- 1Aspetar Orthopaedic and Sports Medicine Hospital, Doha, Qatar
- 2Research Institute for Sport and Exercise, University of Canberra, Canberra, ACT, Australia
Introduction
Musculoskeletal injuries are arguably one of the most severe impediments athletes may encounter in their career. Depending on the severity of the sustained injury, time-loss could be substantial, leading to profound de-conditioning effects within the cardiovascular, metabolic and muscular systems. For instance, following ACL reconstruction, athletes' return to sport may range between 16 and 52 weeks (Anderson et al., 2019). Case studies in elite soccer players have reported profound losses in whole body fat-free mass (5.8 kg), as well as in lean leg mass (0.9–1.5 kg) following immobilization and inactivity during this period (Milsom et al., 2014; Anderson et al., 2019). Moreover, a 4–20% decline in maximal aerobic capacity (VO2max) has been reported following 2–8 weeks of physical de-conditioning, owing largely to the decline in blood volume, and consequently stroke volume and cardiac output (Mujika and Padilla, 2001). In this context, there is a crucial need to optimize rehabilitation programs, such that the physical demands associated with return to sport and beyond are well-tolerated.
Here we propose that an athlete's rehabilitation program may be optimized by incorporating repeated heat exposures or heat acclimation, given the evidence in support of this modality to minimize skeletal muscle and cardiovascular de-conditioning during periods of disuse and reduced physical activity. For example, there is emerging evidence demonstrating remarkable benefits of heat stress on the regulation of muscle mass (Selsby and Dodd, 2005; Selsby et al., 2007; Ihsan et al., 2014; Hafen et al., 2019). Moreover, training in the heat allows for maintaining a lower absolute work load for a given relative exercise intensity, reducing the mechanical demands of the rehabilitating athlete. While it is important to note that some (Shvartz et al., 1977; Sawka et al., 1985; Lorenzo et al., 2010), but not all (Karlsen et al., 2015; Keiser et al., 2015; Neal et al., 2016) studies have reported improved exercise performance in cooler environments following heat acclimation, harnessing the benefits through passive or active heat acclimation remains a promising strategy to minimize cardiovascular de-conditioning in a rehabilitating athlete, whose training load is considerably reduced. This opinion piece presents how repeated heat exposures may be incorporated in the rehabilitation toolbox at various stages of the return to sport journey (Figure 1).
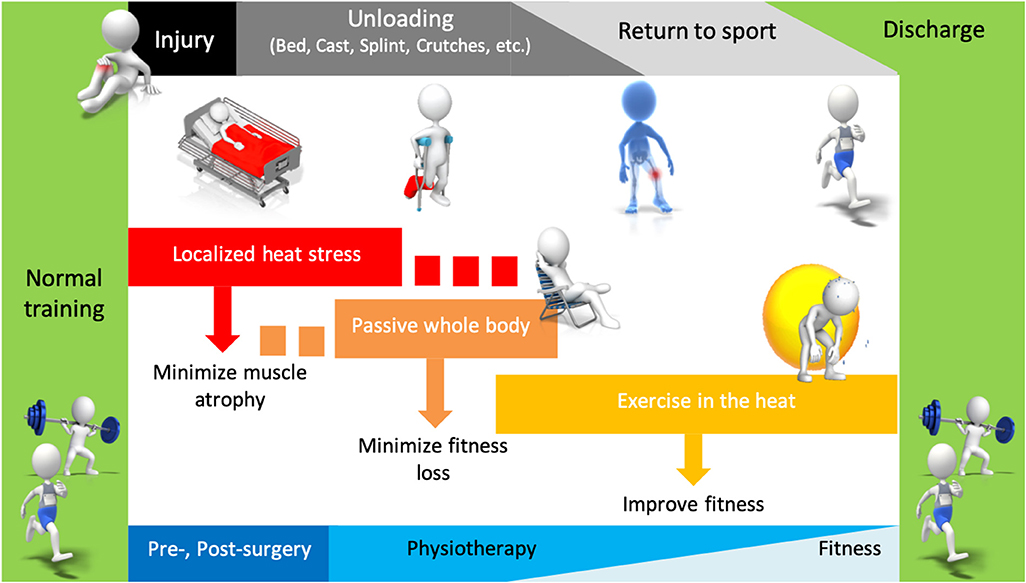
Figure 1. Depiction of how the therapeutic use of passive or active heat stress may be utilized at different stages of rehabilitation to limit muscle atrophy and decline in fitness, optimizing the return to sport.
Muscle Adaptations to Heat Therapy to Minimize Muscle Atrophy When Immobilized
During the first phase of the rehabilitation program, including pre- and post-surgery, heat exposure may be used to minimize the loss in muscle mass (Figure 1). Indeed, the effects of heat stress on the regulation of muscle mass has been relatively well-studied in cell cultures and rodents, demonstrating remarkable benefits. For instance, heat stress has been shown to enhance the recovery of muscle mass within atrophied muscles (Goto et al., 2004; Selsby et al., 2007), as well as attenuate the loss in muscle mass in aging (Ohno et al., 2012), disuse (Selsby and Dodd, 2005), and pharmacological (Tsuchida et al., 2017) models of muscle atrophy. Moreover, heat exposure has been shown to preserve muscle mass and minimize protein degradation in a variety of muscle trauma models including ischemic injury (Garramone et al., 1994), crush injury (Takeuchi et al., 2014), exercise-induced muscle damage (Touchberry et al., 2012), and pharmacologically-induced muscle toxicity (Kojima et al., 2007; Shibaguchi et al., 2016).
The potential for heat-based interventions to be an integrative part of a rehabilitation strategy has, therefore, gathered considerable enthusiasm lately. This surge in interest is in-part due to recent studies in humans demonstrating improved muscle contractile function (Racinais et al., 2017b) or attenuated loss of muscle mass during immobilization (Hafen et al., 2019) following short-term heat exposure. Specifically, Racinais et al. (2017b) showed that 11 days of passive whole body heat exposure (60 min @ 48–50°C, 50% RH) resulted in an increased peak twitch amplitude, improved maximal voluntary torque, as well as the relative torque/electromyographic relationship. Additionally, more recent data by Hafen et al. (2019) showed that the decline in muscle mass following 10 days of lower limb immobilization was in-part attenuated when 120 min of localized heating of the vastus lateralis was administered daily. The preserved muscle mass following heat treatment coincided with the protein abundance of the transcriptional co-activator PGC-1α, HSP 70, and HSP 90, as well as minimized loss of mitochondrial respiratory chain protein content and mitochondrial respiratory function (Hafen et al., 2019). These findings are in general agreement with evidence gathered from cultured cells and rodents, where heat stress had been shown to mitigate muscle atrophic pathways through HSPs, PGC-1α, and mitochondrial signaling (Sandri et al., 2006; Romanello et al., 2010; Cannavino et al., 2015).
In addition to a mitochondrial and HSP centered mechanism, data gathered from cell cultures and rodent models implicate the Akt-mTOR cascade as a potential pathway by which heat stress might preserve muscle mass during immobilization. For instance, acute exposures (30–60 min) to environmental heat (39–41°C) has been shown to increase anabolic signaling (i.e., Akt-mTOR pathway), in line with increased muscle protein content (Goto et al., 2003; Uehara et al., 2004; Ohno et al., 2012; Yoshihara et al., 2013). Apart from initiating protein synthesis per se, activation of Akt has also been shown to suppress protein degradation through inactivating FOXO3, a key transcription factor regulating the expression of atrophic genes (Sandri et al., 2004). Nevertheless, while there is considerable evidence indicating that Akt-mTOR signaling may be up-regulated following heat stress, further evidence involving humans is further needed to verify this pathway within immobilization and injury models.
Passive Heat Acclimation to Minimize De-conditioning Effects When Not Training
In addition to protecting muscle mass, passive heat exposures may also help to maintain cardiovascular fitness from the onset of injury (Figure 1). Indeed, the cardiovascular and thermoregulatory adaptations conferred by repeated heat exposures are somewhat similar to the adaptations acquired through exercise per se, but with some specificities to improve thermoregulation. Importantly, a large part of the cardiovascular and thermoregulatory adaptations conferred by exercise heat stress may also be obtained through passive exposures in the condition that they sufficiently trigger an increase in body temperature, circulation, and sweating (Racinais et al., 2015; Périard et al., 2016). With recent studies demonstrating the accrue of such adaptations following passive heat exposures (Brazaitis and Skurvydas, 2010; Racinais et al., 2017a,b), there is intuitively an interest in developing heat-related therapeutic modalities for injured athletes restricted from training. The purpose of such modalities would be to induce cardiovascular adaptations (e.g., blood volume expansion) that aid in attenuating the loss of fitness, given the deterioration in cardiovascular adaptation substantially accounts for the decrease in VO2max during de-conditioning (Mujika and Padilla, 2001). Practical passive heat acclimation strategies include hot water immersion or sauna bathing. Saunas are typically 80–90°C, whereas water temperature when immersing into a bath should be 40–42°C to induce adaptation, while remaining tolerable. These modalities are typically undertaken for 30–60 min, depending on whether heating was preceded by exercise. Guidelines regarding passive heating strategies are comparable to exercise heat acclimation and include consecutive days of exposure with a minimum of 5–7 exposures for initiating adaptations (Heathcote et al., 2018).
Heat Training to Optimize the Relative/Absolute Workload in Injured Athletes
Once the athlete can progressively load the injured limb but is still unable to undertake heavy training, heat exposure during mild exercise may enhance the re-conditioning process (Figure 1). Indeed, exercise heat exposure provides a unique challenge to the cardiovascular system, which must not only supply blood to exercising muscles (i.e., oxygen delivery), but also to the peripheral vasculature (i.e., heat loss). When prolonged exercise is undertaken under heat stress and whole-body temperature increases, the cardiovascular response is progressively exacerbated compared to that of temperate conditions (Périard et al., 2013). This exacerbation is characterized by a compromise in the maintenance of mean arterial blood pressure and cardiac output, as heart rate drifts upward and stroke volume decreases (Rowell, 1974; Coyle and Gonzalez-Alonso, 2001). This forces the cardiovascular system toward a functional limit (i.e., VO2max) (Nybo et al., 2001; Arngrimsson et al., 2003) with a progressive hyperthermia-induced reduction in VO2max increasing the relative exercise intensity (i.e., %VO2max) for a given absolute workload (Périard et al., 2011; Périard and Racinais, 2015). Thus, the hyperthermia-induced dissociation between relative and absolute exercise intensity (Périard and Racinais, 2015) may allow injured athletes to train at a given heart rate or percentage of VO2max for a lower absolute mechanical load in hot than temperate conditions.
Although the exacerbated cardiovascular response inherent with exercising under heat stress impairs the ability to perform optimally in hot environments, it may provide a pathway for injured athletes to train at high relative intensities without having to produce high levels of mechanical work with an injured limb. For example, injured athletes could exercise at a workload equivalent to a particular %VO2max, which as the session progressed, would result in increasing cardiovascular (Wingo, 2015) and metabolic (Febbraio et al., 1994) responses. To expedite these responses and avoid having to produce high levels of mechanical work in the early part of exercise sessions, prior to the development of whole-body hyperthermia, exercise may be preceded by passive heating.
Ultimately, we suggest that injured athletes may benefit from training in the heat as the cardio-metabolic load associated with a given mechanical workload increases progressively with the development of hyperthermia. This may help maintain fitness or attenuate the loss of aerobic capacity associated with a prolonged rehabilitation process following an injury, particularly in those unable to fully load the injured limb.
Conclusion
In summary, the therapeutic use of either exercise or passive heat exposure is a novel and emerging modality to assist with post-injury rehabilitation or re-conditioning. The use of such modalities can be targeted toward minimizing cardiovascular de-conditioning in athletes who are unable to undertake their usual mechanical loading. Moreover, drawing from the extensive research in rodent and cell cultures, as well as emerging evidence in humans, the use of passive heat application may minimize the decline in muscle mass during immobilization or periods of severely restricted exercise. There is a need though, to undertake further research toward optimizing the delivery of passive and active heat treatment modalities (i.e., whole-body vs. limb only, optimal muscle temperature and duration, dose-response, etc.). Such information would aid in the development of evidence-based heat therapy protocols for both sporting and clinical situations.
Author Contributions
MI, JP and SR conceived and contributed to writing the manuscript. All authors provided critical feedback approved the final version of the manuscript.
Conflict of Interest
The authors declare that the research was conducted in the absence of any commercial or financial relationships that could be construed as a potential conflict of interest.
References
Anderson, L., Close, G. L., Konopinksi, M., Rydings, D., Milsom, J., Hambly, C., et al. (2019). Case study: muscle atrophy, hypertrophy and energy expenditure of a premier league soccer player during rehabilitation from ACL injury. Int. J. Sport Nutr. Exerc. Metab. 29, 559–566. doi: 10.1123/ijsnem.2018-0391
Arngrimsson, S. A., Stewart, D. J., Borrani, F., Skinner, K. A., and Cureton, K. J. (2003). Relation of heart rate to percentV o 2 peak during submaximal exercise in the heat. J. Appl. Physiol. 94, 1162–1168. doi: 10.1152/japplphysiol.00508.2002
Brazaitis, M., and Skurvydas, A. (2010). Heat acclimation does not reduce the impact of hyperthermia on central fatigue. Eur. J. Appl. Physiol. 109, 771–778. doi: 10.1007/s00421-010-1429-3
Cannavino, J., Brocca, L., Sandri, M., Grassi, B., Bottinelli, R., and Pellegrino, M. A. (2015). The role of alterations in mitochondrial dynamics and PGC-1α over-expression in fast muscle atrophy following hindlimb unloading. J. Physiol. 593, 1981–1995. doi: 10.1113/jphysiol.2014.286740
Coyle, E., and Gonzalez-Alonso, J. (2001). Cardiovascular drift during prolonged exercise: new perspectives. Exerc. Sport Sci. Rev. 29, 88–92. doi: 10.1249/00003677-200104000-00009
Febbraio, M., Snow, R., Stathis, C., Hargreaves, M., and Carey, M. (1994). Effect of heat stress on muscle energy metabolism during exercise. J. Appl. Physiol. 77, 2827–2831. doi: 10.1152/jappl.1994.77.6.2827
Garramone, J. R., Winters, R. M., Das, D. K., and Deckers, P. J. (1994). Reduction of skeletal muscle injury through stress conditioning using the heat-shock response. Plastic Reconstr. Surg. 93, 1242–1247. doi: 10.1097/00006534-199405000-00021
Goto, K., Honda, M., Kobayashi, T., Uehara, K., Kojima, A., Akema, T., et al. (2004). Heat stress facilitates the recovery of atrophied soleus muscle in rat. Jpn. J. Physiol. 54, 285–293. doi: 10.2170/jjphysiol.54.285
Goto, K., Okuyama, R., Sugiyama, H., Honda, M., Kobayashi, T., Uehara, K., et al. (2003). Effects of heat stress and mechanical stretch on protein expression in cultured skeletal muscle cells. Pflügers Archiv. 447, 247–253. doi: 10.1007/s00424-003-1177-x
Hafen, P. S., Abbott, K., Bowden, J. A., Lopiano, R., Hancock, C. R., and Hyldahl, R. D. (2019). Daily heat treatment maintains mitochondrial function and attenuates atrophy in human skeletal muscle subjected to immobilization. J. Appl. Physiol. 127, 47–57. doi: 10.1152/japplphysiol.01098.2018
Heathcote, S. L., Hassmen, P., Zhou, S., and Stevens, C. J. (2018). Passive heating: reviewing practical heat acclimation strategies for endurance athletes. Front. Physiol. 9:1851. doi: 10.3389/fphys.2018.01851
Ihsan, M., Watson, G., and Abbiss, C. (2014). PGC-1α mediated muscle aerobic adaptations to exercise, heat and cold exposure. Cell. Mol. Exerc. Physiol. 3:e7. doi: 10.7457/cmep.v3i1.e7
Karlsen, A., Racinais, S., Jensen, M., Nørgaard, S., Bonne, T., and Nybo, L. (2015). Heat acclimatization does not improve VO2max or cycling performance in a cool climate in trained cyclists. Scand. J. Med. Sci. Sports 25, 269–276. doi: 10.1111/sms.12409
Keiser, S., Flück, D., Hüppin, F., Stravs, A., Hilty, M. P., and Lundby, C. (2015). Heat training increases exercise capacity in hot but not in temperate conditions: a mechanistic counter-balanced cross-over study. Am. J. Physiol. Heart Circ. Physiol. 309, H750–H761. doi: 10.1152/ajpheart.00138.2015
Kojima, A., Goto, K., Morioka, S., Naito, T., Akema, T., Fujiya, H., et al. (2007). Heat stress facilitates the regeneration of injured skeletal muscle in rats. J. Orthop. Sci. 12, 74–82. doi: 10.1007/s00776-006-1083-0
Lorenzo, S., Halliwill, J. R., Sawka, M. N., and Minson, C. T. (2010). Heat acclimation improves exercise performance. J. Appl. Physiol. 109, 1140–1147. doi: 10.1152/japplphysiol.00495.2010
Milsom, J., Barreira, P., Burgess, D. J., Iqbal, Z., and Morton, J. P. (2014). Case study: muscle atrophy and hypertrophy in a premier league soccer player during rehabilitation from ACL injury. Int. J. Sport Nutr. Exerc. Metab. 24, 543–552. doi: 10.1123/ijsnem.2013-0209
Mujika, I., and Padilla, S. (2001). Cardiorespiratory and metabolic characteristics of detraining in humans. Med. Sci. Sport. Exerc. 33, 413–421. doi: 10.1097/00005768-200103000-00013
Neal, R. A., Corbett, J., Massey, H. C., and Tipton, M. J. (2016). Effect of short-term heat acclimation with permissive dehydration on thermoregulation and temperate exercise performance. Scand. J. Med. Sci. Sports 26, 875–884. doi: 10.1111/sms.12526
Nybo, L., Jensen, T., Nielsen, B., and González-Alonso, J. (2001). Effects of marked hyperthermia with and without dehydration onV o 2 kinetics during intense exercise. J. Appl. Physiol. 90, 1057–1064. doi: 10.1152/jappl.2001.90.3.1057
Ohno, Y., Yamada, S., Goto, A., Ikuta, A., Sugiura, T., Ohira, Y., et al. (2012). Effects of heat stress on muscle mass and the expression levels of heat shock proteins and lysosomal cathepsin L in soleus muscle of young and aged mice. Mol. Cell. Biochem. 369, 45–53. doi: 10.1007/s11010-012-1367-y
Périard, J. D., Cramer, M. N., Chapman, P. G., Caillaud, C., and Thompson, M. W. (2011). Cardiovascular strain impairs prolonged self-paced exercise in the heat. Exp. Physiol. 96, 134–144. doi: 10.1113/expphysiol.2010.054213
Périard, J. D., and Racinais, S. (2015). Self-paced exercise in hot and cool conditions is associated with the maintenance of% VO2peak within a narrow range. J. Appl. Physiol. 118, 1258–1265. doi: 10.1152/japplphysiol.00084.2015
Périard, J. D., Thompson, M. W., Caillaud, C., and Quaresima, V. (2013). Influence of heat stress and exercise intensity on vastus lateralis muscle and prefrontal cortex oxygenation. Eur. J. Appl. Physiol. 113, 211–222. doi: 10.1007/s00421-012-2427-4
Périard, J. D., Travers, G. J., Racinais, S., and Sawka, M. N. (2016). Cardiovascular adaptations supporting human exercise-heat acclimation. Auton. Neurosci. 196, 52–62. doi: 10.1016/j.autneu.2016.02.002
Racinais, S., Alonso, J.-M., Coutts, A. J., Flouris, A. D., Girard, O., González-Alonso, J., et al. (2015). Consensus recommendations on training and competing in the heat. Scand. J. Med. Sci. Sports 25, 6–19. doi: 10.1111/sms.12467
Racinais, S., Wilson, M. G., Gaoua, N., and Périard, J. D. (2017a). Heat acclimation has a protective effect on the central but not peripheral nervous system. J. Appl. Physiol. 123, 816–824. doi: 10.1152/japplphysiol.00430.2017
Racinais, S., Wilson, M. G., and Périard, J. D. (2017b). Passive heat acclimation improves skeletal muscle contractility in humans. Am. J. Physiol. Regul. Integr. Comp. Physiol. 312, R101–R107. doi: 10.1152/ajpregu.00431.2016
Romanello, V., Guadagnin, E., Gomes, L., Roder, I., Sandri, C., Petersen, Y., et al. (2010). Mitochondrial fission and remodelling contributes to muscle atrophy. EMBO J. 29, 1774–1785. doi: 10.1038/emboj.2010.60
Rowell, L. B. (1974). Human cardiovascular adjustments to exercise and thermal stress. Physiol. Rev. 54, 75–159. doi: 10.1152/physrev.1974.54.1.75
Sandri, M., Lin, J., Handschin, C., Yang, W., Arany, Z. P., Lecker, S. H., et al. (2006). PGC-1α protects skeletal muscle from atrophy by suppressing FoxO3 action and atrophy-specific gene transcription. Proc. Natl. Acad. Sci. U.S.A. 103, 16260–16265. doi: 10.1073/pnas.0607795103
Sandri, M., Sandri, C., Gilbert, A., Skurk, C., Calabria, E., Picard, A., et al. (2004). Foxo transcription factors induce the atrophy-related ubiquitin ligase atrogin-1 and cause skeletal muscle atrophy. Cell 117, 399–412. doi: 10.1016/S0092-8674(04)00400-3
Sawka, M. N., Young, A. J., Cadarette, B. S., Levine, L., and Pandolf, K. B. (1985). Influence of heat stress and acclimation on maximal aerobic power. Eur. J. Appl. Physiol. Occup. Physiol. 53, 294–298. doi: 10.1007/BF00422841
Selsby, J. T., and Dodd, S. L. (2005). Heat treatment reduces oxidative stress and protects muscle mass during immobilization. Am. J. Physiol. Regul. Integr. Comp. Physiol. 289, R134–R139. doi: 10.1152/ajpregu.00497.2004
Selsby, J. T., Rother, S., Tsuda, S., Pracash, O., Quindry, J., and Dodd, S. L. (2007). Intermittent hyperthermia enhances skeletal muscle regrowth and attenuates oxidative damage following reloading. J. Appl. Physiol. 102, 1702–1707. doi: 10.1152/japplphysiol.00722.2006
Shibaguchi, T., Sugiura, T., Fujitsu, T., Nomura, T., Yoshihara, T., Naito, H., et al. (2016). Effects of icing or heat stress on the induction of fibrosis and/or regeneration of injured rat soleus muscle. J. Physiol. Sci. 66, 345–357. doi: 10.1007/s12576-015-0433-0
Shvartz, E., Shapiro, Y., Magazanik, A., Meroz, A., Birnfeld, H., Mechtinger, A., et al. (1977). Heat acclimation, physical fitness, and responses to exercise in temperate and hot environments. J. Appl. Physiol. 43, 678–683. doi: 10.1152/jappl.1977.43.4.678
Takeuchi, K., Hatade, T., Wakamiya, S., Fujita, N., Arakawa, T., and Miki, A. (2014). Heat stress promotes skeletal muscle regeneration after crush injury in rats. Acta Histochem. 116, 327–334. doi: 10.1016/j.acthis.2013.08.010
Touchberry, C. D., Gupte, A. A., Bomhoff, G. L., Graham, Z. A., Geiger, P. C., and Gallagher, P. M. (2012). Acute heat stress prior to downhill running may enhance skeletal muscle remodeling. Cell Stress Chaperones 17, 693–705. doi: 10.1007/s12192-012-0343-5
Tsuchida, W., Iwata, M., Akimoto, T., Matsuo, S., Asai, Y., and Suzuki, S. (2017). Heat stress modulates both anabolic and catabolic signaling pathways preventing dexamethasone-induced muscle atrophy in vitro. J. Cell. Physiol. 232, 650–664. doi: 10.1002/jcp.25609
Uehara, K., Goto, K., Kobayashi, T., Kojima, A., Akema, T., Sugiura, T., et al. (2004). Heat-stress enhances proliferative potential in rat soleus muscle. Jpn. J. Physiol. 54, 263–271. doi: 10.2170/jjphysiol.54.263
Wingo, J. (2015). Exercise intensity prescription during heat stress: a brief review. Scand. J. Med. Sci. Sports 25, 90–95. doi: 10.1111/sms.12381
Keywords: heat acclimation, heat therapy, physiotherapy (rehabilitation), muscle hypertrophy, muscle atrophy, passive heating, thermal therapy
Citation: Ihsan M, Périard JD and Racinais S (2019) Integrating Heat Training in the Rehabilitation Toolbox for the Injured Athlete. Front. Physiol. 10:1488. doi: 10.3389/fphys.2019.01488
Received: 22 July 2019; Accepted: 21 November 2019;
Published: 11 December 2019.
Edited by:
Billy Sperlich, Julius Maximilian University of Würzburg, GermanyReviewed by:
Christopher James Tyler, University of Roehampton London, United KingdomGeoffrey M. Minett, Queensland University of Technology, Australia
Copyright © 2019 Ihsan, Périard and Racinais. This is an open-access article distributed under the terms of the Creative Commons Attribution License (CC BY). The use, distribution or reproduction in other forums is permitted, provided the original author(s) and the copyright owner(s) are credited and that the original publication in this journal is cited, in accordance with accepted academic practice. No use, distribution or reproduction is permitted which does not comply with these terms.
*Correspondence: Sébastien Racinais, c2ViYXN0aWVuLnJhY2luYWlzQGFzcGV0YXIuY29t