- 1Department of Pharmacy, Southeast University, Dhaka, Bangladesh
- 2Pharmakon Neuroscience Research Network, Dhaka, Bangladesh
- 3Institute of Synthetic Biology, Shenzhen Institute of Advanced Technology (SIAT), Chinese Academy of Sciences (CAS), Shenzhen, China
- 4Research Unit, Induced Resistance and Plant Bioprotection, EA 4707, SFR Condorcet FR CNRS 3417, Faculty of Sciences, University of Reims Champagne-Ardenne, Reims Cedex, France
- 5Pre-clinical Research Unit, King Fahd Medical Research Center, King Abdulaziz University, Jeddah, Saudi Arabia
- 6Department of Medical Laboratory Technology, Faculty of Applied Medical Sciences, King Abdulaziz University, Jeddah, Saudi Arabia
- 7Department of Biology, College of Science, Princess Nourah bint Abdulrahman University, Riyadh, Saudi Arabia
- 8Pharmaceutical Research Institute, Albany College of Pharmacy and Health Sciences, New York, NY, United States
- 9Department of Zoology, College of Science, King Saud University, Riyadh, Saudi Arabia
- 10Pharmacology Department, Faculty of Veterinary Medicine, Suez Canal University, Ismailia, Egypt
- 11Riphah Institute of Pharmaceutical Sciences, Riphah International University, Lahore, Pakistan
- 12Department of Pharmacology, Faculty of Pharmaceutical Sciences, Government College University Faisalabad, Faisalabad, Pakistan
- 13Department of Pharmaceutical Chemistry, Faculty of Pharmacy, University of Dhaka, Dhaka, Bangladesh
Alzheimer’s disease (AD) is the most common form of dementia, which causes abnormalities in learning, thinking, memory, as well as behavior. Generally, symptoms of AD develop gradually and aggravate over time, and consequently severely interfere with daily activities. Furthermore, obesity is one of the common risk factors for dementia. Dysregulation of adipokine and adipocyte dysfunction are assumed to be accountable for the high risk of obesity in people that develop many related disorders such as AD. Moreover, it has been observed that the dysfunction of adipose is connected with changes in brain metabolism, brain atrophy, cognitive decline, impaired mood, neuroinflammation, impaired insulin signaling, and neuronal dysfunction in people with obesity. Conversely, the pathological mechanisms, as well as the molecular players which are involved in this association, have been unclear until now. In this article, we discuss the impact of adiponectin (AdipoQ) on obesity-related Alzheimer’s dementia.
Introduction
Alzheimer’s disease (AD) is the most frequent type of dementia among elderly individuals (Kabir et al., 2020a; Uddin et al., 2020d). It is characterized by the presence of two major hallmarks in the brain such as neurofibrillary tangles, predominantly formed by hyperphosphorylated tau proteins, and senile plaques, mainly composed of amyloid-beta (Aβ) peptides (Kabir et al., 2020b; Mamun et al., 2020). The latter hallmark is a major product of the proteolytic cleavage of the amyloid precursor protein (APP) by β- and γ-secretase enzymes (Patterson et al., 2008; Uddin et al., 2020a). A major characteristic of AD is sporadic memory damages related to hippocampal affectation. However, several pathological changes, such as oxidative stress, insulin resistance, neuroinflammation, or mitochondrial damage, associated with obesity also result in AD pathological development (Najem et al., 2014; O’Brien et al., 2017; Ferreira et al., 2018; Uddin and Kabir, 2019).
Copious reports regarding the increase in body fat mass as well as the possibility of suffering from dementia have been published in the past few years (Whitmer et al., 2005; Businaro et al., 2012; Kivimäki et al., 2018). But, their outcomes are disputed and at several times inconclusive. It appears that it is significant to distinguish between being overweight at middle-age and in late-life (Xu et al., 2011), precisely, obesity in middle-aged life and reduction of bodyweight in the preclinical phase typify dementia (Singh-Manoux et al., 2018). Indeed, in a meta-analysis of 21 trials, some authors have revealed that midlife obesity (i.e., <65years) is associated with the development of dementia, but not after 65years (Pedditizi et al., 2016). Another study investigated 1,349,857 individuals from 39 diverse cohorts with body mass index (BMI) data measured at baseline (Kivimäki et al., 2018). This study found that 20years before dementia diagnosis, increased BMI was strongly related to a higher risk of dementia in mid-life. Analyses also demonstrated that this risk was inverted among late-life where an elevated BMI could even be a defensive factor against the risk of dementia (Kivimäki et al., 2018).
Adiponectin (AdipoQ) is a 30 KDa adipokine encoded by the AdipoQ gene, mostly produced by adipocytes and is rich in human plasma (Dendana et al., 2018). AdipoQ is recognized to enhance the insulin susceptibility of target organs including muscles and liver, finally controlling fatty acid metabolism and peripheral glucose levels (Hotta et al., 2001; Yamauchi et al., 2001, 2002). Also, being a metabolic controller, AdipoQ is additionally identified for its antioxidant and anti-inflammatory effects (Takemura et al., 2007; Liu et al., 2015b). The central nervous system (CNS) is not relieved from the negative impact of obesity since obesity-related adipose malfunction has been connected to distorted neuroinflammation, brain metabolism, brain atrophy, neuronal dysfunction, cognitive decline, and impaired mood (Luppino et al., 2010; Gustafson, 2012; Arshad et al., 2018). People who suffer from obesity are under higher threat to disease progression in the case of age-linked cognitive failure, mild cognitive impairment (MCI), vascular dementia, and AD (Frisardi et al., 2010). Dysregulation of AdipoQ signaling may disrupt brain homeostasis and increase the risk of cognitive impairment. A better understanding of the impact of AdipoQ in AD is thus crucial. Particularly, restoring typical AdipoQ signaling might establish constructive, disease-modifying therapeutics against AD. Therefore, this article presents recent studies about AdipoQ on obesity-related AD.
AdipoQ and Brain Targets
AdipoQ was primarily identified in 1995 in 3T3-L1 adipocyte differentiation (Scherer et al., 1995). It is considered as one of the most abundant adipokines taking into account its plasma levels compared to several other hormones (Matsuzawa, 2005; Thundyil et al., 2012; Polito et al., 2018). AdipoQ self-assembles into bigger shapes inducing homotrimers that further self-assemble to construct hexamers or dodecamers. A globular AdipoQ resulting from the degradation of the monomer was additionally recognized (Waki et al., 2003). AdipoQ is largely produced and discharged from adipocytes, but, it is currently well-documented that these adipokines are produced by the liver, placenta, epithelial cells, pituitary cells, osteoblasts, and myocytes (Wilkinson et al., 2007; Psilopanagioti et al., 2009; Thundyil et al., 2012). Remarkably, various reports demonstrated AdipoQ mRNA expression in human pituitary (Psilopanagioti et al., 2009) and chicken diencephalon (Maddineni et al., 2005; Wilkinson et al., 2007). In the human pituitary, AdipoQ might have a role in the discharge of gonadotrophs and somatotrophs (Thundyil et al., 2012). Also, it controls a wide variety of metabolic actions such as the regulation of body-weight, lipid catabolism, glucose regulation, anti-atherogenic process, insulin sensitivity as well as endothelial function (Berg et al., 2002; Okamoto et al., 2002; Stefan and Stumvoll, 2002; Whitehead et al., 2006; Thundyil et al., 2012). Such activities are induced by three diverse receptors, namely T-cadherin (CDH13), AdipoQ receptor 1 (AdipoQR1), and AdipoQ receptor 2 (AdipoQR2), and involve various signaling cascades such as AMP-activated protein kinase (AMPK), peroxisome proliferator-activated receptor (PPAR)-α, c-Jun N-terminal kinases (JNK), p38 mitogen-activated protein kinases (p38-MAPK), and nuclear factor kappa B (NF-κB). These receptors look to be largely synthesized in the brain of various mammals including humans, mice, and rats, and their expression has been reported in distinct brain parts such as the hypothalamus, pituitary, and subcortical and cortical neurons (Degawa-Yamauchi et al., 2003; Yamauchi et al., 2003; Fry et al., 2006; Hoyda et al., 2007; Psilopanagioti et al., 2009; Thundyil et al., 2010, 2012).
Thundyil et al. (2012) studied the expression of AdipoQ receptors in the CNS and demonstrated that AdipoQR1 is mostly released in the brainstem, hypothalamus, and the pituitary gland whereas AdipoQR2 seems to be mainly released in the cortex. Moreover, AdipoQR1 is intensely released in neurons and a smaller amount in astrocytes since AdipoQR2 is believed to be weakly expressed in neurons and astrocytes (Thundyil et al., 2012). The AdipoQ gene is broadly expressed in the hippocampus and the cortex. On the other hand, the T-cadherin receptor (CDH13), which is one of the receptors mediating AdipoQ activity, seems to be spatially and temporally expressed in various neuronal cells during axon development (Ranscht and Dours-Zimmermann, 1991). Besides, T-cadherin exhibits wide expression in the brain hippocampus, cerebral cortex, amygdala, and basal ganglia in the postnatal telencephalon of Callithrix jacchus (Matsunaga et al., 2013). CDH13 was further expressed by projection neurons within the main as well as accessory olfactory bulbs. Remarkably, AdipoQ deficiency is related to higher levels of inflammatory signals in serious illness or septic patients (Venkatesh et al., 2009; Hillenbrand et al., 2010, 2012). The expression of AdipoQR1 and AdipoQR2 was recently reported in primary human astrocytes and the U373 MG human glioblastoma astrocytoma cell line (Wan et al., 2014). It was further revealed that AdipoQ mediates pro-inflammatory signaling by rising interleukin (IL)-6, and monocyte chemoattractant protein 1 (MCP-1) notably via NF-κB, extracellular signal-regulated kinase (ERK)1/2, and p38-MAPK pathways in human astrocytes (Wan et al., 2014). Additionally, it has been suggested that AdipoQ may also regulate neuroinflammation by decreasing inflammatory cytokine expression through brain endothelial cells (Spranger et al., 2006).
A study reported the decreased expression of total AdipoQ and its high molecular weight oligomers in patients with common variable immunodeficiency (Pecoraro et al., 2017). A further study also revealed different expression patterns of AdipoQR1 and AdipoQR2 in the peripheral blood mononuclear cells of these patients (Polito et al., 2019).
AdipoQ in Cognitive Function
Synapse dysfunction is the major pathological hallmark of cognitive impairment in AD (DeKosky and Scheff, 1990; Terry et al., 1991). Exciting data suggest that Aβ oligomers serve as neurotoxins that accumulate in the brain of AD patients leading to synaptic dysfunction and impaired synaptic plasticity as well as triggering synapse injury (Lambert et al., 1998; Ferreira and Klein, 2011; Forny-Germano et al., 2014). Oligomeric Aβ prevents long term potentiation (LTP) and thus stimulates long term depression (LTD) of hippocampal synapses both in vitro and in vivo (Wang et al., 2002; Shankar et al., 2008; Li et al., 2009; Jürgensen et al., 2011).
Several studies have proposed that AdipoQ signaling directly controls synaptic activity as well as plasticity, and maintaining and increasing cognitive activities in a variety of models. In anesthetized rats, intracerebroventricular administration of AdipoQ potentiates high-frequency stimulation (HFS)-mediated LTP and suppresses low-frequency stimulation (LFS)-mediated LTD (Pousti et al., 2018). Also, AdipoQ administration mediates a chemical LTP, irrespective of presynaptic incentive (Pousti et al., 2018). Treatment with a plant-derived homolog of AdipoQ (osmotin) modulated AdipoQ receptors ameliorated LTP damage and memory discrepancies in AD models (Shah et al., 2017). This activity seems to be induced by the Nogo66 receptor 1 and AdipoQR1 and comprises an outgrowth of neurite development and a rise of the dendritic spine as well as synapse density in the brain hippocampus (Zhang et al., 2016; Yoon et al., 2018).
AdipoQ-knockout mice show elevated excitability of the hippocampal dentate gyrus (DG) granule neurons together with reduced loss of contextual fear memory. AdipoQ and its analog drug AdipoRon, reestablished fear memory loss through AdipoQR2 stimulation and prevention of the excitability of DG neurons (Zhang et al., 2017). Elderly AdipoQ-knockout mice display decreased concentrations of synaptic proteins proposing synapse impairment, and poor scores in contextual fear conditioning and spatial memory tests (Ng et al., 2016). Interestingly, both osmotin and AdipoQ treatments improve learning as well as memory insufficiencies in AD animal models (Ali et al., 2015; Shah et al., 2017). Caloric constraints enhance circulating AdipoQ concentrations and increase cognition in mice perhaps through controlling the AMPK pathway in the mouse hippocampus (Ma et al., 2018). Furthermore, a clinical trial reported that patients with elevated AdipoQ concentrations exert better performances in a prolonged word memory test, advocating the idea that AdipoQ is a protective factor against cognitive failure suggesting a novel therapeutic approach in cognitive decline (Cezaretto et al., 2018).
AdipoQ in Alzheimer’s Hallmarks
Animal Studies
Study suggests that insufficiency in AdipoQ signaling can mediate an AD-like symptom in mice models (Table 1; Ng et al., 2016). Aged AdipoQ-deficient mice recapitulate various features of AD pathology, such as increased levels of Aβ, synapse loss, neuroinflammation, tau hyperphosphorylation, neuronal cell death, and reduced insulin signaling. Significantly, aged AdipoQ deficient mice accomplished poor activity upon fear conditioning behavioral experiments and spatial memory (Ng et al., 2016). This study also indicated that chronic deficiency of AdipoQ in aged mice inactivates AMPK signaling thus causing insulin desensitization and provokes AD-like cognitive impairments as shown in Figure 1. The above interpretations were again validated by another analysis showing that gene-therapy mediated AdipoQR1 suppression further induces AD-like pathogenesis, which comprises dysfunction in the spatial memory as well as learning, insulin signaling deficiency, elevated concentrations of Aβ accretions and hyperphosphorylated tau, neuroinflammation as well as other neurodegenerative events (Kim et al., 2017). These analyses afford convincing reports for the implication of AdipoQ deficiency in the pathogenesis of AD. The nucleus basalis magnocellularis (NBM) is a well-known cholinergic nucleus in the basal forebrain that is disturbed rigorously in AD along with other neurodegenerative disorders. It has been reported that the dysfunction of NBM cholinergic neurons influences cholinergic loss and, more notably, is associated with clinical events of dementia (Arendt et al., 1983; Iraizoz et al., 1999; Liu et al., 2015a). Fascinatingly, NBM was reported to be an eminent site of AdipoQR1 expression (Psilopanagioti et al., 2009). Hence, it is likely that a lack of AdipoQ stimulates the development of AD by stimulating NBM degeneration.
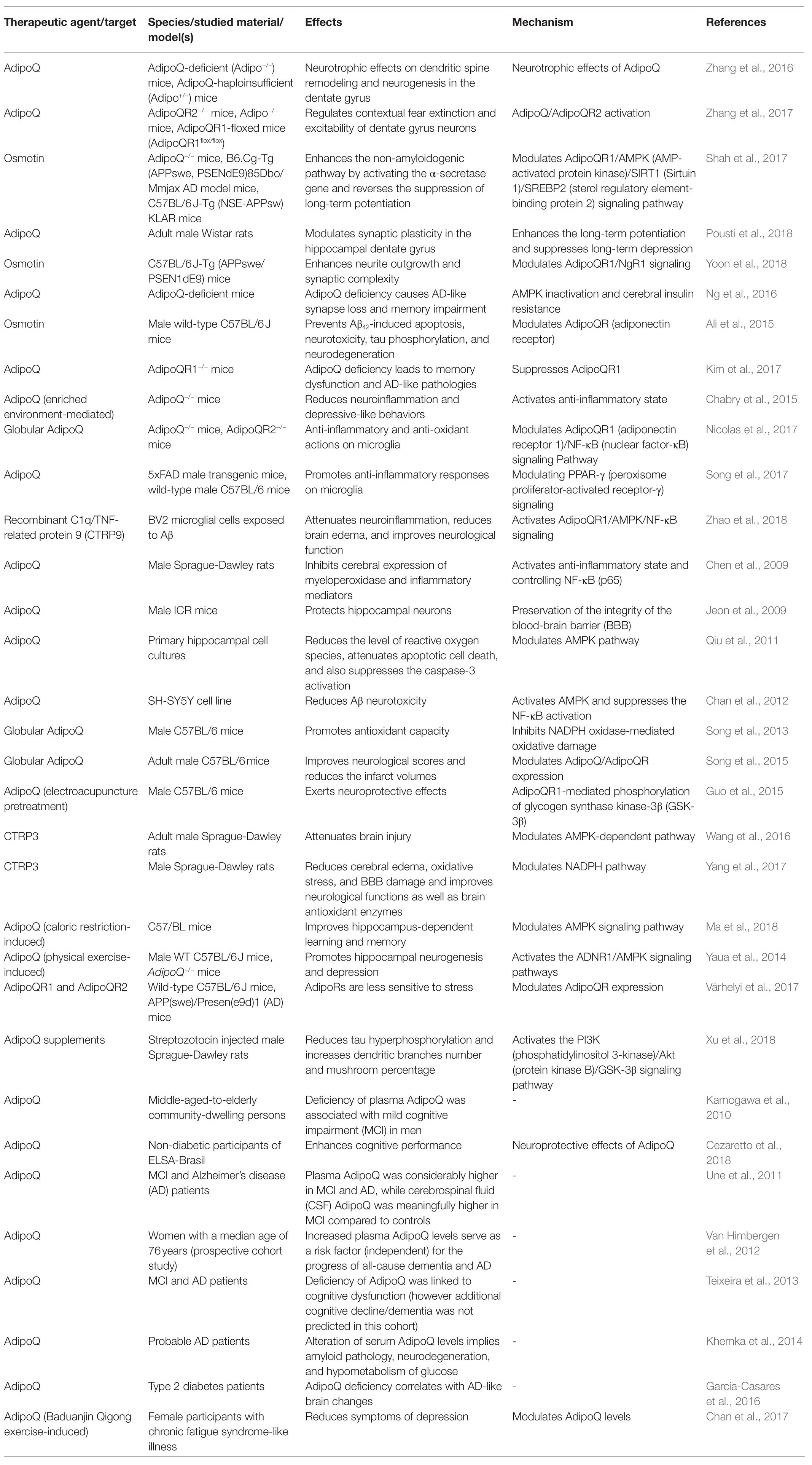
Table 1. Several promising studies of the role of AdipoQ signaling in physiopathological processes associated with Alzheimer’s disease.
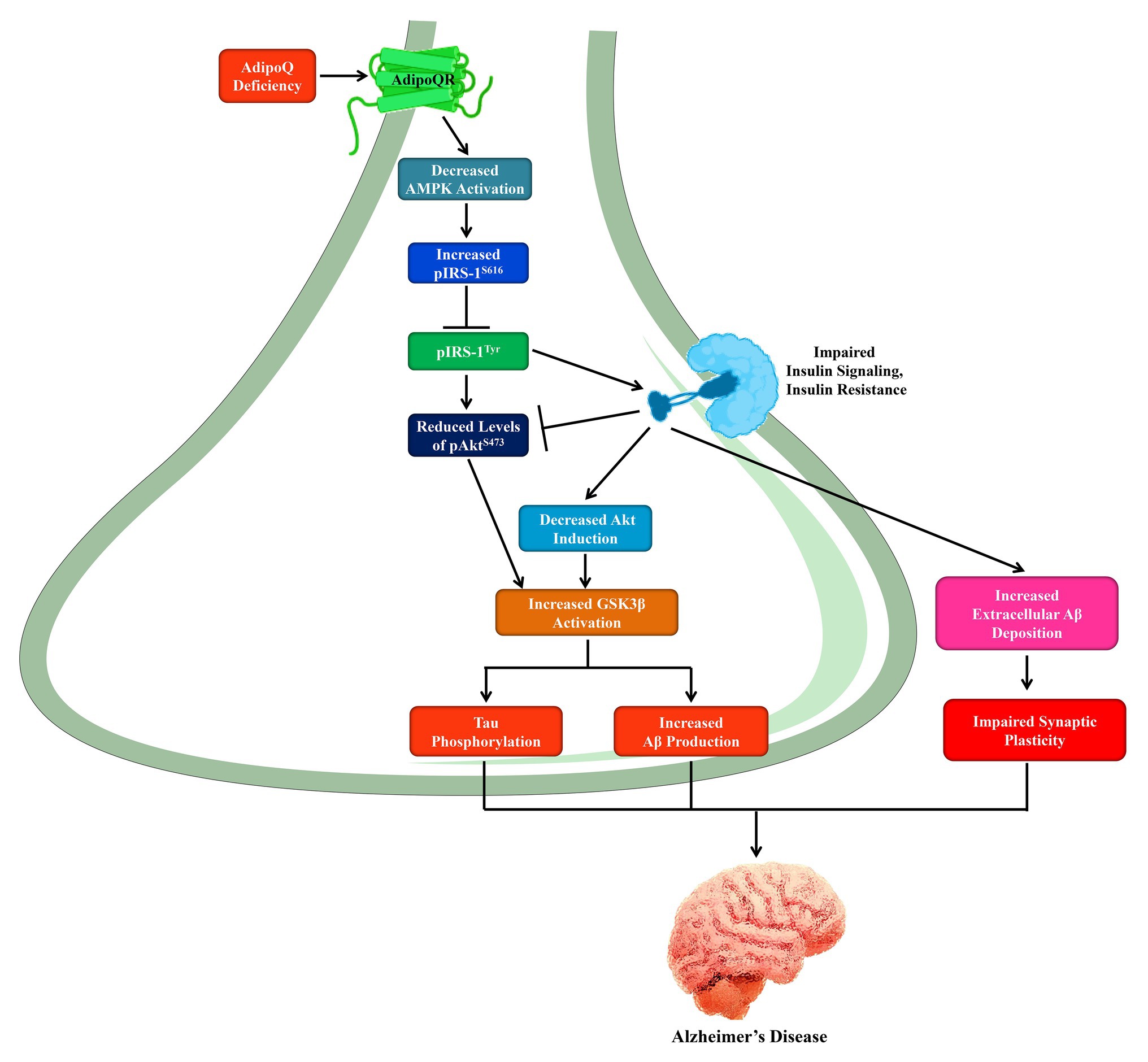
Figure 1. The role of AdipoQ deficiency in the pathogenesis of Alzheimer’s disease through AMPK inactivation. In neurons, AdipoQ deficiency leads to decreased AMPK activation and increased phosphorylation of IRS-1S616 that inhibits the pIRS-1Tyr formation, and thus deactivates insulin signaling. On the other hand, insulin resistance is gradually developed and reduces pAktS473 Levels. Thus, decreased Akt induction and increased GSK3β activation results in increased Aβ production and tau phosphorylation. Furthermore, insulin resistance exacerbates the extracellular Aβ deposition as well as impaired synaptic plasticity. AMPK, AMP-activated protein kinase; IRS, insulin receptor substrate; GSK3β, glycogen synthase kinase 3β; Akt, protein kinase B; Aβ, amyloid beta peptide.
Conversely, loss in AdipoQ activity was also detected in amyloid-induced AD prototypes, while triggering the AdipoQ signaling pathway declines AD-like phenotypes. APP/PS1 mice displaying alterations in the levels of AdipoQ receptor expression seemed less reactive to a stress-mediating mice model as compared to control mice (Várhelyi et al., 2017). In an identical paradigm, osmotin improved AD-like phenotypes, for example, Aβ formation and deposition, synaptic loss and deficient LTP, and cognitive decline and memory loss. AdipoQR1 silencing eliminates the beneficial effects of osmotin and additionally aggravates brain pathology in AD-mice (Shah et al., 2017). Osmotin was also found to diminish Aβ accumulation in the cultured cells of SH-SY5Y human neuroblastoma overexpressing APP. Osmotin actions are induced by stimulation of the AMP kinase, an enzyme downregulated by oligomeric Aβ in hippocampal neurons (Seixas da Silva et al., 2017). An intracerebroventricular dose of AdipoQ recovered cognitive decline and reduced glycogen synthase kinase 3β (GSK3β)-induced tau hyperphosphorylation in AD-related areas in a rat paradigm of streptozotocin-mediated brain pathology (Xu et al., 2018). These reports propose the stimulation of the AdipoQ signaling pathway, predominantly via AdipoQR1, as a novel therapeutic target in AD (Ng and Chan, 2017). Considering this, chronic administration of a widely used anti-AD acetylcholinesterase antagonist (i.e., donepezil) was found to elevate serum concentrations of AdipoQ (Pákáski et al., 2014). Furthermore, thiazolidinediones (TZDs) including pioglitazone and rosiglitazone, as well as PPARγ agonists (i.e., used for type 2 diabetes mellitus; Saltiel and Olefsky, 1996; Malinowski and Bolesta, 2000; Hong et al., 2018) were just repurposed to combat AD. The insulin-sensitizing function of TZDs is in part induced by triggering peripheral AdipoQ receptors and AdipoQ gene expression (Yu et al., 2002; Tsuchida et al., 2005; Nie and Li, 2017). Consequently, it is feasible that AdipoQ exerts part of the experimental benefits of TZDs and donepezil.
AdipoRon, an AdipoQ receptor agonist (Okada-Iwabu et al., 2013), has been shown to regulate hippocampal synaptic transmission and to accelerate fear memory loss in rodents (Zhang et al., 2017). Also, AdipoRon was shown to control dopaminergic neuronal activity in the ventral-tegmental site (Sun et al., 2019) and act as a metabolic and antidepressant controller in a mouse model of depression (Nicolas et al., 2018). Notably, central AdipoRon activities were achieved by peripheral inoculation, and it has been found that this drug passes the blood-brain barrier (BBB) to trigger AdipoQ receptors in the brain. It would be exciting to further examine the effects of this AdipoQ-drug analog with translational potential in AD models.
Human Studies
In humans, analyses relating AdipoQ concentrations to AD are debatable. Improved baseline AdipoQ concentrations in plasma were found to be involved with an elevated risk of developing AD and other forms of dementia in women, but not men (Table 1; van Himbergen et al., 2012). Moreover, increased AdipoQ levels were revealed in the cerebrospinal fluid (CSF) and plasma of individuals with MCI as well as sporadic AD (Une et al., 2011; Khemka et al., 2014), while plasma concentrations of AdipoQ was positively associated with dementia. The relationship between AD and increased blood AdipoQ levels has been advocated by meta-analysis (Ma et al., 2016). Though the afore-mentioned reports suggest elevated AdipoQ concentrations to be related to Alzheimer’s dementia, conflicting effects have also been described. Data analysis has shown decreased concentrations of AdipoQ in MCI and AD individuals. Furthermore, based on the AdipoQ levels, one is not able to foresee the development of cognitive decline from normal conditions to MCI and from MCI to AD, respectively (Teixeira et al., 2013). Another report also revealed that reduced concentrations of AdipoQ in plasma were involved with MCI, although this link was identified in males, but not in females (Kamogawa et al., 2010). From these experiments, it has been found that decreased levels of plasma AdipoQ in diabetic patients is associated with a lower volume of gray-matter and lower glucose uptake in the temporal region of the brain, comparable to what is perceived in AD (García-Casares et al., 2016). Furthermore, another study reveals that AdipoQ concentrations in blood are elevated but the lower levels are reported in CSF of MCI and AD individuals. Existing studies concerning a link between AdipoQ concentrations in blood and CSF to MCI and AD are inconsistent and questionable. Hence, more analyses are needed to explore the precise impact of AdipoQ. Several reports have shown that the relationship between dementia and AdipoQ levels might be sex-dependent (Kamogawa et al., 2010). This might be particularly significant in the case of AD, where a significant sexual dichotomy has been reported with females being at a considerably increased risk of developing AD than males (Uddin et al., 2020c).
AdipoQ in Neuroinflammatory Signaling of Alzheimer’s Disease
The brain of AD patients exerts a prolonged state of low-level inflammation, which is induced by the activation of microglial cells that leads to the secretion of several inflammatory cytokines such as interleukin (IL)-6, tumor necrosis factor-α (TNFα), and IL-1β (Bamberger et al., 2003; Lourenco et al., 2013; Heneka et al., 2015; Hansen et al., 2018; Uddin et al., 2020b). These cytokines induce various harmful incidents in the AD brain such as endoplasmic reticulum stress, neuronal insulin resistance, synaptotoxicity, and finally neurodegeneration (Bomfim et al., 2012; Lourenco et al., 2013; Rizzo et al., 2018).
AdipoQ-deficient mice possess a variety of clinical features in the AD brain, such as decreased concentrations of synaptic proteins, insulin resistance, and the existence of neuroinflammatory markers including astrogliosis, microgliosis, and higher concentrations of the inflammatory cytokines such as IL-1β and TNFα (Ng et al., 2016). Environmental upgrading like housing situations that stimulate social interactions, cognitive engagement, and physical activity, has been found to increase cognitive activities in AD mice. These results are at least in part owing to the alteration of the microglial effect to the insults of neurotoxic Aβ oligomers (Xu et al., 2016; Vieira and Beckman, 2017). Recently, it has been shown that beneficial outcomes of environmental upgrading to the brain are induced by AdipoQ including the advancement of an anti-inflammatory state of microglia with the lower secretion of inflammatory cytokines (Chabry et al., 2015; Nicolas et al., 2015). It has also been reported that globular AdipoQ prevents the microglia inflammatory profile directly in vitro and in vivo (Nicolas et al., 2017) via a mechanism involving NF-κB and AdipoQR1. AdipoQ also regulates the in vitro activation of microglia under Aβ toxicity through PPARγ stimulation (Song et al., 2017). Another report suggesting the anti-inflammatory effects of AdipoQ in the CNS shows that an AdipoQR1 agonist (i.e., CTRP9) reduces neuroinflammatory effects in an in vivo mouse model of intracerebral hemorrhage via AdipoQR1/AMPK/NF-κB signaling pathways (Zhao et al., 2018). Besides, AdipoRon usage was found to block the recruitment of macrophages in an experimental model of spinal cord damage (Zhou et al., 2019).
AdipoQ was reported to prevent the pro-inflammatory response, remarkably by blocking IL-6 discharge from BBB endothelial cells (Spranger et al., 2006). It has been shown that AdipoQ regulates inflammatory signaling indirectly through the BBB by adversely modulating TNFα and IL-6 release. Laboratory analysis of hippocampal neurons has indicated that AdipoQ mediates neuroprotective effects via the AMPK signaling pathway (Qiu et al., 2011). Such effects are additionally supported by experiments demonstrating that AdipoQ knockout mice show enlarged brain injuries and increased neurological deficits after ischemia-reperfusion compared with wild-type mice (Nishimura et al., 2008). These studies provide evidence that the neuroprotective effect of AdipoQ is induced via an endothelial nitric oxide synthase (eNOS)-reliant mechanism (Nishimura et al., 2008). Thus the neuroprotective role of AdipoQ takes part in the regulation of brain inflammation; it was proposed that the lack of AdipoQ in obesity might induce neuroinflammation leading to AD and other dementias.
AdipoQ in Insulin Signaling of Alzheimer’s Disease
Neuronal insulin signaling plays an important role in memory and synaptic plasticity, mostly by controlling glutamate receptor transport (Beattie et al., 2000; Man et al., 2000; Skeberdis et al., 2001; Zhao et al., 2004). Defective insulin signaling is well-reported both in AD individuals and in a number of AD animal models (Steen et al., 2005; Bomfim et al., 2012; Talbot et al., 2012). Impaired insulin signaling is responsible for the neuronal loss and cognitive decline in AD (Ferreira et al., 2014; Vieira et al., 2018). The synaptotoxicity of Aβ is associated with a loss of insulin receptor activity in vivo and in vitro and can be inhibited by using insulin itself and by insulin-sensitizing medications (De Felice et al., 2009; Bomfim et al., 2012; Batista et al., 2018). These findings prompted numerous groups to investigate the activity of various types of anti-diabetic therapies in AD prototypes, and progressive preclinical outcomes triggered researchers to complete human clinical tests (De Felice, 2013; Yarchoan and Arnold, 2014; de la Monte, 2017). In this regard, attention has been focused on the insulin-sensitizing activities of AdipoQ to rectify the abnormal insulin signaling in AD.
A diminished brain insulin signaling cascade was detected, along with various other AD-resembling pathological symptoms, in AdipoQ-deficient mice as well as in AdipoQR1-knockout mice (Ng et al., 2016; Kim et al., 2017). In contrast, AdipoQ was found to enhance insulin susceptibility in the SH-SY5Y neuroblastoma cell line exhibiting insulin resistance, via AdipoQR1 initiation of AMPK (Ng et al., 2016). These studies reveal that AdipoQ possesses the possibility to reestablish neuronal insulin signaling, with potential therapeutic effects for AD and also other neurodegenerative disorders (Bloemer et al., 2018). Nevertheless, more translational analyses using AD models are needed to authenticate the neuroprotective role of AdipoQ as therapeutic interventions to halt brain insulin resistance in AD.
Other studies suggested that insulin resistance (Capurso and Capurso, 2012; Johnson and Olefsky, 2013; Medina-Urrutia et al., 2015) is associated with an excess of adiposity induced by high free fatty acid (Perseghin et al., 2003) and lower levels of plasma AdipoQ (Arita et al., 2012). Furthermore, acute lowering of free fatty acid is linked to decreased AdipoQ levels (Bernstein et al., 2004). A study has revealed that the altered plasma status of both docosahexaenoic acid and other fatty acids unrelated to docosahexaenoic acid are linked to AD (Cunnane et al., 2012).
AdipoQ Paradox in Alzheimer’s Disease
AdipoQ is a well-known neuroprotective agent against various cytotoxicities induced by Aβ as well as MPP+ in vitro (Jung et al., 2006; Chan et al., 2012). In mice brains, AdipoQ is protected from kainic acid-mediated excitotoxicity in the hippocampus (Jeon et al., 2009). Particularly, AdipoQ may control neurogenesis. Regarding this concept, AdipoQ has been reported to incite proliferation of mature hippocampal neural stem/progenitor cells via signaling pathways including GSK3β/p38-MAPK/β-catenin (Zhang et al., 2011). Moreover, it has been shown that physical exercise triggers hippocampal neurogenesis, facilitated by AdipoQ (Yaua et al., 2014). Furthermore, AdipoQ was found to be neurotrophic for spinogenesis and dendritic arborization in the DG in mice brains (Zhang et al., 2016). Several neuropathological characteristics, e.g., compromised motor activity and protein accumulation were improved by AdipoQ treatment in mouse experiments of α-synucleinopathies (Sekiyama et al., 2014). It was previously demonstrated that elderly AdipoQ-defective mice initiated an AD-like pathology linked with dysregulation of insulin receptor signaling (Ng et al., 2016).
The outcome of a cohort study performed by the Mayo Clinic Study of Aging indicated that elevated levels of plasma AdipoQ were interrelated with imaging data for cortical and hippocampal volumes, cognitive declines, and positron emission tomography (Wennberg et al., 2016). Therefore, the obtained results advocate that higher AdipoQ predicts cognitive decline and neurodegeneration in aging (Wennberg et al., 2016). Amazingly, these experimental outcomes were significant in females but not in males, which is consistent with the analyses of the Framingham Heart Study (van Himbergen et al., 2012). Consistent with the prediction that AdipoQ might be included in neurodegenerative pathogenesis, histopathological studies of an autopsy of an AD brain demonstrated that AdipoQ was segregated into the neurofibrillary tangles by tau (Waragai et al., 2016). AdipoQ also co-localized with Lewy bodies in the brain of dementia patients (Sekiyama et al., 2014). Along with plasma data regarding AdipoQ, the overexpression of AdipoQ might be associated with the progression of neurodegenerative disorders like AD. In a retrospective cohort study, Qizilbash et al. (2015) examined the involvement of BMI in developing dementia. Both underweight middle-aged and elderly people displayed an elevated probability of developing dementia within the next two decades. The presence of dementia started to decline with an increase in BMI, with highly obese patients (BMI >40kg/m2) developing a 29% reduction (95% CI: 22-36) in the possibility of developing dementia than those of healthy-weight individuals.
Until now, the mode of hyperadiponectinemia in AD has not been clear. Hyperadiponectinemia in AD is expected to be a compensatory response to the lower efficiency of the insulin/insulin-like growth factor 1 (IGF-1) receptor signaling cascade during neurodegeneration (Waragai et al., 2017). As the disease progresses, AdipoQ might be increased as well as sequestered by tau, which ultimately leads to the aggregation of neurotoxic proteins in the AD brain (Figure 2; Waragai et al., 2016, 2017). A different alternative mechanism is that the misfolding of AdipoQ might downregulate the insulin/AdipoQ signal transduction network causing the reduction of neuroprotective and neurotrophic functions (Waragai et al., 2017). Therefore, it is expected that a mutation of AdipoQ may trigger synaptic dysfunction and neuronal death in AD.
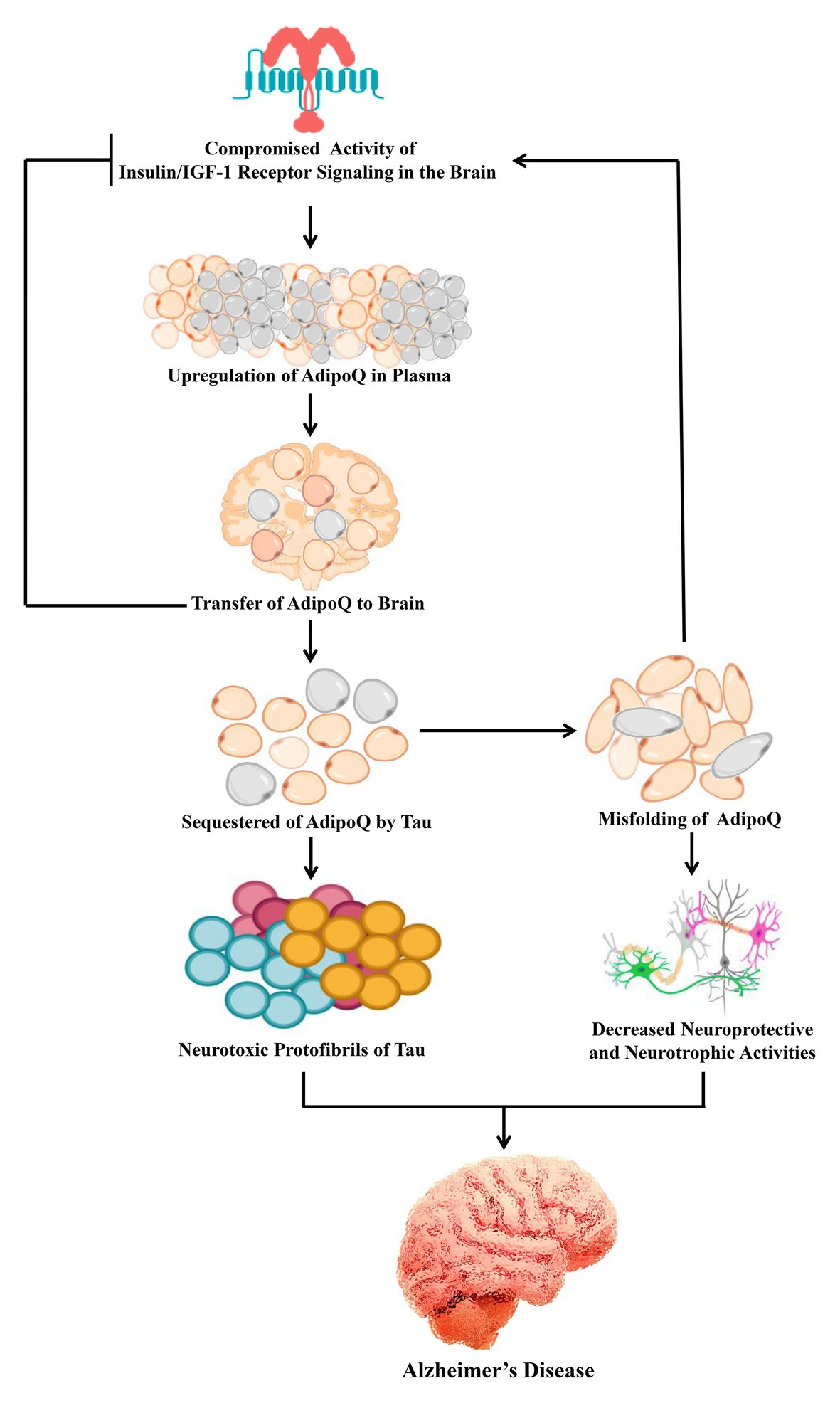
Figure 2. Possible mechanism of AdipoQ signaling in Alzheimer’s disease. In the brain, insulin resistance is a common feature of Alzheimer’s disease. AdipoQ is upregulated to compensate for the compromised activity of insulin/insulin/IGF-1 receptor signaling. As a result the plasma levels of AdipoQ are transferred to the brain and improve the compromised insulin signaling. Furthermore, AdipoQ is sequestered by tau which leads to the formation of neurotoxic protein aggregation. On the other hand, such sequestration of AdipoQ is associated with its misfolding which leads to the downregulation of insulin signaling as well as decreased neuroprotective and neurotrophic activities. IGF-1, insulin-like growth factor 1.
Conclusion
Obesity in middle-aged people might be considered as a critical factor in the development and progression of AD in the elderly. The higher possibility of people with obesity to develop AD reveals the ability of adipose tissue to connect with the brain and influence its activity. AdipoQ signaling has been found to interact with a variety of neuropathological incidents such as Aβ formation and deposition, tau hyperphosphorylation, neuroinflammation, insulin resistance, cognitive damage, and synaptic loss. The phenotypes of AdipoQ or AdipoQ receptor-knockout mice recapitulates most of the AD neuropathological features. Thus, abnormal AdipoQ signaling may induce obesity-mediated harmful effects on the CNS and increase the risk of cognitive impairment and AD. Notably, in the brain, restoring typical AdipoQ signaling might establish constructive, disease-modifying therapeutics to combat AD.
Author Contributions
MU conceived the original, designed the outlines of the study, and prepared the figures for the manuscript. MU, MR, and MS wrote the draft of the manuscript. PJ edited the whole manuscript. MSA, GA, MB-J, SM, MA-D, MFA, and AS revised and improved the draft. All authors have read and approved the final manuscript.
Conflict of Interest
The authors declare that the research was conducted in the absence of any commercial or financial relationships that could be construed as a potential conflict of interest.
Acknowledgments
This work was funded by the Deanship of Scientific Research at Princess Nourah bint Abdulrahman University, through the Fast-Track Research Funding Program. This work was supported by King Saud University, Deanship of Scientific Research, College of Science Research Center.
Abbreviations
AD, Alzheimer’s disease; AdipoQ, adiponectin; Aβ, amyloid-beta; APP, amyloid precursor protein; BMI, body mass index; MCI, mild cognitive impairment; AdipoQR1, AdipoQ receptor 1; AdipoQR2, AdipoQ receptor 2.
References
Ali, T., Yoon, G. H., Shah, S. A., Lee, H. Y., and Kim, M. O. (2015). Osmotin attenuates amyloid beta-induced memory impairment, tau phosphorylation and neurodegeneration in the mouse hippocampus. Sci. Rep. 5:11708. doi: 10.1038/srep11708
Arendt, T., Bigl, V., and Arendt, A. T. A. (1983). Loss of neurons in the nucleus basalis of Meynert in Alzheimer’s disease, paralysis agitans and Korsakoff’s disease. Acta Neuropathol. 61, 101–108.
Arita, Y., Kihara, S., Ouchi, N., Takahashi, M., Maeda, K., Miyagawa, J. -i., et al. (2012). Paradoxical decrease of an adipose-specific protein, adiponectin, in obesity. 1999. Biochem. Biophys. Res. Commun. 425, 560–564. doi: 10.1016/j.bbrc.2012.08.024
Arshad, N. A., Lin, T. S., and Yahaya, M. F. (2018). Metabolic syndrome and its effect on the brain: possible mechanism. CNS Neurol. Disord. Drug Targets 17, 595–603. doi: 10.2174/1871527317666180724143258
Bamberger, M. E., Harris, M. E., McDonald, D. R., Husemann, J., and Landreth, G. E. (2003). A cell surface receptor complex for fibrillar β-amyloid mediates microglial activation. J. Neurosci. 23, 2665–2674. doi: 10.1523/JNEUROSCI.23-07-02665.2003
Batista, A. F., Forny-Germano, L., Clarke, J. R., Lyra e Silva, N. M., Brito-Moreira, J., Boehnke, S. E., et al. (2018). The diabetes drug liraglutide reverses cognitive impairment in mice and attenuates insulin receptor and synaptic pathology in a non-human primate model of Alzheimer’s disease. J. Pathol. 245, 85–100. doi: 10.1002/path.5056
Beattie, E. C., Carroll, R. C., Yu, X., Morishita, W., Yasuda, H., von Zastrow, M., et al. (2000). Regulation of AMPA receptor endocytosis by a signaling mechanism shared with LTD. Nat. Neurosci. 3, 1291–1300. doi: 10.1038/81823
Berg, A. H., Combs, T. P., and Scherer, P. E. (2002). ACRP30/adiponectin: an adipokine regulating glucose and lipid metabolism. Trends Endocrinol. Metab. 13, 84–89. doi: 10.1016/S1043-2760(01)00524-0
Bernstein, E. L., Koutkia, P., Ljungquist, K., Breu, J., Canavan, B., and Grinspoon, S. (2004). Acute regulation of adiponectin by free fatty acids. Metabolism 53, 790–793. doi: 10.1016/j.metabol.2003.12.023
Bloemer, J., Pinky, P. D., Govindarajulu, M., Hong, H., Judd, R., Amin, R. H., et al. (2018). Role of Adiponectin in central nervous system disorders. Neural Plast. 2018:4593530. doi: 10.1155/2018/4593530
Bomfim, T. R., Forny-Germano, L., Sathler, L. B., Brito-Moreira, J., Houzel, J. C., Decker, H., et al. (2012). An anti-diabetes agent protects the mouse brain from defective insulin signaling caused by Alzheimer’s disease-associated Aβ oligomers. J. Clin. Invest. 122, 1339–1353. doi: 10.1172/JCI57256
Businaro, R., Ippoliti, F., Ricci, S., Canitano, N., and Fuso, A. (2012). Alzheimer’s disease promotion by obesity: induced mechanisms-molecular links and perspectives. Curr. Gerontol. Geriatr. Res. 2012:986823. doi: 10.1155/2012/986823
Capurso, C., and Capurso, A. (2012). From excess adiposity to insulin resistance: the role of free fatty acids. Vasc. Pharmacol. 57, 91–97. doi: 10.1016/j.vph.2012.05.003
Cezaretto, A., Suemoto, C. K., Bensenor, I., Lotufo, P. A., De Almeida-Pititto, B., and Ferreira, S. R. G. (2018). Association of adiponectin with cognitive function precedes overt diabetes in the Brazilian longitudinal study of adult health: ELSA. Diabetol. Metab. Syndr. 10:54. doi: 10.1186/s13098-018-0354-1
Chabry, J., Nicolas, S., Cazareth, J., Murris, E., Guyon, A., Glaichenhaus, N., et al. (2015). Enriched environment decreases microglia and brain macrophages inflammatory phenotypes through adiponectin-dependent mechanisms: relevance to depressive-like behavior. Brain Behav. Immun. 50, 275–287. doi: 10.1016/j.bbi.2015.07.018
Chan, K. H., Lam, K. S. L., Cheng, O. Y., Kwan, J. S. C., Ho, P. W. L., Cheng, K. K. Y., et al. (2012). Adiponectin is protective against oxidative stress induced cytotoxicity in amyloid-Beta neurotoxicity. PLoS One 7:e52354. doi: 10.1371/journal.pone.0052354
Chan, J. S. M., Li, A., Ng, S. M., Ho, R. T. H., Xu, A., Yao, T. J., et al. (2017). Adiponectin potentially contributes to the antidepressive effects of baduanjin qigong exercise in women with chronic fatigue syndrome-like illness. Cell Transplant. 26, 493–501. doi: 10.3727/096368916X694238
Chen, B., Liao, W. Q., Xu, N., Xu, H., Wen, J. Y., Yu, C. A., et al. (2009). Adiponectin protects against cerebral ischemia-reperfusion injury through anti-inflammatory action. Brain Res. 1273, 129–137. doi: 10.1016/j.brainres.2009.04.002
Cunnane, S. C., Schneider, J. A., Tangney, C., Tremblay-Mercier, J., Fortier, M., Bennett, D. A., et al. (2012). Plasma and brain fatty acid profiles in mild cognitive impairment and alzheimer’s disease. J. Alzheimers Dis. 29, 691–697. doi: 10.3233/JAD-2012-110629
De Felice, F. G. (2013). Alzheimer’s disease and insulin resistance: translating basic science into clinical applications. J. Clin. Invest. 123, 531–539. doi: 10.1172/JCI64595
De Felice, F. G., Vieira, M. N. N., Bomfim, T. R., Decker, H., Velasco, P. T., Lambert, M. P., et al. (2009). Protection of synapses against Alzheimer’s-linked toxins: insulin signaling prevents the pathogenic binding of Aβ oligomers. Proc. Natl. Acad. Sci. U. S. A. 106, 1971–1976. doi: 10.1073/pnas.0809158106
de la Monte, S. M. (2017). Insulin resistance and neurodegeneration: progress towards the development of new therapeutics for Alzheimer’s disease. Drugs 77, 47–65. doi: 10.1007/s40265-016-0674-0
Degawa-Yamauchi, M., Bovenkerk, J. E., Juliar, B. E., Watson, W., Kerr, K., Jones, R., et al. (2003). Serum Resistin (FIZZ3) protein is increased in obese humans. J. Clin. Endocrinol. Metab. 88, 5452–5455. doi: 10.1210/jc.2002-021808
DeKosky, S. T., and Scheff, S. W. (1990). Synapse loss in frontal cortex biopsies in Alzheimer’s disease: correlation with cognitive severity. Ann. Neurol. 27, 457–464. doi: 10.1002/ana.410270502
Dendana, M., Bahia, W., Finan, R. R., Al-Mutawa, M., and Almawi, W. Y. (2018). Association of adiponectin gene variants with idiopathic recurrent miscarriage according to obesity status: a case-control study. J. Transl. Med. 16:76. doi: 10.1186/s12967-018-1453-3
Ferreira, S. T., Clarke, J. R., and Bomfim, T. R., and De Felice, F. G. (2014). Inflammation, defective insulin signaling, and neuronal dysfunction in Alzheimer’s disease. Alzheimers Dement. 10, S76–S83. doi: 10.1016/j.jalz.2013.12.010
Ferreira, L. S. S., Fernandes, C. S., Vieira, M. N. N., and De Felice, F. G. (2018). Insulin resistance in Alzheimer’s disease. Front. Neurosci. 12:830. doi: 10.3389/fnins.2018.00830
Ferreira, S. T., and Klein, W. L. (2011). The Aβ oligomer hypothesis for synapse failure and memory loss in Alzheimer’s disease. Neurobiol. Learn. Mem. 96, 529–543. doi: 10.1016/j.nlm.2011.08.003
Forny-Germano, L., Lyra, E., Silva, N. M., Batista, A. F., Brito-Moreira, J., Gralle, M., et al. (2014). Alzheimer’s disease-like pathology induced by amyloid-β oligomers in nonhuman primates. J. Neurosci. 34, 13629–13643. doi: 10.1523/JNEUROSCI.1353-14.2014
Frisardi, V., Solfrizzi, V., Seripa, D., Capurso, C., Santamato, A., Sancarlo, D., et al. (2010). Metabolic-cognitive syndrome: a cross-talk between metabolic syndrome and Alzheimer’s disease. Ageing Res. Rev. 9, 399–417. doi: 10.1016/j.arr.2010.04.007
Fry, M., Smith, P. M., Hoyda, T. D., Duncan, M., Ahima, R. S., and Sharkey, K. A., et al. (2006). Area postrema neurons are modulated by the adipocyte hormone adiponectin. J. Neurosci. 26, 9695–9702. doi: 10.1523/JNEUROSCI.2014-06.2006
García-Casares, N., García-Arnés, J. A., Rioja, J., Ariza, M. J., Gutiérrez, A., Alfaro, F., et al. (2016). Alzheimer’s like brain changes correlate with low adiponectin plasma levels in type 2 diabetic patients. J. Diabetes Complicat. 30, 281–286. doi: 10.1016/j.jdiacomp.2015.12.001
Guo, F., Jiang, T., Song, W., Wei, H., Wang, F., Liu, L., et al. (2015). Electroacupuncture attenuates cerebral ischemia-reperfusion injury in diabetic mice through adiponectin receptor 1-mediated phosphorylation of GSK-3β. Mol. Neurobiol. 51, 685–695. doi: 10.1007/s12035-014-8765-y
Gustafson, D. R. (2012). Adiposity and cognitive decline: underlying mechanisms. J. Alzheimers Dis. 30, 203–217. doi: 10.3233/JAD-2012-120487
Hansen, D. V., Hanson, J. E., and Sheng, M. (2018). Microglia in Alzheimer’s disease. J. Cell Biol. 127, 3240–3249. doi: 10.1083/jcb.201709069
Heneka, M. T., Carson, M. J., EI, Khoury J., Landreth, G. E., Brosseron, F., Feinstein, D. L., et al. (2015). Neuroinflammation in Alzheimer’s disease. Lancet Neurol. 14, 388–405. doi: 10.1016/S1474-4422(15)70016-5
Hillenbrand, A., Knippschild, U., Weiss, M., Schrezenmeier, H., Henne-Bruns, D., Huber-Lang, M., et al. (2010). Sepsis induced changes of adipokines and cytokines - septic patients compared to morbidly obese patients. BMC Surg. 10:26. doi: 10.1186/1471-2482-10-26
Hillenbrand, A., Weiss, M., Knippschild, U., Wolf, A. M., and Huber-Lang, M. (2012). Sepsis-induced adipokine change with regard to insulin resistance. Int. J. Inflamm. 2012:972368. doi: 10.1155/2012/972368
Hong, F., Xu, P., and Zhai, Y. (2018). The opportunities and challenges of peroxisome proliferator-activated receptors ligands in clinical drug discovery and development. Int. J. Mol. Sci. 19:2189. doi: 10.3390/ijms19082189
Hotta, K., Funahashi, T., Bodkin, N. L., Ortmeyer, H. K., Arita, Y., and Hansen, B. C., et al. (2001). Circulating concentrations of the adipocyte protein adiponectin are decreased in parallel with reduced insulin sensitivity during the progression to type 2 diabetes in rhesus monkeys. Diabetes 50, 1126–1133. doi: 10.2337/diabetes.50.5.1126
Hoyda, T. D., Fry, M., Ahima, R. S., and Ferguson, A. V. (2007). Adiponectin selectively inhibits oxytocin neurons of the paraventricular nucleus of the hypothalamus. J. Physiol. 585, 805–816. doi: 10.1113/jphysiol.2007.144519
Iraizoz, I., Guijarro, J. L., Gonzalo, L. M., and de, L. S. (1999). Neuropathological changes in the nucleus basalis correlate with clinical measures of dementia. Acta Neuropathol. 98, 186–196. doi: 10.1007/s004010051068
Jeon, B. T., Shin, H. J., Kim, J. B., Kim, Y. K., Lee, D. H., Kim, K. H., et al. (2009). Adiponectin protects hippocampal neurons against kainic acid-induced excitotoxicity. Brain Res. Rev. 61, 81–88. doi: 10.1016/j.brainresrev.2009.05.002
Johnson, A. M. F., and Olefsky, J. M. (2013). The origins and drivers of insulin resistance. Cell 152, 673–684. doi: 10.1016/j.cell.2013.01.041
Jung, T. W., Lee, J. Y., Shim, W. S., Kang, E. S., Kim, J. S., Ahn, C. W., et al. (2006). Adiponectin protects human neuroblastoma SH-SY5Y cells against MPP +-induced cytotoxicity. Biochem. Biophys. Res. Commun. 343, 564–570. doi: 10.1016/j.bbrc.2006.02.186
Jürgensen, S., Antonio, L. L., Mussi, G. E. A., Brito-Moreira, J., Bomfim, T. R., De Felice, F. G., et al. (2011). Activation of D1/D5 dopamine receptors protects neurons from synapse dysfunction induced by amyloid-β oligomers. J. Biol. Chem. 286, 3270–3276. doi: 10.1074/jbc.M110.177790
Kabir, M. T., Uddin, M. S., Al, Mamun A., Jeandet, P., Aleya, L., Mansouri, R. A., et al. (2020a). Combination drug therapy for the management of Alzheimer’s disease. Int. J. Mol. Sci. 21:3272. doi: 10.3390/ijms21093272
Kabir, M. T., Uddin, M. S., Mathew, B., Das, P. K., Perveen, A., and Ashraf, G. M. (2020b). Emerging promise of immunotherapy for Alzheimer’s disease: a new hope for the development of Alzheimer’s vaccine. Curr. Top. Med. Chem. 20, 1214–1234. doi: 10.2174/1568026620666200422105156
Kamogawa, K., Kohara, K., Tabara, Y., Uetani, E., Nagai, T., Yamamoto, M., et al. (2010). Abdominal fat, adipose-derived hormones and mild cognitive impairment: the J-SHIPP study. Dement. Geriatr. Cogn. Disord. 30, 432–439. doi: 10.1159/000321985
Khemka, V. K., Bagchi, D., Bandyopadhyay, K., Bir, A., Chattopadhyay, M., Biswas, A., et al. (2014). Altered serum levels of adipokines and insulin in probable Alzheimer’s disease. J. Alzheimers Dis. 41, 525–533. doi: 10.3233/JAD-140006
Kim, M. W., Abid, N. B., Jo, M. H., Jo, M. G., Yoon, G. H., and Kim, M. O. (2017). Suppression of adiponectin receptor 1 promotes memory dysfunction and Alzheimer’s disease-like pathologies. Sci. Rep. 7:12435. doi: 10.1038/s41598-017-12632-9
Kivimäki, M., Luukkonen, R., Batty, G. D., Ferrie, J. E., Pentti, J., Nyberg, S. T., et al. (2018). Body mass index and risk of dementia: analysis of individual-level data from 1. 3 million individuals. Alzheimers Dement. 14, 601–609. doi: 10.1016/j.jalz.2017.09.016
Lambert, M. P., Barlow, A. K., Chromy, B. A., Edwards, C., Freed, R., and Liosatos, M., et al. (1998). Diffusible, nonfibrillar ligands derived from Abeta1-42 are potent central nervous system neurotoxins. Proc. Natl. Acad. Sci. U. S. A. 95, 6448–6453. doi: 10.1073/pnas.95.11.6448
Li, S., Hong, S., Shepardson, N. E., Walsh, D. M., Shankar, G. M., and Selkoe, D. (2009). Soluble oligomers of amyloid β protein facilitate hippocampal long-term depression by disrupting neuronal glutamate uptake. Neuron 62, 788–801. doi: 10.1016/j.neuron.2009.05.012
Liu, A. K., Chang, R. C., Pearce, R. K., and S, G. (2015a). Nucleus basalis of meynert revisited: anatomy, history and differential involvement in Alzheimer’s and Parkinson’s disease. Acta Neuropathol. 129, 527–540. doi: 10.1007/s00401-015-1392-5
Liu, Y., Palanivel, R., Rai, E., Park, M., Gabor, T. V., Scheid, M. P., et al. (2015b). Adiponectin stimulates autophagy and reduces oxidative stress to enhance insulin sensitivity during high-fat diet feeding in mice. Diabetes 64, 36–48. doi: 10.2337/db14-0267
Lourenco, M. V., Clarke, J. R., Frozza, R. L., Bomfim, T. R., Forny-Germano, L., Batista, A. F., et al. (2013). TNF-α mediates PKR-dependent memory impairment and brain IRS-1 inhibition induced by Alzheimer’s β-amyloid oligomers in mice and monkeys. Cell Metab. 18, 831–843. doi: 10.1016/j.cmet.2013.11.002
Luppino, F. S., De Wit, L. M., Bouvy, P. F., Stijnen, T., Cuijpers, P., Penninx, B. W. J. H., et al. (2010). Overweight, obesity, and depression: a systematic review and meta-analysis of longitudinal studies. Arch. Gen. Psychiatry 67, 220–229. doi: 10.1001/archgenpsychiatry.2010.2
Ma, J. J., Shang, J., Wang, H., Sui, J. R., and Liu, K. D. J. (2016). Serum adiponectin levels are inversely correlated with leukemia: a meta-analysis. J. Cancer Res. Ther. 12, 897–902. doi: 10.4103/0973-1482.186695
Ma, L., Wang, R., Dong, W., and Zhao, Z. (2018). Caloric restriction can improve learning and memory in C57/BL mice probably via regulation of the AMPK signaling pathway. Exp. Gerontol. 102, 28–35. doi: 10.1016/j.exger.2017.11.013
Maddineni, S., Metzger, S., Ocón, O., Hendricks, G., and Ramachandran, R. (2005). Adiponectin gene is expressed in multiple tissues in the chicken: food deprivation influences adiponectin messenger ribonucleic acid expression. Endocrinology 146, 4250–4256. doi: 10.1210/en.2005-0254
Malinowski, J. M., and Bolesta, S. (2000). Rosiglitazone in the treatment of type 2 diabetes mellitus: a critical review. Clin. Ther. 22, 1151–1168; discussion 1149-1150. doi: 10.1016/S0149-2918(00)83060-X
Mamun, A., Uddin, M., Mathew, B., and Ashraf, G. (2020). Toxic tau: structural origins of tau aggregation in Alzheimer’s disease. Neural Regen. Res. 15, 1417–1420. doi: 10.4103/1673-5374.274329
Man, H. Y., Ju, W., and Ahmadian, G. W. Y. (2000). Intracellular trafficking of AMPA receptors in synaptic plasticity. Cell. Mol. Life Sci. 57, 1526–1534. doi: 10.1007/pl00000637
Matsunaga, E., Nambu, S., Oka, M., and Iriki, A. (2013). Differential cadherin expression in the developing postnatal telencephalon of a new world monkey. J. Comp. Neurol. 521, 4027–4060. doi: 10.1002/cne.23389
Matsuzawa, Y. (2005). Adiponectin: identification, physiology and clinical relevance in metabolic and vascular disease. Atheroscler. Suppl. 6, 7–14. doi: 10.1016/j.atherosclerosissup.2005.02.003
Medina-Urrutia, A., Posadas-Romero, C., Posadas-Sánchez, R., Jorge-Galarza, E., Villarreal-Molina, T., González-Salazar, M. D. C., et al. (2015). Role of adiponectin and free fatty acids on the association between abdominal visceral fat and insulin resistance. Cardiovasc. Diabetol. 14:20. doi: 10.1186/s12933-015-0184-5
Najem, D., Bamji-Mirza, M., Chang, N., Liu, Q. Y., and Zhang, W. (2014). Insulin resistance, neuroinflammation, and Alzheimer’s disease. Rev. Neurosci. 25, 509–525. doi: 10.1515/revneuro-2013-0050
Ng, R. C. L., and Chan, K. H. (2017). Potential neuroprotective effects of adiponectin in Alzheimer’s disease. Int. J. Mol. Sci. 18:592. doi: 10.3390/ijms18030592
Ng, R. C. L., Cheng, O. Y., Jian, M., Kwan, J. S. C., Ho, P. W. L., Cheng, K. K. Y., et al. (2016). Chronic adiponectin deficiency leads to Alzheimer’s disease-like cognitive impairments and pathologies through AMPK inactivation and cerebral insulin resistance in aged mice. Mol. Neurodegener. 11:71. doi: 10.1186/s13024-016-0136-x
Nicolas, S., Cazareth, J., Zarif, H., Guyon, A., Heurteaux, C., Chabry, J., et al. (2017). Globular adiponectin limits microglia pro-inflammatory phenotype through an AdipoR1/NF-κB signaling pathway. Front. Cell. Neurosci. 11:352. doi: 10.3389/fncel.2017.00352
Nicolas, S., Debayle, D., Béchade, C., Maroteaux, L., Gay, A. S., Bayer, P., et al. (2018). Adiporon, an adiponectin receptor agonist acts as an antidepressant and metabolic regulator in a mouse model of depression. Transl. Psychiatry 8:159. doi: 10.1038/s41398-018-0210-y
Nicolas, S., Veyssière, J., Gandin, C., Zsürger, N., Pietri, M., Heurteaux, C., et al. (2015). Neurogenesis-independent antidepressant-like effects of enriched environment is dependent on adiponectin. Psychoneuroendocrinology 57, 72–83. doi: 10.1016/j.psyneuen.2015.03.017
Nie, J. M., and Li, H. F. (2017). Metformin in combination with rosiglitazone contribute to the increased serum adiponectin levels in people with type 2 diabetes mellitus. Exp. Ther. Med. 14, 2521–2526. doi: 10.3892/etm.2017.4823
Nishimura, M., Izumiya, Y., Higuchi, A., Shibata, R., Qiu, J., Kudo, C., et al. (2008). Adiponectin prevents cerebral ischemic injury through endothelial nitric oxide synthase-dependent mechanisms. Circulation 117, 216–223. doi: 10.1161/CIRCULATIONAHA.107.725044
O’Brien, P. D., Hinder, L. M., Callaghan, B. C., and Feldman, E. L. (2017). Neurological consequences of obesity. Lancet Neurol. 16, 465–477. doi: 10.1016/S1474-4422(17)30084-4
Okada-Iwabu, M., Yamauchi, T., Iwabu, M., Honma, T., Hamagami, K. I., Matsuda, K., et al. (2013). A small-molecule AdipoR agonist for type 2 diabetes and short life in obesity. Nature 503, 493–499. doi: 10.1038/nature12656
Okamoto, Y., Kihara, S., Ouchi, N., Nishida, M., Arita, Y., Kumada, M., et al. (2002). Adiponectin reduces atherosclerosis in apolipoprotein E-deficient mice. Circulation 106, 2767–2770. doi: 10.1161/01.CIR.0000042707.50032.19
Pákáski, M., Fehér, Á., Juhász, A., Drótos, G., Fazekas, Ö. C., Kovács, J., et al. (2014). Serum adipokine levels modified by donepezil treatment in Alzheimer’s disease. J. Alzheimers Dis. 38, 371–377. doi: 10.3233/JAD-131139
Patterson, C., Feightner, J. W., Garcia, A., Hsiung, G. Y. R., MacKnight, C., and Sadovnick, A. D. (2008). Diagnosis and treatment of dementia: 1. Risk assessment and primary prevention of Alzheimer disease. CMAJ 178, 548–556. doi: 10.1503/cmaj.070796
Pecoraro, A., Nigro, E., Polito, R., Monaco, M. L., Scudiero, O., Mormile, I., et al. (2017). Total and high molecular weight adiponectin expression is decreased in patients with common variable immunodeficiency: correlation with Ig replacement therapy. Front. Immunol. 8:895. doi: 10.3389/fimmu.2017.00895
Pedditizi, E., Peters, R., and Beckett, N. (2016). The risk of overweight/obesity in mid-life and late life for the development of dementia: a systematic review and meta-analysis of longitudinal studies. Age Ageing 45, 14–21. doi: 10.1093/ageing/afv151
Perseghin, G., Petersen, K., and Shulman, G. I. (2003). Cellular mechanism of insulin resistance: potential links with inflammation. Int. J. Obes. 27, S6–S11. doi: 10.1038/sj.ijo.0802491
Polito, R., Nigro, E., Messina, A., Monaco, M. L., Monda, V., Scudiero, O., et al. (2018). Adiponectin and orexin-a as a potential immunity link between adipose tissue and central nervous system. Front. Physiol. 9:982. doi: 10.3389/fphys.2018.00982
Polito, R., Nigro, E., Pecoraro, A., Monaco, M. L., Perna, F., Sanduzzi, A., et al. (2019). Adiponectin receptors and pro-inflammatory cytokines are modulated in common variable immunodeficiency patients: correlation with Ig replacement therapy. Front. Immunol. 10:2812. doi: 10.3389/fimmu.2019.02812
Pousti, F., Ahmadi, R., Mirahmadi, F., Hosseinmardi, N., and Rohampour, K. (2018). Adiponectin modulates synaptic plasticity in hippocampal dentate gyrus. Neurosci. Lett. 662, 227–232. doi: 10.1016/j.neulet.2017.10.042
Psilopanagioti, A., Papadaki, H., Kranioti, E. F., Alexandrides, T. K., and Varakis, J. N. (2009). Expression of adiponectin and adiponectin receptors in human pituitary gland and brain. Neuroendocrinology 89, 38–47. doi: 10.1159/000151396
Qiu, G., Wan, R., Hu, J., Mattson, M. P., Spangler, E., Liu, S., et al. (2011). Adiponectin protects rat hippocampal neurons against excitotoxicity. Age 33, 155–165. doi: 10.1007/s11357-010-9173-5
Qizilbash, N., Gregson, J., Johnson, M. E., Pearce, N., Douglas, I., Wing, K., et al. (2015). BMI and risk of dementia in two million people over two decades: a retrospective cohort study. Lancet Diabetes Endocrinol. 3, 431–436. doi: 10.1016/S2213-8587(15)00033-9
Ranscht, B., and Dours-Zimmermann, M. T. (1991). T-cadherin, a novel cadherin cell adhesion molecule in the nervous system lacks the conserved cytoplasmic region. Neuron 7, 391–402. doi: 10.1016/0896-6273(91)90291-7
Rizzo, F. R., Musella, A., De Vito, F., Fresegna, D., Bullitta, S., Vanni, V., et al. (2018). Tumor necrosis factor and interleukin-1β modulate synaptic plasticity during Neuroinflammation. Neural Plast. 2018:8430123. doi: 10.1155/2018/8430123
Saltiel, A. R., and Olefsky, J. M. (1996). Thiazolidinediones in the treatment of insulin resistance and type II diabetes. Diabetes 45, 1661–1669. doi: 10.2337/diab.45.12.1661
Scherer, P. E., Williams, S., Fogliano, M., Baldini, G., and Lodish, H. F. (1995). A novel serum protein similar to C1q, produced exclusively in adipocytes. J. Biol. Chem. 270, 26746–26749. doi: 10.1074/jbc.270.45.26746
Seixas da Silva, G. S., Melo, H. M., Lourenco, M. V., Lyra e Silva, N. M., De Carvalho, M. B., Alves-Leon, S. V., et al. (2017). Amyloid-β oligomers transiently inhibit AMP-activated kinase and cause metabolic defects in hippocampal neurons. J. Biol. Chem. 292, 7395–7406. doi: 10.1074/jbc.M116.753525
Sekiyama, K., Waragai, M., Akatsu, H., Sugama, S., Takenouchi, T., and Takamatsu, Y., et al. (2014). Disease modifying effect of adiponectin in model of α-synucleinopathies. Ann. Clin. Transl. Neurol. 1, 479–489. doi: 10.1002/acn3.77
Shah, S. A., Yoon, G. H., Chung, S. S., Abid, M. N., Kim, T. H., Lee, H. Y., et al. (2017). Novel osmotin inhibits SREBP2 via the AdipoR1/AMPK/SIRT1 pathway to improve Alzheimer’s disease neuropathological deficits. Mol. Psychiatry 22, 407–416. doi: 10.1038/mp.2016.23
Shankar, G. M., Li, S., Mehta, T. H., Garcia-Munoz, A., Shepardson, N. E., Smith, I., et al. (2008). Amyloid-β protein dimers isolated directly from Alzheimer’s brains impair synaptic plasticity and memory. Nat. Med. 14, 837–842. doi: 10.1038/nm1782
Singh-Manoux, A., Dugravot, A., Shipley, M., Brunner, E. J., Elbaz, A., Sabia, S., et al. (2018). Obesity trajectories and risk of dementia: 28 years of follow-up in the Whitehall II study. Alzheimers Dement. 14, 178–186. doi: 10.1016/j.jalz.2017.06.2637
Skeberdis, V. A., Lan, J. Y., Zheng, X., Zukin, R. S., and Bennett, M. V. L. (2001). Insulin promotes rapid delivery of N-methyl-D-aspartate receptors to the cell surface by exocytosis. Proc. Natl. Acad. Sci. U. S. A. 98, 3561–3566. doi: 10.1073/pnas.051634698
Song, J., Choi, S. M., and Kim, B. C. (2017). Adiponectin regulates the polarization and function of microglia via PPAR-γ signaling under amyloid β toxicity. Front. Cell. Neurosci. 11:64. doi: 10.3389/fncel.2017.00064
Song, W., Guo, F., Zhong, H., Liu, L., Yang, R., Wang, Q., et al. (2015). Therapeutic window of globular adiponectin against cerebral ischemia in diabetic mice: the role of dynamic alteration of adiponectin/adiponectin receptor expression. Sci. Rep. 5:17310. doi: 10.1038/srep17310
Song, W., Huo, T., Guo, F., Wang, H., Wei, H., Yang, Q., et al. (2013). Globular adiponectin elicits neuroprotection by inhibiting NADPH oxidase-mediated oxidative damage in ischemic stroke. Neuroscience 248, 136–144. doi: 10.1016/j.neuroscience.2013.05.063
Spranger, J., Verma, S., Göhring, I., Bobbert, T., Seifert, J., Sindler, A. L., et al. (2006). Adiponectin does not cross the blood-brain barrier but modifies cytokine expression of brain endothelial cells. Diabetes 55, 141–147. doi: 10.2337/diabetes.55.01.06.db05-1077
Steen, E., Terry, B. M., Rivera, E. J., Cannon, J. L., Neely, T. R., Tavares, R., et al. (2005). Impaired insulin and insulin-like growth factor expression and signaling mechanisms in Alzheimer’s disease - is this type 3 diabetes? J. Alzheimers Dis. 7, 63–80. doi: 10.3233/JAD-2005-7107
Stefan, N., and Stumvoll, M. (2002). Adiponectin-its role in metabolism and beyond. Horm. Metab. Res. 34, 469–474. doi: 10.1055/s-2002-34785
Sun, F., Lei, Y., You, J., Li, C., Sun, L., Garza, J., et al. (2019). Adiponectin modulates ventral tegmental area dopamine neuron activity and anxiety-related behavior through AdipoR1. Mol. Psychiatry 24, 126–144. doi: 10.1038/s41380-018-0102-9
Takemura, Y., Ouchi, N., Shibata, R., Aprahamian, T., Kirber, M. T., Summer, R. S., et al. (2007). Adiponectin modulates inflammatory reactions via calreticulin receptor-dependent clearance of early apoptotic bodies. J. Clin. Invest. 117, 375–386. doi: 10.1172/JCI29709
Talbot, K., Wang, H. Y., Kazi, H., Han, L. Y., Bakshi, K. P., Stucky, A., et al. (2012). Demonstrated brain insulin resistance in Alzheimer’s disease patients is associated with IGF-1 resistance, IRS-1 dysregulation, and cognitive decline. J. Clin. Invest. 122, 1316–1338. doi: 10.1172/JCI59903
Teixeira, A. L., Diniz, B. S., Campos, A. C., Miranda, A. S., Rocha, N. P., Talib, L. L., et al. (2013). Decreased levels of circulating adiponectin in mild cognitive impairment and Alzheimer’s disease. NeuroMolecular Med. 15, 115–121. doi: 10.1007/s12017-012-8201-2
Terry, R. D., Masliah, E., Salmon, D. P., Butters, N., DeTeresa, R., Hill, R., et al. (1991). Physical basis of cognitive alterations in alzheimer’s disease: synapse loss is the major correlate of cognitive impairment. Ann. Neurol. 30, 572–580. doi: 10.1002/ana.410300410
Thundyil, J., Pavlovski, D., Sobey, C. G., and Arumugam, T. V. (2012). Adiponectin receptor signalling in the brain. Br. J. Pharmacol. 165, 313–327. doi: 10.1111/j.1476-5381.2011.01560.x
Thundyil, J., Tang, S. C., Okun, E., Shah, K., Karamyan, V. T., and Li, Y. I., et al. (2010). Evidence that adiponectin receptor 1 activation exacerbates ischemic neuronal death. Exp. Transl. Stroke Med. 2:15. doi: 10.1186/2040-7378-2-15
Tsuchida, A., Yamauchi, T., Takekawa, S., Hada, Y., Ito, Y., Maki, T., et al. (2005). Peroxisome proliferator-activated receptor (PPAR) α activation increases adiponectin receptors and reduces obesity-related inflammation in adipose tissue: comparison of activation of PPARα, PPARγ, and their combination. Diabetes 54, 3358–3370. doi: 10.2337/diabetes.54.12.3358
Uddin, M. S., and Kabir, M. T. (2019). “Oxidative stress in Alzheimer’s disease: molecular hallmarks of underlying vulnerability” in Biological, diagnostic and therapeutic advances in Alzheimer’s disease. eds. G. M. Ashraf and A. Athanasios (Singapore: Springer), 91–115.
Uddin, M. S., Kabir, M. T., Al, Mamun A., Barreto, G. E., Rashid, M., Perveen, A., et al. (2020b). Pharmacological approaches to mitigate neuroinflammation in Alzheimer’s disease. Int. Immunopharmacol. 84:106479. doi: 10.1016/j.intimp.2020.106479
Uddin, M. S., Kabir, M. T., Jeandet, P., Mathew, B., Ashraf, G. M., Perveen, A., et al. (2020a). Novel anti-Alzheimer’s therapeutic molecules targeting amyloid precursor protein processing. Oxidative Med. Cell. Longev. 2020, 1–19. doi: 10.1155/2020/7039138
Uddin, M. S., Rahman, M. M., Jakaria, M., Rahman, M. S., Hossain, M. S., Islam, A., et al. (2020c). Estrogen signaling in Alzheimer’s disease: molecular insights and therapeutic targets for Alzheimer’s dementia. Mol. Neurobiol. 57, 2654–2670. doi: 10.1007/s12035-020-01911-8
Uddin, M. S., Tewari, D., Sharma, G., Kabir, M. T., Barreto, G. E., Bin-Jumah, M. N., et al. (2020d). Molecular mechanisms of ER stress and UPR in the pathogenesis of Alzheimer’s disease. Mol. Neurobiol. 57, 2902–2919. doi: 10.1007/s12035-020-01929-y
Une, K., Takei, Y. A., Tomita, N., Asamura, T., Ohrui, T., Furukawa, K., et al. (2011). Adiponectin in plasma and cerebrospinal fluid in MCI and Alzheimer’s disease. Eur. J. Neurol. 18, 1006–1009. doi: 10.1111/j.1468-1331.2010.03194.x
van Himbergen, T. M., Beiser, A. S., Ai, M., Seshadri, S., Otokozawa, S., Au, R., et al. (2012). Biomarkers for insulin resistance and inflammation and the risk for all-cause dementia and Alzheimer disease: results from the Framingham heart study. Arch. Neurol. 69, 594–600. doi: 10.1001/archneurol.2011.670
Várhelyi, Z. P., Kálmán, J., Oláh, Z., Ivitz, E. V., Fodor, E. K., Sántha, M., et al. (2017). Adiponectin receptors are less sensitive to stress in a transgenic mouse model of Alzheimer’s disease. Front. Neurosci. 11:199. doi: 10.3389/fnins.2017.00199
Venkatesh, B., Hickman, I., Nisbet, J., Cohen, J., and Prins, J. (2009). Changes in serum adiponectin concentrations in critical illness: a preliminary investigation. Crit. Care 13:R105. doi: 10.1186/cc7941
Vieira, M. N. N., and Beckman, D. (2017). Training microglia to resist Alzheimer’s disease. J. Neurosci. 37, 477–479. doi: 10.1523/JNEUROSCI.3345-16.2017
Vieira, M. N. N., Lima-Filho, R. A. S., and De Felice, F. G. (2018). Connecting Alzheimer’s disease to diabetes: underlying mechanisms and potential therapeutic targets. Neuropharmacology 136, 160–171. doi: 10.1016/j.neuropharm.2017.11.014
Waki, H., Yamauchi, T., Kamon, J., Ito, Y., Uchida, S., Kita, S., et al. (2003). Impaired multimerization of human adiponectin mutants associated with diabetes. Molecular structure and multimer formation of adiponectin. J. Biol. Chem. 278, 40352–40363. doi: 10.1074/jbc.M300365200
Wan, Z., Mah, D., Simtchouk, S., Klegeris, A., and Little, J. P. (2014). Globular adiponectin induces a pro-inflammatory response in human astrocytic cells. Biochem. Biophys. Res. Commun. 446, 37–42. doi: 10.1016/j.bbrc.2014.02.077
Wang, H. W., Pasternak, J. F., Kuo, H., Ristic, H., Lambert, M. P., and Chromy, B., et al. (2002). Soluble oligomers of β amyloid (1-42) inhibit long-term potentiation but not long-term depression in rat dentate gyrus. Brain Res. 924, 133–140. doi: 10.1016/S0006-8993(01)03058-X
Wang, S., Zhou, Y., Bo, Y., Li, L., Yu, S., Chen, Y., et al. (2016). C1q/tumor necrosis factor-related protein-3 attenuates brain injury after intracerebral hemorrhage via AMPK-dependent pathway in rat. Front. Cell. Neurosci. 10:237. doi: 10.3389/fncel.2016.00237
Waragai, M., Adame, A., Trinh, I., Sekiyama, K., Takamatsu, Y., Une, K., et al. (2016). Possible involvement of adiponectin, the anti-diabetes molecule, in the pathogenesis of Alzheimer’s disease. J. Alzheimers Dis. 52, 1453–1459. doi: 10.3233/JAD-151116
Waragai, M., Ho, G., Takamatsu, Y., Sekiyama, K., Sugama, S., and Takenouchi, T., et al. (2017). Importance of adiponectin activity in the pathogenesis of Alzheimer’s disease. Ann. Clin. Transl. Neurol. 4, 591–600. doi: 10.1002/acn3.436
Wennberg, A. M. V., Gustafson, D., Hagen, C. E., Roberts, R. O., Knopman, D., Jack, C., et al. (2016). Serum Adiponectin levels, neuroimaging, and cognition in the Mayo Clinic study of aging. J. Alzheimers Dis. 53, 573–581. doi: 10.3233/JAD-151201
Whitehead, J. P., Richards, A. A., Hickman, I. J., Macdonald, G. A., and Prins, J. B. (2006). Adiponectin - a key adipokine in the metabolic syndrome. Diabetes Obes. Metab. 8, 264–280. doi: 10.1111/j.1463-1326.2005.00510.x
Whitmer, R. A., Gunderson, E. P., Barrett-Connor, E., Quesenberry, C. P., and Yaffe, K. (2005). Obesity in middle age and future risk of dementia: a 27 year longitudinal population based study. Br. Med. J. 330, 1360–1362. doi: 10.1136/bmj.38446.466238.E0
Wilkinson, M., Brown, R., Imran, S. A., and Ur, E. (2007). Adipokine gene expression in brain and pituitary gland. Neuroendocrinology 6, 7–14. doi: 10.1159/000108635
Xu, W. L., Atti, A. R., Gatz, M., Pedersen, N. L., and Johansson, B. F. L. (2011). Midlife overweight and obesity increase late-life dementia risk: a population-based twin study. Neurology 76, 1568–1574. doi: 10.1212/WNL.0b013e3182190d09
Xu, Z. P., Gan, G. S., Liu, Y. M., Xiao, J. S., Liu, H. X., Mei, B., et al. (2018). Adiponectin attenuates streptozotocin-induced tau hyperphosphorylation and cognitive deficits by rescuing PI3K/Akt/GSK-3β pathway. Neurochem. Res. 43, 316–323. doi: 10.1007/s11064-017-2426-2
Xu, H., Gelyana, E., Rajsombath, M., Yang, T., Li, S., and Selkoe, D. (2016). Environmental enrichment potently prevents microglia-mediated neuroinflammation by human amyloid β-protein oligomers. J. Neurosci. 36, 9041–9056. doi: 10.1523/JNEUROSCI.1023-16.2016
Yamauchi, T., Kamon, J., Ito, Y., Tsuchida, A., Yokomizo, T., Kita, S., et al. (2003). Cloning of adiponectin receptors that mediate antidiabetic metabolic effects. Nature 423, 762–769. doi: 10.1038/nature01705
Yamauchi, T., Kamon, J., Minokoshi, Y., Ito, Y., Waki, H., Uchida, S., et al. (2002). Adiponectin stimulates glucose utilization and fatty-acid oxidation by activating AMP-activated protein kinase. Nat. Med. 8, 1288–1295. doi: 10.1038/nm788
Yamauchi, T., Kamon, J., Waki, H., Terauchi, Y., Kubota, N., Hara, K., et al. (2001). The fat-derived hormone adiponectin reverses insulin resistance associated with both lipoatrophy and obesity. Nat. Med. 7, 941–946. doi: 10.1038/90984
Yang, B., Wang, S., Yu, S., Chen, Y., Li, L., Zhang, H., et al. (2017). C1q/tumor necrosis factor-related protein 3 inhibits oxidative stress during intracerebral hemorrhage via PKA signaling. Brain Res. 1657, 176–184. doi: 10.1016/j.brainres.2016.11.016
Yarchoan, M., and Arnold, S. E. (2014). Repurposing diabetes drugs for brain insulin resistance in Alzheimer disease. Diabetes 63, 2253–2261. doi: 10.2337/db14-0287
Yaua, S. Y., Lia, A., Hooc, R. L. C., Chingb, Y. P., Christied, B. R., Leea, T. M. C., et al. (2014). Physical exercise-induced hippocampal neurogenesis and antidepressant effects are mediated by the adipocyte hormone adiponectin. Proc. Natl. Acad. Sci. U. S. A. 111, 15810–15815. doi: 10.1073/pnas.1415219111
Yoon, G., Shah, S. A., Ali, T., and Kim, M. O. (2018). The Adiponectin homolog osmotin enhances neurite outgrowth and synaptic complexity via AdipoR1/NgR1 signaling in Alzheimer’s disease. Mol. Neurobiol. 55, 6673–6686. doi: 10.1007/s12035-017-0847-1
Yu, J. G., Javorschi, S., Hevener, A. L., Kruszynska, Y. T., Norman, R. A., and Sinha, M., et al. (2002). The effect of thiazolidinediones on plasma adiponectin levels in normal, obese, and type 2 diabetic subjects. Diabetes 51, 2968–2974. doi: 10.2337/diabetes.51.10.2968
Zhang, D., Guo, M., Zhang, W., and Lu, X. Y. (2011). Adiponectin stimulates proliferation of adult hippocampal neural stem/progenitor cells through activation of p38 mitogen-activated protein kinase (p38MAPK)/glycogen synthase kinase 3β (GSK-3β)/β-catenin signaling cascade. J. Biol. Chem. 286, 44913–44920. doi: 10.1074/jbc.M111.310052
Zhang, D., Wang, X., and Lu, X. Y. (2016). Adiponectin exerts neurotrophic effects on dendritic arborization, spinogenesis, and neurogenesis of the dentate gyrus of male mice. Endocrinology 157, 2853–2869. doi: 10.1210/en.2015-2078
Zhang, D., Wang, X., Wang, B., Garza, J. C., Fang, X., Wang, J., et al. (2017). Adiponectin regulates contextual fear extinction and intrinsic excitability of dentate gyrus granule neurons through AdipoR2 receptors. Mol. Psychiatry 22, 1044–1055. doi: 10.1038/mp.2016.58
Zhao, W. Q., Chen, H., Quon, M. J., and Alkon, D. L. (2004). Insulin and the insulin receptor in experimental models of learning and memory. Eur. J. Pharmacol. 490, 71–81. doi: 10.1016/j.ejphar.2004.02.045
Zhao, L., Chen, S., Sherchan, P., Ding, Y., Zhao, W., Guo, Z., et al. (2018). Recombinant CTRP9 administration attenuates neuroinflammation via activating adiponectin receptor 1 after intracerebral hemorrhage in mice. J. Neuroinflammation 15:215. doi: 10.1186/s12974-018-1256-8
Keywords: Alzheimer’s disease, adipokine, AdipoQ, adipocyte dysfunction, obesity
Citation: Uddin MS, Rahman MM, Sufian MA, Jeandet P, Ashraf GM, Bin-Jumah MN, Mousa SA, Abdel-Daim MM, Akhtar MF, Saleem A and Amran MS (2021) Exploring the New Horizon of AdipoQ in Obesity-Related Alzheimer’s Dementia. Front. Physiol. 11:567678. doi: 10.3389/fphys.2020.567678
Edited by:
Dmitri Samovski, Washington University School of Medicine in St. Louis, United StatesReviewed by:
Aurora Daniele, University of Campania Luigi Vanvitelli, ItalyDaniele Vergara, University of Salento, Italy
Naima Moustaid-Moussa, Texas Tech University, United States
Copyright © 2021 Uddin, Rahman, Sufian, Jeandet, Ashraf, Bin-Jumah, Mousa, Abdel-Daim, Akhtar, Saleem and Amran. This is an open-access article distributed under the terms of the Creative Commons Attribution License (CC BY). The use, distribution or reproduction in other forums is permitted, provided the original author(s) and the copyright owner(s) are credited and that the original publication in this journal is cited, in accordance with accepted academic practice. No use, distribution or reproduction is permitted which does not comply with these terms.
*Correspondence: Md. Sahab Uddin, bXN1LW5ldXJvcGhhcm1hQGhvdG1haWwuY29t, bXN1X25ldXJvcGhhcm1hQGhvdG1haWwuY29t, bXN1Lm5ldXJvcGhhcm1hQGdtYWlsLmNvbQ==, orcid.org/0000-0002-0805-7840