51.1.30158666
González-Vera, C., and Brown, J. H. (2017). Effects of alkalinity and total hardness on growth and survival of postlarvae freshwater prawns, Macrobrachium rosenbergii (De Man 1879). Aquaculture 473, 521–527. doi: 10.1016/j.aquaculture.2017.03.016
Hadfield, C., Clayton, L., Cohrs, D., and Murphy, D. (2012). Acute morbidity and mortality in invertebrates and fish following exposure to potassium-deficient saltwater. J. Fish. Dis. 35, 549–553. doi: 10.1111/j.1365-2761.2012.01379.x
Harvey, H. W. (1957). The Chemistry and Fertility of Sea Waters. Cambridge: Cambridge University press, 112–113.
He, W., Yu, T., and Cai, Y. (2010). Analysis of control and use of alkaline soil. J. Anshan. Univ. Sci. Techn. 29, 158–160.
Herbst, D. B., Bogan, M. T., and Lusardi, R. A. (2008). Low specific conductivity limits growth and survival of the New Zealand mud snail from the Upper Owens River, California. West. N. Am. Nat. 68, 324–334.
Hiele, T. V. D., Rijstenbil, J. W., Creemers, J., and Heringa, J. (2014). Composition, treatment and use of saline groundwater for aquaculture in the Netherlands saline groundwater composition and chemistry. World. Aquac. 45, 23–27.
Hincks, S. S., and Mackie, G. L. (1997). Effects of pH, calcium, alkalinity, hardness, and chlorophyll on the survival, growth, and reproductive success of zebra mussel (Dreissena polymorpha) in Ontario lakes. Can. J. Fish. Aquat. Sci. 54, 2049–2057. doi: 10.1139/cjfas-54-9-2049
Hoegh-Guldberg, O., Mumby, P. J., Hooten, A. J., Steneck, R. S., Greenfield, P., Gomez, E., et al. (2007). Coral reefs under rapid climate change and ocean acidification. Science 318, 1737–1742.
Ingram, B. A., Mckinnon, L. J., and Gooley, G. J. (2015). Growth and survival of selected aquatic animals in two saline groundwater evaporation basins: an Australian case study. Aquac. Res. 33, 425–436. doi: 10.1046/j.1365-2109.2002.00691.x
Jielian, W., Baoqing, H., Chungen, W., and Peipei, Y. (2017). Characterization and roles of lysozyme in molluscs. Invertebrate Surviv. J. 14, 432–442.
Jimenez-Lopez, C., Rodriguez-Navarro, A., Dominguez-Vera, J. M., and Garcia-Ruiz, J. M. (2003). Influence of lysozyme on the precipitation of calcium carbonate: a kinetic and morphologic study. Geochim. Cosmochim. Acta. 67, 1667–1676. doi: 10.1016/s0016-7037(02)01275-9
Larsen, E. H., Deaton, L. E., Onken, H., O’Donnell, M., Grosell, M., William, H., et al. (2014). Osmoregulation and excretion. Compr. Physiol. 4, 405–573. doi: 10.1002/cphy.c130004
Latif, M. A. (1992). A Study of the Effects of Alkalinity and Total Hardness on Post-Larvae and Juveniles of the Giant Freshwater Prawn Macrobrachium Rosenbergii (De Man). Ph.D. thesis, University of Stirling, Scotland.
Lee, A., Lee, M., Chen, S., and Chin, T. (2005). Temperature, pH, Mg2+ and aerial exposure time affect the oxygen consumption of hard clam (Meretrix Iusoria). J. Fish. Soci. Taiwan. 32, 301–309.
Lee, C. L. (1997). “Design and operation of a land-based closed recirculating hatchery system for the topshell, Trochus niloticus, using treated bore water,” in Trochus : Status, Hatchery Practice and Nutrition, eds B. Smith and C. Barlow (Neil Balnaves, AC: Northern Territory University), 6–7.
Lin, B., and Wu, T. (1990). Temperature and salinity in relating to the survinal, growth and development of the larvae and spat of Sinonovacula constricta. J. Fish. Chin. 14, 171–178.
Lin, C. C., and Kao, C. H. (2000). Effect of NaCl stress on H2O2 metabolism in rice leaves. Plant Growth Regul. 30, 151–155.
Lin, T., Lai, Q., Lu, J., Yao, Z., Li, Z., Wang, H., et al. (2012). Toxic effects of several saline-alkali factors on Cyclina sinensis. Marin. Fish. 34, 183–188.
Lv, X., Xu, H., Li, L., and Zhao, Y. (2012). Agricultural sustainable utilization and evaluation of saline-alkali land. Soils 44, 203–207.
Marin, F., Le Roy, N., and Marie, B. (2012). The formation and mineralization of mollusk shell. Front. Biosci. 4, 1099–1125. doi: 10.2741/s321
Millero, F. J., Woosley, R., Ditrolio, B., and Waters, J. (2009). Effect of ocean acidification on the speciation of metals in seawater. Oceanography 2009, 72–85. doi: 10.5670/oceanog.2009.98
Moon, T. W., and Pritchard, A. W. (1970). Metabolic adaptations in vertically-separated populations of Mytilus californianus Conrad. J. Exp. Mar. Biol. Ecol. 5, 35–46. doi: 10.1016/0022-0981(70)90027-4
Nilsen, I. W., Øverbø, K., Sandsdalen, E., Sandaker, E., Sletten, K., and Myrnes, B. (1999). Protein purification and gene isolation of chlamysin, a cold-active lysozyme-like enzyme with antibacterial activity. FEBS Lett. 464, 153–158. doi: 10.1016/s0014-5793(99)01693-2
Olsen,ØM., Nilsen, I. W., Sletten, K., and Myrnes, B. (2003). Multiple invertebrate lysozymes in blue mussel (Mytilus edulis). Comp. Biochem. Physiol. B. Biochem. Mol. Biol. 136, 107–115. doi: 10.1016/s1096-4959(03)00174-x
Orr, J. C., Fabry, V. J., Aumont, O., Bopp, L., Doney, S. C., Feely, R. A., et al. (2005). Anthropogenic ocean acidification over the twenty-first century and its impact on calcifying organisms. Nature 437, 681–686. doi: 10.1038/nature04095
Otto, J., and Pierce, S. K. (1981). An interaction of extra- and intracellular osmoregulatory mechanisms in the bivalve mollusc Rangia cuneate. Mar. Biol. 61, 193–198. doi: 10.1007/bf00386659
Pan, L., Luan, Z., and Jin, C. (2006). Effects of Na+/K+ and Mg2+/Ca2+ ratios in saline groundwaters on Na+–K+-ATPase activity, survival and growth of Marsupenaeus japonicus postlarvae. Aquaculture 261, 1396–1402. doi: 10.1016/j.aquaculture.2006.09.031
Peng, M., Liu, X., Niu, D., Ye, B., Lan, T., Dong, Z., et al. (2019). Survival, growth and physiology of marine bivalve (Sinonovacula constricta) in long-term low-salt culture. Sci. Rep. 9:2819. doi: 10.1038/s41598-019-39205-2
Peng, M., Niu, D., Chen, Z., Lan, T., Dong, Z., Tran, T.-N., et al. (2017). Expression of a novel complement C3 gene in the razor clam Sinonovacula constricta and its role in innate immune response and hemolysis. Dev. Comp. Immunol. 73, 184–192. doi: 10.1016/j.dci.2017.03.027
Peng, M., Ye, B., Liu, X., Niu, D., Lan, T., Dong, Z., et al. (2018). Effects of alkalinity and ph on survival, growth, and enzyme activities in juveniles of the razor clam, Sinonovacula constricta. Front. Physiol. 9:552. doi: 10.3389/fphys.2018.00552
Pfeifer, S., Schiedek, D., and Dippner, J. W. (2005). Effect of temperature and salinity on acetylcholinesterase activity, a common pollution biomarker, in Mytilus sp. from the south-western Baltic Sea. J. Exp. Mar. Biol. Ecol. 320, 93–103. doi: 10.1016/j.jembe.2004.12.020
Pierce, S. K. (1971). Volume regulation and valve movements by marine mussels. Comp. Biochem. Physiol. 39, 103–117. doi: 10.1016/0300-9629(71)90350-1
Qadir, M., Schubert, S., Ghafoor, A., and Murtaza, G. (2001). Amelioration strategies for sodic soils: a review. Land Degrad. Dev. 12, 357–386. doi: 10.1002/ldr.458
Quinn, D. M. (1987). Acetylcholinesterase: enzyme structure, reaction dynamics, and virtual transition states. Chem. Rev. 87, 955–979. doi: 10.1021/cr00081a005
Raizada, S., Purushothaman, C., Sharma, V., Harikrishna, V., Rahaman, M., Agrahari, R., et al. (2015). Survival and growth of tiger shrimp (Penaeus monodon) in inland saline water supplemented with potassium. Proc. Natl. Acad. Sci. India Sect. B. Biol. Sci. 85, 491–497. doi: 10.1007/s40011-014-0372-1
Rickwood, C. J., and Galloway, T. S. (2004). Acetylcholinesterase inhibition as a biomarker of adverse effect: a study of Mytilus edulis exposed to the priority pollutant chlorfenvinphos. Aquat. Toxicol. 67, 45–56. doi: 10.1016/j.aquatox.2003.11.004
Roy, L. A., Davis, D. A., Saoud, I. P., Boyd, C. A., Pine, H. J., and Boyd, C. E. (2010). Shrimp culture in inland low salinity waters. Rev. Aquac. 2, 191–208. doi: 10.1111/j.1753-5131.2010.01036.x
Roy, L. A., Davis, D. A., Saoud, I. P., and Henry, R. P. (2007). Effects of varying levels of aqueous potassium and magnesium on survival, growth, and respiration of the Pacific white shrimp, Litopenaeus vannamei, reared in low salinity waters. Aquaculture 262, 461–469. doi: 10.1016/j.aquaculture.2006.10.011
Saoud, I. P., Davis, D. A., and Rouse, D. B. (2003). Suitability studies of inland well waters for Litopenaeus vannamei culture. Aquaculture 217, 373–383. doi: 10.1016/s0044-8486(02)00418-0
Shi, Y. (2009). Studies on Water Quality Characteristics and Change Regulations of Culture Ponds with Heavy Saline-Alkaline Soil. Shanghai: Shanghai Ocean University.
Shumway, S. E. (1977). Effect of salinity fluctuation on the osmotic pressure and Na+, Ca2+ and Mg2+ ion concentrations in the hemolymph of bivalve molluscs. Mar. Biol. 41, 153–177. doi: 10.1007/bf00394023
Smith, L. H. Jr., and Pierce, S. K. (1987). Cell volume regulation by molluscan erythrocytes during hypoosmotic stress: Ca2+ effects on ionic and organic osmolyte effluxes. Biol. Bull. 173, 407–418. doi: 10.2307/1541553
Solomon, S., Qin, D., Manning, M., Chen, Z., and Marquis, M. (2007). Climate Change 2007: The Physical Science Basis: Contribution of Working Group I to the Fourth Assessment Report of the Intergovernmental Panel on Climate Change. New York, NY: Cambridge University Press.
Sprung, M. (1987). Ecological requirements of developing Dreissena polymorpha eggs. Arch. Fuer Hydrobiol. Suppl. 79, 69–86.
Suzuki, M., and Nagasawa, H. (2013). Mollusk shell structures and their formation mechanism. Can. J. Zool. 91, 349–366. doi: 10.1139/cjz-2012-0333
Swinehart, J. H., Crowe, J. H., Gianninia, A. P., and Rosenbaum, D. A. (1980). Effects of divalent ion concentration on amino acid and divalent cation fluxes in gills of the bivalve mollusc, Mytilus californianus. J. Exp. Zool. 212, 389–396. doi: 10.1002/jez.1402120311
Tavabe, K. R., Rafiee, G., Frinsko, M., and Daniels, H. (2013). Effects of different calcium and magnesium concentrations separately and in combination on Macrobrachium rosenbergii (de Man) larviculture. Aquaculture 412, 160–166. doi: 10.1016/j.aquaculture.2013.07.023
Tian, X. (2000). Studies on the features of the holbaojinao quarternary salt lake in min-north Erdos basin. J. Geomech. 6, 84–89.
Tomlinson, G., Mutus, B., and McLennan, I. (1981). Activation and inactivation of acetylcholinesterase by metal ions. Can. J. Biochem. 59, 728–735. doi: 10.1139/o81-101
Van Der Borght, O., and Van Puymbroeck, S. (1966). Calcium metabolism in a freshwater mollusc: quantitative importance of water and food as supply for calcium during growth. Nature 210, 791–793. doi: 10.1038/210791a0
Virkar, R. A. (1966). The role of free amino acids in the adaptation to reduced salinity in the sipunculid Golfingia gouldii. Comp. Biochem. Physiol. 18, 617–625. doi: 10.1016/0010-406x(66)90245-3
Wang, J., Jin, H., Zhong, T., and Chen, Z. (2011). Review on sustainable utilization of salt-affected land. Acta Geogr. Sin. 66, 673–684.
Wang, Y., Lin, J., Li, Q., Hu, M., Wu, F., Sha, Y., et al. (2014). Characterization of the subpopulation and flow cytometric analysis of immune-related parameters of haemocytes in the green-lipped mussel Perna viridis. J. Fish. Chin. 38, 385–399.
Watts, J. A., and Pierce, S. K. (1978). A correlation between the activity of divalent cation activated adenosine triphosphatase in the cell membrane and low salinity tolerance of the ribbed mussel, Modiolus demissus demissus. J. Exp. Zool. 204, 49–56. doi: 10.1002/jez.1402040106
Wu, Z., Pan, L., and Dong, S. (2003). Effects of nine metal ions on digestive enzyme activities of Sinonovacula constricta. J. Fisher. Sci. Chin. 10, 297–300.
Xue, Q., Hellberg, M. E., Schey, K. L., Itoh, N., Eytan, R. I., Cooper, R. K., et al. (2010). A new lysozyme from the eastern oyster, Crassostrea virginica, and a possible evolutionary pathway for i-type lysozymes in bivalves from host defense to digestion. BMC Evol. Biol. 10:213. doi: 10.1186/1471-2148-10-213
Yang, J. (2011). Effects of Varying Levels of Potassium Supplementation to the Low Salinity Waters or Diets on Growth and Physiological Characteristics of Shrimp, Litopenaeus vannamei, Boone. Zhanjiang: Guangdong Ocean University.
Yao, Z., Wang, H., Zhou, K., Ying, C., and Lai, Q. (2010). Effects of water carbonate alkalinity and pH on survival rate of post-larval Litopenaeus vannamei. Chin. J. Ecol. 29, 945–950.
Zhang, Z., and Zhang, Z. (1999). Advance in research on the effects of pH, salinity and alkalinity on freshwater cultured species. J. Fisher. Sci. Chin. 6, 95–98.
Keywords: Sinonovacula constricta, Ca2+ and Mg2+ ions, survive, growth, physiology
Citation: Peng M, Li Z, Liu X, Niu D, Lan T, Ye B, Dong Z and Li J (2019) Tolerance, Growth, and Physiological Responses of the Juvenile Razor Clam (Sinonovacula constricta) to Environmental Ca2+ and Mg2+ Concentrations. Front. Physiol. 10:911. doi: 10.3389/fphys.2019.00911
Received: 03 April 2019; Accepted: 03 July 2019;
Published: 17 July 2019.
Edited by:
Alexssandro Geferson Becker, Universidade Federal do Paraná, Setor Palotina, BrazilReviewed by:
Tiziano Verri, University of Salento, ItalyZélia Cristina Pereira Velez, University of Algarve, Portugal
Copyright © 2019 Peng, Li, Liu, Niu, Lan, Ye, Dong and Li. This is an open-access article distributed under the terms of the Creative Commons Attribution License (CC BY). The use, distribution or reproduction in other forums is permitted, provided the original author(s) and the copyright owner(s) are credited and that the original publication in this journal is cited, in accordance with accepted academic practice. No use, distribution or reproduction is permitted which does not comply with these terms.
*Correspondence: Jiale Li, amxsaTIwMDlAMTI2LmNvbQ==
†These authors have contributed equally to this work