- 1Division of Pulmonary, Critical Care, and Sleep Medicine, Department of Medicine, University of California, San Diego, San Diego, CA, United States
- 2Laboratorio de Fisiología Comparada/Fisiología del Transporte de Oxígen, Facultad de Ciencias y Filosofía, Universidad Peruana Cayetano Heredia, Lima, Peru
- 3Faculty of Medicine, University of Brasília, Brasília, Brazil
Andean highlanders are challenged by chronic hypoxia and many exhibit elevated hematocrit (Hct) and blunted ventilation compared to other high-altitude populations. While many Andeans develop Chronic Mountain Sickness (CMS) and excessive erythrocytosis, Hct varies markedly within Andean men and women and may be driven by individual differences in ventilatory control and/or sleep events which exacerbate hypoxemia. To test this hypothesis, we quantified relationships between resting ventilation and ventilatory chemoreflexes, sleep desaturation, breathing disturbance, and Hct in Andean men and women. Ventilatory measures were made in 109 individuals (n = 63 men; n = 46 women), and sleep measures in 45 of these participants (n = 22 men; n = 23 women). In both men and women, high Hct was associated with low daytime SpO2 (p < 0.001 and p < 0.002, respectively) and decreased sleep SpO2 (mean, nadir, and time <80%; all p < 0.02). In men, high Hct was also associated with increased end-tidal PCO2 (p < 0.009). While ventilatory responses to hypoxia and hypercapnia did not predict Hct, decreased hypoxic ventilatory responses were associated with lower daytime SpO2 in men (p < 0.01) and women (p < 0.009) and with lower nadir sleep SpO2 in women (p < 0.02). Decreased ventilatory responses to CO2 were associated with more time below 80% SpO2 during sleep in men (p < 0.05). The obstructive apnea index and apnea-hypopnea index also predicted Hct and CMS scores in men after accounting for age, BMI, and SpO2 during sleep. Finally, heart rate response to hypoxia was lower in men with higher Hct (p < 0.0001). These data support the idea that hypoventilation and decreased ventilatory sensitivity to hypoxia are associated with decreased day time and nighttime SpO2 levels that may exacerbate the stimulus for erythropoiesis in Andean men and women. However, interventional and longitudinal studies are required to establish the causal relationships between these associations.
Introduction
Chronic Mountain Sickness (CMS) in native and long-term high-altitude residents is characterized by excessive erythrocytosis and results in severe hypoxemia, sleep disturbance, and neurological symptoms (León-Velarde et al., 2005; Villafuerte and Corante, 2016). In the southern Andes of Peru at 3,825 m, the estimated average prevalence of excessive erythrocytosis is 6% of adult men and 3% of adult women; while in the central Andes at 4,340 m, the prevalence increases to 25 and 15%, respectively (Monge et al., 1989, 1992; De Ferrari et al., 2014). Age is a compounding risk factor whereby the prevalence of excessive erythrocytosis is >30% in highlanders by their mid-50s in both sexes above 4,000 m, including about 77% of post-menopausal women (Monge et al., 1989, 1992; León-Velarde et al., 1997).
Individuals with excessive erythrocytosis are at higher risk of pulmonary hypertension and adverse cardiovascular events (Penaloza et al., 1971; Penaloza and Arias-Stella, 2007; Corante et al., 2018). While CMS and excessive erythrocytosis are highly prevalent in Andeans (Villafuerte and Corante, 2016), these conditions are rare among Tibetans who tend to exhibit sea-level hemoglobin concentration despite residence at comparable altitudes (Wu et al., 2005). The range of hematocrit (Hct) is notable among Andeans (Beall et al., 1998), and individual variation in traits that affect oxygen (O2) delivery, including ventilatory control and its impact on sleep disordered breathing, may contribute to the excessive production of red blood cells.
Andeans also demonstrate notable hypoventilation and a lower average hypoxic ventilatory response (HVR) compared to Tibetans (Zhuang et al., 1993; Beall et al., 1997). While acute HVR appears to be reduced in Andeans with or without CMS, lower ventilatory sensitivities to CO2 have been observed specifically in individuals with CMS compared to healthy Andean controls (Fatemian et al., 2003; Leon-Velarde et al., 2003). Lower central and peripheral chemoreflex set points might lead CMS individuals to hypoventilate at a given CO2 partial pressure (PCO2), resulting in lower arterial O2 saturation and increased erythropoietic responses.
Ventilatory sensitivities to O2 and CO2 also play a key role in sleep disordered breathing in highlanders (Spicuzza et al., 2004; Julian et al., 2013). High ventilatory sensitivity to hypoxia can lead to Cheyne-Stokes respiration (periodic breathing, waxing, and waning breathing patterns) at high altitude (Lahiri et al., 1983; Masuyama et al., 1989; Goldenberg et al., 1992; Küpper et al., 2008), while low ventilatory sensitivity to hypoxia or CO2 can lead to more severe desaturation during sleep and/or prolonged desaturation periods (Azarbarzin et al., 2019). Both situations can lead to maladaptive cardiovascular outcomes. Sleep disordered breathing is more prevalent in Peruvian highlanders than lowlanders at sea level (Pham et al., 2017a), and nocturnal hypoxemia and sleep apnea events are separately associated with excessive erythrocytosis and glucose intolerance, respectively (Pham et al., 2017b). The severity, frequency, and duration of intermittent desaturation on top of chronic hypoxemia likely influence erythropoiesis and other hypoxia-related pathways that contribute to negative cardiovascular outcomes.
We aimed to determine whether higher Hct is associated with lower ventilatory chemosensitivity and/or more frequent or severe desaturation events during sleep, and tested whether the uniquely low chemoreflex sensitivities observed in this population may contribute to more severe desaturation during sleep. By examining Hct, ventilatory chemoreflexes, and sleep quality in the same individuals, we identified, for the first time, associations between individual ventilatory control and sleep disturbance profiles that are linked to Hct in both men and women. We also show decreased heart rate response (HRR) to hypoxia in men with high Hct. These findings highlight relevant individual and sex-specific profiles that should be prioritized for functional investigation in future studies.
Materials and Methods
Ethical Approval
This study was conducted in accordance with the Declaration of Helsinki, except for registration in a database, and was approved by the University of California, San Diego Human Research Protection Program. Participants provided written consent in their native language (Spanish).
Participants and Preliminary Screening Visit
This study took place at the Instituto de Investigaciones de la Altura laboratory in the city of Cerro de Pasco, Peru (∼4,340 m; population ∼70,000). Men and women who were lifelong residents of Cerro de Pasco were recruited by word of mouth and flyers. Inclusion criteria was defined as individuals 18 to 65 years old with at least three previous generations of self-reported high-altitude (>2,500 m) Andean ancestry (self-identified ancestry and geographical location of their parents and grandparents). Women of reproductive age completed a urine pregnancy test to verify they were not pregnant. Participants were excluded if they had a self-reported history of pulmonary, cardiovascular, or renal disease to rule out secondary CMS cases (Villafuerte and Corante, 2016). Participants were also excluded if they were current smokers or regular drinkers, had recently undergone blood transfusions or phlebotomies, had traveled to low-altitude (<4,000 m) during the previous six months, or demonstrated abnormal cardiac or pulmonary function during screening procedures (EKG and spirometry). Table 1 provides an overview of the study population demographics.
Participants were asked not to drink alcohol or caffeine, nor consume/chew coca leaves or tea, for 8 h prior to testing and were asked to refrain from taking anti-inflammatory drugs for 24 h prior to testing. Medical histories and physical examinations were performed during a preliminary screening session to verify no prior history of cardiovascular or pulmonary disease or current use of interfering medications that would preclude ventilatory control assessment. During this visit, participants were assessed for presence and severity of CMS based on the Qinghai CMS scoring criteria, which considers hemoglobin concentration at the altitude of residence and the presence and severity of the following symptoms: breathlessness and/or palpitations, sleep disturbance, cyanosis, dilation of veins, paresthesia, headache, and tinnitus (Wu et al., 1997, 1998; León-Velarde et al., 2005).
Average Hct was determined from duplicate microcentrifuged blood samples obtained from a single fingertip capillary blood draw. Individuals were determined to have excessive erythrocytosis if they had an Hct ≥ 63 % for men or ≥57% for women, which are equivalent to the thresholds for hemoglobin in the Qinghai CMS Score criteria (Wu et al., 1997, 1998; León-Velarde et al., 2005). Our group has previously determined that mean corpuscular hemoglobin concentration is effectively similar between men with Hct ≤ 54% (n = 84, MCHC = 34.1 ± 1.8 g/dl) and Hct ≥ 63% (n = 95, MCHC = 33.0 ± 1.7 g/dl). For this, and practical reasons, we only measured Hct in this study.
Ventilatory control was measured in 109 individuals [n = 63 men and 46 women (31 pre and 15 post menopause)] and sleep parameters in a subset of this group [n = 45; 22 men and 23 women (14 pre and 9 post menopause)].
Ventilatory Chemoreflex Measurements
Participants completed a 10-min abbreviated version of the ventilatory response protocol to allow acclimation to the devices and determine appropriate individual gas flows to reach their target SpO2 and end-tidal PCO2 (PETCO2) values. Participants returned after a >30-min rest period to complete the full protocol. This rest period and preparation for the next test allowed sufficient time for recovery from hypoxic ventilatory decline induced by the screening session hypoxia exposure (Easton et al., 1988; Robbins, 2007).
We used a protocol developed over several years for measuring the steady-state isocapnic HVR. This method is a modification of the protocol utilized by the Severinghaus laboratory (Sato et al., 1992, 1994) as previously described (Hupperets et al., 2004; Basaran et al., 2016) (Figure 1 illustrates the experimental setup). During testing, participants sat in a chair in a semi-recumbent position and a mask was placed over the mouth and nose (7600 V2 Oro-Nasal Mask, Hans Rudolph Inc., Shawnee, KS, United States); leaks were checked by having the participant inhale against a closed inspiratory valve to ensure a vacuum was produced. The mask was connected to a one-way, vented partial rebreathing circuit with a non-rebreathing valve (2700 Series, Large, Hans Rudolph Inc.). A three-way valve upstream of the mask allowed either room air or N2/O2/CO2 gas mixtures to flow into the circuit. O2 and CO2 were continuously sampled from the non-rebreathing valve directly in front of the mouth by CO2 (Model 17515A, VacuMed, Ventura, CA, United States) and O2 (Model 17620, VacuMed) analyzers which pulled gas at a rate of 200 ml/min.
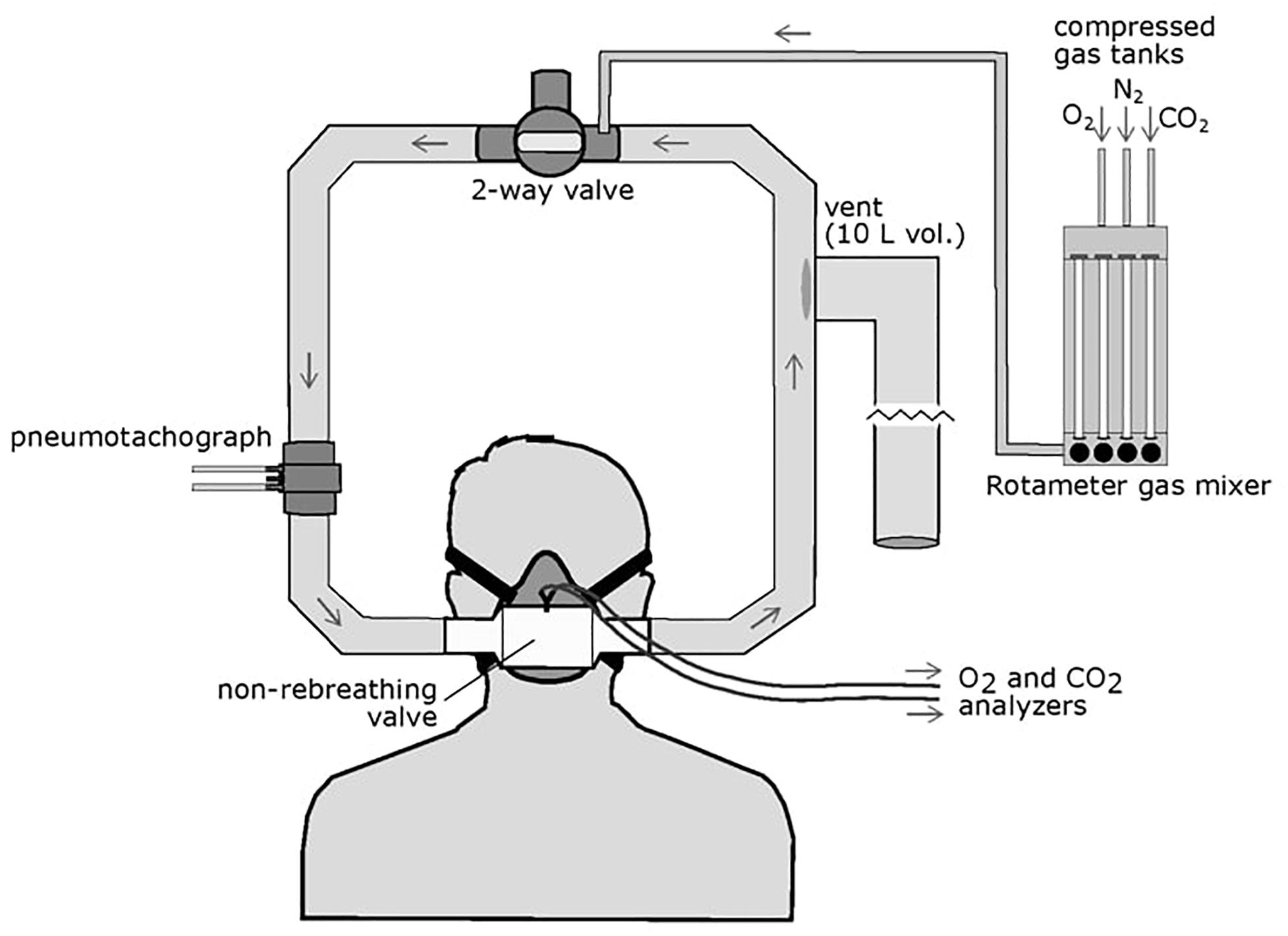
Figure 1. Schematic of the HVR experimental setup. The participant wore an oral-nasal mask attached to a non-rebreathing valve which allowed one-way airflow through the circuit. A two-way valve can be opened to allow entry or room air of closed to allow flow of mixed gases through the circuit. Gas mixtures are controlled via a rotameter attached to compressed O2, N2, and CO2 gas tanks and delivered to the breathing circuit. Inspired flow is measured by a pneumotachograph upstream of the mask. A large volume (10 L), low resistance vent is located downstream of the non-rebreathing valve to prevent pressure build up in the circuit but did not allow room air to enter the circuit.
Gas analyzers were calibrated daily using a compressed gas mixture with 4% CO2, 16% O2, and nitrogen balance (Airgas, Radnor, PA, United States). Inspiratory flow was measured by a Fleisch pneumotachograph upstream of the non-rebreathing valve and mask and connected to a carrier demodulator (model CD15, Validyne, Northridge, CA, United States). SpO2 and heart rate were measured by a pulse oximeter (Nellcor model N395, Medtronic, Minneapolis, MN, United States) with a surface probe placed on the forehead. All analog signals were processed through a PowerLab 8/30 (ADInstruments, Colorado Springs, CO, United States) and sent digitally to a laptop computer (HP Probook, HP Inc., Palo Alto, CA, United States). Raw data was recorded in LabChart 8 (ADInstruments, Colorado Springs, CO, United States).
Gas mixtures were manually controlled with a three-channel rotameter flow meter (Matheson Gas Products, Montgomeryville, PA, United States) that delivered mixtures upstream of the mask at flow rates sufficient to prevent rebreathing. Participants breathed ambient air for 5 min followed by 10 min of mild hyperoxia (simulating 30–40% FIO2 at sea level). This hyperoxic phase was intended to reverse hypoxic ventilatory decline in these participants due to continuous hypoxemia at high-altitude (Pamenter and Powell, 2016). To determine the HVR, participants then breathed a normoxic gas mixture (159 mmHg PO2, simulating 21% FIO2 at sea-level and increasing SpO2 above resting levels during room air breathing) for 5 min followed by 5 min of hypoxia during which we targeted 3 min of stable SpO2 between 80 and 85%. While this SpO2 level is near the resting value for this group, to determine the HVR, ventilation at this treatment level was compared to the “sea-level” experimental condition at which saturation levels were 97.3 ± 2.4% across all subjects. Isocapnia was achieved throughout the hypoxic phase by manually adding CO2 to maintain the average PETCO2 value from the last 1 min of the normoxic period. To determine ventilatory responses to CO2 (hypercapnic ventilatory response, HCVR) and a combined hypoxic and hypercapnic stimulus (hypercapnic HVR), participants then breathed normoxic air (159 mmHg PO2) with PETCO2 5 mmHg higher than their previous isocapnic value for 5 min. This was followed by 5 min of isocapnic hypoxia in which SpO2 was stable at a value between 80 and 85%. Target PETCO2 values were maintained within 1 mmHg of the target isocapnic value.
The HVR and hypercapnic HVR were calculated as the change in ventilation per decrease in SpO2. HCVR was calculated as the change in ventilation per mmHg increase in PETCO2. The HRR to hypoxia was calculated as the change in heart rate per decrease in SpO2 during the HVR treatment steps.
Sleep Studies
Forty-eight participants who completed ventilatory chemoreflex measurements also completed sleep studies. Three participants were excluded due to unacceptable oximetry recordings, leaving a final cohort of 45 participants (Table 2). Participants were instrumented each night with a limited channel polysomnogram (Respironics Alice PDx, Murrysville, PA, United States). This recording included nasal pressure, finger pulse oximetry, thoracic and abdominal effort bands, electro-oculogram, two channel electroencephalogram, and chin electromyogram. Each subject was also fitted with a WatchPAT device, consisting of fingertip peripheral arterial tonometry and pulse oximetry (Itamar Medical, Caesarea, Israel), which was used in the event of an Alice PDx device failure. Studies were scored by a registered polysomnographic sleep technologist using American Academy of Sleep Medicine criteria for scoring and Chicago criteria for events (Berry et al., 2012).
Statistical Analysis
We tested the hypotheses that (1) ventilatory chemosensitivity (HVR and HCVR), and sleep disordered breathing [apnea-hypopnea index (AHI), obstructive apnea index (OAI), and/or desaturation events] would predict Hct and (2) individuals with higher Hct or CMS scores would have lower ventilatory chemosensitivity and increased measures of sleep disordered breathing. All statistical analyses were conducted in R Studio (R Studio, Inc.). Univariate associations and multivariate models were conducted in men and women independently due to the large effect of sex on Hct. Multivariate models were screened for collinearity and predictors were removed if they had a variance inflation factor (VIF) greater than 4 (O’Brien, 2007). Models for daytime variables in men were Hct or CMS Score ∼Age + BMI + SpO2 + HVR + PETCO2. Models for nighttime variables in men were Hct or CMS Score ∼Age + BMI + SpO2 + AHI + OAI. Models for women were Hct or CMS Score ∼Age + Menopause + BMI + SpO2 + HVR + PETCO2 and Hct or CMS Score ∼Age + Menopause + BMI + SpO2 + AHI + OAI, respectively. All variables in multivariate models were treated as continuous except menopause status (pre or post). Daytime SpO2 is the resting saturation measured during room air breathing. Nighttime SpO2 predictors were nadir SpO2, mean SpO2, or time spent below 80% SpO2. In the event of collinearity, the best SpO2 predictor based on highest R2 (nadir SpO2, mean SpO2, or time spent below 80% SpO2) was used in the final models. Values are presented as mean ± standard deviation throughout the manuscript.
Results
Low Daytime Saturation Is Associated With Hypoventilation and CMS Symptoms
Low daytime SpO2 measurements were significantly correlated with increased Hct in both men and women (p < 0.001 and p < 0.002, respectively; Figure 2A) and with high CMS scores in men only (p < 0.001 and r2 = 0.12). HVR was not significantly associated with Hct nor CMS scores in men or women. Post-menopausal women had lower HVR (pre: 0.0009 ± 0.002 l/min/%SpO2/kg, post: 0.00006 ± 0.001 l/min/%SpO2/kg; p < 0.05) and lower resting SpO2 compared to their pre-menopausal counterparts (pre: 85.7 ± 4.5%, post: 82.2 ± 4.7%, p < 0.03). PETCO2, a measure of resting alveolar ventilation, correlated with both Hct and CMS score in men (p < 0.009 and p < 0.03, respectively) and showed a non-significant trend with Hct in women (p = 0.055; Figure 2B), although these relationships display substantial variation.
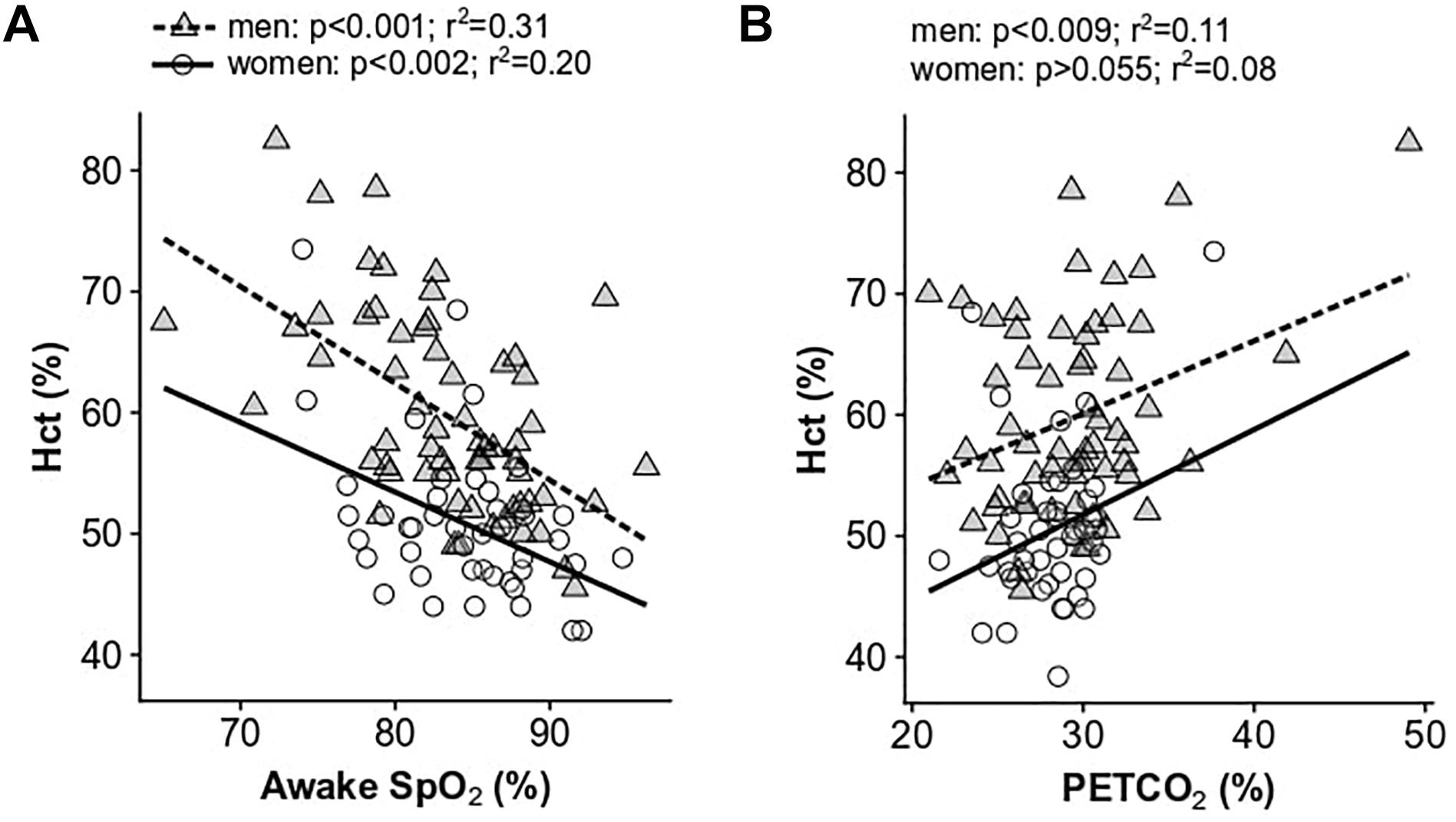
Figure 2. Univariate relationships between hematocrit and resting awake SpO2 (A) and resting end-tidal PCO2 (B). Data for women are shown as open circles and solid lines, data for men are shown as gray triangles and dashed lines.
In multivariate models, resting daytime SpO2 was significantly associated with Hct in men (p < 0.0001), predicting 34% of the variance. In women, resting daytime SpO2 (p < 0.01), age (p < 0.006), and menopause status (p < 0.001) were associated with Hct, with the model predicting 43% of the variance. Daytime SpO2 was the only significant predictor of CMS scores in men (p < 0.02; 24% of the variance explained) and women (p < 0.05; 26% of the variance explained). There was no collinearity in the model predictors for Hct or CMS score, and all VIFs were less than 2. Results from multivariate models are provided in Table 3.
Sleep Desaturation and Sleep-Disordered Breathing Are Associated With Hematocrit and CMS Symptoms
Men and women demonstrated substantially lower sleep mean and nadir SpO2 compared to awake values [Figure 3; F(2,84) = 190.5, p < 0.0001]: mean resting awake saturation (men: 84.6 ± 4.4; women: 85.2 ± 4.5%), mean sleep saturation (men: 80.3 ± 2.8; women: 80.3 ± 2.9%), and mean nadir sleep saturation (men: 71.0 ± 5.8; women: 71.5 ± 5.0%). Men and women with higher Hct had lower night-time mean and nadir SpO2 as well as greater time spent <80% SpO2 (Figures 4A–C). Participants with lower mean sleep SpO2 also had higher CMS scores (Figure 4E). No univariate association was detected between the AHI and Hct or CMS score. However, OAI was associated with Hct in men (Figure 4D), and there was a trend toward greater OAI and higher CMS score in men (p > 0.06).
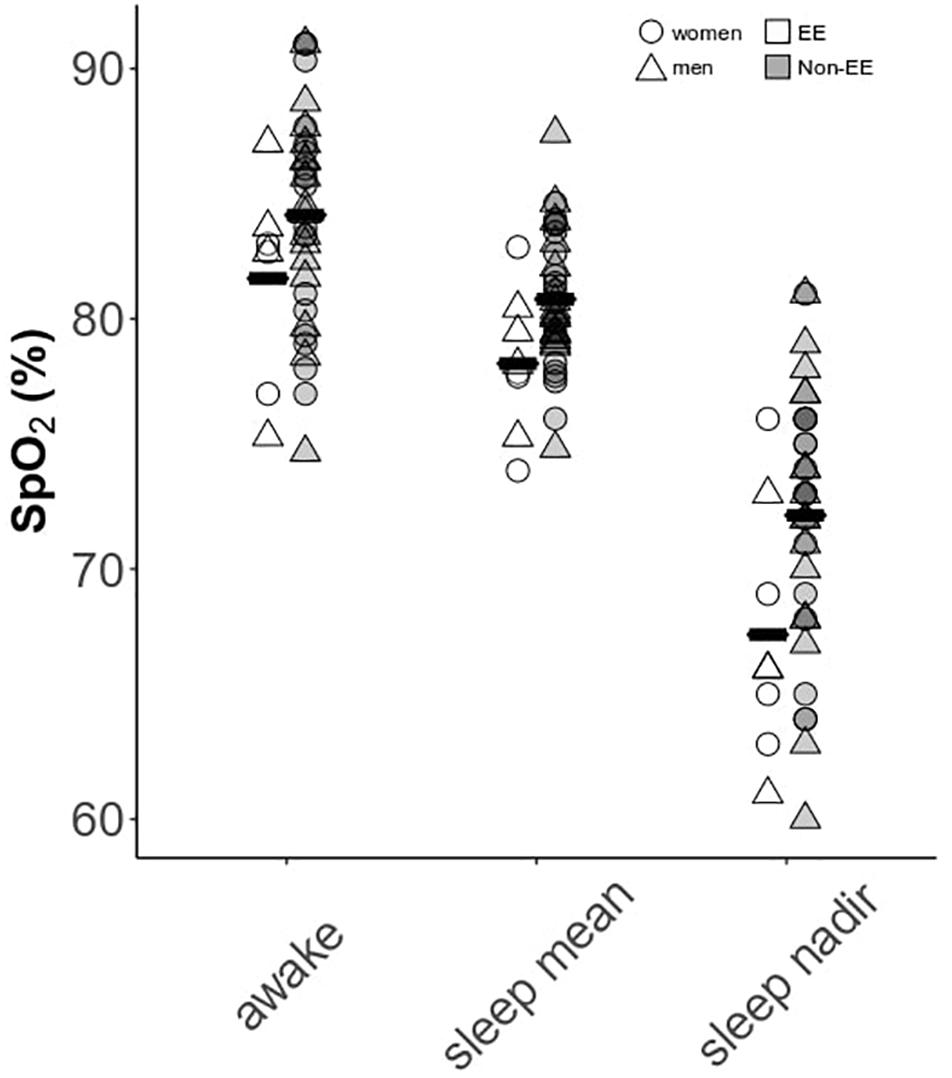
Figure 3. Awake versus sleep SpO2 parameters in men and women. Data are separated by the presence or absence of excessive erythrocytosis (EE). Means for each group are provided as solid black bars. N = 45 subjects who completed sleep studies.
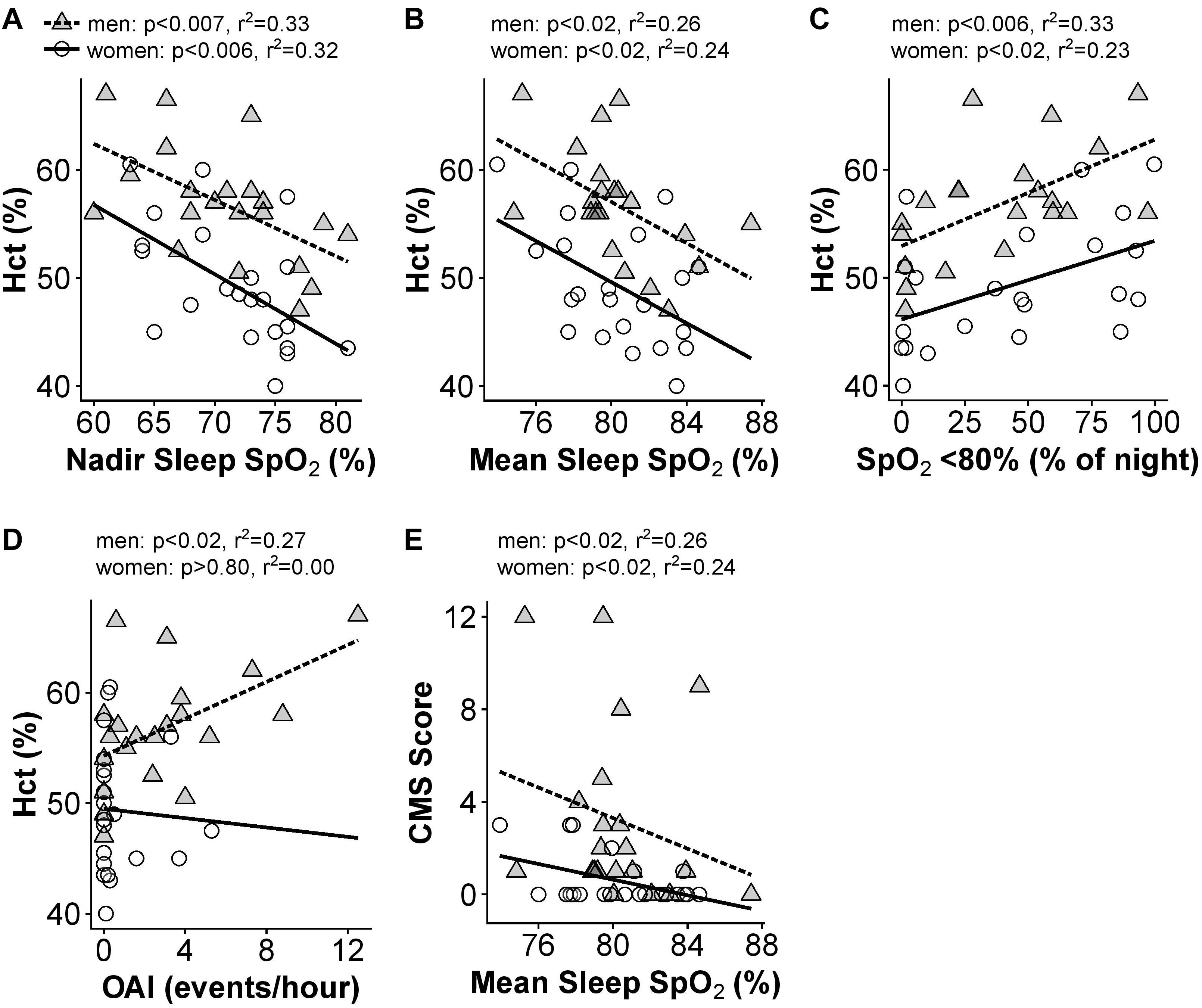
Figure 4. Significant univariate relationships between sleep variables and hematocrit (A–D) and CMS Score (E). Data for women are shown as open circles and solid lines, data for men are shown as gray triangles and dashed lines. N = 45 subjects who completed sleep studies.
In multivariate models, the three measures of night-time SpO2 (mean, nadir, and time below 80%) showed significant collinearity (VIF > 4). As a result, we tested which SpO2 measure provided the best predictive power in the Hct and CMS score models (Hct or CMS score ∼age + SpO2 + AHI + OAI). For Hct in men, nadir SpO2 and percent of the night below 80% SpO2 were similar (R2 = 0.58), while mean sleep SpO2 had slightly less predictive power (R2 = 0.53). The model including nadir sleep SpO2 gave the highest F value in both men [F(4,16) = 5.52, p < 0.01] and women [F(4,17) = 4.54, p < 0.05)], and nadir sleep O2 saturation was the only significant predictor of Hct in women (p < 0.02), while AHI and OAI were also significant predictors of Hct in men (p < 0.05 for both). For CMS Scores, mean sleep SpO2 gave the highest F value [F(4,16) = 3.4, p < 0.05, R2 = 0.46], and AHI and OAI also predicted CMS scores (p < 0.007 and p < 0.003, respectively) in men. The multivariate models did not produce any significant predictive power for CMS scores in women (p > 0.05 regardless of O2 saturation measure included). All VIFs in these multivariate models were 3.2 or lower. Table 4 provides results for multivariate models of sleep parameters.
Ventilatory Chemoreflexes Are Associated With Daytime and Sleep O2 Saturation
Our hypothesis that ventilatory chemoreflexes would predict Hct was not supported (Table 3). However, to determine if ventilatory chemoreflexes were associated with day or night-time SpO2, we examined the relationship of all SpO2 and sleep variables with the HVR, HCVR, and hypercapnic HVR in the sleep study cohort. Men with high HVRs maintained high mean daytime SpO2 (r2 = 0.39, p < 0.003; Figure 5A), and elevated hypercapnic HVR was associated with increased SpO2 in both men (r2 = 0.37, p < 0.01) and women (r2 = 0.35, p < 0.01; Figure 5B). The time spent below 80% SpO2 during sleep was significantly associated with the hypercapnic HVR (r2 = 0.25, p < 0.05) and HCVR (r2 = 0.21, p < 0.05) in men and showed a non-significant trend with HVR in women (r2 = 0.19, p < 0.06) (Figure 5C). In women, the nadir sleep O2 saturation was associated with the hypercapnic HVR as well (r2 = 0.31, p < 0.01; Figure 5D). No measures of ventilatory sensitivity were associated with the OAI or AHI.
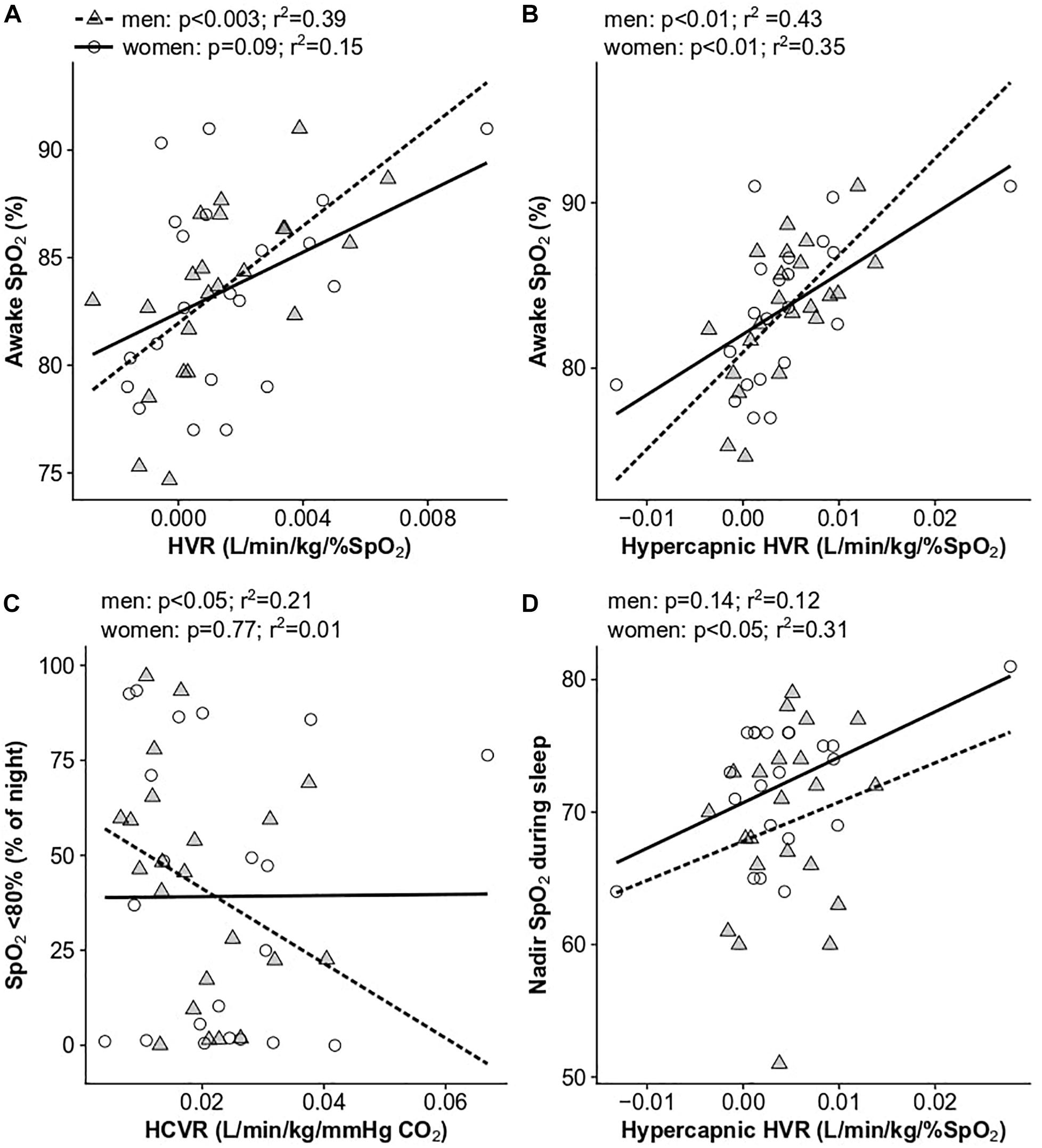
Figure 5. Significant univariate relationships between ventilatory chemoreflexes and SpO2 parameters: (A) HVR and awake SpO2, (B) hypercapnic HVR and awake SpO2, (C) HCVR and the percent of the night spent below 80% SpO2, and (D) hypercapnic HVR and nadir sleep SpO2. Data for women are shown as open circles and solid lines, data for men are shown as gray triangles and dashed lines. N = 45 subjects who completed sleep studies.
Men With Higher Hematocrit Have Lower Heart Rate Responses to Hypoxia
Men with lower Hct had larger increases in the HRR to acute hypoxia, while men with high Hct had very low HRR to hypoxia, or none (p < 0.001, r2 = 0.22; Figure 6A). A similar trend was observed in women (Figure 6), although the difference was not significant. These results were upheld when correcting for an interactive effect with age (men: p < 0.005; women: p < 0.5). The HRR to hypoxia decreased significantly with age in women (p < 0.008, r2 = 0.15) and was lower in post-menopausal women (post: 0.74 ± 0.45 beats/min/%SpO2, pre: 1.20 ± 0.70 beats/min/%SpO2; p < 0.01). The HRR to hypoxia was also associated with daytime SpO2 in both men and women (men: p < 0.01, r2 = 0.16, women: p < 0.0001, r2 = 0.30; Figure 6B). In contrast to the HRR to hypoxia, there was no relationship between the HRR to CO2 and Hct or SpO2 in men or women (p > 0.1 for all comparisons in men and women). Neither the HRR to hypoxia nor CO2 were associated with AHI, OAI, or sleep SpO2 measures in men or women before or after correcting for age.
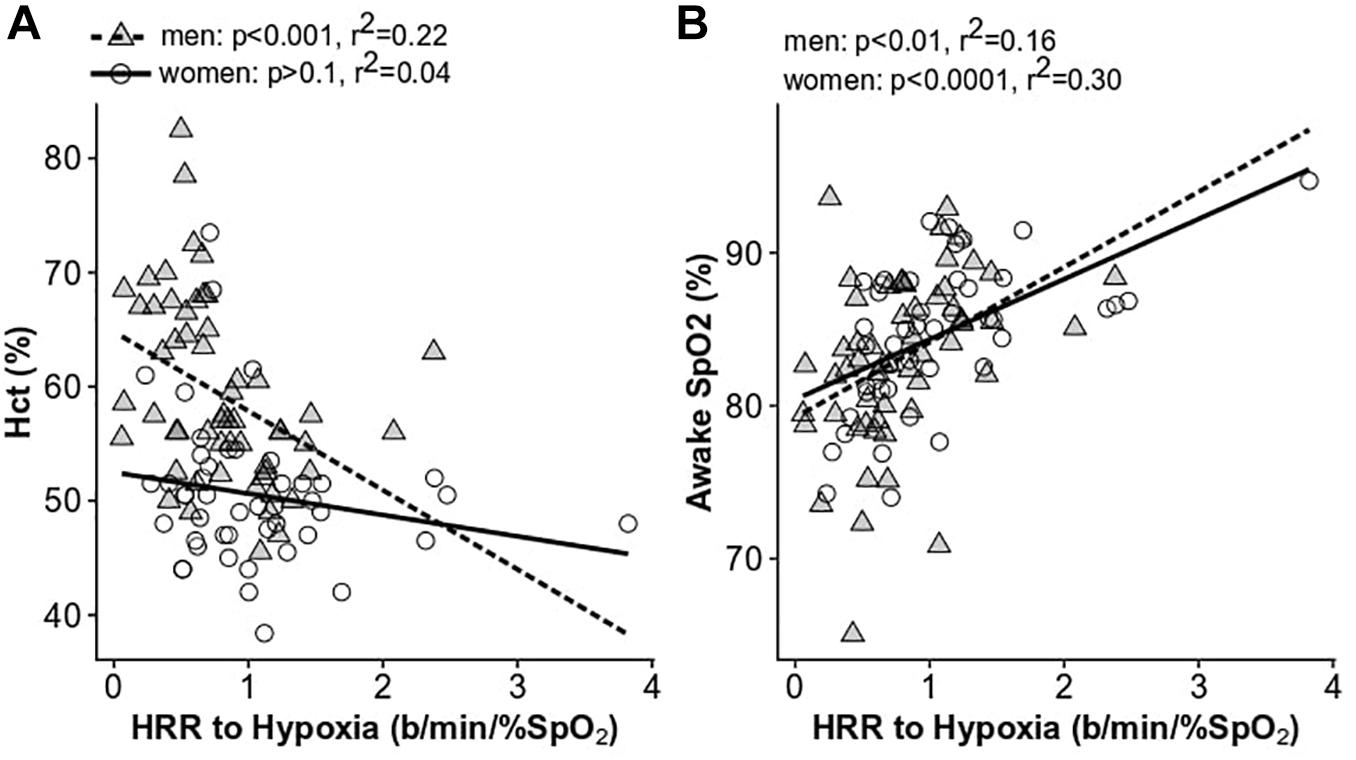
Figure 6. The relationship between the heart rate response to hypoxia and hematocrit (A) and daytime SpO2 (B). Women are shown as open circles and solid lines; men are shown as gray triangles and dashed lines.
Discussion
Ventilation
While HVR was not directly associated with Hct as we predicted, it was associated with daytime saturation in both men and women (Figures 5A,B), indicating blunted HVR may play a role in exacerbating hypoxemia and the development of excessive erythrocytosis. Furthermore, the increased PETCO2 in individuals with high Hct may indicate a decrease in baseline ventilatory drive, further exacerbating hypoxemia in this group. These results are in agreement with previous studies demonstrating higher PETCO2 values in high-altitude residents with CMS compared to those without CMS, but no differences, or very modest differences, across these groups in the acute HVR (Severinghaus et al., 1966; Lahiri et al., 1983; Fatemian et al., 2003).
In multivariate models, daytime SpO2 was the only significant predictor of Hct or CMS score in men. Since SpO2 is so strongly associated with Hct, it is not surprising that higher CMS scores are associated with lower SpO2 levels. In women, Hct was predicted by SpO2, age, and menopausal status (Table 3). CMS is more common after menopause (León-Velarde et al., 1997, 2001), which is consistent with the higher CMS scores reported by older women in this cohort and higher Hct values in older and post-menopausal women.
Sleep
All SpO2 measures taken during sleep were associated with Hct in men and women (Figures 4A–C), which supports previous findings regarding mean and time spent below 80% SpO2 in this population (Spicuzza et al., 2004; Villafuerte et al., 2016; Pham et al., 2017b). Mean sleep SpO2 was also associated with CMS score in men only (Figure 4E). The severe desaturation events during sleep may explain why Villafuerte et al. (2016) showed serum Epo during sleep was significantly higher in men with CMS, in contrast to findings of similar Epo levels in CMS and healthy male participants during the day (León-Velarde et al., 1991; Villafuerte et al., 2014; Hsieh et al., 2016). Villafuerte et al. (2016) also showed that mean sleep SpO2, total sleep time below 80% SpO2, and the Epo-to-soluble Epo receptor ratio (an Epo availability index) were significant predictors of Hct in men. Finally, Julian et al. (2013) found that men with excessive erythrocytosis had lower nocturnal SpO2 as well as higher levels of the oxidative stress marker 8-iso-PGF2alpha. The results presented here demonstrate that the relationships between sleep SpO2 and Hct previously identified in men are present in women as well.
AHI did not display a univariate association with Hct in men or women. This result supports the findings of Spicuzza et al. (2004) and Pham et al. (2017b) who report no difference in AHI across men with or without excessive erythrocytosis, but contrasts with Julian et al. (2013) who found higher AHI during REM sleep in men with excessive erythrocytosis. In contrast, OAI was positively associated with Hct in men (Figure 4D), which differs from the results reported by Spicuzza et al. (2004) who found only one excessive erythrocytosis participant who displayed obstructive apneas among the 10 CMS and 10 non-CMS participants examined; this difference may be attributed to differences in sample size or sampling method and the fact that the average age of our male participants was 10 years greater than that of Spicuzza et al. (2004). However, we also conducted multivariate models which controlled for age to determine the best predictors of Hct and CMS score. In these models, OAI and AHI were both predictors of Hct and CMS score in men but not women.
While we did not find that differences in chemosensitivity accounted for the presence or absence of sleep disordered breathing, control of breathing clearly influences factors such as respiratory event duration and arousal from sleep. Unlike Spicuzza et al. (2004), we did not see a significant relationship between the HCVR and AHI, but men with lower HCVR did spend more time below 80% SpO2 at night (Figure 5C). The hypercapnic HVR was associated with nadir SpO2 during sleep in women (Figure 5D), but not men, indicating sex-specific differences may underlie distinct outcomes that could be attributed to prolonged versus frequent desaturation events. All this considered, it is possible that low ventilatory drive contributes to larger reductions in nighttime SpO2 and exacerbates erythropoiesis and/or other commonly associated outcomes. Metrics to characterize patterns of hypoxemia (e.g., prolonged versus frequent desaturation events) (Azarbarzin et al., 2019) may be useful to better understand which individuals are at highest risk of excessive erythrocytosis and CMS.
Several studies have shown that intermittent hypoxia resulting from sleep apnea is associated with systemic hypertension, coronary artery disease, heart failure, and metabolic dysfunction (Jean-Louis et al., 2008; Badran et al., 2014; Dewan et al., 2015; Floras, 2015; Torres et al., 2015; Ryan, 2017, 2018). Therefore, patterns of hypoxia during sleep at high altitude likely contribute to the poor cardiometabolic outcomes observed in Andeans (Pham et al., 2017b). Efforts to assess further the molecular effects of intermittent hypoxemia during sleep, on top of continuous chronic hypoxia in this population (Prabhakar and Semenza, 2012), will provide important clues into the development of CMS and related cardiometabolic pathologies. Longitudinal studies that examine the progression of excessive erythrocytosis and CMS are needed to address better the cascade of events underlying these outcomes in this population.
Heart Rate Response to Hypoxia
We also found that Hct was associated with the HRR to hypoxia in men (Figure 6). Men with excessive erythrocytosis have a lower HRR to acute hypoxia after correcting for age (p < 0.05) (Kronenberg and Drage, 1973). This provides further evidence of blunted chemoreceptor sensitivity or autonomic outflow in this group. Previous work has also demonstrated a lower HRR to orthostasis in CMS patients compared to non-CMS high-altitude residents despite exceptional orthostatic tolerance in both groups (Claydon et al., 2004a). CMS patients also have lower reflex vasoconstriction and impaired cerebral blood flow autoregulation at sea level compared to non-CMS highlanders (Claydon et al., 2004b). It was suggested that these responses may result from a higher “set point” of the carotid baroreceptor-vascular resistance reflex (Moore et al., 2006). Lower carotid chemoreceptor and baroreceptor function may be a compensatory response to chronically elevated sympathetic activity resulting from chronic hypoxemia. It may also be the typical response to chronic hypoxia exposure as previous evidence suggests blunted hypoxic ventilatory chemosensitivity in sojourners as a function of time spent at high-altitude (Zhuang et al., 1993). In either case, loss of chemoreceptor function puts individuals with excessive erythrocytosis at higher risk of severe desaturation during exertion or apneic periods during sleep.
Conclusion
We demonstrate that lower SpO2 indices during sleep and during the day are associated with higher Hct in Andean men and, for the first time, in women. When controlling for age and SpO2, OAI and AHI also predicted Hct and CMS scores in men. While the HVR is blunted in Andeans with and without excessive erythrocytosis, lower hypoxic chemosensitivity was associated with lower daytime SpO2 and may therefore play a role in excessive erythrocytosis development. While these results support previous work in this population, we are the first to provide paired sleep and ventilatory chemoreflex measurements in the same Andean men and women, and the first to show that the HRR to hypoxia is also blunted in excessive erythrocytosis in men. A limitation of this study is that we cannot determine if more severe hypoxemia, or other phenotypes associated with Hct, are a cause or effect of excessive erythrocytosis. Interventional and longitudinal studies are required to determine the causal relationships between these associations.
Data Availability Statement
The raw data supporting the conclusions of this article will be made available by the authors, without undue reservation, to any qualified researcher.
Ethics Statement
This study was conducted in accordance with the Declaration of Helsinki, except for registration in a database, and was approved by the University of California, San Diego Human Research Protection Program. Participants provided written consent in their native language (Spanish).
Author Contributions
TS and FV designed and oversaw the study. EH, JO, DG, CA-R, PD, MD, NC, GV-G, JM, EG, and FP participated in participant screening and data collection. Sleep scoring was performed by PD and data analyses were performed by EH, AM, and JO. EH, JO, TS, and FV produced the original draft and all authors contributed to the final manuscript.
Funding
Funding for this work was provided by a Wellcome Trust grant 107544/Z/15/Z to FV, R01HL145470 and American Physiological Society Giles F. Filley Memorial Award to TS, NIH R01HL081823 to FP, and the UC, San Diego Center for Physiological Genomics of Low Oxygen (CPLGO). AM is supported by NIH R01HL085188, K24HL132105, and T32HL134632 and co-investigator on R21HL121794, R01HL119201, and R01HL081823. JO is supported by NIH F32HL131306 and the ATS Foundation. EH is supported by NIH F32HL131218. ResMed Inc. provided a philanthropic donation to UC San Diego in support of a sleep center but had no role in the design or conduct of this research.
Conflict of Interest
The authors declare that the research was conducted in the absence of any commercial or financial relationships that could be construed as a potential conflict of interest.
Acknowledgments
The authors thank all of the study participants as well as the staff at the Instituto de Investigaciones de la Altura laboratory for their support.
References
Azarbarzin, A., Sands, S. A., Stone, K. L., Taranto-Montemurro, L., Messineo, L., Terrill, P. I., et al. (2019). The hypoxic burden of sleep apnoea predicts cardiovascular disease-related mortality: the osteoporotic fractures in men study and the sleep heart health study. Eur. Heart J. 40, 1149a–1157a. doi: 10.1093/eurheartj/ehy624
Badran, M., Ayas, N., and Laher, I. (2014). Cardiovascular complications of sleep apnea: role of oxidative stress. Oxid. Med. Cell. Longev. 2014:985258. doi: 10.1155/2014/985258
Basaran, K. E., Villongco, M., Ho, B., Ellis, E., Zarndt, R., Antonova, J., et al. (2016). Ibuprofen blunts ventilatory acclimatization to sustained hypoxia in humans. PLoS ONE 11:e0146087. doi: 10.1371/journal.pone.0146087
Beall, C. M., Brittenham, G. M., Strohl, K. P., Blangero, J., Williams-Blangero, S., Goldstein, M. C., et al. (1998). Hemoglobin concentration of high-altitude tibetans and bolivian aymara. Am. J. Phys. Anthropol. 106, 385–400. doi: 10.1002/(SICI)1096-8644(199807)106:3<385::AID-AJPA10>3.0.CO;2-X
Beall, C. M., Strohl, K. P., Blangero, J., Williams-Blangero, S., Almasy, L. A., Decker, M. J., et al. (1997). Ventilation and hypoxic ventilatory response of Tibetan and Aymara high altitude natives. Am. J. Phys. Anthro 104, 427–447. doi: 10.1002/(SICI)1096-8644(199712)104:4<427::AID-AJPA1>3.0.CO;2-P
Berry, R. B., Brooks, R., Gamaldo, C. E., Harding, S. M., Marcus, C., and Vaughn, B. V. (2012). The AASM Manual for the Scoring of Sleep and Associated Events: Rules, Terminology and Technical Specifications. Illinois, Ill.: American Academy of Sleep Medicine, 176.
Claydon, V. E., Norcliffe, L. J., Moore, J. P., Rivera-Ch, M., Leon-Velarde, F., Appenzeller, O., et al. (2004a). Orthostatic tolerance and blood volumes in Andean high altitude dwellers. Exp. Physiol. 89, 565–571. doi: 10.1113/expphysiol.2004.027698
Claydon, V. E., Norcliffe, L. J., Moore, J. P., Rivera, M., Leon-Velarde, F., Appenzeller, O., et al. (2004b). Cardiovascular responses to orthostatic stress in healthy altitude dwellers, and altitude residents with chronic mountain sickness. Exp. Physiol. 90, 103–110. doi: 10.1113/expphysiol.2004.028399
Corante, N., Anza-Ramírez, C., Figueroa-Mujíca, R., Macarlupú, J. L., Vizcardo-Galindo, G., Bilo, G., et al. (2018). Excessive erythrocytosis and cardiovascular risk in andean highlanders. High Alt. Med. Biol. 19, 221–231. doi: 10.1089/ham.2017.0123
De Ferrari, A., Miranda, J. J., Gilman, R. H., Dávila-Román, V. G., León-Velarde, F., Rivera-Ch, M., et al. (2014). Prevalence, clinical profile, iron status, and subject-specific traits for excessive erythrocytosis in andean adults living permanently at 3,825 meters above sea level. Chest 146, 1327–1336. doi: 10.1378/chest.14-0298
Dewan, N. A., Nieto, F. J., and Somers, V. K. (2015). Intermittent hypoxemia and OSA: implications for comorbidities. Chest 147, 266–274. doi: 10.1378/chest.14-0500
Easton, P., Slykerman, L. J., and Anthonisen, N. R. (1988). Recovery of the ventilatory response to hypoxia in normal adults. J. Appl. Physiol. 64, 521–528. doi: 10.1152/jappl.1988.64.2.521
Fatemian, M., Gamboa, A., Leon-Velarde, F., Rivera-ch, M., Palacios, J.-A., and Robbins, P. A. (2003). Ventilatory response to CO2 in high-altitude natives and patients with chronic mountain sickness. J. Appl. Physiol. 94, 1279–1287. doi: 10.1152/japplphysiol.00859.2002
Floras, J. S. (2015). Hypertension and sleep apnea. Can. J. Cardiol. 31, 889–897. doi: 10.1016/j.cjca.2015.05.003
Goldenberg, F., Richalet, J. P., Onnen, I., and Antezana, A. M. (1992). Sleep apneas and high altitude newcomers. Int. J. Sports Med. 13, S34–S36. doi: 10.1055/s-2007-1024586
Hsieh, M. M., Callacondo, D., Rojas-Camayo, J., Quesada-Olarte, J., Wang, X., Uchida, N., et al. (2016). SENP1, but not fetal hemoglobin, differentiates andean highlanders with chronic mountain sickness from healthy individuals among andean highlanders. Exp. Hematol. 44, 483–490.e2. doi: 10.1016/j.exphem.2016.02.010
Hupperets, M. D. W., Hopkins, S. R., Pronk, M. G., Tiemessen, I. J. H., Garcia, N., Wagner, P. D., et al. (2004). Increased hypoxic ventilatory response during 8 weeks at 3800 m altitude. Respir. Physiol. Neurobiol. 142, 145–152. doi: 10.1016/j.resp.2004.06.011
Jean-Louis, G., Zizi, F., Clark, L. T., Brown, C. D., and McFarlane, S. I. (2008). Obstructive sleep apnea and cardiovascular disease: role of the metabolic syndrome and its components. J. Clin. Sleep Med. 4, 261–272.
Julian, C. G., Vargas, E., Gonzales, M., Dávila, R. D., Ladenburger, A., Reardon, L., et al. (2013). Sleep-disordered breathing and oxidative stress in preclinical chronic mountain sickness (excessive erythrocytosis). Respir. Physiol. Neurobiol. 186, 188–196. doi: 10.1016/j.resp.2013.01.016
Kronenberg, R. S., and Drage, C. W. (1973). Attenuation of the ventilatory and heart rate responses to hypoxia and hypercapnia with aging in normal men. J. Clin. Invest. 52, 1812–1819. doi: 10.1172/JCI107363
Küpper, T., Schöffl, V., and Netzer, N. (2008). Cheyne stokes breathing at high altitude: a helpful response or a troublemaker? Sleep Breath. 12, 123–127. doi: 10.1007/s11325-007-0155-5
Lahiri, S., Maret, K., and Sherpam, M. G. (1983). Dependence of high altitude sleep apnea on ventilatory sensitivity to hypoxia. Repir. Physiol. 52, 281–301. doi: 10.1016/0034-5687(83)90086-5
Leon-Velarde, F., Gamboa, A., Rivera-ch, M., Palacios, J., and Robbins, P. A. (2003). Peripheral chemoreflex function in high-altitude natives and patients with chronic mountain sickness. J. Appl. Physiol. 94, 1269–1278. doi: 10.1152/japplphysiol.00858.2002
León-Velarde, F., Maggiorini, M., Reeves, J. T., Aldashev, A., Asmus, I., Bernardi, L., et al. (2005). Consensus statement on chronic and subacute high altitude diseases. High Alt. Med. Biol. 6, 147–157. doi: 10.1089/ham.2005.6.147
León-Velarde, F., Monge, C. C., Vidal, A., Carcagno, M., Criscuolo, M., and Bozzini, C. E. (1991). Serum immunoreactive erythropoietin in high altitude natives with and without excessive erythrocytosis. Exp. Hematol. 19, 257–260.
León-Velarde, F., Ramos, M. A., Hernández, J. A., De Idiáquez, D., Muñoz, L. S., Gaffo, A., et al. (1997). The role of menopause in the development of chronic mountain sickness. Am. J. Physiol. – Regul. Integr. Comp. Physiol. 272, R90–R94. doi: 10.1152/ajpregu.1997.272.1.r90
León-Velarde, F., Rivera-Chira, M., Tapia, R., Huicho, L., and Monge-C, C. (2001). Relationship of ovarian hormones to hypoxemia in women residents of 4,300 m. Am. J. Physiol. – Regul. Integr. Comp. Physiol. 280, 488–493. doi: 10.1152/ajpregu.2001.280.2.r488
Masuyama, S., Kohchiyama, S., Shinozaki, T., Okita, S., Kunitomo, F., Tojima, H., et al. (1989). Periodic breathing at high altitude and ventilatory responses to O2 and CO2. Jpn. J. Physiol. 39, 559–570. doi: 10.2170/jjphysiol.39.523
Monge, C., Arregui, A., and León-Velarde, F. (1992). Pathophysiology and epidemiology of chronic mountain sickness. Int. J. Sports Med. 13, S79–S81. doi: 10.1055/s-2007-1024603
Monge, C. C., Leon-Velarde, F., and Arrehui, A. (1989). Increasing prevalence of excessive erythrocytosis with age among healthy high-altitude miners. N. Engl. J. Med. 321:1271. doi: 10.1056/NEJM199311113292002
Moore, J. P., Claydon, V. E., Norcliffe, L. J., Rivera-Ch, M. C., Lèon-Velarde, F., Appenzeller, O., et al. (2006). Carotid baroreflex regulation of vascular resistance in high-altitude Andean natives with and without chronic mountain sickness. Exp. Physiol. 91, 907–913. doi: 10.1113/expphysiol.2005.033084
O’Brien, R. M. (2007). A caution regarding rules of thumb for variance inflation factors. Qual. Quant. 41, 673–690. doi: 10.1007/s11135-006-9018-6
Pamenter, M. E., and Powell, F. L. (2016). Time domains of the hypoxic ventilatory response and their molecular basis. Compr. Physiol. 6, 1345–1385. doi: 10.1002/cphy.c150026
Penaloza, D., and Arias-Stella, J. (2007). The heart and pulmonary circulation at high altitudes. Circulation 115, 1132–1146. doi: 10.1161/circulationaha.106.624544
Penaloza, D., Sime, F., and Ruiz, L. (1971). “Corpulmonale in chronic mountain sickness: present concept of Monge’s disease,” in High Altitude Physiology: Cardiac and Respiratory Aspects, eds R. Porter and J. Knight (Edinburgh: Churchill Livingstone), 41–60.
Pham, L. V., Meinzen, C., Arias, R. S., Schwartz, N. G., Rattner, A., Miele, C. H., et al. (2017a). Cross-Sectional comparison of sleep-disordered breathing in native peruvian highlanders and lowlanders. High Alt. Med. Biol. 18, 11–19. doi: 10.1089/ham.2016.0102
Pham, L. V., Miele, C. H., Schwartz, N. G., Arias, R. S., Rattner, A., Gilman, R. H., et al. (2017b). Cardiometabolic correlates of sleep disordered breathing in andean highlanders. Eur. Respir. J. 49:1601705. doi: 10.1016/S2214-109X(16)30265-0.Cost-effectiveness
Prabhakar, N. R., and Semenza, G. L. (2012). Adaptive and maladaptive cardiorespiratory responses to continuous and intermittent hypoxia mediated by hypoxia-inducible factors 1 and 2. Physiol. Rev. 92, 967–1003. doi: 10.1152/physrev.00030.2011
Robbins, P. A. (2007). Role of the peripheral chemoreflex in the early stages of ventilatory acclimatization to altitude. Respir. Physiol. Neurobiol. 158, 237–242. doi: 10.1016/j.resp.2007.03.008
Ryan, S. (2017). Adipose tissue inflammation by intermittent hypoxia: mechanistic link between obstructive sleep apnoea and metabolic dysfunction. J. Physiol. 595, 2423–2430. doi: 10.1113/JP273312
Ryan, S. (2018). Mechanisms of cardiovascular disease in obstructive sleep apnoea. J. Thorac. Dis. 10, S4201–S4211. doi: 10.21037/jtd.2018.08.56
Sato, M., Severinghaus, J. W., and Bickler, P. (1994). Time course of augmentation and depression ventilatory responses at altitude of hypoxic ventilatory responses at altitude. J. Appl. Physiol. 77, 313–316. doi: 10.1152/jappl.1994.77.1.313
Sato, M., Severinghaus, J. W., Powell, F. L., Xu, F. D., and Spellman, M. J. (1992). Augmented hypoxic ventilatory response in men at altitude. J. Appl. Physiol. 73, 101–107. doi: 10.1152/jappl.1992.73.1.101
Severinghaus, J. W., Bainton, C. R., and Carcelen, A. (1966). Respiratory insensitivity to hypoxia in chronically hypoxic man. Respir. Physiol. 1, 308–334. doi: 10.1016/0034-5687(66)90049-1
Spicuzza, L., Casiraghi, N., Gamboa, A., Keyl, C., Schneider, A., Mori, A., et al. (2004). Sleep-related hypoxaemia and excessive erythrocytosis in Andean high-altitude natives. Eur. Respir. J. 23, 41–46. doi: 10.1183/09031936.03.00000703
Torres, G., Sánchez-De-La-Torre, M., and Barbé, F. (2015). Relationship between OSA and hypertension. Chest 148, 824–832. doi: 10.1378/chest.15-0136
Villafuerte, F., Corante, N., Anza-Ramírez, C., and Figueroa-Mujica, R. (2016). Plasma soluble erythropoietin receptor is decreased during sleep in Andean highlanders with chronic mountain sickness. J. Appl. Physiol. 121, 53–58. doi: 10.1152/japplphysiol.00107.2016
Villafuerte, F. C., and Corante, N. (2016). Chronic mountain sickness: clinical aspects, etiology, management, and treatment. High Alt. Med. Biol. 17, 61–69. doi: 10.1089/ham.2016.0031
Villafuerte, F. C., Macarlupu, J. L., Anza-Ramirez, C., Corrales-Melgar, D., Vizcardo-Galindo, G., Corante, N., et al. (2014). Decreased plasma soluble erythropoietin receptor in high-altitude excessive erythrocytosis and Chronic Mountain Sickness. J. Appl. Physiol. 117, 1356–1362. doi: 10.1152/japplphysiol.00619.2014
Wu, T., Li, S., and Ward, M. P. (2005). Tibetans at extreme altitude. Wilderness Environ. Med. 16, 47–54. doi: 10.1580/PR04-04.1
Wu, T. Y., Chen, Q. H., Li, W. S., Wei, C. Y., Li, Y., Wang, X. Z., et al. (1997). The study of diagnostic criteria of chronic mountain sickness [in Chinese]. Chin. J. High Alt. Med. 7, 1–6.
Wu, T.-Y., Chen, Q. H., Li, Y., Wei, C. Y., Ren, Z. H., Li, W. S., et al. (1998). Pathophysiology of high altitude excessive polycythemia: I. A study of optimal hematocrit [in Chinese]. Chin. J. High Alt. Med. 8, 1–4.
Keywords: hypoxia, sleep, control of breathing, excessive erythrocytosis, high altitude
Citation: Heinrich EC, Orr JE, Gilbertson D, Anza-Ramirez C, DeYoung PN, Djokic MA, Corante N, Vizcardo-Galindo G, Macarlupu JL, Gaio E, Powell FL, Malhotra A, Villafuerte FC and Simonson TS (2020) Relationships Between Chemoreflex Responses, Sleep Quality, and Hematocrit in Andean Men and Women. Front. Physiol. 11:437. doi: 10.3389/fphys.2020.00437
Received: 12 February 2020; Accepted: 08 April 2020;
Published: 06 May 2020.
Edited by:
Ovidiu Constantin Baltatu, Khalifa University, United Arab EmiratesReviewed by:
Robert Naeije, Université libre de Bruxelles, BelgiumMelissa L. Bates, University of Iowa, United States
Martin Burtscher, University of Innsbruck, Austria
Copyright © 2020 Heinrich, Orr, Gilbertson, Anza-Ramirez, DeYoung, Djokic, Corante, Vizcardo-Galindo, Macarlupu, Gaio, Powell, Malhotra, Villafuerte and Simonson. This is an open-access article distributed under the terms of the Creative Commons Attribution License (CC BY). The use, distribution or reproduction in other forums is permitted, provided the original author(s) and the copyright owner(s) are credited and that the original publication in this journal is cited, in accordance with accepted academic practice. No use, distribution or reproduction is permitted which does not comply with these terms.
*Correspondence: Francisco C. Villafuerte, ZnJhbmNpc2NvLnZpbGxhZnVlcnRlQHVwY2gucGU=; Tatum S. Simonson, dHNpbW9uc29uQGhlYWx0aC51Y3NkLmVkdQ==
†Present address: Erica C. Heinrich, Division of Biomedical Sciences, School of Medicine, University of California, Riverside, Riverside, CA, United States
‡These authors have contributed equally to this work and share senior authorship