- 1Center for Reproductive Medicine, Ren Ji Hospital, School of Medicine, Shanghai Jiao Tong University, Shanghai, China
- 2Shanghai Key Laboratory for Assisted Reproduction and Reproductive Genetics, Shanghai, China
- 3School of Life Sciences, Yunnan University, Kunming, China
The fetal membranes are equipped with high capacity of cortisol regeneration through the reductase activity of 11β-hydroxysteroid dehydrogenase 1 (11β-HSD1). The expression of 11β-HSD1 in the fetal membranes is under the feedforward induction by cortisol, which is potentiated by proinflammatory cytokines. As a result, the abundance of 11β-HSD1 increases with gestational age and furthermore at parturition with an escalation of cortisol concentration in the fetal membranes. Accumulated cortisol takes parts in a number of crucial events pertinent to the onset of labor in the fetal membranes, including extracellular matrix (ECM) remodeling and stimulation of prostaglandin output. Cortisol remodels the ECM through multiple approaches including induction of collagen I, III, and IV degradation, as well as inhibition of their cross-linking. These effects of cortisol are executed through activation of the autophagy, proteasome, and matrix metalloprotease 7 pathways, as well as inhibition of the expression of cross-linking enzyme lysyl oxidase in mesenchymal cells of the membranes. With regard to prostaglandin output, cortisol not only increases prostaglandin E2 and F2α syntheses through induction of their synthesizing enzymes such as cytosolic phospholipase A2, cyclooxygenase 2, and carbonyl reductase 1 in the amnion, but also decreases their degradation through inhibition of their metabolizing enzyme 15-hydroxyprostaglandin dehydrogenase in the chorion. Taking all together, data accumulated so far denote that the feedforward cortisol regeneration by 11β-HSD1 in the fetal membranes is a requisite event in the onset of parturition, and the effects of cortisol on prostaglandin synthesis and ECM remodeling may be enhanced by proinflammatory cytokines in chorioamnionitis.
Introduction
Glucocorticoids are essential for life, and it regulates a variety of important cardiovascular, metabolic, and immunologic functions in the maintenance of homeostasis (Schmid et al., 1995; Beato and Klug, 2000; Rhen and Cidlowski, 2005). Cortisol is the most important endogenous glucocorticoid in humans. The de novo synthesis of cortisol from cholesterol takes place primarily in the zona fasciculata of the adrenal cortex (Miller and Auchus, 2011). After secretion into the circulation, most of cortisol is bound by corticosteroid-binding protein (CBG) and to a lesser extent by albumin (Bae and Kratzsch, 2015; Meyer et al., 2016). There is approximately only 5 to 10% of cortisol that remains free in the circulation, which is important for the actions of cortisol as only the free fraction of cortisol is biologically active (Lewis et al., 2005). In compensation, glucocorticoid target organs develop a way to enhance cortisol concentrations within the cells through regeneration of cortisol by 11β-hydroxysteroid dehydrogenase 1 (11β-HSD1) (Chapman et al., 1997; Tomlinson et al., 2004; Chapman et al., 2013; Morgan et al., 2014). 11β-HSD1 is a microsomal reductase catalyzing the regeneration of cortisol from biologically inactive 17α-hydroxy-11-dehydrocorticosterone (cortisone), which derives mostly from the oxidase action of 11β-HSD2 in the mineralocorticoid target organs (Figure 1; Tannin et al., 1991; Albiston et al., 1994; Chapman et al., 2013). 11β-HSD2 is a counterpart enzyme of 11β-HSD1 and functions in an opposite way to 11β-HSD1 converting biologically active cortisol to inactive cortisone (Figure 1). Because 11β-HSD2 does not metabolize aldosterone, 11β-HSD2 is utilized by the mineralocorticoid target organs as a pre-receptor gate to ensure the indiscriminating mineralocorticoid receptor being occupied only by aldosterone but not by cortisol (White et al., 1997a,b,c). This differential expression pattern of 11β-HSD1 and 11β-HSD2 in glucocorticoid and mineralocorticoid target organs is developed perfectly to ensure the efficiency of cortisol’s actions and the specificity of aldosterone’s actions in their respective target organs.
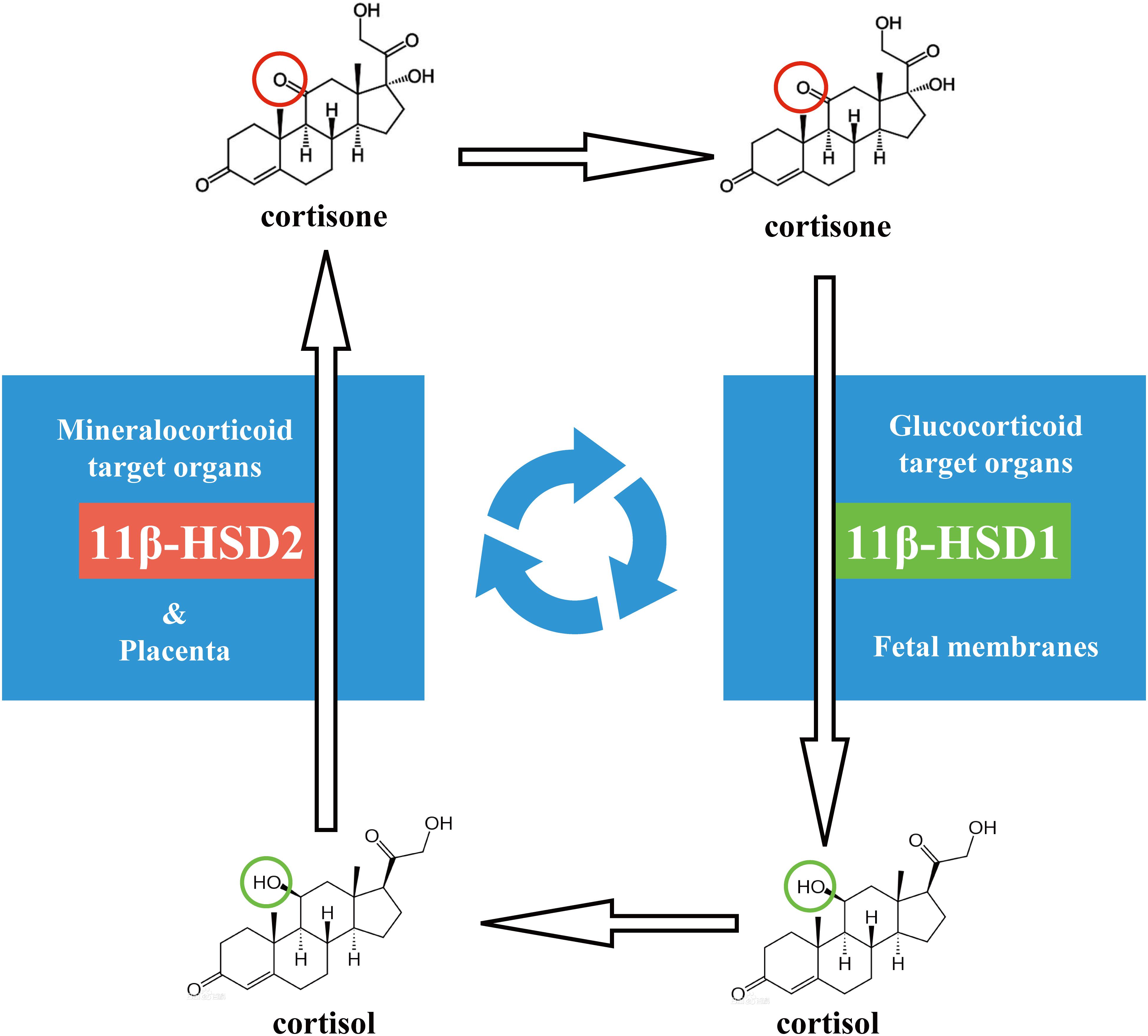
Figure 1. Recycle of cortisol and cortisone between placenta and fetal membranes in human pregnancy.
In pregnancy, the placenta is responsible for nourishing and protecting the fetus as well as maintaining pregnancy by producing a plethora of hormones and immune factors. Attached to the edge of the discoid placenta is the atrophied chorionic villi, also known as the smooth chorion or chorion leave, which fuses with the amniotic membrane extended from the fetal surface of the placenta, and together they form the reflected fetal membranes (Leiser and Kaufmann, 1994; Ferner and Mess, 2011). The fetal membranes not only enclose the fetus bathed in the amniotic fluid but also become a source of initiating signals for parturition toward the end of gestation (Okazaki et al., 1981; Myatt and Sun, 2010; Menon, 2016; Wang et al., 2018; Menon and Moore, 2020). Like the specific distribution of 11β-HSD1 and 11β-HSD2 in glucocorticoid and mineralocorticoid target organs, the distribution of 11β-HSD1 and 11β-HSD2 in the placenta and fetal membranes also adopts a unique tissue-specific pattern (Sun et al., 1997; Yang et al., 2016). Although the placenta is not a typical mineralocorticoid target organ, it boasts abundant 11β-HSD2 but scarce 11β-HSD1 (Albiston et al., 1994; Sun et al., 1997; Yang et al., 2016). It is known that 11β-HSD2 in the placenta functions as a glucocorticoid barrier by inactivating maternal cortisol to cortisone so that the fetus can be protected from the growth-restricting effects of excessive maternal glucocorticoids (Osinski, 1960; Burton and Waddell, 1999; Drake et al., 2007). This function of 11β-HSD2 in the placenta is substantiated by its distinct distribution in the syncytiotrophoblast, the outmost layer of placental villi that immerse directly in the maternal blood (Krozowski et al., 1995; Ni et al., 2009; Li et al., 2011, 2013; Zhang et al., 2015; Zuo et al., 2017). In contrast to the placenta, the fetal membranes express abundant 11β-HSD1 with barely detectable 11β-HSD2 (Sun et al., 1997), which can utilize cortisone derived from both maternal mineralocorticoid organs and the placenta to regenerate cortisol (Figure 1; Murphy, 1977, 1979). The expression of 11β-HSD1 in the fetal membranes increases with gestational age and further increases in parturition with its abundance atop all fetal tissues by the end of gestation (Murphy, 1977, 1981; Alfaidy et al., 2003). This cortisol-regenerating capacity of the fetal membranes is even regarded as a supplemental extra-adrenal source of glucocorticoids in pregnancy (Murphy, 1977, 1981; Tanswell et al., 1977). Why should the fetal membranes be equipped with such a unique cortisol-regenerating capacity in pregnancy? Given that the smooth chorion is actually atrophied chorion villi, it is plausible to question whether this cortisol regeneration activity of the fetal membranes is merely an irrelevant by-product of pregnancy or a finely mapped-out event, which is absolutely required toward the end of gestation. In this review article, we will try to answer these questions by summarizing data from our laboratory as well as others.
Feedforward Cortisol Regeneration in the Fetal Membranes
The fetal membrane components differ in different species (Mess et al., 2003). Human fetal membranes comprised the amnion and smooth chorion. The smooth chorion is the outer layer that connects the maternal decidua and can be subdivided into a trophoblast layer and a connective tissue layer adjacent to the amnion. The amnion is composed of a single layer of amnion epithelial cells sitting on a basement membrane and a tough compact layer that contains abundant interstitial fibers and fibroblasts (Bourne, 1960; Parry and Strauss, 3rd., 1998; Wang et al., 2018). Studies of 11β-HSD1 in the fetal membranes of other species are sparse. However, 11β-HSD1 has been localized to the placenta in a number of species including rat, sheep, and baboon (Burton and Waddell, 1994; Pepe et al., 1996; Yang et al., 1997). Immunohistochemical staining of human fetal membranes shows that 11β-HSD1 distributes in whole membrane layers (Sun et al., 1997; Wang et al., 2012; Liu et al., 2016a). In the amnion, 11β-HSD1 distributes in both epithelial and fibroblast cells, whereas in the smooth chorion, 11β-HSD1 is localized to trophoblasts, as well as fibroblasts (Sun et al., 1997; Wang et al., 2012; Liu et al., 2016a). Notably, in contrast to the expression of 11β-HSD2 in trophoblasts of human placenta chorionic villi, trophoblasts of the smooth chorion express mainly 11β-HSD1 rather than 11β-HSD2 (Sun et al., 1997). These discrepancies are suggestive of a unique role of 11β-HSD1 rather than a by-product of atrophied villi in the fetal membranes.
Intriguingly, cortisol, despite being a product of 11β-HSD1, induces rather than inhibits 11β-HSD1 expression in both smooth chorion trophoblasts and amnion fibroblasts (Sun et al., 2002; Sun and Myatt, 2003; Li et al., 2006; Yang et al., 2007), thus setting up a positive feedback loop between cortisol regeneration and 11β-HSD1 expression in the fetal membranes. This feedforward expression pattern of 11β-HSD1 in the fetal membranes may account for its increasing expression with gestational age (Alfaidy et al., 2003) and its further increase at parturition (Liu et al., 2016a).
The actions of glucocorticoids and proinflammatory cytokines usually oppose each other at sites of inflammation. However, in the fetal membranes, proinflammatory cytokines induce the expression of 11β-HSD1 not only on their own, but also in synergy with glucocorticoids (Sun and Myatt, 2003; Li et al., 2006; Lu et al., 2019c). Given that inflammation is a common cause of both term and preterm birth, and overproduction of proinflammatory cytokines is a common feature of inflammation (Bollapragada et al., 2009; Menon et al., 2010; Romero et al., 2014; Singh et al., 2019), the synergy between glucocorticoids and proinflammatory cytokines in the induction of 11β-HSD1 expression is particularly noteworthy because this synergy is very likely to generate even more cortisol under conditions of chorioamnionitis. These distinct features of 11β-HSD1 expression in the fetal membranes denote again that the expression of 11β-HSD1 in the fetal membranes may be a requisite event in the end of normal gestation and may even be more intriguing in the condition of chorioamnionitis. In non-gestational tissues, proinflammatory cytokines have also been shown to induce 11β-HSD1 expression either on its own (Tomlinson et al., 2001; Yong et al., 2002; Ignatova et al., 2009; Esteves et al., 2014) or in synergy with glucocorticoids (Rae et al., 2004; Kaur et al., 2010), which is regarded as a self-restraining mechanism to avoid over immune responses in inflammation. Is cortisol regeneration by 11β-HSD1 in the fetal membranes simply a self-restraining mechanism to contain inflammation or does it mean more than an anti-inflammatory role in pregnancy? Convincing evidence has accumulated that glucocorticoids derived from fetal adrenal glands can trigger parturition in a number of animal species (Anderson et al., 1975; Flint et al., 1978; First and Bosc, 1979; Morrison et al., 1983; Fowden et al., 2008). However, adrenal glands of human fetus produce mainly dehydroepiandrosterone sulfate (DHEAS) rather than glucocorticoids (Mesiano and Jaffe, 1997; Ishimoto and Jaffe, 2011). This feature of human fetal adrenal glands may also explain why systemic administration of synthetic glucocorticoids such as betamethasone apparently does not induce labor (Craft et al., 1976). This is probably due to the strong negative feedback of synthetic glucocorticoids on the fetal hypothalamic–pituitary–adrenal axis, which may result in diminished production of both DHEAS and cortisol when synthetic glucocorticoids pass through the placenta and enter the fetal circulation. Because DHEAS is a precursor for estrogen synthesis in the placenta, diminished DHEAS will lead to reduced estrogen production (Ogueh et al., 1999), which is essential for preparation of the myometrium for contraction (Mesiano, 2001). Therefore, it is very likely that the feedforward cortisol regeneration by 11β-HSD1 in the fetal membranes is a compensatory mechanism in parturition for the insufficient cortisol synthesis by fetal adrenal glands in humans. In other words, local regeneration of cortisol in the fetal membranes is probably more important than cortisol derived from fetal adrenal glands in human parturition. This notion is endorsed by increased abundance of glucocorticoid receptor (GR) in the fetal membranes at parturition (Sun et al., 1996), suggesting that cortisol regenerated by 11β-HSD1 in the fetal membranes has enhanced local actions pertinent to human parturition.
Role of Cortisol Regeneration in the Rupture of Fetal Membranes
During the gestational period, a tough and tensile amniotic sac is required for holding and protecting the fetus bathed in the amniotic fluid. The tensile strength of the fetal membranes is believed to derive mainly from the rich content of collagenous fibers in the compact layer of the amnion (Bourne, 1962; Parry and Strauss, 3rd., 1998; Oyen et al., 2006). Needless to say, the fetal membranes should break for the delivery of fetus at parturition, and rupture of membranes can, in turn, promote labor, suggesting that the fetal membranes are a source of labor initiating signals. As a matter of fact, the fetal membranes are indeed among the gestational tissues that give rise to signals leading to parturition (Parry and Strauss, 3rd., 1998; Menon, 2016; Menon et al., 2016). These multiple source signals may need to work in a coordinating manner to start labor at term. However, intensified signals from the fetal membranes as elicited by membrane rupture or infection can sometimes even start labor alone no matter at term and preterm, which highlights the importance of membrane rupture in parturition. In line with the rupture of membranes, the fetal membranes become increasingly weak toward the end of gestation. Our serial studies indicate that local regeneration of cortisol is an important attributor to membrane weakening and rupture (Liu et al., 2016a; Wang et al., 2016, 2019a; Mi et al., 2017, 2018).
The initial inspiration that has encouraged us to investigate whether cortisol regeneration is involved in membrane rupture comes from the side effects of clinical usage of glucocorticoids. Based on their potent anti-inflammatory properties, synthetic glucocorticoids are widely prescribed to treat a variety of acute and chronic inflammatory conditions (Rhen and Cidlowski, 2005). Among the side effects of glucocorticoids, skin atrophy is frequently encountered (Sterry and Asadullah, 2002; Schoepe et al., 2006). Fibroblasts have been shown to be the target cells of glucocorticoids for this side effect (Nuutinen et al., 2001; Oishi et al., 2002; Schoepe et al., 2006). It has been demonstrated that glucocorticoids reduce tensile strength and elasticity of the skin by decreasing the synthesis of extracellular matrix (ECM) proteins and increasing their turnover in fibroblasts (Cutroneo et al., 1975, 1981; Shull and Cutroneo, 1986; Nuutinen et al., 2001; Oishi et al., 2002; Boudon et al., 2017). For the same reason, glucocorticoids are being used topically to prevent excessive scar formation (Berliner et al., 1967; Jalali and Bayat, 2007). These clinical observations have prompted us to envisage that the feedforward cortisol regeneration in the fetal membranes may be related to ECM remodeling for membrane rupture.
It is now known that the tensile strength of the amnion is largely attributed to collagen I and III contents in the compact layer (Bourne, 1960; Malak et al., 1993; Bryant-Greenwood, 1998). Abundance of collagen I and III in the amnion decreases after the middle trimester to a nadir at term (Skinner et al., 1981; Casey and MacDonald, 1996; Hampson et al., 1997). In preterm premature rupture of membranes, a common cause of preterm labor, collagen content is further decreased (Skinner et al., 1981; Hampson et al., 1997; Stuart et al., 2005). These findings suggest that decreased collagen abundance characterizes the process of membrane rupture. We investigated whether cortisol regenerated in the fetal membranes is involved in the reduction of collagens in cultured primary human amnion fibroblasts, which are the major source of ECM proteins in the amnion compact layer (Casey and MacDonald, 1996). We have demonstrated that cortisol decreases the abundance of collagen I and III protein in a concentration-dependent manner with no effects on their mRNA abundance in amnion fibroblasts. Further mechanistic studies have revealed that cortisol decreases collagen I and III protein abundance by activating the autophagic and proteasome pathways, respectively (Mi et al., 2017, 2018). In addition to collagen I and III, collagen IV is essential for the maintenance of epithelial basal membrane as well as for the ECM structural protein assembling in the amnion (Malak et al., 1993; Bryant-Greenwood, 1998). For this reason, collagen IV is known as another crucial determinant of membrane integrity. By using human amnion fibroblasts, we have found that cortisol also causes the breakdown of collagen IV in a concentration-dependent manner through drastic induction of the expression of matrix metalloprotease 7 (MMP-7), also known as matrilysin, via activation of the AP-1 transcription factor (Wang et al., 2019a). These inhibitory effects of cortisol on collagen I, III, and IV have shown to be GR-mediated effects and have been confirmed in amnion tissue explant experiments. These findings are also substantiated by concurrent increases in cortisol, 11β-HSD1, MMP-7, and markers for autophagy and decreases in collagen I, III, and IV in the amnion tissue collected from spontaneous labor with membrane rupture (Liu et al., 2016a; Mi et al., 2017, 2018; Wang et al., 2019a, b).
The tensile strength of the amnion is determined not only by collagen abundance, but also by the degree of their cross-linking. Cross-linked collagens become tough and resistant to the breakdown by MMPs (Vater et al., 1979). It is now known that the cross-linking of collagens is catalyzed by lysyl oxidase (LOX), a copper-dependent amine oxidase (Kagan and Trackman, 1991). It has been shown that LOX protein and enzyme activity decrease dramatically in the amnion with advancing gestational age and further decrease in spontaneous labor with membrane rupture (Casey and MacDonald, 1997; Liu et al., 2016a, b). We have demonstrated that both cortisol and cortisone inhibit LOX expression in human amnion fibroblasts, and the effect of cortisone is abolished with inhibition of 11β-HSD1 (Liu et al., 2016a). Again, these effects have proved to be mediated by GR and have been replicated in amnion tissue explant experiments.
In addition to the effects mediated directly by GR, an alternative mechanism is also present in ECM remodeling effects of cortisol. It has been shown that serum amyloid A1 (SAA1), an acute phase protein produced primarily by the liver, could be produced locally in the fetal membranes (Li et al., 2017), where SAA1 exerts extensive ECM remodeling effects including induction of MMP-1, MMP-2, MMP-8, MMP-9, and MMP-13; inhibition of LOX-like 1; and evoking collagen degradation (Wang et al., 2019b, c). Moreover, SAA1 can induce the expression of 11β-HSD1, and cortisol can in turn stimulate the expression of SAA1 (Lu et al., 2019b, c), thus formulating a mutual reinforcing mechanism in the production of SAA1 and cortisol in the fetal membranes. These findings are suggestive of existence of an alternative mechanism through induction of SAA1 to remodel the ECM structure by cortisol in the fetal membranes. Taken together, all these findings of cortisol’s actions on collagens, LOX, and MMP-7 in human amnion fibroblasts are supportive of the view that cortisol regenerated by 11β-HSD1 plays an important role in the ECM remodeling for membrane rupture at parturition (Figure 2).
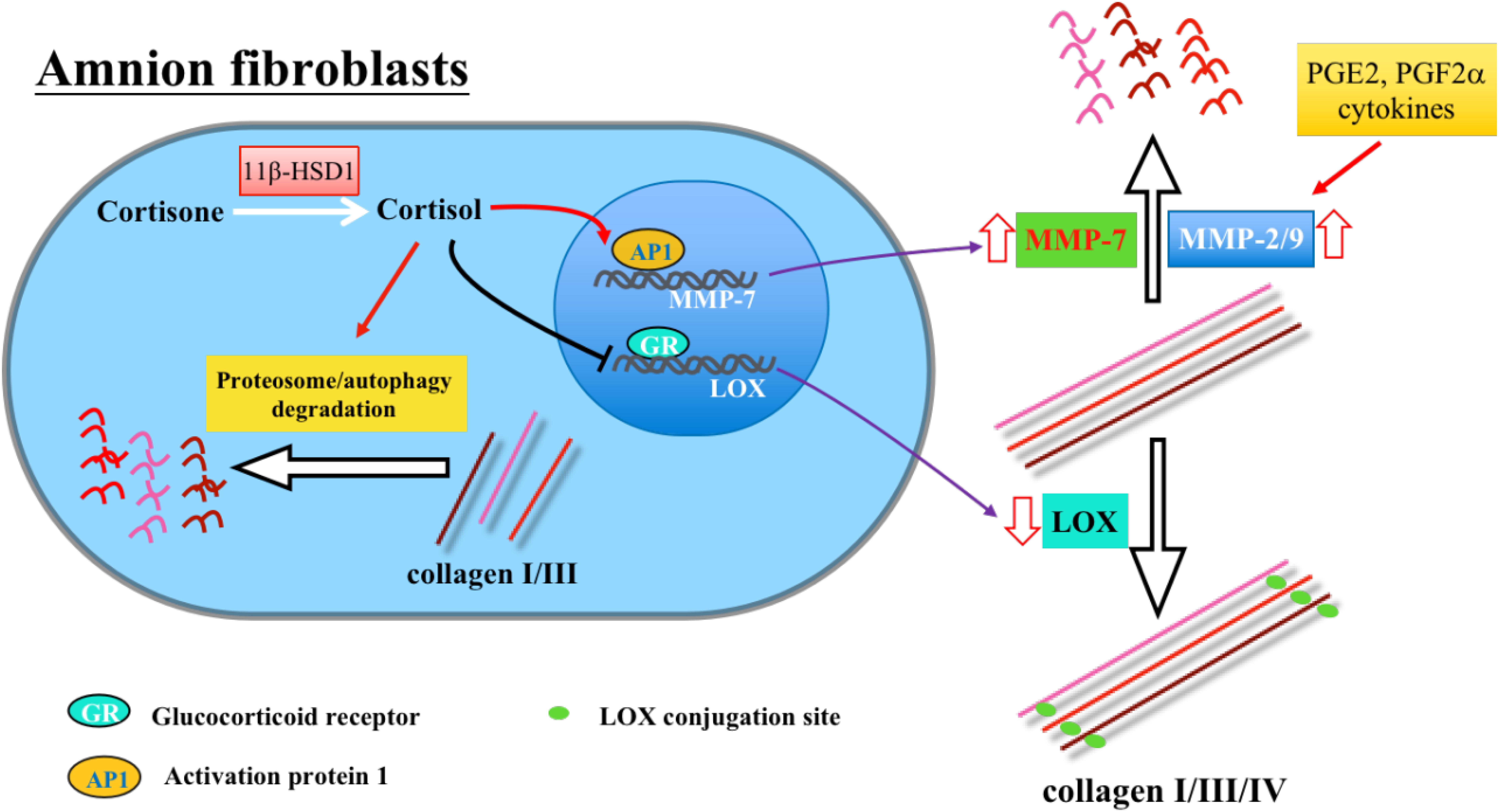
Figure 2. Extracellular matrix (ECM) remodeling effects of cortisol regenerated by 11β-HSD1 in the fetal membranes.
Role of Cortisol Regeneration in the Stimulation of Prostaglandin E2 and F2α Output in the Fetal Membranes
Prostaglandins, particularly prostaglandin E2 (PGE2) and prostaglandin F2α (PGF2α), play crucial roles in human parturition (Challis et al., 1997; Romero et al., 2014). They increase myometrium contractility, ripen the cervix, and promote fetal membrane rupture (Challis et al., 1997; Romero et al., 2014). Although all gestational tissues including fetal membranes and maternal decidua/myometrium are virtually capable of PGE2 and PGF2α synthesis, the fetal amnion and maternal decidua/myometrium are recognized to synthesize the most PGE2 and PGF2α, respectively (Duchesne et al., 1978; Mitchell et al., 1978; Okazaki et al., 1981; Rehnstrom et al., 1983). In the amnion, the capacity of PGE2 synthesis in interstitial fibroblasts is approximately 5 times more than the capacity in epithelial cells (Sun et al., 2003). In addition, amnion fibroblasts are also capable of synthesizing PGF2α, although not as much as PGE2 (Guo et al., 2014). As narrated above, glucocorticoids are the most widely used class of anti-inflammatory drugs with prominent inhibitory effects on the synthesis of proinflammatory cytokines, as well as prostaglandins (Rhen and Cidlowski, 2005). These inhibitory effects of glucocorticoids on prostaglandin synthesis are known to be executed mostly through inhibition of cyclooxygenase 2 (COX-2) expression, the rate-limiting enzyme in prostaglandin synthesis (Goppelt-Struebe et al., 1989; Lasa et al., 2001; Lim et al., 2014). However, it has been noted for a long time that there are parallel increases in cortisol and prostaglandin levels in the maternal circulation toward the end of human gestation, and this apparent contradiction is described as a gestational paradox (Casey et al., 1985). Several groups have confirmed that this paradoxical phenomenon holds true in human amnion fibroblasts (Potestio et al., 1988; Zakar et al., 1995; Blumenstein et al., 2000; Sun et al., 2003, 2006). It has been shown that both cortisol and synthetic glucocorticoids stimulate rather than inhibit the expression of COX-2 in human amnion fibroblasts. Moreover, glucocorticoids also induce the expression of cytosolic phospholipase A2 (cPLA2) in human amnion fibroblasts (Sun et al., 2003; Guo et al., 2008, 2010). Cytosolic phospholipase A2 catalyzes the formation of arachidonic acid, a rate-limiting substrate in prostaglandin synthesis, from membrane phospholipids. The mechanism underlying the paradoxical induction of cPLA2 and COX-2 by glucocorticoids is fascinating because glucocorticoids inhibit the expression of proinflammatory cytokines in amnion fibroblasts at the same time. We set out to delineate this paradoxical mechanism and it turned out to be a very complicated mechanism. It is revealed that glucocorticoids induce cPLA2 and COX-2 expression through stimulation of the cAMP/PKA pathway with subsequent activation of multiple transcription factors including CREB and STAT3, and so on (Zhu et al., 2009; Guo et al., 2010; Wang et al., 2015; Lu et al., 2017, 2019a). Interestingly, the classical inflammatory transcription factor, nuclear factor κB, is nevertheless inhibited by glucocorticoids (Guo, 2010), which is responsible for the inhibition of proinflammatory cytokine expression in human amnion fibroblasts, a situation resembling most of non-gestational tissues.
As depicted above, the amnion is also capable of synthesizing PGF2α, although not as much as PGE2 (Guo et al., 2014). There are multiple pathways for PGF2α synthesis in amnion fibroblast. In addition to PGF synthase (PGFS)–catalyzed formation of PGF2α from PGH2, PGF2α can also be converted from PGE2 by the enzyme carbonyl reductase 1 (CBR1) (Ziboh et al., 1977). We have found that cortisol significantly induces the synthesis of PGF2α through induction of CBR1 but not PGFS in amnion fibroblasts (Sun et al., 2003; Guo et al., 2014). This induction of CBR1 by cortisol was revealed to be GR-mediated enhancement of CBR1 transcription (Guo et al., 2014).
During the gestational period, there is abundant expression of prostaglandin degrading enzyme 15-hydroxyprostaglandin dehydrogenase (PGDH) in trophoblasts of the smooth chorion, which is known as a prostaglandin barrier (Cheung et al., 1992; Johnson et al., 2004). PGDH catalyzes NAD+-linked oxidation of 15 (S)-hydroxyl group of prostaglandins resulting in inactivation of their biological activities (Tai et al., 2002). Therefore, PGE2 and PGF2α synthesized in the amnion are mostly blocked from reaching the uterus by this barrier. Studies have shown that the abundant expression of PGDH in chorion trophoblasts is maintained mostly by progesterone (Challis et al., 1999; Patel and Challis, 2002). However, this maintenance is eventually undermined by increasing concentrations of cortisol derived from the feedforward regeneration through 11β-HSD1 (Patel et al., 1999a, b; Patel and Challis, 2002). The involvement of 11β-HSD1 is supported by findings that inhibition of PGDH by cortisone is reversed by 11β-HSD1 inhibitor (Patel et al., 1999b). It has been suggested that accumulating cortisol may compete with progesterone for progesterone receptor, thereby attenuating the maintaining effect of progesterone on PGDH expression and leading to progressively undermined prostaglandin barrier in the smooth chorion at term (Patel et al., 2003). Like the situation of cPLA2 and COX-2 induction by glucocorticoids, the inhibition of PGDH by glucocorticoids in the smooth chorion is also a kind of paradox, which is in marked contrast to the induction of PGDH expression by glucocorticoids in most of non-gestational tissues (Xun et al., 1991; Tong and Tai, 2005). All these findings of glucocorticoids on the induction of cPLA2/COX-2/CBR1 in amnion fibroblasts and inhibition of PGDH in chorion trophoblasts are supportive of a role of cortisol regeneration in the stimulation of PGE2 and PGF2α output either by induction of their synthesis or by inhibition of their degradation in the fetal membranes (Figure 3).
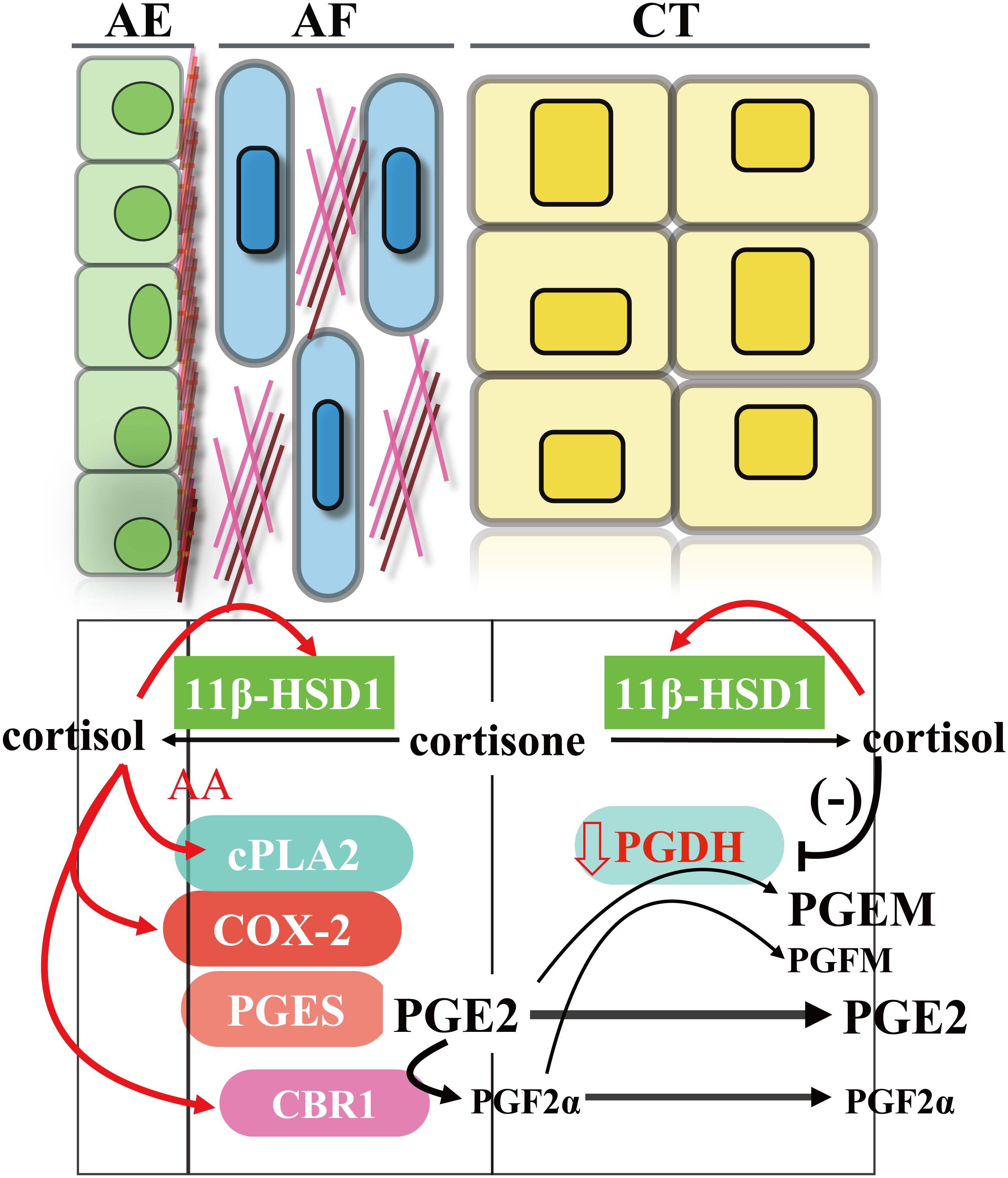
Figure 3. Induction of PGE2 and PGF2α output by cortisol regenerated through 11β-HSD1 in human fetal membranes. AE, amnion epithelial cells; AF, amnion fibroblasts; CT, smooth chorion trophoblasts; AA, arachidonic acid.
Summary and Perspectives
In pregnancy, there is increasing cortisol regeneration by 11β-HSD1 toward the end of gestation. 11β-HSD1 is expressed in virtually all cell types in the fetal membranes. Although the smooth chorion of the fetal membranes is considered as atrophied chorionic villi, the fetal membranes including the smooth chorion are endocrinologically active in pregnancy. Cortisol regeneration by 11β-HSD1 is one of such endocrine activities. Cortisol regenerated by 11β-HSD1 is involved not only in ECM remodeling for membrane rupture but also in the upregulation of PGE2 and PGF2α outputs. Both are requisite events for the onset of parturition. In addition to the actions described in this review, it remains to be uncovered whether cortisol regenerated by 11β-HSD1 in the fetal membranes possesses other unknown actions pertinent to the onset of labor. Another interesting issue remains to be clarified is the apparent contradictions between the concurrent induction of prostaglandins and inhibition of proinflammatory cytokines by glucocorticoids in the fetal membranes. Because both prostaglandins and proinflammatory cytokines are prolabor factors involved in ECM remodeling and uterine contractile activities (Keelan et al., 2003), it would be interesting to understand how these actions of cortisol on prostaglandins and proinflammatory cytokines are balanced in the fetal membrane at parturition, particularly in the situation of chorioamnionitis. Nevertheless, these effects of cortisol on ECM remodeling and prostaglandin output might be even enhanced in chorioamnionitis, given the potentiation of cortisol regeneration by proinflammatory cytokines. These apparent contradictions may represent a unique feature how glucocorticoids work locally in the fetal membranes in the promotion of labor. Simultaneous inhibition of proinflammatory cytokines and stimulation of prostaglandins by glucocorticoids may avoid deleterious effects of proinflammatory cytokines on the fetus on the one hand but save the labor promoting effects of prostaglandins on the other hand. Finally, it would be helpful to find a suitable animal model to replicate those findings in humans, such as the expression pattern of 11β-HSD1 across gestational age and those actions of glucocorticoids on ECM remodeling, prostaglandin synthesis, and degradation in the fetal membranes. What is more important is to test whether local artificial manipulation of 11β-HSD1 and GR expression in the fetal membranes can indeed change the course of gestation in the right animal model. Taking all together, we can conclude that cortisol regeneration in the fetal membranes is not a coincidental but a requisite event in parturition.
Author Contributions
KS conceived the idea. KS, W-SW, and C-MG contributed to manuscript writing and figure preparation.
Funding
This work was supported by the National Natural Science Foundation of China (81830042).
Conflict of Interest
The authors declare that the research was conducted in the absence of any commercial or financial relationships that could be construed as a potential conflict of interest.
References
Albiston, A. L., Obeyesekere, V. R., Smith, R. E., and Krozowski, Z. S. (1994). Cloning and tissue distribution of the human 11 beta-hydroxysteroid dehydrogenase type 2 enzyme. Mol. Cell. Endocrinol. 105, R11–R17. doi: 10.1016/0303-7207(94)90176-7
Alfaidy, N., Li, W., Macintosh, T., Yang, K., and Challis, J. (2003). Late gestation increase in 11beta-hydroxysteroid dehydrogenase 1 expression in human fetal membranes: a novel intrauterine source of cortisol. J. Clin. Endocrinol. Metab. 88, 5033–5038. doi: 10.1210/jc.2002-021915
Anderson, A. B., Flint, A. P., and Turnbull, A. C. (1975). Mechanism of action of glucocorticoids in induction of ovine parturition: effect on placental steroid metabolism. J. Endocrinol. 66, 61–70.
Bae, Y. J., and Kratzsch, J. (2015). Corticosteroid-binding globulin: modulating mechanisms of bioavailability of cortisol and its clinical implications. Best Pract. Res. Clin. Endocrinol. Metab. 29, 761–772. doi: 10.1016/j.beem.2015.09.001
Beato, M., and Klug, J. (2000). Steroid hormone receptors: an update. Hum. Reprod. Update 6, 225–236.
Berliner, D. L., Williams, R. J., Taylor, G. N., and Nabors, C. J. Jr. (1967). Decreased scar formation with topical corticosteroid treatment. Surgery 61, 619–625.
Blumenstein, M., Hansen, W. R., Deval, D., and Mitchell, M. D. (2000). Differential regulation in human amnion epithelial and fibroblast cells of prostaglandin E(2) production and prostaglandin H synthase-2 mRNA expression by dexamethasone but not tumour necrosis factor-alpha. Placenta 21, 210–217. doi: 10.1053/plac.1999.0473
Bollapragada, S., Youssef, R., Jordan, F., Greer, I., Norman, J., and Nelson, S. (2009). Term labor is associated with a core inflammatory response in human fetal membranes, myometrium, and cervix. Am. J. Obstet. Gynecol. 200, 104.e1-11. doi: 10.1016/j.ajog.2008.08.032
Boudon, S. M., Vuorinen, A., Geotti-Bianchini, P., Wandeler, E., Kratschmar, D. V., Heidl, M., et al. (2017). Novel 11beta-hydroxysteroid dehydrogenase 1 inhibitors reduce cortisol levels in keratinocytes and improve dermal collagen content in human ex vivo skin after exposure to cortisone and UV. PLoS One 12:e0171079. doi: 10.1371/journal.pone.0171079
Bourne, G. (1962). The foetal membranes. A review of the anatomy of normal amnion and chorion and some aspects of their function. Postgrad. Med. J. 38, 193–201. doi: 10.1136/pgmj.38.438.193
Bourne, G. L. (1960). The microscopic anatomy of the human amnion and chorion. Am. J. Obstet. Gynecol. 79, 1070–1073. doi: 10.1016/0002-9378(60)90512-3
Bryant-Greenwood, G. D. (1998). The extracellular matrix of the human fetal membranes: structure and function. Placenta 19, 1–11. doi: 10.1016/s0143-4004(98)90092-3
Burton, P. J., and Waddell, B. J. (1994). 11 beta-Hydroxysteroid dehydrogenase in the rat placenta: developmental changes and the effects of altered glucocorticoid exposure. J. Endocrinol. 143, 505–513. doi: 10.1677/joe.0.1430505
Burton, P. J., and Waddell, B. J. (1999). Dual function of 11beta-hydroxysteroid dehydrogenase in placenta: modulating placental glucocorticoid passage and local steroid action. Biol. Reprod. 60, 234–240. doi: 10.1095/biolreprod60.2.234
Casey, M. L., and MacDonald, P. C. (1996). Interstitial collagen synthesis and processing in human amnion: a property of the mesenchymal cells. Biol. Reprod. 55, 1253–1260. doi: 10.1095/biolreprod55.6.1253
Casey, M. L., and MacDonald, P. C. (1997). Lysyl oxidase (ras recision gene) expression in human amnion: ontogeny and cellular localization. J. Clin. Endocrinol. Metab. 82, 167–172. doi: 10.1210/jcem.82.1.3668
Casey, M. L., Macdonald, P. C., and Mitchell, M. D. (1985). Despite a massive increase in cortisol secretion in women during parturition, there is an equally massive increase in prostaglandin synthesis. A paradox? J. Clin. Invest. 75, 1852–1857. doi: 10.1172/JCI111899
Challis, J. R., Lye, S. J., and Gibb, W. (1997). Prostaglandins and parturition. Ann. N. Y. Acad. Sci. 828, 254–267.
Challis, J. R., Patel, F. A., and Pomini, F. (1999). Prostaglandin dehydrogenase and the initiation of labor. J. Perinat. Med. 27, 26–34.
Chapman, K., Holmes, M., and Seckl, J. (2013). 11beta-hydroxysteroid dehydrogenases: intracellular gate-keepers of tissue glucocorticoid action. Physiol. Rev. 93, 1139–1206. doi: 10.1152/physrev.00020.2012
Chapman, K. E., Kotelevtsev, Y. V., Jamieson, P. M., Williams, L. J., Mullins, J. J., and Seckl, J. R. (1997). Tissue-specific modulation of glucocorticoid action by the 11 beta-hydroxysteroid dehydrogenases. Biochem. Soc. Trans. 25, 583–587. doi: 10.1042/bst0250583
Cheung, P. Y., Walton, J. C., Tai, H. H., Riley, S. C., and Challis, J. R. (1992). Localization of 15-hydroxy prostaglandin dehydrogenase in human fetal membranes, decidua, and placenta during pregnancy. Gynecol. Obstet. Invest. 33, 142–146. doi: 10.1159/000294868
Craft, I., Brummer, V., Horwell, D., and Morgan, H. (1976). Betamethazone induction of labour. Proc. R. Soc. Med. 69, 827–828.
Cutroneo, K. R., Rokowski, R., and Counts, D. F. (1981). Glucocorticoids and collagen synthesis: comparison of in vivo and cell culture studies. Coll. Relat. Res. 1, 557–568. doi: 10.1016/s0174-173x(81)80037-4
Cutroneo, K. R., Stassen, F. L., and Cardinale, G. J. (1975). Anti-inflammatory steroids and collagen metabolism: glucocorticoid-mediated decrease of prolyl hydroxylase. Mol. Pharmacol. 11, 44–51.
Drake, A. J., Tang, J. I., and Nyirenda, M. J. (2007). Mechanisms underlying the role of glucocorticoids in the early life programming of adult disease. Clin. Sci. 113, 219–232. doi: 10.1042/CS20070107
Duchesne, M. J., Thaler-Dao, H., and De Paulet, A. C. (1978). Prostaglandin synthesis in human placenta and fetal membranes. Prostaglandins 15, 19–42.
Esteves, C. L., Kelly, V., Breton, A., Taylor, A. I., West, C. C., Donadeu, F. X., et al. (2014). Proinflammatory cytokine induction of 11beta-hydroxysteroid dehydrogenase type 1 (11beta-HSD1) in human adipocytes is mediated by MEK, C/EBPbeta, and NF-kappaB/RelA. J. Clin. Endocrinol. Metab. 99, E160–E168. doi: 10.1210/jc.2013-1708
Ferner, K., and Mess, A. (2011). Evolution and development of fetal membranes and placentation in amniote vertebrates. Respir. Physiol. Neurobiol. 178, 39–50. doi: 10.1016/j.resp.2011.03.029
First, N. L., and Bosc, M. J. (1979). Proposed mechanisms controlling parturition and the induction of parturition in swine. J. Anim. Sci. 48, 1407–1421. doi: 10.2527/jas1979.4861407x
Flint, A. P., Kingston, E. J., Robinson, J. S., and Thorburn, G. D. (1978). Initiation of parturition in the goat: evidence for control by foetal glucocorticoid through activation of placental C21-steroid 17alpha-hydroxylase. J. Endocrinol. 78, 367–378. doi: 10.1677/joe.0.0780367
Fowden, A. L., Forhead, A. J., and Ousey, J. C. (2008). The endocrinology of equine parturition. Exp. Clin. Endocrinol. Diabetes 116, 393–403. doi: 10.1055/s-2008-1042409
Goppelt-Struebe, M., Wolter, D., and Resch, K. (1989). Glucocorticoids inhibit prostaglandin synthesis not only at the level of phospholipase A2 but also at the level of cyclo-oxygenase/PGE isomerase. Br. J. Pharmacol. 98, 1287–1295. doi: 10.1111/j.1476-5381.1989.tb12676.x
Guo, C., Li, J., Myatt, L., Zhu, X., and Sun, K. (2010). Induction of Galphas contributes to the paradoxical stimulation of cytosolic phospholipase A2alpha expression by cortisol in human amnion fibroblasts. Mol. Endocrinol. 24, 1052–1061. doi: 10.1210/me.2009-0488
Guo, C., Wang, W., Liu, C., Myatt, L., and Sun, K. (2014). Induction of PGF2αlpha synthesis by cortisol through GR dependent induction of CBR1 in human amnion fibroblasts. Endocrinology 155, 3017–3024. doi: 10.1210/en.2013-1848
Guo, C., Yang, Z., Li, W., Zhu, P., Myatt, L., and Sun, K. (2008). Paradox of glucocorticoid-induced cytosolic phospholipase A2 group IVA messenger RNA expression involves glucocorticoid receptor binding to the promoter in human amnion fibroblasts. Biol. Reprod. 78, 193–197. doi: 10.1095/biolreprod.107.063990
Guo, C. M. (2010). Molecular Mechanism of the Paradoxical Induction of Cytosolic Phospholipase A2 by Glucocorticoids in Human Amnion Fibroblasts. Doctoral thesis, Fudan University, Shanghai.
Hampson, V., Liu, D., Billett, E., and Kirk, S. (1997). Amniotic membrane collagen content and type distribution in women with preterm premature rupture of the membranes in pregnancy. Br. J. Obstet. Gynaecol. 104, 1087–1091. doi: 10.1111/j.1471-0528.1998.tb10107.x
Ignatova, I. D., Kostadinova, R. M., Goldring, C. E., Nawrocki, A. R., Frey, F. J., and Frey, B. M. (2009). Tumor necrosis factor-alpha upregulates 11beta-hydroxysteroid dehydrogenase type 1 expression by CCAAT/enhancer binding protein-beta in HepG2 cells. Am. J. Physiol. Endocrinol. Metab. 296, E367–E377. doi: 10.1152/ajpendo.90531.2008
Ishimoto, H., and Jaffe, R. B. (2011). Development and function of the human fetal adrenal cortex: a key component in the feto-placental unit. Endocr. Rev. 32, 317–355. doi: 10.1210/er.2010-0001
Jalali, M., and Bayat, A. (2007). Current use of steroids in management of abnormal raised skin scars. Surgeon 5, 175–180. doi: 10.1016/s1479-666x(07)80045-x
Johnson, R. F., Mitchell, C. M., Clifton, V., and Zakar, T. (2004). Regulation of 15-hydroxyprostaglandin dehydrogenase (PGDH) gene activity, messenger ribonucleic acid processing, and protein abundance in the human chorion in late gestation and labor. J. Clin. Endocrinol. Metab. 89, 5639–5648. doi: 10.1210/jc.2004-0540
Kagan, H. M., and Trackman, P. C. (1991). Properties and function of lysyl oxidase. Am. J. Respir. Cell Mol. Biol. 5, 206–210.
Kaur, K., Hardy, R., Ahasan, M. M., Eijken, M., Van Leeuwen, J. P., Filer, A., et al. (2010). Synergistic induction of local glucocorticoid generation by inflammatory cytokines and glucocorticoids: implications for inflammation associated bone loss. Ann. Rheum. Dis. 69, 1185–1190. doi: 10.1136/ard.2009.107466
Keelan, J. A., Blumenstein, M., Helliwell, R. J., Sato, T. A., Marvin, K. W., and Mitchell, M. D. (2003). Cytokines, prostaglandins and parturition–a review. Placenta 24, (Suppl A) S33–S46.
Krozowski, Z., Maguire, J. A., Stein-Oakley, A. N., Dowling, J., Smith, R. E., and Andrews, R. K. (1995). Immunohistochemical localization of the 11 beta-hydroxysteroid dehydrogenase type II enzyme in human kidney and placenta. J. Clin. Endocrinol. Metab. 80, 2203–2209. doi: 10.1210/jcem.80.7.7608280
Lasa, M., Brook, M., Saklatvala, J., and Clark, A. R. (2001). Dexamethasone destabilizes cyclooxygenase 2 mRNA by inhibiting mitogen-activated protein kinase p38. Mol. Cell. Biol. 21, 771–780. doi: 10.1128/MCB.21.3.771-780.2001
Leiser, R., and Kaufmann, P. (1994). Placental structure: in a comparative aspect. Exp. Clin. Endocrinol. 102, 122–134.
Lewis, J. G., Bagley, C. J., Elder, P. A., Bachmann, A. W., and Torpy, D. J. (2005). Plasma free cortisol fraction reflects levels of functioning corticosteroid-binding globulin. Clin. Chim. Acta 359, 189–194. doi: 10.1016/j.cccn.2005.03.044
Li, J., Wang, W., Liu, C., Wang, W., Li, W., Shu, Q., et al. (2013). Critical role of histone acetylation by p300 in human placental 11beta-HSD2 expression. J. Clin. Endocrinol. Metab. 98, E1189–E1197. doi: 10.1210/jc.2012-4291
Li, J. N., Ge, Y. C., Yang, Z., Guo, C. M., Duan, T., Myatt, L., et al. (2011). The Sp1 transcription factor is crucial for the expression of 11beta-hydroxysteroid dehydrogenase type 2 in human placental trophoblasts. J. Clin. Endocrinol. Metab. 96, E899–E907. doi: 10.1210/jc.2010-2852
Li, W., Gao, L., Wang, Y., Duan, T., Myatt, L., and Sun, K. (2006). Enhancement of cortisol-induced 11beta-hydroxysteroid dehydrogenase type 1 expression by interleukin 1beta in cultured human chorionic trophoblast cells. Endocrinology 147, 2490–2495. doi: 10.1210/en.2005-1626
Li, W., Wang, W., Zuo, R., Liu, C., Shu, Q., Ying, H., et al. (2017). Induction of pro-inflammatory genes by serum amyloid A1 in human amnion fibroblasts. Sci. Rep. 7:693. doi: 10.1038/s41598-017-00782-9
Lim, W., Park, C., Shim, M. K., Lee, Y. H., Lee, Y. M., and Lee, Y. (2014). Glucocorticoids suppress hypoxia-induced COX-2 and hypoxia inducible factor-1alpha expression through the induction of glucocorticoid-induced leucine zipper. Br. J. Pharmacol. 171, 735–745. doi: 10.1111/bph.12491
Liu, C., Guo, C., Wang, W., Zhu, P., Li, W., Mi, Y., et al. (2016a). Inhibition of lysyl oxidase by cortisol regeneration in human amnion: implications for rupture of fetal membranes. Endocrinology 157, 4055–4065. doi: 10.1210/en.2016-1406
Liu, C., Zhu, P., Wang, W., Li, W., Shu, Q., Chen, Z. J., et al. (2016b). Inhibition of lysyl oxidase by prostaglandin E2 via EP2/EP4 receptors in human amnion fibroblasts: implications for parturition. Mol. Cell. Endocrinol. 424, 118–127. doi: 10.1016/j.mce.2016.04.016
Lu, J., Wang, W., Mi, Y., Zhang, C., Ying, H., Wang, L., et al. (2017). AKAP95-mediated nuclear anchoring of PKA mediates cortisol-induced PTGS2 expression in human amnion fibroblasts. Sci. Signal. 10:eaac6160. doi: 10.1126/scisignal.aac6160
Lu, J. W., Wang, W. S., Zhou, Q., Gan, X. W., Myatt, L., and Sun, K. (2019a). Activation of prostaglandin EP4 receptor attenuates the induction of cyclooxygenase-2 expression by EP2 receptor activation in human amnion fibroblasts: implications for parturition. FASEB J. 33, 8148–8160. doi: 10.1096/fj.201802642R
Lu, Y., Wang, W. S., Lin, Y. K., Lu, J. W., Li, W. J., Zhang, C. Y., et al. (2019b). Enhancement of cortisol-induced SAA1 transcription by SAA1 in the human amnion. J. Mol. Endocrinol. 62, 149–158. doi: 10.1530/JME-18-0263
Lu, Y., Zhou, Q., Lu, J. W., Wang, W. S., and Sun, K. (2019c). Involvement of STAT3 in the synergistic induction of 11beta-HSD1 by SAA1 and cortisol in human amnion fibroblasts. Am. J. Reprod. Immunol. 82:e13150. doi: 10.1111/aji.13150
Malak, T. M., Ockleford, C. D., Bell, S. C., Dalgleish, R., Bright, N., and Macvicar, J. (1993). Confocal immunofluorescence localization of collagen types I, III, IV, V and VI and their ultrastructural organization in term human fetal membranes. Placenta 14, 385–406. doi: 10.1016/s0143-4004(05)80460-6
Menon, R. (2016). Human fetal membranes at term: dead tissue or signalers of parturition? Placenta 44, 1–5. doi: 10.1016/j.placenta.2016.05.013
Menon, R., Bonney, E. A., Condon, J., Mesiano, S., and Taylor, R. N. (2016). Novel concepts on pregnancy clocks and alarms: redundancy and synergy in human parturition. Hum. Reprod. Update 22, 535–560. doi: 10.1093/humupd/dmw022
Menon, R., and Moore, J. J. (2020). Fetal membranes, not a mere appendage of the placenta, but a critical part of the fetal-maternal interface controlling parturition. Obstet. Gynecol. Clin. North Am. 47, 147–162. doi: 10.1016/j.ogc.2019.10.004
Menon, R., Taylor, R. N., and Fortunato, S. J. (2010). Chorioamnionitis–a complex pathophysiologic syndrome. Placenta 31, 113–120. doi: 10.1016/j.placenta.2009.11.012
Mesiano, S. (2001). Roles of estrogen and progesterone in human parturition. Front. Horm. Res. 27, 86–104. doi: 10.1159/000061038
Mesiano, S., and Jaffe, R. B. (1997). Developmental and functional biology of the primate fetal adrenal cortex. Endocr. Rev. 18, 378–403. doi: 10.1210/edrv.18.3.0304
Mess, A., Blackburn, D. G., and Zeller, U. (2003). Evolutionary transformations of fetal membranes and reproductive strategies. J. Exp. Zool. A Comp. Exp. Biol. 299, 3–12. doi: 10.1002/jez.a.10287
Meyer, E. J., Nenke, M. A., Rankin, W., Lewis, J. G., and Torpy, D. J. (2016). Corticosteroid-binding globulin: a review of basic and clinical advances. Horm. Metab. Res. 48, 359–371. doi: 10.1055/s-0042-108071
Mi, Y., Wang, W., Lu, J., Zhang, C., Wang, Y., Ying, H., et al. (2018). Proteasome-mediated degradation of collagen III by cortisol in amnion fibroblasts. J. Mol. Endocrinol. 60, 45–54. doi: 10.1530/JME-17-0215
Mi, Y., Wang, W., Zhang, C., Liu, C., Lu, J., Li, W., et al. (2017). Autophagic degradation of collagen 1A1 by cortisol in human amnion fibroblasts. Endocrinology 158, 1005–1014. doi: 10.1210/en.2016-1829
Miller, W. L., and Auchus, R. J. (2011). The molecular biology, biochemistry, and physiology of human steroidogenesis and its disorders. Endocr. Rev. 32, 81–151. doi: 10.1210/er.2010-0013
Mitchell, M. D., Bibby, J., Hicks, B. R., and Turnbull, A. C. (1978). Specific production of prostaglandin E by human amnion in vitro. Prostaglandins 15, 377–382. doi: 10.1016/0090-6980(78)90177-6
Morgan, S. A., Mccabe, E. L., Gathercole, L. L., Hassan-Smith, Z. K., Larner, D. P., Bujalska, I. J., et al. (2014). 11beta-HSD1 is the major regulator of the tissue-specific effects of circulating glucocorticoid excess. Proc. Natl. Acad. Sci. U.S.A. 111, E2482–E2491. doi: 10.1073/pnas.1323681111
Morrison, D. G., Humes, P. E., and Godke, R. A. (1983). The use of dimenhydrinate in conjunction with dexamethasone for induction of parturition in beef cattle. Theriogenology 19, 221–233. doi: 10.1016/0093-691x(83)90008-0
Murphy, B. E. (1977). Chorionic membrane as an extra-adrenal source of foetal cortisol in human amniotic fluid. Nature 266, 179–181. doi: 10.1038/266179a0
Murphy, B. E. (1979). Cortisol and cortisone in human fetal development. J. Steroid Biochem. 11, 509–513.
Murphy, B. E. (1981). Ontogeny of cortisol-cortisone interconversion in human tissues: a role for cortisone in human fetal development. J. Steroid Biochem. 14, 811–817. doi: 10.1016/0022-4731(81)90226-0
Myatt, L., and Sun, K. (2010). Role of fetal membranes in signaling of fetal maturation and parturition. Int. J. Dev. Biol. 54, 545–553. doi: 10.1387/ijdb.082771lm
Ni, X. T., Duan, T., Yang, Z., Guo, C. M., Li, J. N., and Sun, K. (2009). Role of human chorionic gonadotropin in maintaining 11beta-hydroxysteroid dehydrogenase type 2 expression in human placental syncytiotrophoblasts. Placenta 30, 1023–1028. doi: 10.1016/j.placenta.2009.10.005
Nuutinen, P., Autio, P., Hurskainen, T., and Oikarinen, A. (2001). Glucocorticoid action on skin collagen: overview on clinical significance and consequences. J. Eur. Acad. Dermatol. Venereol. 15, 361–362.
Ogueh, O., Jones, J., Mitchell, H., Alaghband-Zadeh, J., and Johnson, M. R. (1999). Effect of antenatal dexamethasone therapy on maternal plasma human chorionic gonadotrophin, oestradiol and progesterone. Hum. Reprod. 14, 303–306. doi: 10.1093/humrep/14.2.303
Oishi, Y., Fu, Z. W., Ohnuki, Y., Kato, H., and Noguchi, T. (2002). Molecular basis of the alteration in skin collagen metabolism in response to in vivo dexamethasone treatment: effects on the synthesis of collagen type I and III, collagenase, and tissue inhibitors of metalloproteinases. Br. J. Dermatol. 147, 859–868. doi: 10.1046/j.1365-2133.2002.04949.x
Okazaki, T., Casey, M. L., Okita, J. R., Macdonald, P. C., and Johnston, J. M. (1981). Initiation of human parturition. XII. Biosynthesis and metabolism of prostaglandins in human fetal membranes and uterine decidua. Am. J. Obstet. Gynecol. 139, 373–381.
Oyen, M. L., Calvin, S. E., and Landers, D. V. (2006). Premature rupture of the fetal membranes: is the amnion the major determinant? Am. J. Obstet. Gynecol. 195, 510–515. doi: 10.1016/j.ajog.2006.02.010
Parry, S., and Strauss, J. F, 3rd. (1998). Premature rupture of the fetal membranes. N. Engl. J. Med. 338, 663–670.
Patel, F. A., and Challis, J. R. (2002). Cortisol/progesterone antagonism in regulation of 15-hydroxysteroid dehydrogenase activity and mRNA levels in human chorion and placental trophoblast cells at term. J. Clin. Endocrinol. Metab. 87, 700–708. doi: 10.1210/jcem.87.2.8245
Patel, F. A., Clifton, V. L., Chwalisz, K., and Challis, J. R. (1999a). Steroid regulation of prostaglandin dehydrogenase activity and expression in human term placenta and chorio-decidua in relation to labor. J. Clin. Endocrinol. Metab. 84, 291–299. doi: 10.1210/jcem.84.1.5399
Patel, F. A., Sun, K., and Challis, J. R. (1999b). Local modulation by 11beta-hydroxysteroid dehydrogenase of glucocorticoid effects on the activity of 15-hydroxyprostaglandin dehydrogenase in human chorion and placental trophoblast cells. J. Clin. Endocrinol. Metab. 84, 395–400. doi: 10.1210/jcem.84.2.5442
Patel, F. A., Funder, J. W., and Challis, J. R. (2003). Mechanism of cortisol/progesterone antagonism in the regulation of 15-hydroxyprostaglandin dehydrogenase activity and messenger ribonucleic acid levels in human chorion and placental trophoblast cells at term. J. Clin. Endocrinol. Metab. 88, 2922–2933. doi: 10.1210/jc.2002-021710
Pepe, G. J., Waddell, B. J., Burch, M. G., and Albrecht, E. D. (1996). Interconversion of cortisol and cortisone in the baboon placenta at midgestation: expression of 11beta-hydroxysteroid dehydrogenase type 1 messenger RNA. J. Steroid Biochem. Mol. Biol. 58, 403–410. doi: 10.1016/0960-0760(96)00049-0
Potestio, F. A., Zakar, T., and Olson, D. M. (1988). Glucocorticoids stimulate prostaglandin synthesis in human amnion cells by a receptor-mediated mechanism. J. Clin. Endocrinol. Metab. 67, 1205–1210. doi: 10.1210/jcem-67-6-1205
Rae, M. T., Niven, D., Critchley, H. O., Harlow, C. R., and Hillier, S. G. (2004). Antiinflammatory steroid action in human ovarian surface epithelial cells. J. Clin. Endocrinol. Metab. 89, 4538–4544. doi: 10.1210/jc.2003-032225
Rehnstrom, J., Ishikawa, M., Fuchs, F., and Fuchs, A. R. (1983). Stimulation of myometrial and decidual prostaglandin production by amniotic fluid from term, but not midtrimester pregnancies. Prostaglandins 26, 973–981. doi: 10.1016/0090-6980(83)90158-2
Rhen, T., and Cidlowski, J. A. (2005). Antiinflammatory action of glucocorticoids–new mechanisms for old drugs. N. Engl. J. Med. 353, 1711–1723. doi: 10.1056/NEJMra050541
Romero, R., Dey, S. K., and Fisher, S. J. (2014). Preterm labor: one syndrome, many causes. Science 345, 760–765. doi: 10.1126/science.1251816
Schmid, W., Cole, T. J., Blendy, J. A., and Schutz, G. (1995). Molecular genetic analysis of glucocorticoid signalling in development. J. Steroid Biochem. Mol. Biol. 53, 33–35. doi: 10.1016/0960-0760(95)00038-2
Schoepe, S., Schacke, H., May, E., and Asadullah, K. (2006). Glucocorticoid therapy-induced skin atrophy. Exp. Dermatol. 15, 406–420.
Shull, S., and Cutroneo, K. R. (1986). Glucocorticoids change the ratio of type III to type I procollagen extracellularly. Coll. Relat. Res. 6, 295–300. doi: 10.1016/s0174-173x(86)80013-9
Singh, N., Herbert, B., Sooranna, G., Das, A., Sooranna, S. R., Yellon, S. M., et al. (2019). Distinct preterm labor phenotypes have unique inflammatory signatures and contraction associated protein profilesdagger. Biol. Reprod. 101, 1031–1045. doi: 10.1093/biolre/ioz144
Skinner, S. J., Campos, G. A., and Liggins, G. C. (1981). Collagen content of human amniotic membranes: effect of gestation length and premature rupture. Obstet. Gynecol. 57, 487–489.
Sterry, W., and Asadullah, K. (2002). “Topical glucocorticoid therapy in dermatology,” in Recent Advances in Glucocorticoid Receptor Action. Ernst Schering Research Foundation Workshop, eds A. C. B Cato, H Schäcke, and K Asadullah, (Berlin: Springer) 39–54.
Stuart, E. L., Evans, G. S., Lin, Y. S., and Powers, H. J. (2005). Reduced collagen and ascorbic acid concentrations and increased proteolytic susceptibility with prelabor fetal membrane rupture in women. Biol. Reprod. 72, 230–235. doi: 10.1095/biolreprod.104.033381
Sun, K., He, P., and Yang, K. (2002). Intracrine induction of 11beta-hydroxysteroid dehydrogenase type 1 expression by glucocorticoid potentiates prostaglandin production in the human chorionic trophoblast. Biol. Reprod. 67, 1450–1455. doi: 10.1095/biolreprod.102.005892
Sun, K., Ma, R., Cui, X., Campos, B., Webster, R., Brockman, D., et al. (2003). Glucocorticoids induce cytosolic phospholipase A2 and prostaglandin H synthase type 2 but not microsomal prostaglandin E synthase (PGES) and cytosolic PGES expression in cultured primary human amnion cells. J. Clin. Endocrinol. Metab. 88, 5564–5571. doi: 10.1210/jc.2003-030875
Sun, K., and Myatt, L. (2003). Enhancement of glucocorticoid-induced 11beta-hydroxysteroid dehydrogenase type 1 expression by proinflammatory cytokines in cultured human amnion fibroblasts. Endocrinology 144, 5568–5577. doi: 10.1210/en.2003-0780
Sun, K., Qu, X., Gao, L., and Myatt, L. (2006). Dexamethasone fails to inhibit the induction of cytosolic phospholipase A(2) expression by interleukin-1beta in cultured primary human amnion fibroblasts. Placenta 27, 164–170. doi: 10.1016/j.placenta.2005.03.004
Sun, K., Yang, K., and Challis, J. R. (1997). Differential expression of 11 beta-hydroxysteroid dehydrogenase types 1 and 2 in human placenta and fetal membranes. J. Clin. Endocrinol. Metab. 82, 300–305. doi: 10.1210/jcem.82.1.3681
Sun, M., Ramirez, M., Challis, J. R., and Gibb, W. (1996). Immunohistochemical localization of the glucocorticoid receptor in human fetal membranes and decidua at term and preterm delivery. J. Endocrinol. 149, 243–248. doi: 10.1677/joe.0.1490243
Tai, H. H., Ensor, C. M., Tong, M., Zhou, H., and Yan, F. (2002). Prostaglandin catabolizing enzymes. Prostaglandins Other Lipid Mediat. 68–69, 483–493.
Tannin, G. M., Agarwal, A. K., Monder, C., New, M. I., and White, P. C. (1991). The human gene for 11 beta-hydroxysteroid dehydrogenase. Structure, tissue distribution, and chromosomal localization. J. Biol. Chem. 266, 16653– 16658.
Tanswell, A. K., Worthington, D., and Smith, B. T. (1977). Human amniotic membrane corticosteroid 11-oxidoreductase activity. J. Clin. Endocrinol. Metab. 45, 721–725.
Tomlinson, J. W., Moore, J., Cooper, M. S., Bujalska, I., Shahmanesh, M., Burt, C., et al. (2001). Regulation of expression of 11beta-hydroxysteroid dehydrogenase type 1 in adipose tissue: tissue-specific induction by cytokines. Endocrinology 142, 1982–1989. doi: 10.1210/endo.142.5.8168
Tomlinson, J. W., Walker, E. A., Bujalska, I. J., Draper, N., Lavery, G. G., Cooper, M. S., et al. (2004). 11beta-hydroxysteroid dehydrogenase type 1: a tissue-specific regulator of glucocorticoid response. Endocr. Rev. 25, 831–866. doi: 10.1210/er.2003-0031
Tong, M., and Tai, H. H. (2005). 15-Hydroxyprostaglandin dehydrogenase can be induced by dexamethasone and other glucocorticoids at the therapeutic level in A549 human lung adenocarcinoma cells. Arch. Biochem. Biophys. 435, 50–55. doi: 10.1016/j.abb.2004.11.031
Vater, C. A., Harris, E. D. Jr., and Siegel, R. C. (1979). Native cross-links in collagen fibrils induce resistance to human synovial collagenase. Biochem. J. 181, 639–645. doi: 10.1042/bj1810639
Wang, L. Y., Wang, W. S., Wang, Y. W., Lu, J. W., Lu, Y., Zhang, C. Y., et al. (2019a). Drastic induction of MMP-7 by cortisol in the human amnion: implications for membrane rupture at parturition. FASEB J. 33, 2770–2781. doi: 10.1096/fj.201801216R
Wang, W. S., Li, W. J., Wang, Y. W., Wang, L. Y., Mi, Y. B., Lu, J. W., et al. (2019b). Involvement of serum amyloid A1 in the rupture of fetal membranes through induction of collagen I degradation. Clin. Sci. 133, 515–530. doi: 10.1042/CS20180950
Wang, Y. W., Wang, W. S., Wang, L. Y., Bao, Y. R., Lu, J. W., Lu, Y., et al. (2019c). Extracellular matrix remodeling effects of serum amyloid A1 in the human amnion: Implications for fetal membrane rupture. Am. J. Reprod. Immunol. 81:e13073. doi: 10.1111/aji.13073
Wang, W., Chen, Z. J., Myatt, L., and Sun, K. (2018). 11beta-HSD1 in human fetal membranes as a potential therapeutic target for preterm birth. Endocr. Rev. 39, 241–260. doi: 10.1210/er.2017-00188
Wang, W., Guo, C., Li, W., Li, J., Wang, W., Myatt, L., et al. (2012). Involvement of GR and p300 in the induction of H6PD by cortisol in human amnion fibroblasts. Endocrinology 153, 5993–6002. doi: 10.1210/en.2012-1531
Wang, W., Guo, C., Zhu, P., Lu, J., Li, W., Liu, C., et al. (2015). Phosphorylation of STAT3 mediates the induction of cyclooxygenase-2 by cortisol in the human amnion at parturition. Sci. Signal. 8:ra106. doi: 10.1126/scisignal.aac6151
Wang, W., Liu, C., and Sun, K. (2016). Induction of amnion epithelial apoptosis by cortisol via tPA/Plasmin system. Endocrinology 157, 4487–4498. doi: 10.1210/en.2016-1464
White, P. C., Mune, T., and Agarwal, A. K. (1997a). 11 beta-Hydroxysteroid dehydrogenase and the syndrome of apparent mineralocorticoid excess. Endocr. Rev. 18, 135–156. doi: 10.1210/edrv.18.1.0288
White, P. C., Mune, T., Rogerson, F. M., Kayes, K. M., and Agarwal, A. K. (1997b). 11 beta-Hydroxysteroid dehydrogenase and its role in the syndrome of apparent mineralocorticoid excess. Pediatr. Res. 41, 25–29.
White, P. C., Mune, T., Rogerson, F. M., Kayes, K. M., and Agarwal, A. K. (1997c). Molecular analysis of 11 beta-hydroxysteroid dehydrogenase and its role in the syndrome of apparent mineralocorticoid excess. Steroids 62, 83–88. doi: 10.1016/s0039-128x(96)00164-x
Xun, C. Q., Ensor, C. M., and Tai, H. H. (1991). Regulation of synthesis and activity of NAD(+)-dependent 15-hydroxy-prostaglandin dehydrogenase (15-PGDH) by dexamethasone and phorbol ester in human erythroleukemia (HEL) cells. Biochem. Biophys. Res. Commun. 177, 1258–1265. doi: 10.1016/0006-291x(91)90677-y
Yang, K., Langlois, D. A., Campbell, L. E., Challis, J. R., Krkosek, M., and Yu, M. (1997). Cellular localization and developmental regulation of 11 beta-hydroxysteroid dehydrogenase type 1 (11 beta-HSD1) gene expression in the ovine placenta. Placenta 18, 503–509. doi: 10.1016/0143-4004(77)90003-0
Yang, Q., Wang, W., Liu, C., Wang, Y., and Sun, K. (2016). Compartmentalized localization of 11beta-HSD 1 and 2 at the feto-maternal interface in the first trimester of human pregnancy. Placenta 46, 63–71. doi: 10.1016/j.placenta.2016.08.079
Yang, Z., Guo, C., Zhu, P., Li, W., Myatt, L., and Sun, K. (2007). Role of glucocorticoid receptor and CCAAT/enhancer-binding protein alpha in the feed-forward induction of 11beta-hydroxysteroid dehydrogenase type 1 expression by cortisol in human amnion fibroblasts. J. Endocrinol. 195, 241–253. doi: 10.1677/JOE-07-0303
Yong, P. Y., Harlow, C., Thong, K. J., and Hillier, S. G. (2002). Regulation of 11beta-hydroxysteroid dehydrogenase type 1 gene expression in human ovarian surface epithelial cells by interleukin-1. Hum. Reprod. 17, 2300–2306. doi: 10.1093/humrep/17.9.2300
Zakar, T., Hirst, J. J., Mijovic, J. E., and Olson, D. M. (1995). Glucocorticoids stimulate the expression of prostaglandin endoperoxide H synthase-2 in amnion cells. Endocrinology 136, 1610–1619. doi: 10.1210/endo.136.4.7895671
Zhang, N., Wang, W., Li, W., Liu, C., Chen, Y., Yang, Q., et al. (2015). Inhibition of 11beta-HSD2 expression by triclosan via induction of apoptosis in human placental syncytiotrophoblasts. J. Clin. Endocrinol. Metab. 100, E542–E549. doi: 10.1210/jc.2014-4376
Zhu, X. O., Yang, Z., Guo, C. M., Ni, X. T., Li, J. N., Ge, Y. C., et al. (2009). Paradoxical stimulation of cyclooxygenase-2 expression by glucocorticoids via a cyclic AMP response element in human amnion fibroblasts. Mol. Endocrinol. 23, 1839–1849. doi: 10.1210/me.2009-0201
Ziboh, V. A., Lord, J. T., and Penneys, N. S. (1977). Alterations of prostaglandin E2-9-ketoreductase activity in proliferating skin. J. Lipid Res. 18, 37–43.
Keywords: 11β-HSD1, collagen, prostaglandins, glucocorticoids, fetal membranes
Citation: Wang W-S, Guo C-M and Sun K (2020) Cortisol Regeneration in the Fetal Membranes, A Coincidental or Requisite Event in Human Parturition? Front. Physiol. 11:462. doi: 10.3389/fphys.2020.00462
Received: 05 March 2020; Accepted: 16 April 2020;
Published: 25 May 2020.
Edited by:
Ramkumar Menon, The University of Texas Medical Branch at Galveston, United StatesReviewed by:
Hieu D. Hoang, Columbia University, United StatesAngela Leigh Cumberland, RMIT University, Australia
Steven M. Yellon, Loma Linda University School of Medicine, United States
Copyright © 2020 Wang, Guo and Sun. This is an open-access article distributed under the terms of the Creative Commons Attribution License (CC BY). The use, distribution or reproduction in other forums is permitted, provided the original author(s) and the copyright owner(s) are credited and that the original publication in this journal is cited, in accordance with accepted academic practice. No use, distribution or reproduction is permitted which does not comply with these terms.
*Correspondence: Kang Sun, c3VuZ2FuZ3JlbmppQGhvdG1haWwuY29t
†These authors have contributed equally to this work