- 1Department of Pathology, Lake Erie College of Osteopathic Medicine, Erie, PA, United States
- 2Department of Pathology, Virginia Commonwealth University, Richmond, VA, United States
Muscle cachexia is a catabolic response, usually takes place in various fatal diseases, such as sepsis, burn injury, and chronic kidney disease. Muscle cachexia is also a common co-morbidity seen in the vast majority of advanced cancer patients, often associated with low quality of life and death due to general organ dysfunction. The triggering events and underlying molecular mechanisms of muscle wasting are not yet clearly defined. Our recent study has shown that the ectopic expression of Twist1 in muscle progenitor cells is sufficient to drive muscle structural protein breakdown and attendant muscle atrophy, reminiscent of muscle cachexia. Intriguingly, muscle Twist1 expression is highly induced in cachectic muscles from several mouse models of pancreatic ductal adenocarcinoma (PDAC), raising the interesting possibility that Twist1 may mediate PDAC-driven muscle cachexia. Along these lines, both genetic and pharmacological inactivation of Twist1 function was highly significant at protecting against cancer cachexia, which translated into a significant survival benefit in the experimental PDAC animals. From a translational perspective, elevated expression of Twist1 is also detected in cancer patients with severe muscle wasting, implicating a role of Twist1 in cancer cachexia, and further providing a possible target for therapeutic attenuation of cachexia to improve cancer patient survival. In this article, we will briefly summarize how Twist1 acts as a master regulator of tumor-induced cachexia, and discuss the relevance of our findings to muscle wasting diseases in general. The mechanism of decreased muscle mass in various catabolic conditions is thought to rely on similar pathways, and, therefore, Twist1-induced cancer cachexia may benefit diverse groups of patients with clinical complications associated with loss of muscle mass and functions, beyond the expected benefits for cancer patients.
Introduction
Cachexia, a hypercatabolic state, is a commonly encountered adverse effect of cancer, and markedly impairs the quality of life by harmfully impacting both the physical and psychosocial behaviors. An international consensus reached in 2011 explained cancer cachexia as a multifactorial condition with continuing skeletal muscle loss that is not reversible by standard nutritional support, ultimately leading to functional impairment (Fearon et al., 2011). A skeletal muscle index <7.26 kg/m2 in males and <5.45 kg/m2 in females is considered as cachexia. Of relevance, the majority of patients with pancreatic tumors display signs of cachexia at the time of diagnosis (Fearon et al., 2006). Even the overweight pancreatic cancer patients develop cachexia (sarcopenic obesity), and cachexia hidden in obesity causes extensive muscle loss, with the pathological accumulation of adipose tissue that influences the overall survival of the patients (Tan et al., 2009). In cancer patients, cachexia is a progressive process that evolves through various stages – from pre-cachexia to cachexia to refractory cachexia (irreversible stage). It is important to find measures to reverse from cachexia into pre-cachectic stages to provide relief to the affected patients. Besides cancer, cachexia can also occur in a wide range of disorders, ranging from infections to chronic kidney diseases to cerebrovascular diseases, including stroke and chronic obstructive pulmonary diseases (Reid et al., 2013; Scherbakov et al., 2013; Morley, 2014). Severe muscle wasting or cachexia is noted in up to 75% of chronic kidney disease patients undergoing hemodialysis treatment (Mak et al., 2011).
The underlying mechanism of cachexia, in tumor and other catabolic disorders, are not yet clearly understood. Various catabolic conditions are associated with altered expression and regulations of transcription factors and nuclear cofactors that induce a specific group of genes, which are believed to execute the final steps of muscle atrophy. Two muscle-specific ubiquitin ligases, MuRF1 and Atrogin1/MAFbx, are essential for the degradation of muscle proteins, including myosin heavy chain (MHC) and eukaryotic initiation factor 3f (Elf-3f) (Clarke et al., 2007; Lagirand-Cantaloube et al., 2008). Transcription factors, FOXO1 is an important regulator of muscle atrophy, and is shown to be affected by sepsis and elevated levels of glucocorticoids (Stitt et al., 2004; Crossland et al., 2008; Waddell et al., 2008; Reed et al., 2012; Xu et al., 2012; Huynh et al., 2013). It is a key regulator of genes involved in muscle wasting, including Atrogin-1 and MuRF1 (Li et al., 2007; Leger et al., 2009; Pomies et al., 2016). FOXO1 also regulates genes involved in the autophagy-lysosomal proteolytic pathway (Sengupta et al., 2009; Milan et al., 2015). MyoD is a muscle-specific transcription factor that regulates muscle cell differentiation (Davis et al., 1987). Recently, MyoD-induced muscle cell differentiation is shown to be mediated by Twist1 through miR-206 (Koutalianos et al., 2015). Our recent studies suggest that the transcription factor Twist1 is also actively involved in the regulation of cancer-induced muscle wasting presumably owing to its ability to induce the expression of MuRF1 and Atrogin1, thereby causing muscle protein degradation and attendant muscle cachexia (Parajuli et al., 2018).
Of particular clinical importance, developing effective treatments to curb cachexia and muscle wasting disorders are essential for improving the quality of health and survival of the cancer patients and beyond. Tumor necrosis factor-alpha (TNF-α), interleukin 1 (IL-1), interleukin 6 (IL-6) and interferon-gamma (IFN-γ) are the main cytokines that are thought to be involved in the evolvement of cachexia, in general (Fong et al., 1989; Strassmann et al., 1993; Kayacan et al., 2006; White, 2017). However, clinically targeting these cytokines showed mixed results. For instance, in a clinical trial, infliximab (anti-TNF-α monoclonal antibody) showed no improvement of cachexia in cancer patients (Jatoi et al., 2010). In contrast, treating tumor patients with a humanized monoclonal anti-IL-6 antibody increased hemoglobin levels and reduced muscle wasting (Rigas et al., 2010).
Twist1
Twist was initially identified in Drosophila (Thisse et al., 1987). Later, Twist isoforms have been identified in humans and mice (Wolf et al., 1991; Wang et al., 1997). Twist1 is a member of the basic helix-loop-helix (bHLH) transcription factor family that controls the activity of genes essential for embryogenesis and organogenesis (el Ghouzzi et al., 1997; Wang et al., 1997; Pan et al., 2009). Human and mouse Twist1 proteins share a very high amino acid sequence identity (96%). The Twist1 protein is involved in the generation and maturation of cells that eventually form the musculoskeletal system. Notably, during development, Twist proteins transiently inhibit Runx2 function, causing osteoblast-specific gene expression that leads to osteoblast differentiation (Bialek et al., 2004). Of relevance, human Twist1 is highly expressed in fetal myoblasts, and its level diminishes in the later stages of development (Koutsoulidou et al., 2011). Mutations in the TWIST1 gene in human is associated with craniosynostosis (premature closure of the sutures between the bones of the skull), as noted in the Saethre-Chotzen syndrome-affected individuals (Howard et al., 1997). Heterozygous Twist1 knockout mice showed craniofacial and limb abnormalities, mimicking clinical features of Saethre-Chotzen syndrome patients. Of note, homozygous Twist1 knockout mice were embryonically lethal, suggesting a crucial role of this gene in embryonic survival and development (Chen and Behringer, 1995). In adult mice, Twist1 is expressed in a limited number of tissues, including fibroblasts of the mammary glands and dermal papilla cells of the hair follicles (Xu et al., 2013). Consequently, inducible knockout of Twist1 in adult mice did not affect their overall health and viability, implicating a more important role of Twist1 during early development than in adult life (Xu et al., 2013).
Studies using breast cancer cell lines have shown an important role of Twist1 in epithelial-to-mesenchymal transformation, intravasation and metastasis (Xu et al., 2017); more importantly, genetically ablating the Twist1 function effectively inhibited breast tumor cell intravasation and lung metastasis (Xu et al., 2017). In a similar line of study, Twist1 overexpression has shown to be associated with the progression of several human malignant tumors, including pancreatic ductal adenocarcinoma (PDAC) (Lee and Bar-Sagi, 2010; Qin et al., 2012).
The role of Twist1 in myogenesis is not clear. In Drosophila, Twist has been shown to enhance myogenesis, while in mouse myoblasts (C2C12) and human embryonic stem cells (embryoid bodies), Twist1 has shown to inhibit muscle cell differentiation (Hebrok et al., 1994; Rohwedel et al., 1995; Cao et al., 2008; Koutsoulidou et al., 2011). Moreover, overexpression of Twist1 reverses the process of muscle cell differentiation (Hjiantoniou et al., 2008; Mastroyiannopoulos et al., 2013). Recently, we have shown that induction of Twist1 is also related to muscle cachexia during the progression of cancer (Parajuli et al., 2018).
Twist1 Activation and Cancer-Induced Cachexia
Studies have shown that numerous hormones, cytokines, and tumor-derived factors play key roles in the initiation and propagation of cancer cachexia by involving several major intracellular signaling systems (Benny Klimek et al., 2010). ActRIIB is a high-affinity activin type two receptor that facilitates the signaling of various factors, including myostatin, and activin (Lee and McPherron, 2001; Souza et al., 2008). Induced expression of activin could cause cachexia in tumor-free mice (Chen et al., 2014). Myostatin is a secreted protein of the TGF-β family, which is mostly expressed in skeletal muscle, including muscle progenitor satellite cells. In a mouse model of pancreatic cancer-induced cachexia, therapeutic reduction of TGF-β resulted in reduced cachexia and increased survival (Greco et al., 2015). Furthermore, increased signaling activity through ActRIIB pathway has shown to be involved in both tumorigenesis and cancer-induced cachexia (Wildi et al., 2001; Costelli et al., 2008; Zhou et al., 2010). Intriguingly, blocking the bioactivities of ActRIIB has been shown to reverse cancer-induced cachexia and cardiac atrophy, and this response resulted in the extended lifespan of the experimental animals even without reducing the tumor growth (Zhou et al., 2010).
As mentioned, two muscle-specific ubiquitin ligases, MuRF1, and Atrogin1/MAFbx are essential to muscle protein degradation, including MHC and Elf-3f (Clarke et al., 2007; Lagirand-Cantaloube et al., 2008). Myostatin can induce the expression of MuRF1 and Atrogin1/MAFbx as well as Twist1 (Parajuli et al., 2018), and genetic inactivation of myostatin has shown to protect against cancer-induced cachexia (Gallot et al., 2014). Selectively inducing Twis1 in mesenchymal stem cells using mouse genetics tools caused severe hypotrophy of the skeletal muscle (Parajuli et al., 2018). Interestingly, when similar in vivo studies were conducted on muscle progenitor cells (satellite cells), overexpression of Twist1 resulted in the loss of muscle mass in adult mice. Morphological analysis of the Twist1 overexpressing atrophic muscle showed markedly reduced myofiber diameters, as compared to the control animals, clearly demonstrating the in vivo role of Twist1 hyperactivity in muscle atrophy (Parajuli et al., 2018).
Therapeutic Potential of Twist1 in Cancer
In a healthy-weight individual, skeletal muscle comprises almost 40% of total human body mass (Rolfe and Brown, 1997). Studies have shown that patients with pancreatic cancer have often developed severe cachexia, which is associated with substantial weight loss and skeletal muscle atrophy. Noteworthy, conventional nutritional support cannot fully reverse the loss of muscle function in these patients. Almost one-third of cachectic patients develop severe respiratory muscle dysfunction, causing death due to cardiopulmonary failure in pancreatic cancer patients (Bachmann et al., 2008). In experimental models of pancreatic cancer, reducing cachexia can improve overall survival, despite persistent tumor growth, suggesting that cachexia is an important determinant of survival in tumor patients (Tisdale, 2010).
Our studies have shown that tumor-derived Activin A acts on the muscle to upregulate the expression of Twist1, which in turn induces the synthesis of the muscle-specific ubiquitin ligases, MuRF1 and Atrogin1, thereby causing muscle cachexia by facilitating muscle protein degradation (Figure 1; Parajuli et al., 2018). In experimental studies, serum activin levels correlated with PDAC-induced cachexia and eventual mortality (Zhong et al., 2019). In the murine model of PDAC-induced cachexia, activins (activin-βA, or Inhba) are expressed, both in tumor cells and tumor stromal cells. Treatment with an activin inhibitor in a murine model of PDAC-induced cachexia reduced weight loss and cachexia with the resultant effect being prolonged survival (Zhong et al., 2019). Moreover, using the pharmacological drug JQ1, a small molecule that suppresses Twist1 activity by blunting its binding to MuRF1 and Atrogin1 promoters, muscle cachexia could be reversed in PDAC mice deleted of Twist1, indicating that inhibition of Twist1 activity in muscle in indispensable for preventing muscle cachexia. Treatment with JQ1 prevented weight loss, which was associated with increased muscle mass and myofiber size, in turn resulting in improved muscle function and better survival of the PDAC-induced cachectic mice (Parajuli et al., 2018). It is important to emphasize that the apparent survival benefit of these experimentally induced tumor models (due to suppression of Twist1 activity) was mostly related to the reversal of muscle cachexia, and not due to shrinkage of tumor (Parajuli et al., 2018). These in vivo observations suggest that Twist1 could be a therapeutic target to reduce muscle mass loss in tumor and other chronic debilitating diseases, not only to improve quality of life, but also to increase disease-free survival.
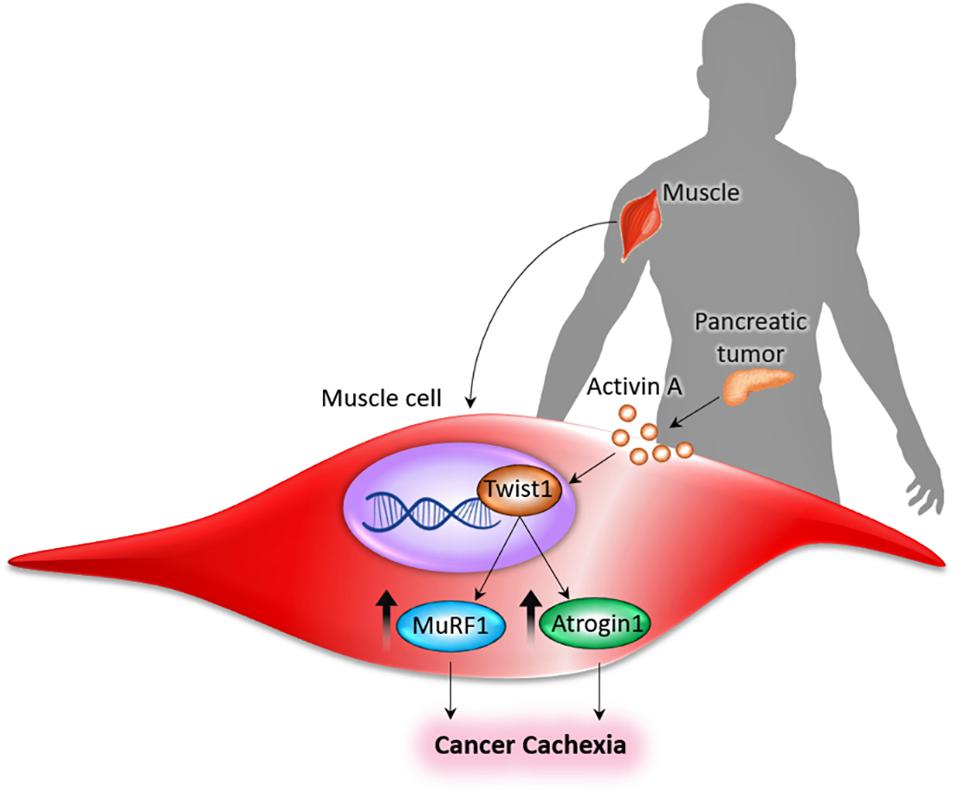
Figure 1. Pancreas-muscle axis. Tumor-derived Activin A acts on the muscle to upregulate the expression of Twist1, which in turn induces the synthesis of the muscle-specific ubiquitin ligases (MuRF1 and Atrogin1), thereby causing cancer cachexia by facilitating muscle protein degradation (Parajuli et al., 2018).
In mice with chronic kidney disease, a two- to three-fold increase in myostatin expression was detected in muscle (Zhang et al., 2011); after 7 days of treatment with the anti-myostatin peptibody, muscle weights in mice with chronic kidney disease was significantly greater than those in vehicle-treated chronic kidney disease mice. Such gain of muscle mass was also reflected in the body weight gain of mice with chronic kidney disease that were treated with the anti-myostatin peptibody (Zhang et al., 2011). Furthermore, the elevated level of activin A was detected in various tissues in mice with chronic kidney disease (Williams et al., 2018). Interestingly, experimentally induced chronic kidney disease animals also showed higher expression of Atrogin-1 and MuRF-1 (Avin et al., 2016). Whether such an increase in the expression of activin A in chronic kidney disease leads to the activation of Twist1 to induce the expression of Atrogin-1 and MuRF-1 needs further studies.
Given the similarities in the general mechanisms governing muscle cachexia, one would surmise that the development of a therapeutic strategy to reduce the disease burden associated with reduced muscle function and cachexia would also benefit patients beyond tumor (Table 1). For instance, in chronic kidney disease patients undergoing hemodialysis treatment, the stable weight patients have better survival than those with weight loss (Villain et al., 2015). Of clinical importance, muscle wasting or cachexia occurs in up to 75% of chronic kidney disease patients on hemodialysis (Mak et al., 2011). Despite such widespread occurring of muscle wasting and its adverse impact on the survival of chronic kidney disease patients, there is no selective and effective clinical treatment of cachexia in patients with chronic kidney disease. Reducing the abundance of MuRF1 and atrogin-1 in skeletal muscles of the tumor and chronic diseases through targeting upstream regulators would likely to attenuate muscle cachexia (Zhang et al., 2018).
Conclusion
Cachexia occurs in many end-stage illnesses, including cancers, chronic kidney diseases, chronic cardiac diseases, chronic obstructive pulmonary diseases, chronic liver diseases, severe burns, HIV infections, rheumatoid arthritis, and aging (Mattox, 2017; von Haehling et al., 2017; Baracos et al., 2018; Scicchitano et al., 2018; Thakur et al., 2018). Roughly, 30% of patients with chronic lung, liver, heart or kidney diseases develop cachexia, while around 50% of cancer patients develop that syndrome, either as a direct consequence of the disease itself or as a consequence of treatment. Since cachexia cannot always be reversed by nutritional supplements, its underlying mechanism is different than that of an eating disorder, such as anorexia. Moreover, cachexia usually affects the loss of the muscular component of the body, while starvation initially initiates the loss of fat mass (Morley et al., 2006). The overall devastating impact of cachexia on patients with chronic diseases can not only reduce physical activities and quality of life but more importantly, can shorten survival (Farkas et al., 2013). Hence, developing effective treatments to reduce the progression of cachexia and muscle wasting disorders are essential clinical need to reduce disease burden and improve the quality of life and survival of the cancer patients and beyond. Twist1 promotes epithelial-mesenchymal transition, invasion, metastasis, and chemotherapy resistance in cancer cells and thus is a potential target for cancer therapy (Kang and Massague, 2004; Vernon and LaBonne, 2004; Yang et al., 2004; Lee et al., 2006; Yuen et al., 2007). Our recent identification of Twist1 as a master regulator of tumor-induced cachexia provides a promising therapeutic target to attenuate cachexia to improve cancer patient survival. In fact, pharmacological inactivation of the Twist1 function showed promising effects of protecting cancer-induced cachexia, with a significant survival benefit in the experimental model of pancreatic carcinoma (Parajuli et al., 2018). Based on the inducible Twist1 knockout mice studies, it appears that Twist1 has rather a non-essential role in adult animals (Xu et al., 2013), and therefore, targeting Twist1 to manipulate tumor-induced cachexia would be a suitable drug target that is likely to exert minimal advert effects in adult patients. Further studies are needed to determine the effects of suppressing Twist1 function in muscle wasting diseases, in general.
Author Contributions
MR and AA outlined and drafted the manuscript. Both authors contributed to the article and approved the submitted version.
Conflict of Interest
The authors declare that the research was conducted in the absence of any commercial or financial relationships that could be construed as a potential conflict of interest.
Acknowledgments
Thanks to Dr. Nuraly Akimbekov of Al-Farabi Kazakh National University (Kazakhstan) for help in drawing the illustration.
Abbreviations
Atrogin1/MAFbx, atrogin1/muscle atrophy F-box; bHLH, basic helix-loop-helix Runx2; Elf-3f, eukaryotic initiation factor 3f FOXO1; HIV, human immunodeficiency viruses; IL-1, interleukin 1; IL-6, interleukin 6; INF- γ, interferon-gamma; MHC, myosin heavy chain; miR-206, microRNA-206; MuRF1, muscle RING finger 1; PDAC, pancreatic ductal adenocarcinoma; TGF- β, transforming growth factor- β; TNF- α, tumor necrosis factor-alpha; Twist1, twist family bHLH transcription factor 1.
References
Avin, K. G., Chen, N. X., Organ, J. M., Zarse, C., O’neill, K., Conway, R. G., et al. (2016). Skeletal muscle regeneration and oxidative stress are altered in chronic kidney disease. PLoS One 11:e0159411. doi: 10.1371/journal.pone.0159411
Bachmann, J., Heiligensetzer, M., Krakowski-Roosen, H., Buchler, M. W., Friess, H., and Martignoni, M. E. (2008). Cachexia worsens prognosis in patients with resectable pancreatic cancer. J. Gastrointest. Surg. 12, 1193–1201. doi: 10.1007/s11605-008-0505-z
Baracos, V. E., Martin, L., Korc, M., Guttridge, D. C., and Fearon, K. C. H. (2018). Cancer-associated cachexia. Nat. Rev. Dis. Primers 4:17105.
Benny Klimek, M. E., Aydogdu, T., Link, M. J., Pons, M., Koniaris, L. G., and Zimmers, T. A. (2010). Acute inhibition of myostatin-family proteins preserves skeletal muscle in mouse models of cancer cachexia. Biochem. Biophys. Res. Commun. 391, 1548–1554. doi: 10.1016/j.bbrc.2009.12.123
Bialek, P., Kern, B., Yang, X., Schrock, M., Sosic, D., Hong, N., et al. (2004). A twist code determines the onset of osteoblast differentiation. Dev. Cell 6, 423–435. doi: 10.1016/s1534-5807(04)00058-9
Cao, F., Wagner, R. A., Wilson, K. D., Xie, X., Fu, J. D., Drukker, M., et al. (2008). Transcriptional and functional profiling of human embryonic stem cell-derived cardiomyocytes. PLoS One 3:e3474. doi: 10.1371/journal.pone.0003474
Chen, J. L., Walton, K. L., Winbanks, C. E., Murphy, K. T., Thomson, R. E., Makanji, Y., et al. (2014). Elevated expression of activins promotes muscle wasting and cachexia. FASEB J. 28, 1711–1723. doi: 10.1096/fj.13-245894
Chen, Z. F., and Behringer, R. R. (1995). twist is required in head mesenchyme for cranial neural tube morphogenesis. Genes Dev. 9, 686–699. doi: 10.1101/gad.9.6.686
Clarke, B. A., Drujan, D., Willis, M. S., Murphy, L. O., Corpina, R. A., Burova, E., et al. (2007). The E3 Ligase MuRF1 degrades myosin heavy chain protein in dexamethasone-treated skeletal muscle. Cell Metab. 6, 376–385. doi: 10.1016/j.cmet.2007.09.009
Costelli, P., Muscaritoli, M., Bonetto, A., Penna, F., Reffo, P., Bossola, M., et al. (2008). Muscle myostatin signalling is enhanced in experimental cancer cachexia. Eur. J. Clin. Invest. 38, 531–538. doi: 10.1111/j.1365-2362.2008.01970.x
Crossland, H., Constantin-Teodosiu, D., Gardiner, S. M., Constantin, D., and Greenhaff, P. L. (2008). A potential role for Akt/FOXO signalling in both protein loss and the impairment of muscle carbohydrate oxidation during sepsis in rodent skeletal muscle. J. Physiol. 586, 5589–5600. doi: 10.1113/jphysiol.2008.160150
Davis, R. L., Weintraub, H., and Lassar, A. B. (1987). Expression of a single transfected cDNA converts fibroblasts to myoblasts. Cell 51, 987–1000. doi: 10.1016/0092-8674(87)90585-x
el Ghouzzi, V., Le Merrer, M., Perrin-Schmitt, F., Lajeunie, E., Benit, P., Renier, D., et al. (1997). Mutations of the TWIST gene in the Saethre-Chotzen syndrome. Nat. Genet. 15, 42–46.
Farkas, J., Von Haehling, S., Kalantar-Zadeh, K., Morley, J. E., Anker, S. D., and Lainscak, M. (2013). Cachexia as a major public health problem: frequent, costly, and deadly. J. Cachexia Sarcopenia Muscle 4, 173–178. doi: 10.1007/s13539-013-0105-y
Fearon, K., Strasser, F., Anker, S. D., Bosaeus, I., Bruera, E., Fainsinger, R. L., et al. (2011). Definition and classification of cancer cachexia: an international consensus. Lancet Oncol. 12, 489–495. doi: 10.1016/s1470-2045(10)70218-7
Fearon, K. C., Voss, A. C., and Hustead, D. S. (2006). Definition of cancer cachexia: effect of weight loss, reduced food intake, and systemic inflammation on functional status and prognosis. Am. J. Clin. Nutr. 83, 1345–1350. doi: 10.1093/ajcn/83.6.1345
Fong, Y., Moldawer, L. L., Marano, M., Wei, H., Barber, A., Manogue, K., et al. (1989). Cachectin/TNF or IL-1 alpha induces cachexia with redistribution of body proteins. Am. J. Physiol. 256, R659–R665.
Gallot, Y. S., Durieux, A. C., Castells, J., Desgeorges, M. M., Vernus, B., Plantureux, L., et al. (2014). Myostatin gene inactivation prevents skeletal muscle wasting in cancer. Cancer Res. 74, 7344–7356. doi: 10.1158/0008-5472.can-14-0057
Greco, S. H., Tomkotter, L., Vahle, A. K., Rokosh, R., Avanzi, A., Mahmood, S. K., et al. (2015). TGF-beta blockade reduces mortality and metabolic changes in a validated murine model of pancreatic cancer cachexia. PLoS One 10:e0132786. doi: 10.1371/journal.pone.0132786
Hebrok, M., Wertz, K., and Fuchtbauer, E. M. (1994). M-twist is an inhibitor of muscle differentiation. Dev. Biol. 165, 537–544. doi: 10.1006/dbio.1994.1273
Hjiantoniou, E., Anayasa, M., Nicolaou, P., Bantounas, I., Saito, M., Iseki, S., et al. (2008). Twist induces reversal of myotube formation. Differentiation 76, 182–192. doi: 10.1111/j.1432-0436.2007.00195.x
Howard, T. D., Paznekas, W. A., Green, E. D., Chiang, L. C., Ma, N., Ortiz De Luna, R. I., et al. (1997). Mutations in TWIST, a basic helix-loop-helix transcription factor, in Saethre-Chotzen syndrome. Nat. Genet. 15, 36–41. doi: 10.1038/ng0197-36
Huynh, T., Uaesoontrachoon, K., Quinn, J. L., Tatem, K. S., Heier, C. R., Van Der Meulen, J. H., et al. (2013). Selective modulation through the glucocorticoid receptor ameliorates muscle pathology in mdx mice. J. Pathol. 231, 223–235. doi: 10.1002/path.4231
Jatoi, A., Ritter, H. L., Dueck, A., Nguyen, P. L., Nikcevich, D. A., Luyun, R. F., et al. (2010). A placebo-controlled, double-blind trial of infliximab for cancer-associated weight loss in elderly and/or poor performance non-small cell lung cancer patients (N01C9). Lung Cancer 68, 234–239. doi: 10.1016/j.lungcan.2009.06.020
Kang, Y., and Massague, J. (2004). Epithelial-mesenchymal transitions: twist in development and metastasis. Cell 118, 277–279.
Kayacan, O., Karnak, D., Beder, S., Gullu, E., Tutkak, H., Senler, F. C., et al. (2006). Impact of TNF-alpha and IL-6 levels on development of cachexia in newly diagnosed NSCLC patients. Am. J. Clin. Oncol. 29, 328–335. doi: 10.1097/01.coc.0000221300.72657.e0
Koutalianos, D., Koutsoulidou, A., Mastroyiannopoulos, N. P., Furling, D., and Phylactou, L. A. (2015). MyoD transcription factor induces myogenesis by inhibiting Twist-1 through miR-206. J. Cell Sci. 128, 3631–3645. doi: 10.1242/jcs.172288
Koutsoulidou, A., Mastroyiannopoulos, N. P., Furling, D., Uney, J. B., and Phylactou, L. A. (2011). Endogenous TWIST expression and differentiation are opposite during human muscle development. Muscle Nerve 44, 984–986. doi: 10.1002/mus.22241
Lagirand-Cantaloube, J., Offner, N., Csibi, A., Leibovitch, M. P., Batonnet-Pichon, S., Tintignac, L. A., et al. (2008). The initiation factor eIF3-f is a major target for atrogin1/MAFbx function in skeletal muscle atrophy. EMBO J. 27, 1266–1276. doi: 10.1038/emboj.2008.52
Lee, K. E., and Bar-Sagi, D. (2010). Oncogenic KRas suppresses inflammation-associated senescence of pancreatic ductal cells. Cancer Cell 18, 448–458. doi: 10.1016/j.ccr.2010.10.020
Lee, S. J., and McPherron, A. C. (2001). Regulation of myostatin activity and muscle growth. Proc. Natl. Acad. Sci. U.S.A. 98, 9306–9311. doi: 10.1073/pnas.151270098
Lee, T. K., Poon, R. T., Yuen, A. P., Ling, M. T., Kwok, W. K., Wang, X. H., et al. (2006). Twist overexpression correlates with hepatocellular carcinoma metastasis through induction of epithelial-mesenchymal transition. Clin. Cancer Res. 12, 5369–5376. doi: 10.1158/1078-0432.ccr-05-2722
Leger, B., Senese, R., Al-Khodairy, A. W., Deriaz, O., Gobelet, C., Giacobino, J. P., et al. (2009). Atrogin-1, MuRF1, and FoXO, as well as phosphorylated GSK-3beta and 4E-BP1 are reduced in skeletal muscle of chronic spinal cord-injured patients. Muscle Nerve 40, 69–78. doi: 10.1002/mus.21293
Li, H. H., Willis, M. S., Lockyer, P., Miller, N., Mcdonough, H., Glass, D. J., et al. (2007). Atrogin-1 inhibits Akt-dependent cardiac hypertrophy in mice via ubiquitin-dependent coactivation of Forkhead proteins. J. Clin. Invest. 117, 3211–3223. doi: 10.1172/jci31757
Mak, R. H., Ikizler, A. T., Kovesdy, C. P., Raj, D. S., Stenvinkel, P., and Kalantar-Zadeh, K. (2011). Wasting in chronic kidney disease. J. Cachexia Sarcopenia Muscle 2, 9–25.
Mastroyiannopoulos, N. P., Antoniou, A. A., Koutsoulidou, A., Uney, J. B., and Phylactou, L. A. (2013). Twist reverses muscle cell differentiation through transcriptional down-regulation of myogenin. Biosci. Rep. 33:e00083.
Mattox, T. W. (2017). Cancer cachexia: cause, diagnosis, and treatment. Nutr. Clin. Pract. 32, 599–606. doi: 10.1177/0884533617722986
Milan, G., Romanello, V., Pescatore, F., Armani, A., Paik, J. H., Frasson, L., et al. (2015). Regulation of autophagy and the ubiquitin-proteasome system by the FoxO transcriptional network during muscle atrophy. Nat. Commun. 6:6670.
Morley, J. E. (2014). Chronic obstructive pulmonary disease: a disease of older persons. J. Am. Med. Dir. Assoc. 15, 151–153.
Morley, J. E., Thomas, D. R., and Wilson, M. M. (2006). Cachexia: pathophysiology and clinical relevance. Am. J. Clin. Nutr. 83, 735–743. doi: 10.1093/ajcn/83.4.735
Pan, D., Fujimoto, M., Lopes, A., and Wang, Y. X. (2009). Twist-1 is a PPARdelta-inducible, negative-feedback regulator of PGC-1alpha in brown fat metabolism. Cell 137, 73–86. doi: 10.1016/j.cell.2009.01.051
Parajuli, P., Kumar, S., Loumaye, A., Singh, P., Eragamreddy, S., Nguyen, T. L., et al. (2018). Twist1 activation in muscle progenitor cells causes muscle loss akin to cancer cachexia. Dev. Cell. 45, 712.e6–725.e6.
Pomies, P., Blaquiere, M., Maury, J., Mercier, J., Gouzi, F., and Hayot, M. (2016). Involvement of the FoxO1/MuRF1/Atrogin-1 signaling pathway in the oxidative stress-induced atrophy of cultured chronic obstructive pulmonary disease myotubes. PLoS One 11:e0160092. doi: 10.1371/journal.pone.0160092
Qin, Q., Xu, Y., He, T., Qin, C., and Xu, J. (2012). Normal and disease-related biological functions of Twist1 and underlying molecular mechanisms. Cell Res. 22, 90–106. doi: 10.1038/cr.2011.144
Reed, S. A., Sandesara, P. B., Senf, S. M., and Judge, A. R. (2012). Inhibition of FoxO transcriptional activity prevents muscle fiber atrophy during cachexia and induces hypertrophy. FASEB J. 26, 987–1000. doi: 10.1096/fj.11-189977
Reid, J., Noble, H. R., Porter, S., Shields, J. S., and Maxwell, A. P. (2013). A literature review of end-stage renal disease and cachexia: understanding experience to inform evidence-based healthcare. J. Ren. Care 39, 47–51. doi: 10.1111/j.1755-6686.2013.00341.x
Rigas, J. R., Schuster, M., Orlov, S. V., Milovanovic, B., Prabhash, K., and Smith, J. T. (2010). Efect of ALD518, a humanized anti-IL-6 antibody, on lean body mass loss and symptoms in patients with advanced non-small cell lung cancer (NSCLC): results of a phase II randomized, double-blind safety and efficacy trial. J. Clin. Oncol. 28(15_Suppl.):7622. doi: 10.1200/jco.2010.28.15_suppl.7622
Rohwedel, J., Horak, V., Hebrok, M., Fuchtbauer, E. M., and Wobus, A. M. (1995). M-twist expression inhibits mouse embryonic stem cell-derived myogenic differentiation in vitro. Exp. Cell Res. 220, 92–100. doi: 10.1006/excr.1995.1295
Rolfe, D. F., and Brown, G. C. (1997). Cellular energy utilization and molecular origin of standard metabolic rate in mammals. Physiol. Rev. 77, 731–758. doi: 10.1152/physrev.1997.77.3.731
Scherbakov, N., Von Haehling, S., Anker, S. D., Dirnagl, U., and Doehner, W. (2013). Stroke induced Sarcopenia: muscle wasting and disability after stroke. Int. J. Cardiol. 170, 89–94. doi: 10.1016/j.ijcard.2013.10.031
Scicchitano, B. M., Dobrowolny, G., Sica, G., and Musaro, A. (2018). Molecular insights into muscle homeostasis, atrophy and wasting. Curr. Genomics 19, 356–369. doi: 10.2174/1389202919666180101153911
Sengupta, A., Molkentin, J. D., and Yutzey, K. E. (2009). FoxO transcription factors promote autophagy in cardiomyocytes. J. Biol. Chem. 284, 28319–28331. doi: 10.1074/jbc.m109.024406
Souza, T. A., Chen, X., Guo, Y., Sava, P., Zhang, J., Hill, J. J., et al. (2008). Proteomic identification and functional validation of activins and bone morphogenetic protein 11 as candidate novel muscle mass regulators. Mol. Endocrinol. 22, 2689–2702. doi: 10.1210/me.2008-0290
Stitt, T. N., Drujan, D., Clarke, B. A., Panaro, F., Timofeyva, Y., Kline, W. O., et al. (2004). The IGF-1/PI3K/Akt pathway prevents expression of muscle atrophy-induced ubiquitin ligases by inhibiting FOXO transcription factors. Mol. Cell. 14, 395–403. doi: 10.1016/s1097-2765(04)00211-4
Strassmann, G., Masui, Y., Chizzonite, R., and Fong, M. (1993). Mechanisms of experimental cancer cachexia. Local involvement of IL-1 in colon-26 tumor. J. Immunol. 150, 2341–2345.
Tan, B. H., Birdsell, L. A., Martin, L., Baracos, V. E., and Fearon, K. C. (2009). Sarcopenia in an overweight or obese patient is an adverse prognostic factor in pancreatic cancer. Clin. Cancer Res. 15, 6973–6979. doi: 10.1158/1078-0432.ccr-09-1525
Thakur, S. S., Swiderski, K., Ryall, J. G., and Lynch, G. S. (2018). Therapeutic potential of heat shock protein induction for muscular dystrophy and other muscle wasting conditions. Philos. Trans. R. Soc. Lond. B Biol. Sci. 373:20160528. doi: 10.1098/rstb.2016.0528
Thisse, B., El Messal, M., and Perrin-Schmitt, F. (1987). The twist gene: isolation of a Drosophila zygotic gene necessary for the establishment of dorsoventral pattern. Nucleic Acids Res. 15, 3439–3453. doi: 10.1093/nar/15.8.3439
Vernon, A. E., and LaBonne, C. (2004). Tumor metastasis: a new twist on epithelial-mesenchymal transitions. Curr. Biol. 14, R719–R721.
Villain, C., Ecochard, R., Genet, L., Jean, G., Kuentz, F., Lataillade, D., et al. (2015). Impact of BMI variations on survival in elderly hemodialysis patients. J. Ren. Nutr. 25, 488–493. doi: 10.1053/j.jrn.2015.05.004
von Haehling, S., Ebner, N., Dos Santos, M. R., Springer, J., and Anker, S. D. (2017). Muscle wasting and cachexia in heart failure: mechanisms and therapies. Nat. Rev. Cardiol. 14, 323–341. doi: 10.1038/nrcardio.2017.51
Waddell, D. S., Baehr, L. M., Van Den Brandt, J., Johnsen, S. A., Reichardt, H. M., Furlow, J. D., et al. (2008). The glucocorticoid receptor and FOXO1 synergistically activate the skeletal muscle atrophy-associated MuRF1 gene. Am. J. Physiol. Endocrinol. Metab. 295, E785–E797.
Wang, S. M., Coljee, V. W., Pignolo, R. J., Rotenberg, M. O., Cristofalo, V. J., and Sierra, F. (1997). Cloning of the human twist gene: its expression is retained in adult mesodermally-derived tissues. Gene 187, 83–92. doi: 10.1016/s0378-1119(96)00727-5
White, J. P. (2017). IL-6, cancer and cachexia: metabolic dysfunction creates the perfect storm. Transl. Cancer Res. 6, S280–S285.
Wildi, S., Kleeff, J., Maruyama, H., Maurer, C. A., Buchler, M. W., and Korc, M. (2001). Overexpression of activin A in stage IV colorectal cancer. Gut 49, 409–417. doi: 10.1136/gut.49.3.409
Williams, M. J., Sugatani, T., Agapova, O. A., Fang, Y., Gaut, J. P., Faugere, M. C., et al. (2018). The activin receptor is stimulated in the skeleton, vasculature, heart, and kidney during chronic kidney disease. Kidney Int. 93, 147–158. doi: 10.1016/j.kint.2017.06.016
Wolf, C., Thisse, C., Stoetzel, C., Thisse, B., Gerlinger, P., and Perrin-Schmitt, F. (1991). The M-twist gene of Mus is expressed in subsets of mesodermal cells and is closely related to the Xenopus X-twi and the Drosophila twist genes. Dev. Biol. 143, 363–373. doi: 10.1016/0012-1606(91)90086-i
Xu, J., Li, R., Workeneh, B., Dong, Y., Wang, X., and Hu, Z. (2012). Transcription factor FoxO1, the dominant mediator of muscle wasting in chronic kidney disease, is inhibited by microRNA-486. Kidney Int. 82, 401–411. doi: 10.1038/ki.2012.84
Xu, Y., Lee, D. K., Feng, Z., Bu, W., Li, Y., Liao, L., et al. (2017). Breast tumor cell-specific knockout of Twist1 inhibits cancer cell plasticity, dissemination, and lung metastasis in mice. Proc. Natl. Acad. Sci. U.S.A. 114, 11494–11499. doi: 10.1073/pnas.1618091114
Xu, Y., Liao, L., Zhou, N., Theissen, S. M., Liao, X. H., Nguyen, H., et al. (2013). Inducible knockout of Twist1 in young and adult mice prolongs hair growth cycle and has mild effects on general health, supporting Twist1 as a preferential cancer target. Am. J. Pathol. 183, 1281–1292. doi: 10.1016/j.ajpath.2013.06.021
Yang, J., Mani, S. A., Donaher, J. L., Ramaswamy, S., Itzykson, R. A., Come, C., et al. (2004). Twist, a master regulator of morphogenesis, plays an essential role in tumor metastasis. Cell 117, 927–939. doi: 10.1016/j.cell.2004.06.006
Yuen, H. F., Chan, Y. P., Wong, M. L., Kwok, W. K., Chan, K. K., Lee, P. Y., et al. (2007). Upregulation of Twist in oesophageal squamous cell carcinoma is associated with neoplastic transformation and distant metastasis. J. Clin. Pathol. 60, 510–514. doi: 10.1136/jcp.2006.039099
Zhang, A., Li, M., Wang, B., Klein, J. D., Price, S. R., and Wang, X. H. (2018). miRNA-23a/27a attenuates muscle atrophy and renal fibrosis through muscle-kidney crosstalk. J. Cachexia Sarcopenia Muscle 9, 755–770. doi: 10.1002/jcsm.12296
Zhang, L., Rajan, V., Lin, E., Hu, Z., Han, H. Q., Zhou, X., et al. (2011). Pharmacological inhibition of myostatin suppresses systemic inflammation and muscle atrophy in mice with chronic kidney disease. FASEB J. 25, 1653–1663. doi: 10.1096/fj.10-176917
Zhong, X., Pons, M., Poirier, C., Jiang, Y., Liu, J., Sandusky, G. E., et al. (2019). The systemic activin response to pancreatic cancer: implications for effective cancer cachexia therapy. J. Cachexia Sarcopenia Muscle 10, 1083–1101. doi: 10.1002/jcsm.12461
Keywords: activin A, twist1, MuRF1, atrogin1, muscle atrophy
Citation: Razzaque MS and Atfi A (2020) Regulatory Role of the Transcription Factor Twist1 in Cancer-Associated Muscle Cachexia. Front. Physiol. 11:662. doi: 10.3389/fphys.2020.00662
Received: 14 February 2020; Accepted: 25 May 2020;
Published: 23 June 2020.
Edited by:
Marcella Canton, University of Padova, ItalyReviewed by:
Xu Yan, Victoria University, AustraliaYu-Chiang Lai, University of Birmingham, United Kingdom
Copyright © 2020 Razzaque and Atfi. This is an open-access article distributed under the terms of the Creative Commons Attribution License (CC BY). The use, distribution or reproduction in other forums is permitted, provided the original author(s) and the copyright owner(s) are credited and that the original publication in this journal is cited, in accordance with accepted academic practice. No use, distribution or reproduction is permitted which does not comply with these terms.
*Correspondence: Mohammed S. Razzaque, bXJhenphcXVlQGxlY29tLmVkdQ==; bW9oYW1tZWQucmF6emFxdWVAdW1iLmVkdQ==