- 1Department of Structural and Functional Biology, Institute of Biology, University of Campinas, Campinas, Brazil
- 2Institute of Biomedical Sciences, Area of Physiological Sciences, Federal University of Uberlândia, Uberlândia, Brazil
Peripheral inflammatory hyperalgesia depends on the sensitization of primary nociceptive neurons. Inflammation drives molecular alterations not only locally but also in the dorsal root ganglion (DRG) where interleukin-1 beta (IL-1β) and purinoceptors are upregulated. Activation of the P2X7 purinoceptors by ATP is essential for IL-1β maturation and release. At the DRG, P2X7R are expressed by satellite glial cells (SGCs) surrounding sensory neurons soma. Although SGCs have no projections outside the sensory ganglia these cells affect pain signaling through intercellular communication. Therefore, here we investigated whether activation of P2X7R by ATP and the subsequent release of IL-1β in DRG participate in peripheral inflammatory hyperalgesia. Immunofluorescent images confirmed the expression of P2X7R and IL-1β in SGCs of the DRG. The function of P2X7R was then verified using a selective antagonist, A-740003, or antisense for P2X7R administered in the L5-DRG. Inflammation was induced by CFA, carrageenan, IL-1β, or PGE2 administered in rat’s hind paw. Blockage of P2X7R at the DRG reduced the mechanical hyperalgesia induced by CFA, and prevented the mechanical hyperalgesia induced by carrageenan or IL-1β, but not PGE2. It was also found an increase in P2X7 mRNA expression at the DRG after peripheral inflammation. IL-1β production was also increased by inflammatory stimuli in vivo and in vitro, using SGC-enriched cultures stimulated with LPS. In LPS-stimulated cultures, activation of P2X7R by BzATP induced the release of IL-1β, which was blocked by A-740003. In summary, our data suggest that peripheral inflammation leads to the activation of P2X7R expressed by SGCs at the DRG. Then, ATP-induced activation of P2X7R mediates the release of IL-1β from SGC. This evidence places the SGC as an active player in the establishment of peripheral inflammatory hyperalgesia and highlights the importance of the events in DRG for the treatment of inflammatory diseases.
Introduction
In the nociceptive system, primary afferent neurons transduce the injury information at its peripheral endings and transmit the resulting signal to the central nervous system (CNS). At this path, the electric signal will pass through an important structure that is often ignored, the sensory ganglia, which can be a dorsal root ganglion (DRG) or trigeminal ganglia. At the sensory ganglia resides the soma of nociceptors and other somesthetic neurons as well as specialized glial cells, the satellite glial cells (SGC). Besides the important role in metabolic support, events at DRG are known to participate in the development of pathological pain (Devor, 1999; Esposito et al., 2019). Primary afferent neurons at the DRG do not interact with one another through synaptic contact. A layer of satellite glial cells (SGCs) tightly wrap each neuronal soma, which in turn is enclosed by connective tissue to create a physically isolated unit (Devor, 1999; Hanani, 2005; Pannese, 2010; Esposito et al., 2019). This singular structure suggests active participation of SGCs in the processing of sensory information by communicating with neurons (Hanani, 2005; Costa and Moreira Neto, 2015; Fan et al., 2019). Several studies have described intercellular communication in the sensory ganglia involving gap junctions (Huang et al., 2010), calcium-dependent somatic exocytosis of transmitters (Huang and Neher, 1996), and purinergic receptors also known as purinoceptors (Gu et al., 2010; Verderio and Matteoli, 2011). Purinergic signaling through ATP release at the DRG is suggested to mediate the development of chronic pain (for review see Kobayashi et al., 2013; Magni et al., 2018).
ATP is a ubiquitous molecule found in all tissues and cells and is released into the extracellular milieu in both physiological and pathological conditions (Fitz, 2007). Receptors activated by extracellular ATP include two families of the P2 purinoceptors: the ligand-gated ionotropic channels P2X and the metabotropic G protein-coupled P2Y receptors (Burnstock, 2016). Purinoceptors have a widespread tissue distribution, including peripheral (PNS) and CNS, and they are known to partake in pain mechanisms and inflammation (North, 2016; Magni et al., 2018). For example, activation of neuronal P2X3 receptors mediates acute nociception, and the microglial P2X4 and P2X7 receptors are associated with neuropathic and inflammatory pain (Toulme et al., 2010; Inoue and Tsuda, 2012; Fabbretti, 2013; Burnstock, 2016). Increasing evidence suggest ATP as a major transmitter released in the sensory ganglia (Zhang et al., 2007; Kobayashi et al., 2013; Goto et al., 2017). Therefore, the purinoceptors in DRG may have an important role boosting intercellular communication (Gu et al., 2010; Verderio and Matteoli, 2011; Huang et al., 2013), particularly for pain signaling (Jarvis, 2010; Hanani, 2012; Kobayashi et al., 2013; Lemes et al., 2018).
After peripheral tissue injury or inflammation, molecular alterations in the DRG are involved in the development of hyperexcitability of nociceptive neurons (Krames, 2014; Berta et al., 2017). Examples of those alterations include the upregulation of P2X receptors (Kushnir et al., 2011) and upregulation of the proinflammatory cytokine interleukin-1β (IL-1β) (Guo et al., 2007; Araldi et al., 2013). IL-1β was the first proinflammatory cytokine described to be involved in inflammatory pain and hyperalgesia (Ferreira et al., 1988). Several reports emphasize that a key process for IL-1β maturation and secretion is the ATP-induced activation of the purinergic P2X7 receptors (P2X7R), selectively expressed in macrophages and microglia (Ferrari et al., 2006; Clark et al., 2010; Stoffels et al., 2015; Giuliani et al., 2017). Within the P2X family, P2X7R has the highest threshold for ATP-induced activation and will trigger downstream mechanisms only when extracellular ATP reaches pathological concentration (North, 2002). Undoubtfully P2X7R stands out among other purinoceptors for playing a central role in several pathologic conditions (De Marchi et al., 2016), including inflammatory diseases (Savio et al., 2018) and neuropathic pain (Zhang et al., 2020). Besides, studies have reported that P2X7R are expressed in SGC within DRG (Kobayashi et al., 2005; Zhang et al., 2005), suggesting a role for visceral hyperalgesia (Liu et al., 2015) and acute nociception (Lemes et al., 2018). Therefore, in this study, we hypothesized that ATP-induced activation of P2X7R and the subsequent release of IL-1β by SGCs could be a major process in the DRG for the establishment of inflammatory hyperalgesia in the peripheral tissue.
Materials and Methods
Animals
Experiments were performed using 180–250 g Wistar rats obtained from the University of Campinas Multidisciplinary Center for Biological Investigation (UNICAMP-CEMIB). Animals were housed in plastic cages with soft bedding (four/cage) on a 12-h light/dark cycle (lights on at 06:00 A.M.), with controlled humidity (60–80%) and temperature (22–25°C). Food and water were available ad libitum. Experimental protocols were approved by the Committee on Animal Research of the University of Campinas (CEUA – UNICAMP, protocol number: 3022-1). Animal care and handling procedures were in accordance with International Association for the Study of Pain (IASP) guidelines for the use of animals in pain research (Zimmermann, 1983). A total of 90 rats (males) were used for in vivo experiments and molecular analysis, and other 10 animals (males and females) were used for the in vitro experiments. Based on previous studies from our group, in inflammatory models, pain sensitivity and cytokine expression change according to estrous cycle in females (Joseph et al., 2003; Torres-Chávez et al., 2011). However, sexual dimorphism is abolished upon removal of the hormonal factors. For this reason, we used cultures of DRG cells from both male and female rats. During the experiments, animals were simply randomized into treatments. All efforts were made to minimize animal discomfort and to reduce the number of animals used.
Hyperalgesia Induction
Complete Freund’s adjuvant (CFA 50 μL/paw, #F5881, Sigma Aldrich, St. Louis, MO, United States), λ-carrageenan (100 μg/paw, #22049, Sigma Aldrich, St. Louis, MO, United States), Interleukin 1 beta (IL-1β, 0.5 pg/paw, National Institute of Biological Standards and Control, South Mimms, Hertfordshire, United Kingdom) or PGE2 (100 ng/paw, #P5640, Sigma Aldrich, St. Louis, MO, United States) were administered subcutaneously (intraplantar) in the rat’s hind paw (right side) which is within the peripheral field of the L5 DRG (Araldi et al., 2013). The mechanical stimulus was then applied to the same area to measure hyperalgesia by electronic von Frey test.
In vivo Treatments
A potent selective antagonist for P2X7R (A-740003; Tocris Bioscience, Bristol, United Kingdom) was administrated in the L5 DRG (right side) immediately before intraplantar injection of the inflammatory agent (right hind paw). A-740003 was diluted in a vehicle solution of 10% dimethyl sulfoxide (DMSO) + 10% propylene glycol + 80% sterile saline (NaCl 0.9%) and administrated at doses of 0.01, 0.10, and 1.00 mM. The concentrations were calculated based on the effective antihyperalgesic dose of 142 mg/kg used for systemic administration (i.p.) in similar inflammatory pain-like behaviors models by Honore et al. (2006). For intraganglionar administration, using rats with approximately 0.2 kg, we calculated concentrations 10-, 100-, and 1000-times lower (0.028, 0.28, and 2.8 mg/6 μl), which corresponds to the doses of 0.01, 0.10, and 1.00 mM.
The antisense (AS) oligonucleotide (ODN) for P2X7R (TTTCCTTATAGTACTTGGC) or a mismatch sequence (MM, TTCCGTTAAAGAAGTAGGC) were diluted in sterile saline and administrated in the L5 DRG (right side, 30 μg/5 μl) once a day for 4 days to allow the knockdown of the P2X7R prior to the intraplantar injection of the inflammatory agent in the right hind paw. To demonstrate the relative expression of P2X7R was not altered solely by the repeated intraganglionar injections, we also used non-treated DRG (on the contralateral side of the inflammation) in the RT-qPCR analysis as a control for basal gene expression. All ganglionar treatments in this work were administered ipsilateral to the inflammation.
Ganglionar Drug Administration
The intraganglionar injection technique was performed as previously described (Ferrari et al., 2007; Araldi et al., 2013). Briefly, rats were anesthetized by inhalation of 2–3% isoflurane and an ultra-fine needle (32 G) was inserted through a punctured skin toward the intervertebral space between L5 and L6 vertebrae. Smooth movements of the needle were performed until a paw flinch reflex was observed and 5 μL of solution was injected. The paw-flinch reflex was used as a sign that the needle tip has reached the distal nerve insertion of the L5 DRG. This ganglionar administration is restricted to the injected L5 DRG and it does not reach the opposite ganglion, nor the spinal cord between L1-T13 segments (Oliveira et al., 2009).
Mechanical Hyperalgesia Evaluation by Electronic von Frey Test
The withdrawal threshold of the treated hind paw was measured using an electronic von Frey aesthesiometer (Insight, Ribeirão Preto, SP, Brazil) as previously described (Vivancos et al., 2004). All experiments were performed by the same experimenter blind to all treatments, between 9:00 AM and 4:00 PM. Rats were kept in a quiet room for 1 h prior to any manipulation. Then, each animal was placed in an acrylic cage (12 cm × 20 cm × 17 cm) with a wire grid floor providing access to the plantar surface of the rat’s hind paw. Animals were allowed to acclimate in cages for 20 min before the test. A polypropylene tip (10 μL, #T-300, Axygen, Corning, NY, United States) adapted to a hand-held force transducer was positioned perpendicularly to the plantar surface of the rat’s hind paw aiming the L5 peripheral field. Then, a gradually increasing pressure (80 g maximum) was applied until animal voluntarily withdraw its paw. This mechanical stimulus was repeated up to six times separated by a 1-min interval to prevent mechanical sensitization. The withdrawal threshold was defined as the average force (g) required to animals withdraw the stimulated paw in three consistently measurements (differences < 10%). Animals were tested before and after treatments and the results (intensity of hyperalgesia) are expressed as the variation of the mechanical threshold by subtracting the baseline values (obtained before treatment) from those obtained after the treatments (Δ mechanical threshold in grams).
Primary DRG Cultures
Dissociated DRG cell cultures were prepared as previously described (Linhart et al., 2003). Male Wistar rats (100 g) were euthanized under anesthesia and DRGs from lumbar and thoracic spine were harvested and transferred to Hank’s buffered saline solution (HBSS, #H2387, Sigma Aldrich, St. Louis, MO, United States) containing 10 mM Hepes. Cells were dissociated by incubating DRGs at 37°C in 0.28 U/mL collagenase (type II; #C6885, Sigma Aldrich, St. Louis, MO, United States) for 75 min and then in 0.25% trypsin (#T4549, Sigma Aldrich, St. Louis, MO, United States) for 12 min. After three washes with DMEM (#D5523, Sigma Aldrich, St. Louis, MO, United States) containing 10% fetal bovine serum (FBS, #10100147, Thermo Fisher Scientific, Waltham, MA, United States) and penicillin 50 U/mL + streptomycin 50 mg/mL (#15140122, Thermo Fisher Scientific, Waltham, MA, United States), cells were dissociated using a fire-polished glass Pasteur pipette. Dissociated cells were then plated in dishes coated with Matrigel (#354234, Corning, Corning, NY, United States) and the cultures were maintained in a humid 5% CO2 atmosphere at 37°C. Calcium experiments were performed after 3 days in vitro to allow the growth of satellite glial cells. The cultures media (DMEM + 10% FBS + 50 U/mL penicillin and 50 mg/mL streptomycin) was changed every other day.
Cell Culture Treatments for Calcium Imaging
Cultures were stimulated with 2′(3′)-O-(4-Benzoylbenzoyl)adenosine 5′-triphosphate triethylammonium salt (BzATP, #B6396, Sigma Aldrich, St. Louis, MO, United States), the main agonist for P2X7R (Donnelly-Roberts et al., 2009). BzATP was prepared 10-fold the final concentration (1 mM solution for 100 μM final concentration) in HBSS and administered directly in the buffered solution wherein the cultures were maintained during image acquisition. Some cultures were previously incubated with A-740003 (#3701, Tocris Bioscience, Bristol, United Kingdom), a selective antagonist of P2X7R (Honore et al., 2006) for 10 min. A-740003 was diluted at final concentrations (1 μM) in HBSS and maintained in cultures during agonist stimulus. To confirm cellular viability in those cultures with non-responsive cells after the incubation with the P2X7R antagonist, a stimulus of capsaicin (10 μM final concentration) was administered at the end of the experiment.
Intracellular Calcium Imaging
Calcium recordings were performed after 18–24 h of culture. Cells were loaded with the Ca2 + indicator Fluo-3 AM 10 μM (#F23915, Thermo Fisher Scientific, Waltham, MA, United States) in HBSS containing 10 mM Hepes for 1 h. After loading, cells were washed three times and kept with HBSS as described. All drugs were administered directly in buffered solution during image acquisition and the Ca2 + dynamics was recorded for 3 min after agonist addition. Fluorescence was excited at 506 nm and images of emitted fluorescence (at 526 nm) were acquired for each second by confocal microscope (Zeiss LSM510 Meta) in the Advanced Microscopy Center of ICBIM/UFU. Data are presented as ΔF/F0, where F0 is the baseline, to normalize for differences in cell loading. As the field of view contained 20–40 SGCs in the focal plane, recordings were made from several cells simultaneously.
SGC-Enriched Cultures
Satellite glial cells were isolated from DRG cell cultures (see section Primary DRG Cultures) using a protocol adapted from England et al. (2001), Castillo et al. (2013). DRG cell cultures were kept for 4 days in vitro when glial cells are numerous. To isolate SGCs, 1 mL of fresh culture media (DMEM + 10% FBS + 50 U/mL penicillin and 50 mg/mL streptomycin) was added and all cells were gently detached from the culture dishes using a fire-polished Pasteur pipette. The 1 mL of cell suspension was collected and centrifuged at 3000 g during 5 min at room temperature to remove debris. Then 250 μL of the cell suspension was plated again on new uncoated 24-well plates (1 DRG culture was split into 4 SGC-enriched cultures). The absence of coating prevents the attachment of neurons and does not affect attachment or growth of the glial cells (Capuano et al., 2009). Also, the culture media was changed 2 h after the cells plating to remove any cell (mostly neurons) in suspension. The SGC-enriched cultures were then maintained in a humid 5% CO2 atmosphere at 37°C for 5–7 days in vitro or until the SGCs have proliferated at a confluence up to 70%. Media was changed every other day.
Cell Culture Treatments for Cytokine Measurement
For the IL-1β synthesis and release experiments, SGCs were cultured in 24-well plates and an adapted protocol from Colomar et al. (2003), Rampe et al. (2004) was used to induce IL-1β synthesis in SGC-enriched cultures. Cultures were maintained with fresh medium (DMEM supplemented as previously described) or stimulated with lipopolysaccharides (LPS, 1 μg/mL; #L8274, Sigma Aldrich, St. Louis, MO, United States) for 24 h kept in a humid 5% CO2 atmosphere at 37°C. Some cultures were then incubated for 15 min with A74000 (1 μM) that was prepared 10-fold the final concentration and administered directly in cultures medium. After incubation, cultures were treated for 30 min with BzATP (100 μM) also prepared 10-fold the final concentration. All drugs were diluted in the culture medium. After treatments, the supernatant was collected and mixed with protease inhibitors (10 mM EDTA and 20 kl/ml aprotinine; Sigma Aldrich, St. Louis, MO, United States). Forthwith the cells were lysed with an ice-cold solution of Phosphate Buffer Saline (PBS) containing 0.1% Triton X-100 and protease inhibitors to obtain the cell lysates.
Enzyme-Linked Immunosorbent Assay (ELISA)
An adaptation of ELISA (Teixeira et al., 2010) was used to determine whether P2X7R was able to regulate the synthesis and releasing of IL-1β by SGCs. The cells lysates or the supernatant were centrifuged at 10,000 rpm for 15 min at 4°C, and the supernatants were stored at −80°C for posterior use to evaluate the protein levels of IL-1β. IL-1β was quantified by Rat IL-1β/IL-1F2 DuoSet ELISA Kit (#DY501, R&D Systems, Minneapolis, MN, United States) and all procedures followed the instructions of the manufacturer. All samples were running in duplicates and procedures were repeated at least twice.
DRG Immunohistochemistry
Animals were terminally anesthetized with ketamine (i.p. 85 mg/kg)/xylazine (i.p. 10 mg/kg) and perfused through the ascending aorta with saline solution followed by 4% paraformaldehyde (PFA, pH 7.4, 4°C). After perfusion, L5 DRG were removed and post-fixed in 4% PFA for 2 h, which was then replaced with 30% sucrose for 48 h at 4°C. Each DRG were embedded in optimum cutting temperature and sections of 14 μm were made in a cryostat using gelatinized slides. The sections were processed for immunofluorescence, first incubated in 0.1 M glycine for 30 min at room temperature, to inactivate free aldehydes, and then blocked with 2% BSA in 0.2% Triton X-100 for 1 h at room temperature. The mixture of rabbit polyclonal anti-P2X7R (1:200, #APR-004, Alomone Labs, Jerusalem, ISR), goat polyclonal anti-TRPV1 (1:100, #AF3066, R&D Systems, Minneapolis, MN, United States) and mouse monoclonal anti-glutamine synthetase (1:200, #MAB302, EMD Millipore, Darmstadt, DEU) were incubated for 2 h at room temperature. A mixture of mouse monoclonal anti-GFAP (1:100, #G3893, Sigma Aldrich, St. Louis, MO, United States) and goat polyclonal anti-IL-1β (1:100, #AF-501-NA, R&D Systems, Minneapolis, MN, United States) was incubated overnight at 4°C. Finally, a mixture of Alexa 488 (A21206), Alexa 546 (A11058) and Alexa 594 (A11058) conjugated secondary antibodies (1:1000 each, Thermo Fisher Scientific, Waltham, MA, United States) were incubated for 1 h at room temperature and then nuclei were stained with DAPI 0.25 μg/mL (#D9542, Sigma Aldrich, St. Louis, MO, United States) for 10 min. Sections were examined in the National Institute of Science and Technology in Photonics Applied to Cell Biology (INFABIC/UNICAMP) using a confocal laser-scanning microscope (Zeiss LSM780-NLO).
RNA Extraction, cDNA Synthesis, and qPCR Experiment
RNA was extracted from whole DRG tissue by TRIZOL® Reagent (#15596026, Thermo Fisher Scientific, Waltham, MA, United States) according to manufacturer instructions. Subsequently, total RNA was quantified by ultra-low volume spectrophotometer (Epoch Microplate Spectrometer, BioTek Instruments, Winooski, VT, United States) and 500 ng of RNA were used to perform cDNA synthesis (SuperScript® VILO cDNA Synthesis Kit, #11755, Thermo Fisher Scientific, Waltham, MA, United States). Real-Time PCR (RT-qPCR) experiment was performed for relative quantification of P2X7R and Il-1β to an index of endogenous control genes (Arfgef1, G3bp2, Mtm8, Ppp1cc, Serpinb6) by 2−ΔΔCT method (see Table 1 for the primer sequences). The RT-qPCR reactions were conducted using SYBR Green as a fluorescent signal (SYBR Power Up Master Mix, #4367659, Thermo Fisher Scientific, Waltham, MA, United States). Primers were designed using Primer3 software available at NCBI’s Pick Primer tool1 between exons when possible and its specificity to the gene of interest was validated by comparison with the rat genome using BLAST tool from NCBI webpage1. Technical triplicates for all samples as well as negative controls were used for each gene.
Statistical Analysis
The statistical analysis was performed using Prism v.6 (GraphPad Software Inc., La Jolla, CA, United States). Statistical significance between groups (defined as P < 0.05) was calculated by one-way ANOVA and Bonferroni post hoc test, otherwise by using the two-tailed Student’s t-test between two groups. Sample sizes are indicated in the figure legends and in the text. Data in the figures are expressed as mean ± S.E.M. The long line above two or more data sets indicates no statistical difference between those groups. The symbol (#) indicates statistical difference in comparison to saline-treated group (hind paw treatments). The symbol (∗) indicates statistical difference in comparison to vehicle-treated group (DRG treatments; ∗P < 0.05; ∗∗P < 0.01; ∗∗∗P < 0.001).
Results
The Selective Blockade of P2X7R in DRG Reduces the Mechanical Hyperalgesia Induced by CFA, Carrageenan, and IL-1β, but Not PGE2
To evaluate the participation of P2X7R expressed in DRG during the development of peripheral inflammatory hyperalgesia, different doses of the selective P2X7R antagonist A-740003 (0.01 mM, 0.1 mM or 1.0 mM/5 μL) were administered in the right L5 DRG immediately before intraplantar administration of CFA (50 μL/paw) in the ipsilateral hind paw. The electronic von Frey test was conducted 6 h later to measure the mechanical hyperalgesia. As shown in Figure 1A, A-740003 decreased the CFA-induced mechanical hyperalgesia compared to vehicle-treated group (One-way ANOVA and Bonferroni post-test; F = 11.84, P < 0.001, n = 6). Also, the intraganglionar administration of A-740003 (0.1 mM/5 μL) in animals treated with saline intraplantar did not change the mechanical nociceptive threshold, which excludes any alterations in the mechanical baseline. Thus, this dose was used in the following experiments. To confirm the selective effect of A-740003 (0.1 mM) on other models of inflammatory hyperalgesia, we administrated carrageenan (100 μg/paw), IL-1β (0.5 pg/paw) or PGE2 (100 ng/paw) in the right hind paw and measured the mechanical hyperalgesia 3 h later. A-740003 (0.1 mM/5 μL) administered in the ipsilateral L5 DRG immediately before the inflammatory stimuli prevented the hyperalgesia induced by carrageenan and IL-1β (Figures 1B,C; Unpaired t-test; t = 23.35 and t = 5.89 respectively, P < 0.001, n = 6), but did not affect the hyperalgesia induced by PGE2 (Figure 1D; Unpaired Student’s t-test; t = 0.23, P = 0.816, n = 6).
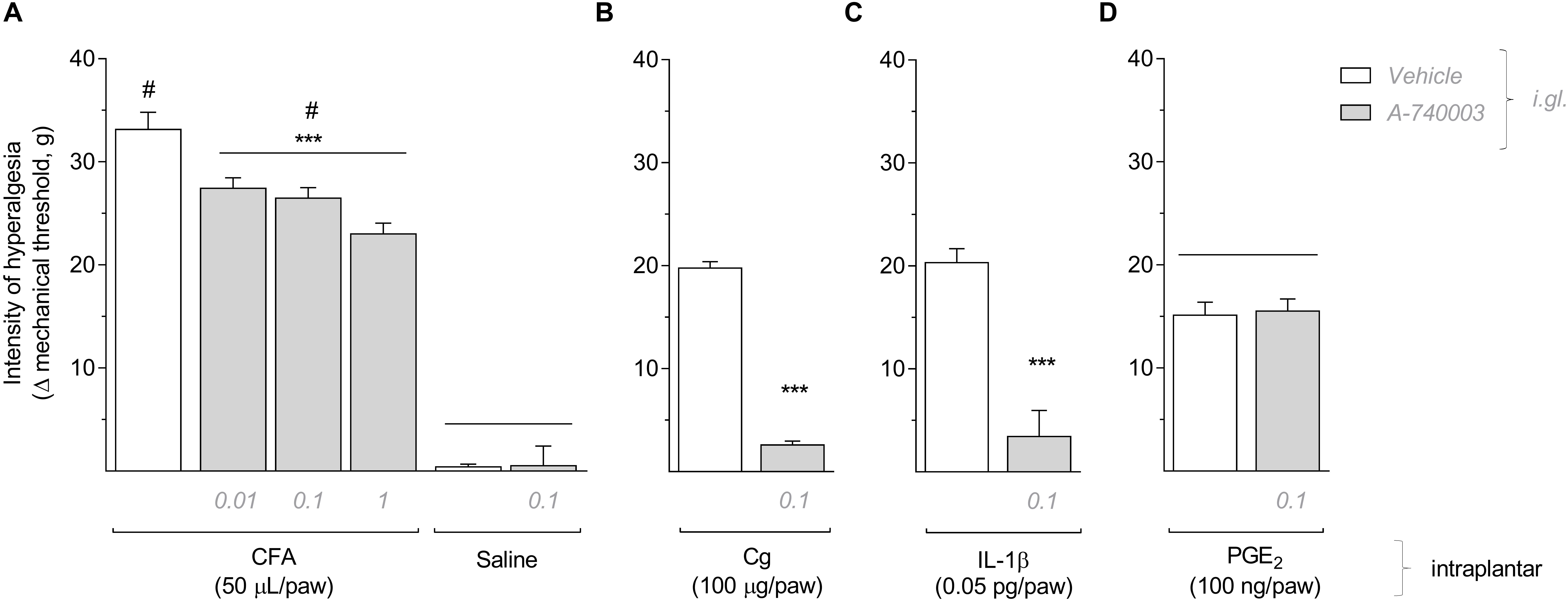
Figure 1. Selective blockade of P2X7R expressed on satellite glial cells reduced the mechanical hyperalgesia induced by CFA, Carrageenan or IL-1b, but not PGE2. (A) A-740003 (0.01, 0.1, and 1.0 mM/5 μL) administered in DRG (i.gl.) immediately before intraplantar administration of CFA significantly reduced the mechanical hyperalgesia evaluated 6 h later (One-way ANOVA and Bonferroni post-test; P < 0.001, n = 6 animals). The intraganglionar administration of A-740003 (0.1 mM/5 μL) in saline-treated animals did not change the mechanical baseline threshold. (B) Mechanical hyperalgesia evaluated 3 h after intraplantar administration of Carrageenan (Cg) or IL-1b (C) was prevented by the treatment with A-740003 (0.1 mM/5 μL) in DRG (Student’s t-test; P < 0.001, n = 6 animals), but it did not affect PGE2-induced mechanical hyperalgesia (D). Data are expressed as mean ± SEM. A line above two data sets indicates no statistical difference between those groups; (#) indicates statistical difference comparing to the saline group; (***) indicates statistical difference comparing to the vehicle solution group.
Knockdown of the P2X7R in DRG Reduces the Mechanical Hyperalgesia Induced by CFA
To knock down the expression of P2X7R in the DRG, we administered an antisense ODN for P2X7R or a mismatch sequence (MM), as control, in the right L5 DRG (30 μg/5 μl) once a day for 4 days before CFA injection (50 μL/paw) in the ipsilateral hind paw. Similarly to the selective blockade with A-740003, the P2X7R knockdown using the antisense (AS) also significantly reduced the mechanical hyperalgesia, assessed 6 h after CFA injection, when compared to the MM treatment (Figure 2A; One-way ANOVA and Bonferroni post-test; F = 331.9, P < 0.001, n = 6). To confirm the P2X7R knockdown, we analyzed the levels of P2X7R expression by RT-qPCR in all treated DRG (AS, MM, or saline) and in non-treated DRG (contralateral) as a control for basal expression (Figure 2B). For this 2−ΔΔCT analysis, we used an index of endogenous controls (Serpinb6, Mtmr9, Arfgef1, and G3bp2). The relative expression of P2X7R in the AS-treated DRG was significantly reduced compared to the MM treatment. However, in the MM-treated DRG the expression of P2X7R was already 4-fold increased after 6 h of the CFA inflammation (One-way ANOVA and Bonferroni post-test; F = 58.14, P < 0.001, n = 5). The expression of P2X7R in the saline-treated DRG did not differ from the basal expression in the contralateral DRG. This result demonstrates the P2X7R expression was not altered solely by the repeated intraganglionar injections.
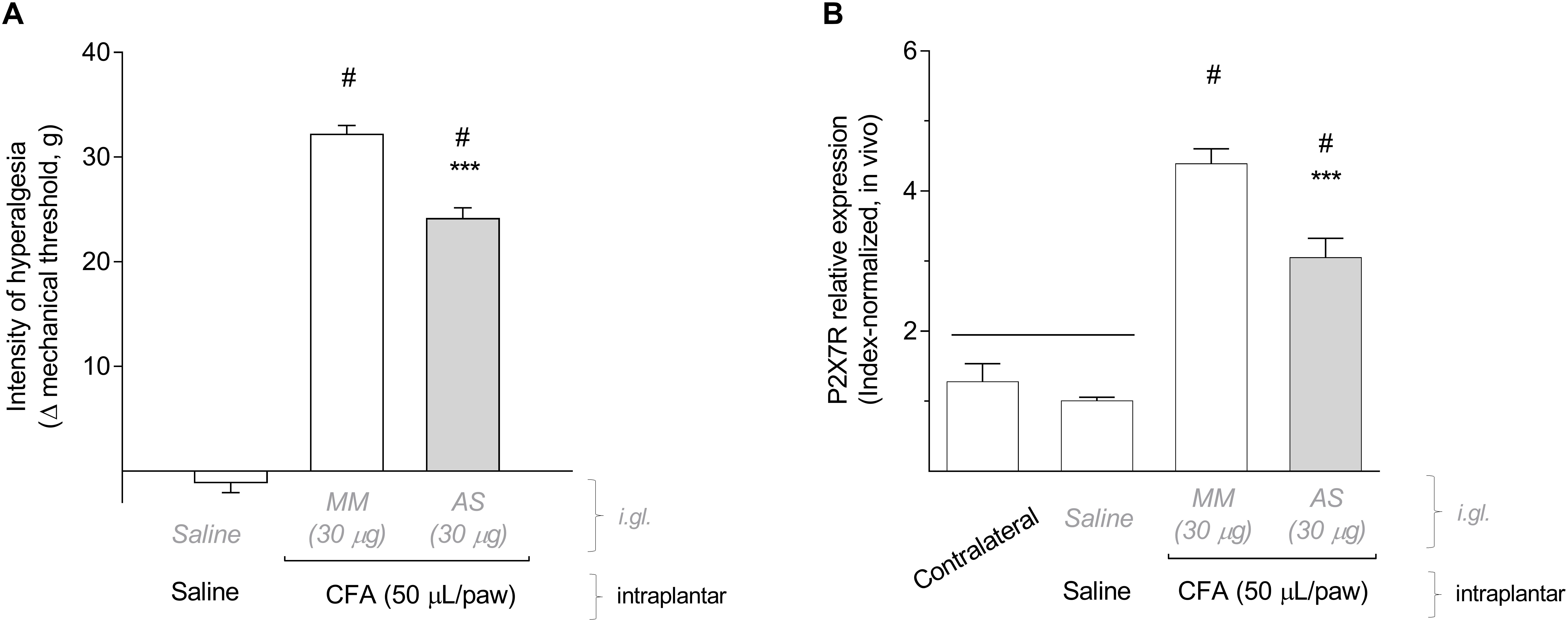
Figure 2. The knockdown of P2X7R in DRG reduced the mechanical hyperalgesia induced by CFA. (A) Antisense ODN (AS) for P2X7R (30 mg/5 ml daily for 4 days) administered in DRG before intraplantar administration of CFA significantly reduced the mechanical hyperalgesia assessed 6 h later (One-way ANOVA and Bonferroni post-test; P < 0.001, n = 6 animals). (B) Treatment with antisense ODN (AS) significantly reduced the CFA-induced upregulation of P2X7R expression in the injected DRG. Relative expression of P2X7R in the saline-treated DRGs did not differ from the contralateral non-treated DRGs (One-way ANOVA and Bonferroni post-test; P < 0.001, n = 5 animals). Data are expressed as mean ± SEM. A line above two data sets indicates no statistical difference between those groups; (#) indicates statistical difference comparing to the control groups; (***) indicates statistical difference comparing to the mismatch group (MM).
P2X7R Are Expressed by Satellite Glial Cells in DRG and Can Be Functionally Activated and Blocked in vitro
As shown in Figure 3A, the immunohistochemistry co-staining P2X7R and glutamine synthetase (GS, a glial-specific enzyme) in paraformaldehyde-fixed rat DRG sections confirmed that P2X7R are expressed on SGCs, but not on DRG neurons, after CFA inflammation. Expression of P2X7R in non-inflammatory conditions is very low (as shown in Figure 2B) and hardly detected by immunohistochemistry of the DRG (data not shown). To check P2X7R activation and blockade in SGCs, we evaluated changes in intracellular calcium concentration by confocal microscopy using mixed DRG cell cultures from adult rats. The P2X7R was activated by its agonist BzATP (100 μM) which increased the intracellular calcium concentration in SGCs but not in neurons (Figure 3B). The incubation with P2X7R selective antagonist A-740003 (1 μM), added to cultures 5 min before BzATP, blocked the BzATP-induced intracellular calcium surge in SGCs (Figures 3C,D; Unpaired Student’s t-test; t = 10.70, P < 0.001, n = 5 cultures/group, 20–40 SGCs sampled per culture).
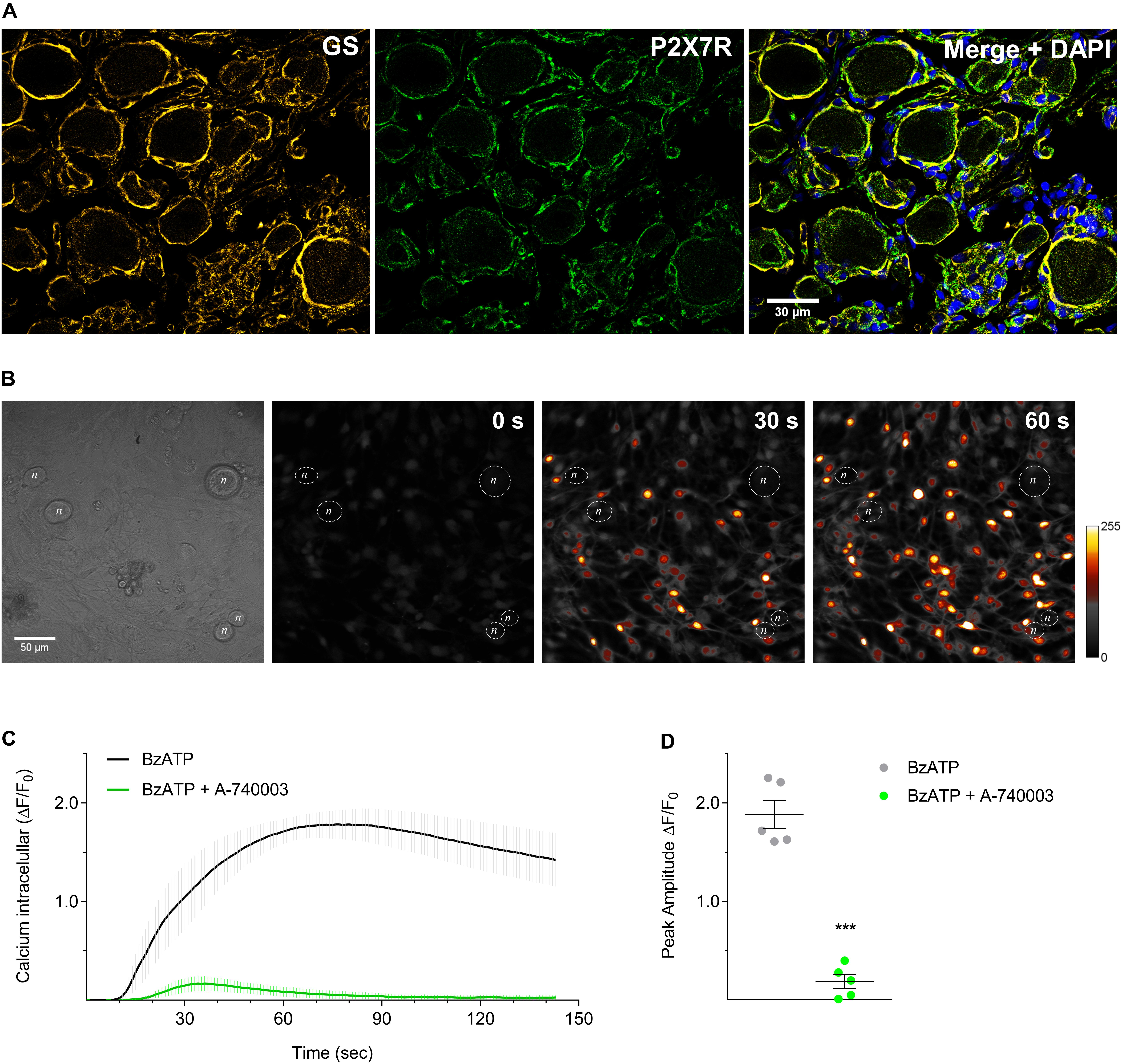
Figure 3. P2X7R are expressed by SGCs in DRG and can be functionally activated and blocked in culture. (A) Co-staining of glutamine synthetase (GS, yellow) and P2X7R (green) in DRG sections of CFA-treated animals. In non-inflammatory condition, expression of P2X7R is very low and hardly detected by DRG immunohistochemistry. The merged picture shows co-localization of P2X7R and glutamine synthetase in SGCs surrounding neurons, and cell nuclei were staining with DAPI (blue). (B) BzATP (100 μM) induced intracellular calcium surge in cultured SGCs (colored artificially) but not in neurons (n). (C) Pretreatment with A-740003 (1 μM, green line) blocked the BzATP-induced intracellular calcium surge (gray line). (D) Maximum values of ΔF/F0 for both response curves showed in (C). Data are expressed as mean ± SEM for n = 5 cultures/group, 20–40 SGCs sampled per culture. Symbol *** indicates statistical difference between the response peaks (Unpaired t-test; P < 0.001).
Activation of P2X7R Increases the Release but Not the Synthesis of IL-1β in Satellite Glial Cells After an Inflammatory Stimulus
To confirm SGCs express IL-1β, we performed an immunohistochemistry co-staining IL-1β (both precursor and mature forms) and glutamine synthetase (GS) in paraformaldehyde-fixed rat DRG sections. Our results showed that IL-1β is expressed on SGCs after CFA-induced inflammation (Figure 4A), in addition to P2X7R (Figure 3A). To evaluate whether the release of IL-1β in DRG is regulated by activation of P2X7R during the peripheral inflammation, we used an in vitro approach. SGC-enriched cultures isolated from DRG were maintained with fresh culture medium (controls) or stimulated with LPS (1 μg/mL) for 24 h. Cultures were then incubated with A-740003 (1 μM) for 15 min followed by treatment with BzATP (100 μM) for 30 min. Supernatant and cell lysates were collected and the levels of IL-1β were measured by ELISA. Our data show P2X7R activation by BzATP without an inflammatory stimulus did not induce IL-1β release by SGCs (Figure 4B, white bars). However, after the LPS inflammatory stimulation, the release of IL-1β was induced into the supernatant of SGCs cultures. Then, P2X7R activation by BzATP significantly increased the release of IL-1β by LPS-stimulated SGCs cultures, which was prevented by the P2X7R blockade with A-740003 (Figure 4B, colored bars; One-way ANOVA and Bonferroni post-test; F = 16.60; P < 0.001, n = 5).
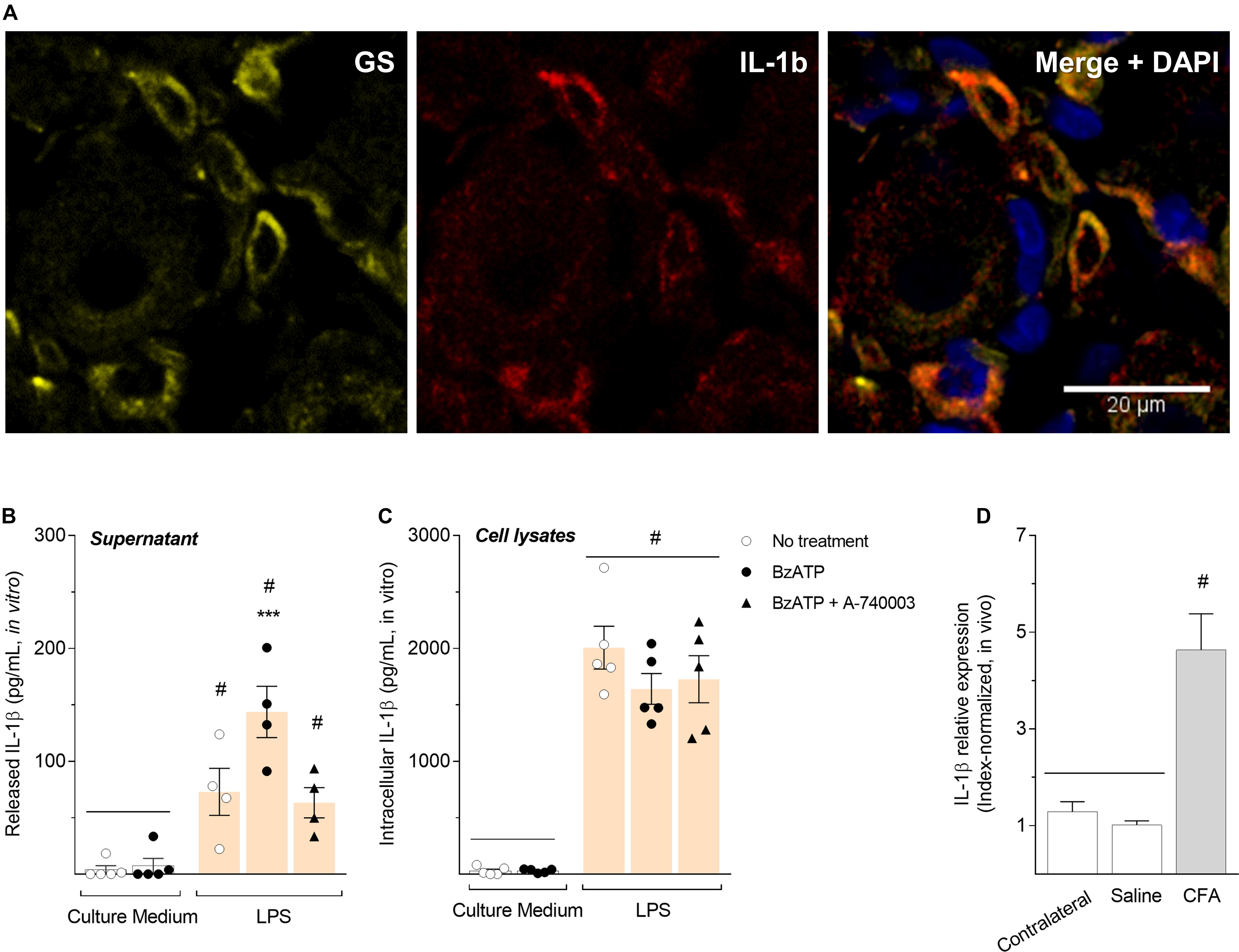
Figure 4. Activation of P2X7R increased the release but not the synthesis of IL-1β in satellite glial cells after an inflammatory stimulus. (A) Co-staining of glutamine synthetase (GS, yellow) and IL-1β (red) in DRG sections. In non-inflammatory condition, expression of IL-1β is very low and hardly detected by DRG immunohistochemistry. The merged picture shows co-localization of IL-1β and glutamine synthetase in SGCs surrounding neurons, and cell nuclei were staining with DAPI (blue). (B) SGC-enriched cultures were stimulated with LPS (1 μg/mL) for 24 h and then incubated with A-740003 (1 μM) for 15 min followed by treatment with BzATP (100 μM) for 30 min. LPS induced the release of IL-1β in the supernatant of SGCs cultures, but BzATP significantly increased the release of IL-1β by SGCs, which was prevented with A-740003. (C) IL-1β synthesis is increased in vitro only in LPS-stimulated cultures, regardless of P2X7R activation or blockade, and (D) in vivo after 6 h of the CFA inflammation in the ipsilateral hind paw. IL-1β relative expression in the contralateral paw had no difference from the saline group. Data are expressed as mean ± SEM. Symbol # indicates statistical difference comparing to control groups and *** indicates statistical difference comparing to LPS-stimulated groups [One-way ANOVA and Bonferroni post-test; P < 0.001, n = 4-5 cultures in (B,C), n = 5 animals in (D)].
Further, we investigated the intracellular levels of IL-1β in the SGCs cultures, to test whether activation of P2X7R is relevant also for IL-1β synthesis. Our results show that intracellular levels of IL-1β were only increased with the LPS inflammatory stimulation, regardless of P2X7R activation or blockade (Figure 4C; One-way ANOVA and Bonferroni post-test; F = 48.12, P < 0.001, n = 5). We also analyzed the expression of IL-1β by RT-qPCR in the L5-DRG of rats treated with CFA (50 μL/paw) or saline, along with non-treated DRG (contralateral) as a control for basal expression. The relative expression of IL-1β was also increased in vivo after 6 h of the CFA inflammation (Figure 4D; One-way ANOVA and Bonferroni post-test; F = 20.05, P < 0.001, n = 5). The expression of IL-1β in the saline-treated DRG did not differ from the basal expression in the contralateral DRG; both levels were very low and hardly detected also by DRG immunohistochemistry (data not shown).
Discussion
Although satellite glial cells (SGCs) have no projections outside the sensory ganglia, in this study, we detected a major process occurring at the dorsal root ganglion (DRG) involving the active participation of SGCs in the development of peripheral inflammatory hyperalgesia. Peripheral inflammatory hyperalgesia is the sensitization of the peripheral terminals of nociceptors due to inflammation. It has been established that administration of inflammatory agents such as the complete Freund’s adjuvant (CFA), carrageenan, or lipopolysaccharide (LPS), leads to an upregulation of proinflammatory mediators, including interleukin-1 beta (IL-1β), in the local tissue (Cunha et al., 2000; Verri et al., 2006; Ren and Torres, 2009). IL-1β as a mediator of the inflammatory hyperalgesia induces the upregulation of cyclooxygenase-2 (COX-2) and stimulates the subsequent release of COX products, such as prostaglandin E2 (PGE2). PGE2 is the main mediator responsible for nociceptive sensitization that causes inflammatory hyperalgesia in both peripheral (Ferreira, 1981; Verri et al., 2006) and the CNS (Samad et al., 2001).
Using a technique to administer drugs directly into the DRG (right L5), we selectively inhibited P2X7R activation in SGC by injecting the selective P2X7R antagonist A-740003 (Honore et al., 2006) or the antisense oligonucleotides knocking down P2X7R expression. The selective blockade of P2X7R expressed on SGCs prevented the mechanical hyperalgesia induced by carrageenan or IL-1β. Also, P2X7R blockade or knockdown significantly reduced the mechanical hyperalgesia assessed 6 h after the CFA administration. Undoubtfully, P2X7R stands out among other purinergic receptors for playing a central role in inflammatory and neuropathic pain-like behavior (Dell’Antonio et al., 2002; Chessell et al., 2005; McGaraughty et al., 2007; Carroll et al., 2009; Honore et al., 2009; Arulkumaran et al., 2011; Luchting et al., 2016; and for review see Savio et al., 2018; Zhang et al., 2020). Besides, studies suggest that P2X7R expressed particularly in DRG have a role for the neuropathic pain-like behavior associated with HIV glycoprotein 120 (Wu et al., 2017), visceral hyperalgesia (Liu et al., 2015), and acute nociception (Lemes et al., 2018). In the present study, we describe for the first time the active participation of P2X7R expressed on SGCs on the establishment of peripheral inflammatory hyperalgesia.
However, we have shown that the selective blockade of P2X7R in DRG did not affect the PGE2-induced hyperalgesia. As a final inflammatory mediator, PGE2 acts on EP receptors and directly stimulates primary afferent neurons. Thus, the administration of PGE2 at a pharmacological dose of 100 ng/paw induces hyperalgesia (Prado et al., 2013). But the model of PGE2 inducing hyperalgesia lacks important upstream events, such as the IL-1β signaling, which is a shared event between the other models of hyperalgesia we used – CFA, carrageenan, and IL-1β (Cunha et al., 2000; Ren and Torres, 2009). In addition to inducing the synthesis of PGE2, IL-1β can also play as a final inflammatory mediator acting at nociceptors, a mechanism that substantially increases neuronal excitability in pain signaling (Cunha et al., 2000; Binshtok et al., 2008; Dinarello, 2018). Therefore, we suggest that a mechanism dependent on IL-1β, but not prostaglandins, could be promoting the somatic release of ATP in DRG and leading to the activation of P2X7R on SGCs.
Our results revealed the constitutive expression of P2X7R in DRG, and the immunofluorescence confirmed the expression of P2X7R in SGCs. However, P2X7R expression was considerably increased (4-fold) after the intraplantar administration of CFA. Other studies, mostly in neuropathic pain-like behavior models, also detected upregulation of P2X receptors in trigeminal SGCs (Kushnir et al., 2011) and specifically upregulation of P2X7R in spinal microglia (Kobayashi et al., 2011; He et al., 2012; Li et al., 2017), and Schwan cells (Song et al., 2015). ATP-induced purinergic signaling is used by glial cells for intercellular communication in both central (Butt, 2011; Verderio and Matteoli, 2011; Puerto et al., 2013) and peripheral nervous system (Gu et al., 2010; Hanani, 2012; Huang et al., 2013). In neurons, ATP accumulates in vesicles near the presynaptic membrane and is released by exocytosis as a neurotransmitter (Burnstock, 2006; Lazarowski, 2012). Particularly in the sensory ganglia, studies have already suggested that vesicles holding ATP can be released by the cell body of sensory neurons following activation with capsaicin (Matsuka et al., 2001) or electrical stimulation (Zhang et al., 2007). Increasing evidence show ATP as a major transmitter released in the sensory ganglia (for review see Kobayashi et al., 2013; Goto et al., 2017); and intercellular communication via purinergic signaling appears to be an essential mechanism for pain (Ding et al., 2000; Toulme et al., 2010; Kobayashi et al., 2013; Burnstock, 2016; Lemes et al., 2018; Magni et al., 2018). Therefore, the upregulation of P2X7R in SGCs could be relevant for boosting intercellular communication in DRG during inflammatory hyperalgesia.
A potential role for P2X7R from SGCs during inflammatory hyperalgesia is the regulation of IL-1β release in DRG. IL-1β is produced from an inactive precursor protein, pro-IL-1β (31 kDa), that remains accumulated at the intracellular compartment. For the IL-1β maturation, a multiprotein complex called inflammasomes is recruited by mechanisms yet not fully understood to cleave the pro-IL-1β into the active form (17 kDa) and ensure its following secretion (Brough and Rothwell, 2007; Garlanda et al., 2013; Malik and Kanneganti, 2017). Several reports emphasize that ATP-mediated P2X7R activation is essential for rapid maturation and release of IL-1β from activated immune cells, including macrophages and microglial cells (Ferrari et al., 2006; Clark et al., 2010; Stoffels et al., 2015; Giuliani et al., 2017). In the present study, we also verified a connection between P2X7R activation and IL-1β release from SGCs after inflammatory stimulation. In SGC-enriched cultures treated with LPS, the activation of P2X7R by BzATP induced the release of IL-1β in the supernatant, which was blocked by A-740003. However, the activation of P2X7R without the inflammatory stimulus did not provoke the release of IL-1β or the synthesis of the pro-IL-1β. These results might indicate the involvement of caspases proteins which participates in the IL-1β maturation (Maelfait et al., 2008).
We found that SGCs activated by the inflammatory stimuli show an increased expression of IL-1β both in vivo and in vitro through a mechanism independent of P2X7R activation. Likewise, other studies have also reported the upregulation of IL-1β in SGCs in consequence of peripheral inflammation or nerve injury (Guo et al., 2007; Takeda et al., 2007; Araldi et al., 2013). Although P2X7R activation has a critical role on IL-1β release, there is no evidence on P2X7R modulation on the expression of the Il-1β precursor (Chessell et al., 2005; Clark et al., 2010; Giuliani et al., 2017). The synthesis of pro-IL-1β is primarily induced by pathological conditions and normally involves activation of toll-like receptors (TLRs) (Dinarello, 2018). In our in vitro approach, LPS itself could be triggering the synthesis of the IL-1β precursor in SGCs through direct stimulation of the toll-like receptors 4 (TLR4) (Tse et al., 2014). However, further investigation is required to understand the mechanism underlying the increase of pro-IL-1β synthesis in DRG in vivo. Possibly, different neuronal mediators might be involved to induce IL-1β synthesis in SGC. Sensory neurons in response to electrical or chemical stimulation can release substance P or glutamate concurrent with ATP (Matsuka et al., 2001; Dissing-Olesen et al., 2014). Also, trigeminal cells in culture stimulated with IL-1β release calcitonin gene-related peptide (CGRP) through a mechanism dependent on COX-2-induced PGE2 synthesis (Neeb et al., 2011). Because SGCs have receptors for CGRP (De Corato et al., 2011), substance P (Matsuka et al., 2001) and NMDA (Ferrari et al., 2014), these neuronal mediators could also participate in neuron-glia signaling in the DRG.
In summary, our study has provided evidence for a specific role of P2X7R in neuronal soma-glia communication associated with the regulation of the IL-1β release in DRG during peripheral hyperalgesia. Our data suggest that inflammatory mediators released at the peripheral level lead to ATP release at the DRG, activating P2X7R at SGC and triggering the release of IL-1β. At the DRG, IL-1β was shown to upregulate cyclooxygenase activity and thus inducing prostaglandin production (Araldi et al., 2013). Prostaglandins are responsible for neuronal sensitization, contributing to the development and maintenance of the inflammatory hyperalgesia. Therefore, peripheral inflammation appears to induce a further inflammatory response at the sensory ganglia that might maintain or amplify neuronal sensitization, increasing pain-like behavior.
This study draws attention to the sensory ganglia as an important pharmacological target and can be used to support the development of new treatments for inflammatory diseases. We have described a pharmacological mechanism of analgesia that is independent of COX inhibition and targets structures outside the CNS. Also, the ganglionic injection is a technique, per se, translational. In humans, it has been used to treat mainly patients with chronic pain (Sapunar et al., 2012). Here we used this technique as a methodological tool to investigate hyperalgesia-related mechanisms restrict to the DRG. It’s noteworthy that the DRG is only partially covered by the dura mater, thus showing good bioavailability to drugs acting in the peripheral nervous system (Esposito et al., 2019). Therefore, DRG could be targeted by the systemic administration of drugs that do not cross the blood-brain barrier. Treatments using those classes of drugs would have reduced side effects without the limitations of using ganglionic injections.
Data Availability Statement
All datasets generated for this study are included in the manuscript.
Ethics Statement
The animal study was reviewed and approved by Committee on Animal Research of the University of Campinas (3022-1).
Author Contributions
AN, CL, and CP conceived the project and designed the study. AN, FF, and SM acquired the data. AN, DA, CT, CS, and CL analyzed and interpreted the data. AN, MP, CL, and CP drafted the manuscript. AN, FF, SM, DA, MP, CT, CS, CL, and CP approved the final version to be submitted.
Funding
This study was supported by São Paulo Research Foundation (FAPESP) grant #2013/08678-6 and by the Coordination for the Improvement of Higher Education Personnel (CAPES – Brazil), Finance Code 001.
Conflict of Interest
The authors declare that the research was conducted in the absence of any commercial or financial relationships that could be construed as a potential conflict of interest.
Acknowledgments
We thank the access to equipment and assistance provided by the Advanced Microscopy Center at the Institute of Biomedical Sciences (ICBIM), Federal University of Uberlândia, and by the National Institute of Science and Technology on Photonics Applied to Cell Biology (INFABIC) at the State University of Campinas (INFABIC is co-funded by FAPESP #08/57906-3 and CNPq 573913/2008-0). We also thank Willians F. Vieira, Ph.D. and Catarine M. Nishijima, Ph.D. for their expertise and assistance throughout our study.
Footnotes
References
Araldi, D., Ferrari, L. F., Lotufo, C. M., Vieira, A. S., Athie, M. C., Figueiredo, J. G., et al. (2013). Peripheral inflammatory hyperalgesia depends on the COX increase in the dorsal root ganglion. Proc. Natl. Acad. Sci. U.S.A. 110, 3603–3608. doi: 10.1073/pnas.1220668110
Arulkumaran, N., Unwin, R. J., and Tam, F. W. (2011). A potential therapeutic role for P2X7 receptor (P2X7R) antagonists in the treatment of inflammatory diseases. Expert Opin. Investig. Drugs 20, 897–915. doi: 10.1517/13543784.2011.578068
Berta, T., Qadri, Y., Tan, P.-H., and Ji, R.-R. (2017). Targeting dorsal root ganglia and primary sensory neurons for the treatment of chronic pain. Expert Opin. Ther. Targets 21, 695–703. doi: 10.1080/14728222.2017.1328057
Binshtok, A. M., Wang, H., Zimmermann, K., Amaya, F., Vardeh, D., Shi, L., et al. (2008). Nociceptors are interleukin-1 sensors. J. Neurosci. 28, 14062–14073. doi: 10.1523/JNEUROSCI.3795-08.2008
Brough, D., and Rothwell, N. J. (2007). Caspase-1-dependent processing of pro-interleukin-1beta is cytosolic and precedes cell death. J. Cell Sci. 120, 772–781. doi: 10.1242/jcs.03377
Burnstock, G. (2006). Historical review: ATP as a neurotransmitter. Trends Pharmacol. Sci. 27, 166–176. doi: 10.1016/j.tips.2006.01.005
Burnstock, G. (2016). “Purinergic Mechanisms and Pain,” in Advances in Pharmacology, Vol. 75, ed. J. E. Barrett (Cambridge, MA: Academic Press), 91–137. doi: 10.1016/bs.apha.2015.09.001
Butt, A. M. (2011). ATP: a ubiquitous gliotransmitter integrating neuron-glial networks. Semin. Cell Dev. Biol. 22, 205–213. doi: 10.1016/j.semcdb.2011.02.023
Capuano, A., De Corato, A., Lisi, L., Tringali, G., Navarra, P., and Dello Russo, C. (2009). Proinflammatory-activated trigeminal satellite cells promote neuronal sensitization: relevance for migraine pathology. Mol. Pain 5:43. doi: 10.1186/1744-8069-5-43
Carroll, W. A., Donnelly-Roberts, D., and Jarvis, M. F. (2009). Selective P2X7 receptor antagonists for chronic inflammation and pain. Purinergic Signal. 5, 63–73. doi: 10.1007/s11302-008-9110-6
Castillo, C., Norcini, M., Martin Hernandez, L. A., Correa, G., Blanck, T. J. J., and Recio-Pinto, E. (2013). Satellite glia cells in dorsal root ganglia express functional NMDA receptors. Neuroscience 240, 135–146. doi: 10.1016/j.neuroscience.2013.02.031
Chessell, I. P., Hatcher, J. P., Bountra, C., Michel, A. D., Hughes, J. P., Green, P., et al. (2005). Disruption of the P2X7 purinoceptor gene abolishes chronic inflammatory and neuropathic pain. Pain 114, 386–396. doi: 10.1016/j.pain.2005.01.002
Clark, A. K., Staniland, A. A., Marchand, F., Kaan, T. K. Y., McMahon, S. B., and Malcangio, M. (2010). P2X7-dependent release of interleukin-1 and nociception in the spinal cord following lipopolysaccharide. J. Neurosci. 30, 573–582. doi: 10.1523/JNEUROSCI.3295-09.2010
Colomar, A., Marty, V., Médina, C., Combe, C., Parnet, P., Amédée, T., et al. (2003). Maturation and release of interleukin-1beta by lipopolysaccharide-primed mouse Schwann cells require the stimulation of P2X7 receptors. J. Biol. Chem. 278, 30732–30740. doi: 10.1074/jbc.M304534200
Costa, F. A. L., and Moreira Neto, F. L. (2015). Satellite glial cells in sensory ganglia: its role in pain. Braz. J. Anesthesiol. 65, 73–81. doi: 10.1016/j.bjan.2013.07.013
Cunha, J. M., Cunha, F. Q., Poole, S., and Ferreira, S. H. (2000). Cytokine-mediated inflammatory hyperalgesia limited by interleukin-1 receptor antagonist. Br. J. Pharmacol. 130, 1418–1424. doi: 10.1038/sj.bjp.0703434
De Corato, A., Lisi, L., Capuano, A., Tringali, G., Tramutola, A., Navarra, P., et al. (2011). Trigeminal satellite cells express functional calcitonin gene-related peptide receptors, whose activation enhances interleukin-1β pro-inflammatory effects. J. Neuroimmunol. 237, 39–46. doi: 10.1016/j.jneuroim.2011.05.013
De Marchi, E., Orioli, E., Dal Ben, D., and Adinolfi, E. (2016). “P2X7 Receptor as a Therapeutic Target,” in Advances in Protein Chemistry and Structural Biology, ed. R. Donev (Cambridge, MA: Academic Press Inc), 39–79.
Dell’Antonio, G., Quattrini, A., Cin, E. D., Fulgenzi, A., and Ferrero, M. E. (2002). Relief of inflammatory pain in rats by local use of the selective P2X7 ATP receptor inhibitor, oxidized ATP. Arthritis Rheumatism 46, 3378–3385. doi: 10.1002/art.10678
Devor, M. (1999). Unexplained peculiarities of the dorsal root ganglion. Pain 82, S27–S35. doi: 10.1016/S0304-3959(99)00135-9
Dinarello, C. A. (2018). Overview of the IL-1 family in innate inflammation and acquired immunity. Immunol. Rev. 281, 8–27. doi: 10.1111/imr.12621
Ding, Y., Cesare, P., Drew, L., Nikitaki, D., and Wood, J. N. (2000). ATP, P2X receptors and pain pathways. J. Auton. Nervous Syst. 81, 289–294. doi: 10.1016/S0165-1838(00)00131-4
Dissing-Olesen, L., LeDue, J. M., Rungta, R. L., Hefendehl, J. K., Choi, H. B., and MacVicar, B. A. (2014). Activation of neuronal NMDA receptors triggers transient ATP-mediated microglial process outgrowth. J. Neurosci. 34, 10511–10527. doi: 10.1523/JNEUROSCI.0405-14.2014
Donnelly-Roberts, D. L., Namovic, M. T., Han, P., and Jarvis, M. F. (2009). Mammalian P2X7 receptor pharmacology: comparison of recombinant mouse, rat and human P2X7 receptors. Br. J. Pharmacol. 157, 1203–1214. doi: 10.1111/j.1476-5381.2009.00233.x
England, S., Heblich, F., James, I. F., Robbins, J., and Docherty, R. J. (2001). Bradykinin evokes a Ca2+-activated chloride current in non-neuronal cells isolated from neonatal rat dorsal root ganglia. J. Physiol. 530, 395–403. doi: 10.1111/j.1469-7793.2001.0395k.x
Esposito, M. F., Malayil, R., Hanes, M., and Deer, T. (2019). Unique characteristics of the dorsal root ganglion as a target for neuromodulation. Pain Med. 20, S23–S30. doi: 10.1093/pm/pnz012
Fabbretti, E. (2013). ATP P2X3 receptors and neuronal sensitization. Front. Cell. Neurosci. 7:236. doi: 10.3389/fncel.2013.00236
Fan, W., Zhu, X., He, Y., Zhu, M., Wu, Z., Huang, F., et al. (2019). The role of satellite glial cells in orofacial pain. J. Neurosci. Res. 97, 393–401. doi: 10.1002/jnr.24341
Ferrari, D., Pizzirani, C., Adinolfi, E., Lemoli, R. M., Curti, A., Idzko, M., et al. (2006). The P2X 7 receptor: a key player in IL-1 processing and release. J. Immunol. 176, 3877–3883. doi: 10.4049/jimmunol.176.7.3877
Ferrari, L. F., Cunha, F. Q., Parada, C. A., and Ferreira, S. H. (2007). A novel technique to perform direct intraganglionar injections in rats. J. Neurosci. Methods 159, 236–243. doi: 10.1016/j.jneumeth.2006.07.025
Ferrari, L. F., Lotufo, C. M., Araldi, D., Rodrigues, M. A., Macedo, L. P., Ferreira, S. H., et al. (2014). Inflammatory sensitization of nociceptors depends on activation of NMDA receptors in DRG satellite cells. Proc. Natl. Acad. Sci. U.S.A. 111, 18363–18368. doi: 10.1073/pnas.1420601111
Ferreira, S. H. (1981). Inflammatory pain, prostaglandin hyperalgesia and the development of peripheral analgesics. Trends Pharmacol. Sci. 2, 183–186. doi: 10.1016/0165-6147(81)90306-0
Ferreira, S. H., Lorenzetti, B. B., Bristow, A. F., and Poole, S. (1988). Interleukin-1β as a potent hyperalgesic agent antagonized by a tripeptide analogue. Nature 334, 698–700. doi: 10.1038/334698a0
Fitz, J. G. (2007). Regulation of cellular ATP release. Trans. Am. Clin. Climatol. Assoc. 118, 199–208.
Garlanda, C., Dinarello, C. A., and Mantovani, A. (2013). The interleukin-1 family: back to the future. Immunity 39, 1003–1018. doi: 10.1016/j.immuni.2013.11.010
Giuliani, A. L., Sarti, A. C., Falzoni, S., and Di Virgilio, F. (2017). The P2X7 receptor-interleukin-1 liaison. Front. Pharmacol. 8:123. doi: 10.3389/fphar.2017.00123
Goto, T., Iwai, H., Kuramoto, E., and Yamanaka, A. (2017). Neuropeptides and ATP signaling in the trigeminal ganglion. Jap. Dental Sci. Rev. 53, 117–124. doi: 10.1016/j.jdsr.2017.01.003
Gu, Y., Chen, Y., Zhang, X., Li, G.-W., Wang, C., and Huang, L.-Y. M. (2010). Neuronal soma–satellite glial cell interactions in sensory ganglia and the participation of purinergic receptors. Neuron Glia Biol. 6, 53–62. doi: 10.1017/S1740925X10000116
Guo, W., Wang, H., Watanabe, M., Shimizu, K., Zou, S., LaGraize, S. C., et al. (2007). Glial-cytokine-neuronal interactions underlying the mechanisms of persistent pain. J. Neurosci. 27, 6006–6018. doi: 10.1523/JNEUROSCI.0176-07.2007
Hanani, M. (2005). Satellite glial cells in sensory ganglia: from form to function. Brain Res. Brain Res. Rev. 48, 457–476. doi: 10.1016/j.brainresrev.2004.09.001
Hanani, M. (2012). Intercellular communication in sensory ganglia by purinergic receptors and gap junctions: implications for chronic pain. Brain Res. 1487, 183–191. doi: 10.1016/j.brainres.2012.03.070
He, W. J., Cui, J., Du, L., Zhao, Y. D., Burnstock, G., Zhou, H. D., et al. (2012). Spinal P2X 7 receptor mediates microglia activation-induced neuropathic pain in the sciatic nerve injury rat model. Behav. Brain Res. 226, 163–170. doi: 10.1016/j.bbr.2011.09.015
Honore, P., Donnelly-Roberts, D., Namovic, M., Zhong, C., Wade, C., Chandran, P., et al. (2009). The antihyperalgesic activity of a selective P2X7 receptor antagonist. A-839977, is lost in IL-1αβ knockout mice. Behav. Brain Res. 204, 77–81. doi: 10.1016/j.bbr.2009.05.018
Honore, P., Donnelly-Roberts, D., Namovic, M. T., Hsieh, G., Zhu, C. Z., Mikusa, J. P., et al. (2006). A-740003 [N-(1-{[(cyanoimino)(5-quinolinylamino) methyl]amino}-2,2-dimethylpropyl)-2-(3,4-dimethoxyphenyl)acetamide], a novel and selective P2X7 receptor antagonist, dose-dependently reduces neuropathic pain in the rat. J. Pharmacol. Exp. Ther. 319, 1376–1385. doi: 10.1124/jpet.106.111559
Huang, L.-Y., and Neher, E. (1996). Ca2+-dependent exocytosis in the somata of dorsal root ganglion neurons. Neuron 17, 135–145. doi: 10.1016/S0896-6273(00)80287-1
Huang, L.-Y. M., Gu, Y., and Chen, Y. (2013). Communication between neuronal somata and satellite glial cells in sensory ganglia. Glia 61, 1571–1581. doi: 10.1002/glia.22541
Huang, T.-Y. Y., Belzer, V., and Hanani, M. (2010). Gap junctions in dorsal root ganglia: possible contribution to visceral pain. Eur. J. Pain 14, e1–e11. doi: 10.1016/j.ejpain.2009.02.005
Inoue, K., and Tsuda, M. (2012). Purinergic systems, neuropathic pain and the role of microglia. Exp. Neurol. 234, 293–301. doi: 10.1016/j.expneurol.2011.09.016
Jarvis, M. F. (2010). The neural-glial purinergic receptor ensemble in chronic pain states. Trends Neurosci. 33, 48–57. doi: 10.1016/j.tins.2009.10.003
Joseph, E. K., Parada, C. A., and Levine, J. D. (2003). Hyperalgesic priming in the rat demonstrates marked sexual dimorphism. Pain 105, 143–150. doi: 10.1016/S0304-3959(03)00175-1
Kobayashi, K., Fukuoka, T., Yamanaka, H., Dai, Y., Obata, K., Tokunaga, A., et al. (2005). Differential expression patterns of mRNAs for P2X receptor subunits in neurochemically characterized dorsal root ganglion neurons in the rat. J. Comp. Neurol. 481, 377–390. doi: 10.1002/cne.20393
Kobayashi, K., Takahashi, E., Miyagawa, Y., Yamanaka, H., and Noguchi, K. (2011). Induction of the P2X7 receptor in spinal microglia in a neuropathic pain model. Neurosci. Lett. 504, 57–61. doi: 10.1016/j.neulet.2011.08.058
Kobayashi, K., Yamanaka, H., and Noguchi, K. (2013). Expression of ATP receptors in the rat dorsal root ganglion and spinal cord. Anat. Sci. Int. 88, 10–16. doi: 10.1007/s12565-012-0163-9
Krames, E. S. (2014). The role of the dorsal root ganglion in the development of neuropathic pain. Pain. Med. 15, 1669–1685. doi: 10.1111/pme.12413
Kushnir, R., Cherkas, P. S., and Hanani, M. (2011). Peripheral inflammation upregulates P2X receptor expression in satellite glial cells of mouse trigeminal ganglia: a calcium imaging study. Neuropharmacology 61, 739–746. doi: 10.1016/j.neuropharm.2011.05.019
Lazarowski, E. R. (2012). Vesicular and conductive mechanisms of nucleotide release. Purinergic Signal. 8, 359–373. doi: 10.1007/s11302-012-9304-9
Lemes, J. B. P., de Campos Lima, T., Santos, D. O., Neves, A. F., de Oliveira, F. S. F. S., Parada, C. A., et al. (2018). Participation of satellite glial cells of the dorsal root ganglia in acute nociception. Neurosci. Lett. 676, 8–12. doi: 10.1016/j.neulet.2018.04.003
Li, J., Li, X., Jiang, X., Yang, M., Yang, R., Burnstock, G., et al. (2017). Microvesicles shed from microglia activated by the P2X7-p38 pathway are involved in neuropathic pain induced by spinal nerve ligation in rats. Purinergic Signal. 13, 13–26. doi: 10.1007/s11302-016-9537-0
Linhart, O., Obreja, O., and Kress, M. (2003). The inflammatory mediators serotonin, prostaglandin e2 and bradykinin evoke calcium influx in rat sensory neurons. Neuroscience 118, 69–74. doi: 10.1016/S0306-4522(02)00960-0
Liu, S., Shi, Q., Zhu, Q., Zou, T., Li, G. G., Huang, A., et al. (2015). P2X7 receptor of rat dorsal root ganglia is involved in the effect of moxibustion on visceral hyperalgesia. Purinergic Signal. 11, 161–169. doi: 10.1007/s11302-014-9439-y
Luchting, B., Heyn, J., Woehrle, T., Rachinger-Adam, B., Kreth, S., Hinske, L. C., et al. (2016). Differential expression of P2X7 receptor and IL-1β in nociceptive and neuropathic pain. J. Neuroinflamm. 13:100. doi: 10.1186/s12974-016-0565-z
Maelfait, J., Vercammen, E., and Janssens, S. (2008). Stimulation of Toll-like receptor 3 and 4 induces interleukin-1beta maturation by caspase-8. J. Exp. Med.e 205, 1967–1973. doi: 10.1084/jem.20071632
Magni, G., Riccio, D., and Ceruti, S. (2018). Tackling chronic pain and inflammation through the purinergic system. Curr. Med. Chem. 25, 3830–3865. doi: 10.2174/0929867324666170710110630
Malik, A., and Kanneganti, T.-D. (2017). Inflammasome activation and assembly at a glance. J. Cell Sci. 130, 3955–3963. doi: 10.1242/jcs.207365
Matsuka, Y., Neubert, J. K., Maidment, N. T., and Spigelman, I. (2001). Concurrent release of ATP and substance P within guinea pig trigeminal ganglia in vivo. Brain Res. 915, 248–255. doi: 10.1016/S0006-8993(01)02888-8
McGaraughty, S., Chu, K. L., Namovic, M. T., Donnelly-Roberts, D. L., Harris, R. R., Zhang, X.-F., et al. (2007). P2X7-related modulation of pathological nociception in rats. Neuroscience 146, 1817–1828. doi: 10.1016/j.neuroscience.2007.03.035
Neeb, L., Hellen, P., Boehnke, C., Hoffmann, J., Schuh-Hofer, S., Dirnagl, U., et al. (2011). IL-1β stimulates COX-2 dependent PGE2 synthesis and CGRP release in rat trigeminal ganglia cells. PLoS One 6:e17360. doi: 10.1371/journal.pone.0017360
North, R. A. (2002). Molecular physiology of P2X receptors. Physiol. Rev. 82, 1013–1067. doi: 10.1152/physrev.00015.2002
North, R. A. (2016). P2X receptors. Philos. Trans. R. Soc. Lond. B Biol. Sci. 371, 20150427. doi: 10.1098/rstb.2015.0427
Oliveira, M. C., Pelegrini-da-Silva, A., Tambeli, C. H., and Parada, C. A. (2009). Peripheral mechanisms underlying the essential role of P2X3,2/3 receptors in the development of inflammatory hyperalgesia. Pain 141, 127–134. doi: 10.1016/j.pain.2008.10.024
Pannese, E. (2010). The structure of the perineuronal sheath of satellite glial cells (SGCs) in sensory ganglia. Neuron Glia Biol. 6, 3–10. doi: 10.1017/S1740925X10000037
Prado, F. C., Araldi, D., Vieira, A. S., Oliveira-Fusaro, M. C. G., Tambeli, C. H., and Parada, C. A. (2013). Neuronal P2X3 receptor activation is essential to the hyperalgesia induced by prostaglandins and sympathomimetic amines released during inflammation. Neuropharmacology 67, 252–258. doi: 10.1016/j.neuropharm.2012.11.011
Puerto, A., Wandosell, F., and Garrido, J. J. (2013). Neuronal and glial purinergic receptors functions in neuron development and brain disease. Front. Cell. Neurosci. 7:197. doi: 10.3389/fncel.2013.00197
Rampe, D., Wang, L., and Ringheim, G. E. (2004). P2X7 receptor modulation of β-amyloid- and LPS-induced cytokine secretion from human macrophages and microglia. J. Neuroimmunol. 147, 56–61. doi: 10.1016/j.jneuroim.2003.10.014
Ren, K., and Torres, R. (2009). Role of interleukin-1β during pain and inflammation. Brain Res. Rev. 60, 57–64. doi: 10.1016/j.brainresrev.2008.12.020
Samad, T. A., Moore, K. A., Sapirstein, A., Billet, S., Allchorne, A., Poole, S., et al. (2001). Interleukin-1β-mediated induction of Cox-2 in the CNS contributes to inflammatory pain hypersensitivity. Nature 410, 471–475. doi: 10.1038/35068566
Sapunar, D., Kostic, S., Banozic, A., and Puljak, L. (2012). Dorsal root ganglion - a potential new therapeutic target for neuropathic pain. J. Pain Res. 5, 31–38. doi: 10.2147/JPR.S26603
Savio, L. E. B., de Andrade Mello, P., da Silva, C. G., and Coutinho-Silva, R. (2018). The P2X7 receptor in inflammatory diseases: angel or demon? Front. Pharmacol. 9:52. doi: 10.3389/fphar.2018.00052
Song, X. M., Xu, X. H., Zhu, J., Guo, Z., Li, J., He, C., et al. (2015). Up-regulation of P2X7 receptors mediating proliferation of Schwann cells after sciatic nerve injury. Purinergic Signal. 11, 203–213. doi: 10.1007/s11302-015-9445-8
Stoffels, M., Zaal, R., Kok, N., van der Meer, J. W. M., Dinarello, C. A., and Simon, A. (2015). ATP-Induced IL-1β specific secretion: true under stringent conditions. Front. Immunol. 6:54. doi: 10.3389/fimmu.2015.00054
Takeda, M., Tanimoto, T., Kadoi, J., Nasu, M., Takahashi, M., Kitagawa, J., et al. (2007). Enhanced excitability of nociceptive trigeminal ganglion neurons by satellite glial cytokine following peripheral inflammation. Pain 129, 155–166. doi: 10.1016/j.pain.2006.10.007
Teixeira, J. M., Oliveira, M. C. G., Parada, C. A., and Tambeli, C. H. (2010). Peripheral mechanisms underlying the essential role of P2X7 receptors in the development of inflammatory hyperalgesia. Eur. J. Pharmacol. 644, 55–60. doi: 10.1016/j.ejphar.2010.06.061
Torres-Chávez, K. E., Fischer, L., Teixeira, J. M., Fávaro-Moreira, N. C., Obando-Pereda, G. A., Parada, C. A., et al. (2011). Sexual dimorphism on cytokines expression in the temporomandibular joint: the role of gonadal steroid hormones. Inflammation 34, 487–498. doi: 10.1007/s10753-010-9256-6
Toulme, E., Tsuda, M., Khakh, B. S., and Inoue, K. (2010). “On the role of ATP-gated P2X receptors in acute,” in Inflammatory and Neuropathic Pain, 2010th Edn, eds L. Kruger and A. R. Light (Boca Raton, FL: CRC Press).
Tse, K. H., Chow, K. B. S., Leung, W. K., Wong, Y. H., and Wise, H. (2014). Primary sensory neurons regulate Toll-like receptor-4-dependent activity of glial cells in dorsal root ganglia. Neuroscience 279, 10–22. doi: 10.1016/j.neuroscience.2014.08.033
Verderio, C., and Matteoli, M. (2011). ATP in neuron-glia bidirectional signalling. Brain Res. Rev. 66, 106–114. doi: 10.1016/j.brainresrev.2010.04.007
Verri, W. A., Cunha, T. M., Parada, C. A., Poole, S., Cunha, F. Q., and Ferreira, S. H. (2006). Hypernociceptive role of cytokines and chemokines: targets for analgesic drug development? Pharmacol. Ther. 112, 116–138. doi: 10.1016/j.pharmthera.2006.04.001
Vivancos, G. G., Verri, W. A. Jr., Cunha, T. M., Schivo, R. S. I, Parada, C. A., Cunha, F. Q., et al. (2004). An electronic pressure-meter nociception paw test for rats. Braz. J. Med. Biol. Res. 37, 391–399. doi: 10.1590/S0100-879X2004000300017
Wu, B., Peng, L., Xie, J., Zou, L., Zhu, Q., Jiang, H., et al. (2017). The P2X 7 receptor in dorsal root ganglia is involved in HIV gp120-associated neuropathic pain. Brain Res. Bull. 135, 25–32. doi: 10.1016/j.brainresbull.2017.09.006
Zhang, W., Zhu, Z., and Liu, Z. (2020). The role and pharmacological properties of the P2X7 receptor in neuropathic pain. Brain Res. Bull. 155, 19–28. doi: 10.1016/j.brainresbull.2019.11.006
Zhang, X., Chen, Y., Wang, C., and Huang, L.-Y. (2007). Neuronal somatic ATP release triggers neuron-satellite glial cell communication in dorsal root ganglia. Proc. Natl. Acad. Sci. U.S.A. 104, 9864–9869. doi: 10.1073/pnas.0611048104
Zhang, X.-F. F., Han, P., Faltynek, C. R., Jarvis, M. F., and Shieh, C.-C. C. (2005). Functional expression of P2X7 receptors in non-neuronal cells of rat dorsal root ganglia. Brain Res. 1052, 63–70. doi: 10.1016/j.brainres.2005.06.022
Keywords: satellite glial cells, P2X7 receptors, interleukin-1beta, dorsal root ganglion, inflammatory hyperalgesia
Citation: Neves AF, Farias FH, de Magalhães SF, Araldi D, Pagliusi M Jr, Tambeli CH, Sartori CR, Lotufo CMdC and Parada CA (2020) Peripheral Inflammatory Hyperalgesia Depends on P2X7 Receptors in Satellite Glial Cells. Front. Physiol. 11:473. doi: 10.3389/fphys.2020.00473
Received: 10 January 2020; Accepted: 17 April 2020;
Published: 25 May 2020.
Edited by:
Peter Santha, University of Szeged, HungaryReviewed by:
Parisa Gazerani, Aalborg University, DenmarkFumimasa Amaya, Kyoto Prefectural University of Medicine, Japan
Copyright © 2020 Neves, Farias, de Magalhães, Araldi, Pagliusi, Tambeli, Sartori, Lotufo and Parada. This is an open-access article distributed under the terms of the Creative Commons Attribution License (CC BY). The use, distribution or reproduction in other forums is permitted, provided the original author(s) and the copyright owner(s) are credited and that the original publication in this journal is cited, in accordance with accepted academic practice. No use, distribution or reproduction is permitted which does not comply with these terms.
*Correspondence: Carlos Amílcar Parada, Y2FwYXJhZGFAdW5pY2FtcC5icg==
†Dionéia Araldi, Departments of Medicine and Oral Surgery, and Division of Neuroscience, University of California, San Francisco, San Francisco, CA, United States