- 1State Key Laboratory for Biology of Plant Diseases and Insect Pests, Institute of Plant Protection, Chinese Academy of Agricultural Sciences, Beijing, China
- 2College of Plant Protection, Shenyang Agricultural University, Shenyang, China
- 3Key Laboratory of Biology and Genetic Improvement of Horticultural Crops, Beijing Vegetable Research Center, Ministry of Agriculture, Beijing, China
- 4Beijing Key Laboratory of Vegetable Germplasm Improvement, Beijing Academy of Agriculture and Forestry Sciences, Beijing, China
Sitobion avenae (Fabricius), Rhopalosiphum padi (Linnaeus), Schizaphis graminum (Rondani), and Metopolophium dirhodum (Walker) (Hemiptera: Aphididae) are important pests of wheat and other cereals worldwide. In this study, the susceptibilities of four wheat aphid species to seven insecticides were assessed. Furthermore, the activities of carboxylesterase (CarE), glutathione S-transferase (GSTs), and cytochrome P450 monooxygenase (P450s) were determined in imidacloprid treated and untreated aphids. The results showed that the susceptibilities of four wheat aphid species to tested insecticides are different and M. dirhodum has shown higher tolerance to most insecticides. Relatively higher CarE and GST activities were observed in M. dirhodum, and P450s activities increased significantly in response to imidacloprid treatment. Moreover, susceptibility to imidacloprid were increased by the oxidase inhibitor piperonyl butoxide in M. dirhodum (20-fold). The results we have obtained imply that P450s may play an important role in imidacloprid metabolic process in M. dirhodum. We suggest that a highly species-specific approach is essential for managing M. dirhodum.
Introduction
The English grain aphid Sitobion avenae, bird cherry-oat aphid Rhopalosiphum padi, greenbug aphid Schizaphis graminum, and Metopolophium dirhodum (Homoptera: Aphididae) are important pests of wheat and other cereals worldwide (Zhang et al., 2019). According to National Bureau of Statistic wheat was cultivated on approximately 25.0 million hectares of agricultural land, of which nearly15 million hectares are infested with cereal aphids, resulting in immeasurable yield losses annually in China (Yan et al., 2016; Gong et al., 2020). Sitobion avenae and R. padi are problematic pest aphid species for diverse wheat cultivars grown in various regions (Lu and Gao, 2009; Hu et al., 2016). Metopolophium dirhodum is the most abundant aphid species on cereals in the continental climate of central Europe (Honek et al., 2018). Wheat aphids cause damage by directly feeding on plants and by vectoring multiple plant pathogenic viruses (Lu et al., 2016). Additionally, these pests feed on wheat in a mixed-population with similar environmental conditions (Hu et al., 2015), but these wheat aphids have subtle different characteristics, such as host plant range and adaptability (Denno et al., 2000; Gianoli, 2000; Honek et al., 2006; Sun et al., 2009).
The management of wheat aphids relies primarily on the application of insecticides. The organophosphate (OP) insecticides parathion and disulfoton were commonly used for controlling S. graminum in the 1990s (Gao and Zhu, 2000). Pyrethroid insecticides were increasingly used for aphids’ management because of their efficient contact activity and environment friendly toxicity profile after many pests developed serious resistance to OP insecticides (Chen et al., 2017; Mackenzie et al., 2018). Neonicotinoids represent a fourth insecticide class after OPs, carbamates and pyrethroids. Imidacloprid, the first commercialized insecticide of this class with high effectiveness against several sucking pests (Tomizawa and Casida, 2003; Lu et al., 2016), and it has been used as a seed treatment for controlling grain aphids in recent years (Miao et al., 2014). However, concerning the environmental impacts and resistance, the application of botanical insecticides such as abamectin, matrine and rotenone has been augmented (Dias et al., 2019; Jesser et al., 2020). New types of insecticides provide more choices for pest management, but the effectiveness needs further studies.
While chemical control is an effective method for managing pests, the wide and intensive use of synthetic insecticides has resulted in resistance to many major insecticide classes in field populations of many pests, including aphids (Zhang et al., 2017). One of the major mechanisms involves the increased activities of esterase, glutathione S-transferase (GSTs), and cytochrome P450 monooxygenase (P450s) (Zhang et al., 2016b). Previous studies indicated that elevated carboxylesterase (CarE) levels contributed to the resistance to OP and carbamate insecticides in S. graminum (Ono et al., 1999) and R. padi (Chen et al., 2007). The increased production of these enzymes, especially P450s have been detected in vast insecticide-resistant pests, such as Musca domestica (Zhang et al., 2010; Feng et al., 2018), Nilaparvata lugens (Mao et al., 2019; Jin et al., 2019), Ceratitis capitata (Arouri et al., 2015), Myzus persicae (Panini et al., 2015), and Helicoverpa armigera (Wang et al., 2019). Moreover, enhanced P450s activities have contributed to neonicotinoid resistance were reported in N. lugens (Bass et al., 2011), Drosophila melanogaster (Harrop et al., 2018), and R. padi (Wang et al., 2018).
We found that the field occurrence of M. dirhodum is more serious than S. avenae and R. padi in the areas such as Beijing and Hebei province where with imidacloprid seed treatment, according to us investigate result in past several years (Li et al., 2019). An earlier investigation revealed that the tissue-specific constitutive overexpression of P450s confers tolerance to imidacloprid in Rhynchophorus ferrugineus (Antony et al., 2019). To uncover the potential mechanism of insensitivity of M. dirhodum to imidacloprid, the main detoxifying enzymes (CarE, GSTs and P450s) activities in four wheat aphids were analyzed, focusing on imidacloprid treated and untreated aphids. The effects of three synergists piperonyl butoxide (PBO), triphenyl phosphate (TPP), and diethyl maleate (DEM) on imidacloprid against four wheat aphids were also detected. Furthermore, the susceptibilities of four wheat aphids to the other six frequently used insecticides, including thiamethoxam, avermectin, beta-cypermethrin, omethoate, matrine, and rotenone belong to class of neonicotinoid, macrolide, pyrethroid, OP and botanical were determined, following an application of leaf dipping method.
Materials and Methods
Insect Populations
The population of Sitobion avenae, Rhopalosiphum padi, Schizaphis graminum, and Metopolophium dirhodum with no exposure to insecticides since collected from Langfang (Hebei, China) in 2013 (N39°30’29”, E 116°36’09”) and reared on wheat seedlings under standard conditions (20 ± 1°C and 60 ± 10% relative humidity with a 16-h light:8-h dark photoperiod).
Pesticides, Synergists, and Other Chemicals
The insecticides used for bioassays included imidacloprid (98% purity; Ningbo Sanjiang Yinong Chemical Co., Ltd., China), thiamethoxam (98% purity; Zhejiang Heben Technology Co., Ltd., China), beta-cypermethrin (97% purity; Jiangsu Yangnong Chemical Co., Ltd., China), omethoate (40% emulsifiable concentrate; Hebei Xinxing Chemical Co., Ltd., China), matrine (40% purity; Nantong Shenyu Green Pharmaceutical Co., Ltd., China), rotenone (40% purity; Inner Mongolia Qingyuanbao Biological Technology Co., Ltd., China), and avermectin (92% purity; Shijiazhuang Shuguang Pharmaceutical Raw Material Medicine Co., Ltd., China). Synergists piperonyl butoxide (PBO; reagent grade), triphenyl phosphate (TPP; reagent grade), and diethyl maleate (DEM; reagent grade) were purchased from Sigma-Aldrich Shanghai Trading Co., Ltd., United States.
Fast blue B salt, α-naphthyl acetate (α-NA), eserine, reduced glutathione (GSH), coomassie brilliant blue G250, 1-chloro-2,4-dinitrochlorobenzene (CDNB), phenylmethylsulfonyl fluoride (PMSF), n-phenylthiourea (PTU), albumin bovine (BSA), ethylenediaminetetraacetic acid (EDTA), DL-dithiothreitol (DTT) were purchased from Sigma-Aldrich Shanghai Trading Co., Ltd., United States.
Bioassay
A leaf-based insecticide bioassay method was performed as previously described (Zuo et al., 2016). Serial dilutions of the active ingredients from the tested insecticides were prepared using 0.1% Tween-80 in water. Wheat leaves containing animate apterous aphids were dipped in the insecticide dilutions for 3 s. Then, the leaves were removed from the solution, and residual droplets on the leaves were adsorbed with clean, dry filter paper. Three replicates of at least 30 aphids were used for each insecticide concentration, and 5 or 6 serial concentrations were used for each insecticide. Leaves dipped in 0.1% Tween-80 were used as a control. The aphids were maintained at 20 ± 1°C and 60 ± 10% relative humidity with a 16-h light/8-h dark photoperiod after treatment. Mortality was assessed after 24 h using a stereomicroscope and the mortality of control in every experiment request lower than 10%. Aphids were scored alive at least one leg can move after being touched with an anatomical needle. The limitation of this whole-body immersion method is the penetration of the compounds would be higher because acetone would help active ingredients to penetrate better in the cuticle.
Enzyme Assays
The activities of enzymes were measured as previously described (Lu et al., 2013) with some modifications. Batches of approximately 40 apterous adult aphids of each species were manually homogenized in 200 μL ice-cold 0.1 M sodium phosphate buffer (pH 7.6) containing 1 mM EDTA, 1 mM DTT, 1 mM PTU, 1 mM PMSF, and 20% glycerol. Homogenates were then centrifuged at 4°C, 12,000 g for 15 min in microcentrifuge (Thermo Fisher, Germany). The supernatant was collected for CarE, GSTs, and P450s activities analyzed. Three replicates were conducted for each aphid species.
Carboxylesterase activity was determined using the α-naphthyl acetate (α-NA) as described earlier with some modifications (Tang et al., 2017). The total reaction volume of 250 μL per well of a 96-well microplate contained 150 μL reaction mix (contained 107 mM α-NA, 107 mM eserine and 0.01 g mL–1 Fast blue B salt), 50 μL of PBS and 50 μL of the enzyme source. Absorbance was measured at 600 nm and 30°C using the kinetic model for 5 min continuously in a microplate reader (FlexStation 3, United States).
The activity of GSTs was determined according to a slightly modified published method involving CDNB (Habig et al., 1974). Briefly, a 300 μL reaction mixture comprising 100 μL CDNB (1.2 mM) substrate solution, 100 μL GSH (6 mM), and 100 μL diluted enzyme solution was prepared, after which the absorbance was measured at 340 nm using the kinetic model for 10 min.
The monooxygenase enzyme activity of four aphids were measured by an insect function oxidase ELISA kit (Huabaitai Biotechnology Corporation, Beijing, China) as previously described (Cui et al., 2018) with slight modifications. The enzyme sources were transferred to microlon ELISA plates according to the manufacturer’s instructions, after which the absorbance at 450 nm was measured and the concentrations were calculated based on a standard curve (y = 0.0043x + 0.0389, R2 = 0.9973), the results are expressed as IU/L.
The protein contents of the enzyme solutions were determined by the Bradford method. A serial albumin bovine (BSA) concentration solutions and samples were measured together and the protein content of samples were calculated by a standard curve based on BSA solutions. Three replicates of 10 μL diluted enzyme solutions were mixed with 200 μL protein assay dye reagent. After a 5 min incubation, the absorbance at 595 nm was measured.
Sample Collection for Inducible Enzyme Assays
Wheat seedlings (about 15 cm high) were immersed in imidacloprid solutions for 5 min, the concentrations were LC50 values that 62.21 mg L–1 for R. padi, 6.79 mg L–1 for S. avenae, 41.11 mg L–1 for S. graminum and 261.91 mg L–1 for M. dirhodum, respectively, then selected to petri dishes containing moistened filter paper after air-dried at room temperature for 30 min. The aphids still live after 24 h were collected for enzyme activities analysis, 120 aphids were collected for each aphid species.
Synergism Bioassays
The effects of three synergists PBO, TPP, and DEM on imidacloprid against four wheat aphids were tested. Stocks of insecticides with synergists were prepared and adjusted to final concentrations by serial dilution with distilled water containing 0.1% (v/v) Tween-80 for the bioassays. The highest concentration of PBO, DEF and DEM used were 625 mg/L and the mortality as low as control (<10%). The bioassay method was performed as described in section “Bioassay.”
Statistical Analysis
The mortality data were corrected based on the control mortality with Abbott’s formula. The LC50 values, 95% confidence intervals and slopes were calculated (Data Processing System software, v.7.05). Toxicity difference ratio (TDR) indicates the tolerance difference among pests. The TDR of tested insecticides to M. dirhodum, S. graminum, R. padi, and S. avenae were calculated based on the LC50 values. Differences in the enzyme activities among aphid species were analyzed using one-way ANOVA followed by Tukey’s multiple comparisons test and Student’s t-test was used to separate the means between control and imidacloprid treatments (p < 0.05) (GraphPad Prism, v.8.0.1).
Results
Bioassay
The result showed that four wheat aphids have different susceptibilities to the tested insecticides. All tested insecticides showed lowest toxicity to M. dirhodum and the toxicity difference ratio (TDR) were greater than 2.0, except matrine. Imidacloprid and avermectin showed highest toxicity to S. avenae, and the TDR were ranged from 0.10 to 4.02, and 0.39 to 1.15; thiamethoxam has highest toxicity to S. graminum, the TDR was ranged from 0.49 to 11.81; omethoate, beta-cypermethrin, matrine, and rotenone showed highest toxicity to R. padi. The toxicity of avermectin to four aphid species is roughly comparable with LC50 ranged from 3.92 to 11.48 mg L–1 (Table 1).
Enzyme Activity
To find out what caused the differential susceptibility to insecticide among the four wheat aphids, and clarify the reasons of high insecticide tolerance in M. dirhodum, especially to imidacloprid, we determined the CarE, GSTs, and P450s activities. The data showed that CarE actives in M. dirhodum and R. padi were significantly higher than that in S. graminum, S. avenae (Figure 1A). The highest GST activities were observed in M. dirhodum with significant difference compared to R. padi (2.51-fold) and S. graminum (2.47-fold) (Figure 1B). However, the P450s activity of M. dirhodum at the same level as R. padi and P450s activity in S. graminum was significantly lower than S. avenae (3.05-fold) (Figure 1C).
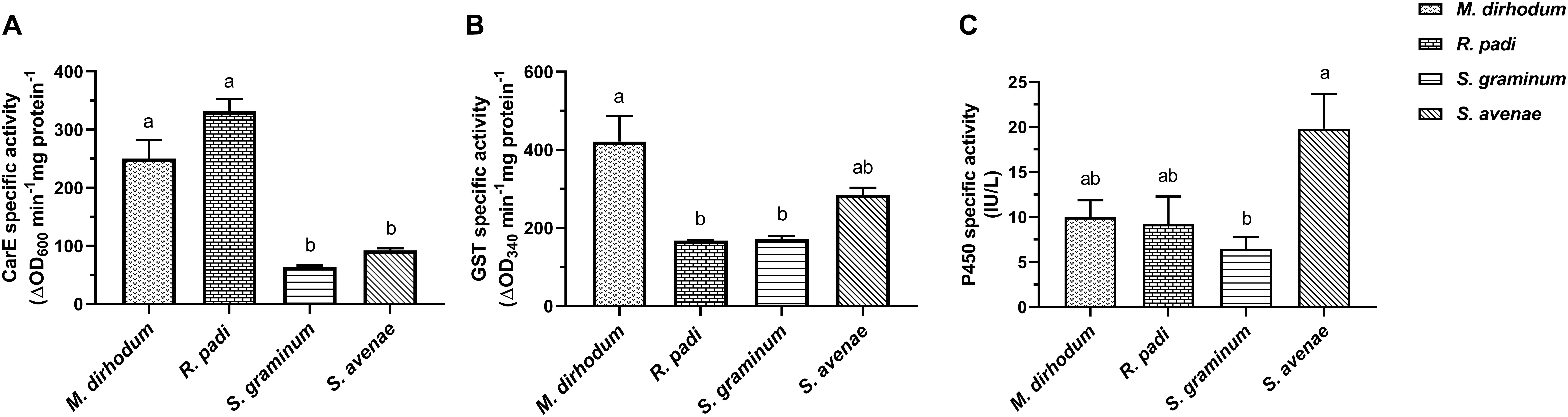
Figure 1. Specific activities of carboxylesterase (CarE, A), glutathione S-transferase (GSTs, B), and cytochrome P450 monooxygenase (P450s, C) in four aphid species in wheat. Data presented as the mean ± SEM (n = 3). Different letters on the bars indicate that the means are significantly different among the enzyme activities of aphid species using one-way ANOVA followed by Tukey’s multiple comparisons test (p < 0.05).
Inducible Enzyme Activity
Furthermore, we analyzed the detoxifying enzyme activities of four wheat aphids after imidacloprid treatment. The activities of P450s in M. dirhodum and S. graminum were significantly higher in imidacloprid-treated aphids than untreated by 5.37 and 5.39-fold (Figure 2C), respectively. However, the activity of P450s was significantly lower in the imidacloprid treated than control in S. avenae. The activities of CarE and GSTs were no difference between treated and untreated among four wheat aphids (Figures 2A,B).
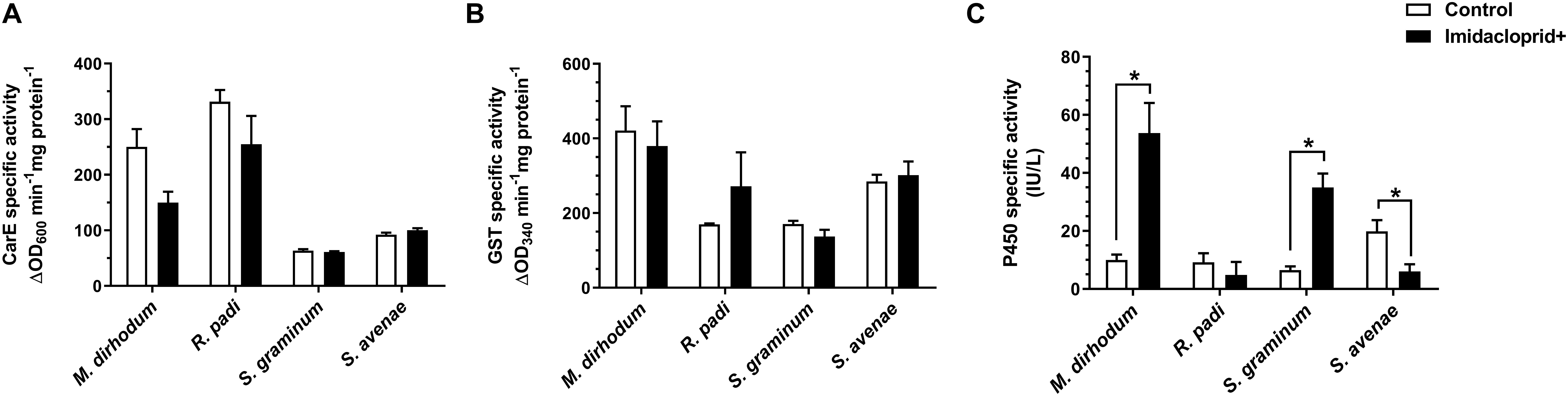
Figure 2. Effect of imidacloprid on detoxifying enzyme activities of carboxylesterase (CarE, A), glutathione S-transferase (GSTs, B), and cytochrome P450 monooxygenase (P450s, C) in four aphid species in wheat. Error bars represent the mean ± SEM (n = 3). Significant differences between imidacloprid treated and control samples were marked by asterisks: *significant at the 0.05 level.
Synergism Bioassay
The effects of PBO, TPP, and DEM on imidacloprid activities against four wheat aphids was presented in Table 2. The susceptibilities of M. dirhodum and S. graminum to imidacloprid were increased by the oxidase inhibitor PBO (20.06 and 2.50-fold), which consistent with the enzyme activities induced by imidacloprid. The susceptibility of R. padi to imidacloprid was increased by TPP (6.88-fold) and DEM (8.32-fold). In contrast, TPP, DEM, and PBO had inconsequential effects on imidacloprid against S. avenae.
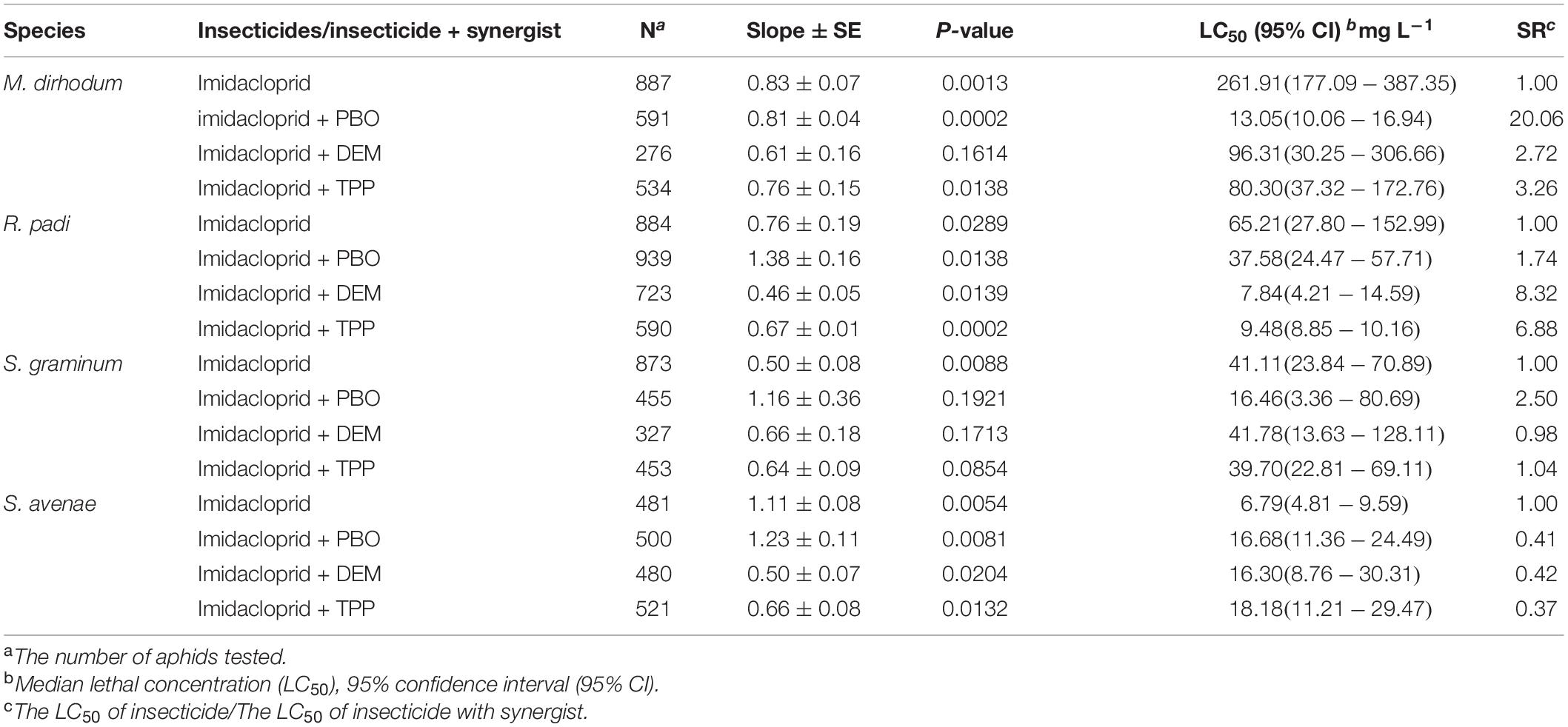
Table 2. Synergistic effects of PBO, DEM, and TPP on imidacloprid against four aphid species in wheat.
Discussion
Detecting the susceptibility of insecticide can help with the assessment when exponential growth is limited by insecticide-dependent factors (Mohammed et al., 2019). In this study, we assessed the tolerance of four wheat aphids to seven insecticides. The data revealed that most of the insecticides have much lower toxicity to M. dirhodum than other three wheat aphids. This means that M. dirhodum has the highest insecticide tolerance (especially for the neonicotinoids imidacloprid and thiamethoxam), whereas R. padi seems more susceptible to most of insecticides that we tested in this study. These results are consistent with the result that M. dirhodum has potential becoming a dominant aphid species in fields (Honek et al., 2006). Similarly, studies comparing the insecticide tolerances between S. avenae and R. padi also proved that most insecticides had lower toxicity to S. avenae (Lu and Gao, 2016).
Developing an appropriate pest control strategy should ideally consider the insecticide tolerance difference among pest species. Bemisia tabaci, an agriculturally important pest worldwide with B and Q two biotypes, different biological characteristics between biotypes B and Q, especially insecticide tolerance, affected the outcome of their competition (Yao et al., 2017). An earlier investigation confirmed that applications of either pyriproxyfen or neonicotinoids may select for biotype Q, enabling it to survive to a greater degree in areas where these insecticides are applied (Horowitz et al., 2005). Thus, B. tabaci biotype Q has supplanted biotype B as the major biotype in China, where it causes serious economic losses (Wang et al., 2010). The results of the current study suggest that M. dirhodum may replace R. padi and S. avenae become dominant species in wheat where imidacloprid and thiamethoxam used.
Insecticide-detoxifying enzymes are important for the metabolism of xenobiotics in insects. The resistance of insects to insecticides are relate to the increases of these detoxifying enzymes activities. Our study confirmed that four wheat aphids have different tolerance to insecticides. Metopolophium dirhodum with the highest tolerance to insecticide may relate to the activity of CarE significantly higher than that in S. graminum and S. avenae. Moreover, the highest activity of GSTs was also observed in M. dirhodum. Another study obtained similar result the authors examined the GSTs activities and molecular weights in M. dirhodum, S. avenae and R. padi, and determined that the highest GSTs active was observed in the extracts from M. dirhodum (Leszczynski et al., 1994). Previous research also indicated that the detoxification efficiency of GSTs is likely higher in S. avenae than R. padi (Lu and Gao, 2009), which is consistent with our results. Although R. padi seems more susceptible to most of insecticides, the activity of CarE at the same level as M. dirhodum, significantly higher than S. avenae and S. graminum.
The accumulation of P450 is the main mechanism underlying the imidacloprid resistance in N. lugens (Zhang et al., 2016a,c; Zimmer et al., 2018). But there was no significant difference between M. dirhodum and other three aphid species in P450 activities. We further determined the detoxifying enzyme activities of four wheat aphids with imidacloprid treated. The subsequent analysis showed greater P450 activities in M. dirhodum than untreated. Furthermore, the imidacloprid susceptibility was suppressed by PBO in M. dirhodum (20.06-fold). These results imply that the P450 activity induced by imidacloprid may be related to the insensitivity of M. dirhodum to imidacloprid. Expression induction of P450 genes by imidacloprid in N. lugens showed some P450 genes (CYP6CS1, CYP6CW1, and CYP6ER1) were up-regulated genes (Zhang et al., 2016a). CYP6 also have been reported to play important roles in imidacloprid resistance in Drosophila melanogaster (Daborn et al., 2001), Bemisia tabaci (Karunker et al., 2008), Myzus persicae (Puinean et al., 2010). The detoxifying enzyme activities in R. padi were not significantly affected by imidacloprid, but TPP significantly increased the imidacloprid effects (6.88-fold). Similarly, a previous study demonstrated that TPP suppressed imidacloprid activity by 2.45-fold in an imidacloprid-resistant R. padi strain (Wang et al., 2018) and another study have proved that GSTs can influences imidacloprid effect (Sillapawattana and Schaffer, 2017) suggesting that GSTs may related to susceptibility to imidacloprid in R. padi. Curiously, the activity of P450s in S. avenae with imidacloprid treated was significantly lower than control, both PBO and TPP show significant antagonism of imidacloprid in S. avenae which need further study, but PBO showed antagonism with some insecticides that have been reported (Ullah et al., 2017).
In conclusion, this study revealed the difference in susceptibilities of four aphid species in wheat to various insecticides. The insensitivity of M. dirhodum to imidacloprid may related to an inducible increase activity of P450.
Data Availability Statement
The raw data supporting the conclusions of this article will be made available by the authors, without undue reservation, to any qualified researcher.
Author Contributions
PG, DC, and XZ conceived and designed the research. PG, CW, and ML conducted the experiments. PG and XAL analyzed the data. PG and XZ wrote the manuscript. YZ and XRL revised the manuscript. All authors have read and approved the manuscript.
Funding
This study was supported by the National Key Research and Development Program of China (2017YFD0201700, 2016YFD0300700, 2018YFD0200500, and 31772165) and China Agriculture Research System (Award Number: CARS-3) National Natural Science Foundation (31772165).
Conflict of Interest
The authors declare that the research was conducted in the absence of any commercial or financial relationships that could be construed as a potential conflict of interest.
References
Antony, B., Johny, J., Abdelazim, M. M., Jakse, J., Al-Saleh, M. A., and Pain, A. (2019). Global transcriptome profiling and functional analysis reveal that tissue-specific constitutive overexpression of cytochrome P450s confers tolerance to imidacloprid in palm weevils in date palm fields. BMC Genomics 20:440. doi: 10.1186/s12864-019-5837-4
Arouri, R., Goff, G. L., Hemden, H., Navarro-Llopis, V., M’saad, M., Castañera, P., et al. (2015). Resistance to lambda-cyhalothrin in Spanish field populations of Ceratitis capitata and metabolic resistance mediated by P450 in a resistant strain. Pest Manage. Sci. 71, 1281–1291. doi: 10.1002/ps.3924
Bass, C., Carvalho, R. A., Oliphant, L., Puinean, A. M., Field, L. M., Nauen, R., et al. (2011). Overexpression of a cytochrome P450 monooxygenase, CYP6ER1, is associated with resistance to imidacloprid in the brown planthopper, Nilaparvata lugens. Insect Mol. Biol. 20, 763–773. doi: 10.1111/j.1365-2583.2011.01105.x
Chen, M., Han, Z., Qiao, X., and Qu, M. (2007). Mutations in acetylcholinesterase genes of Rhopalosiphum padi resistant to organophosphate and carbamate insecticides. Genome 50, 172–179. doi: 10.1139/g07-021
Chen, X., Tie, M., Chen, A., Ma, K., Li, F., Liang, P., et al. (2017). Pyrethroid resistance associated with M918L mutation and detoxifying metabolism in Aphis gossypii from Bt cotton growing regions of China. Pest Manag. Sci. 73, 2353–2359. doi: 10.1002/ps.4622
Cui, L., Wang, Q., Qi, H., Wang, Q., Yuan, H., and Rui, C. (2018). Resistance selection of indoxacarb in Helicoverpa armigera (Hubner) (Lepidoptera: Noctuidae): cross-resistance, biochemical mechanisms and associated fitness costs. Pest Manag. Sci. 74, 2636–2644. doi: 10.1002/ps.5056
Daborn, P., Boundy, S., Yen, J., Pittendrigh, B., and Ffrench-Constant, R. (2001). DDT resistance in Drosophila correlates with Cyp6g1 over-expression and confers cross-resistance to the neonicotinoid imidacloprid. Mol. Genet. Genomics 266, 556–563. doi: 10.1007/s004380100531
Denno, R. F., Peterson, M. A., Gratton, C., Cheng, J. A., Langellotto, G. A., Huberty, A. F., et al. (2000). Feeding-induced changes in plant quality mediate interspecific competition between sap-feeding herbivores. Ecology 81, 1814–1827. doi: 10.1890/0012-9658(2000)081[1814:ficipq]2.0.co;2
Dias, M. L., Auad, A. M., Magno, M. C., Resende, T. T., Fonseca, M. G., and Silva, S. E. B. (2019). Insecticidal activity of compounds of plant origin on Mahanarva spectabilis (Hemiptera: Cercopidae). Insects 10:360. doi: 10.3390/insects10100360
Feng, X. C., Li, M., and Liu, N. N. (2018). Carboxylesterase genes in pyrethroid resistant house flies, Musca domestica. Insect Biochem. Mol. Biol. 92, 30–39. doi: 10.1016/j.ibmb.2017.11.007
Gao, J. R., and Zhu, K. Y. (2000). Comparative toxicity of selected organophosphate insecticides against resistant and susceptible clones of the greenbug, Schizaphis graminum (Homoptera: aphididae). J. Agricult. Food Chem. 48, 4717–4722. doi: 10.1021/jf000548p
Gianoli, E. (2000). Competition in cereal aphids (Homoptera: Aphididae) on wheat plants. Environ. Entomol. 29, 213–219. doi: 10.1093/ee/29.2.213
Gong, P., Li, X., Gao, H., Wang, C., Li, M., Zhang, Y., et al. (2020). Field evolved resistance to pyrethroids, neonicotinoids, organophosphates and macrolides in Rhopalosiphum padi (Linnaeus) and Sitobion avenae (Fabricius) from China. Chemosphere 2020:128747. doi: 10.1016/j.chemosphere.2020.128747
Habig, W. H., Pabst, M. J., and Jakoby, W. B. (1974). Glutathione S-transferases. The first enzymatic step in mercapturic acid formation. J. Chem. Ecol. 249, 7130–7139.
Harrop, T. W., Denecke, S., Yang, Y. T., Chan, J., Daborn, P. J., Perry, T., et al. (2018). Evidence for activation of nitenpyram by a mitochondrial cytochrome P450 in Drosophila melanogaster. Pest Manage. Sci. 74, 1616–1622. doi: 10.1002/ps.4852
Honek, A., Jarosik, V., and Dixon, A. F. (2006). Comparing growth patterns among field populations of cereal aphids reveals factors limiting their maximum abundance. Bull. Entomol. Res. 96, 269–277. doi: 10.1079/ber2006427
Honek, A., Martinkova, Z., Saska, P., and Dixon, A. F. G. (2018). Aphids (Homoptera: Aphididae) on winter wheat: Predicting maximum abundance of Metopolophium dirhodum. J. Econom. Entomol. 111, 1751–1759. doi: 10.1093/jee/toy157
Horowitz, A. R., Kontsedalov, S., Khasdan, V., and Ishaaya, I. (2005). Biotypes B and Q of Bemisia tabaci and their relevance to neonicotinoid and pyriproxyfen resistance. Arch. Insect Biochem. Physiol. 58, 216–225. doi: 10.1002/arch.20044
Hu, X. S., Liu, X. F., Thieme, T., Zhang, G. S., Liu, T. X., and Zhao, H. Y. (2015). Testing the fecundity advantage hypothesis with Sitobion avenae, Rhopalosiphum padi, and Schizaphis graminum (Hemiptera: Aphididae) feeding on ten wheat accessions. Sci. Rep. 5:18549.
Hu, X. S., Liu, Y. J., Wang, Y. H., Wang, Z., Yu, X. L., Wang, B., et al. (2016). Resistance of wheat accessions to the English grain aphid Sitobion avenae. PLoS One 11:e0156158. doi: 10.1371/journal.pone.0156158
Jesser, E., Lorenzetti, A. S., Yeguerman, C., Murray, A. P., Domini, C., and Werdin-González, J. O. (2020). Ultrasound assisted formation of essential oil nanoemulsions: Emerging alternative for Culex pipiens pipiens Say (Diptera: Culicidae) and Plodia interpunctella Hübner (Lepidoptera: Pyralidae) management. Ultrason. Sonochem. 61:104832. doi: 10.1016/j.ultsonch.2019.104832
Jin, R. H., Mao, K. K., Liao, X., Xu, P. F., Li, Z., Ali, E., et al. (2019). Overexpression of CYP6ER1 associated with clothianidin resistance in Nilaparvata lugens (Stal). Pestic. Biochem. Physiol. 154, 39–45. doi: 10.1016/j.pestbp.2018.12.008
Karunker, I., Benting, J., Lueke, B., Ponge, T., Nauen, R., Roditakis, E., et al. (2008). Over-expression of cytochrome P450 CYP6CM1 is associated with high resistance to imidacloprid in the B and Q biotypes of Bemisia tabaci (Hemiptera: Aleyrodidae). Insect Biochem. Mol. Biol. 38, 634–644. doi: 10.1016/j.ibmb.2008.03.008
Leszczynski, B., Matok, M., and Dixon, A. F. (1994). Detoxification of cereal plant allelochemicals by aphids: Activity and molecular weights of glutathione S-transferase in three species of cereal aphids. J. Chem. Ecol. 20, 387–394. doi: 10.1007/bf02064445
Li, Y. P., Li, X. R., Zhang, Y. H., Zhu, X., and Cheng, D. F. (2019). Impact of seed coating with imidacloprid on laboratory populations of Metopolophium dirhodum (Walker) (Homoptera: Aphididae). Plant. Prot. 45, 25–29+36.
Lu, Y. H., and Gao, X. W. (2009). Multiple mechanisms responsible for differential susceptibilities of Sitobion avenae (Fabricius) and Rhopalosiphum padi (Linnaeus) to pirimicarb. Bull. Entomol. Res. 99, 611–617. doi: 10.1017/s0007485309006725
Lu, Y. H., and Gao, X. W. (2016). Inhibiting effects of common insecticides on carboxylesterase activity in Sitobion avenae and Rhopalosiphum padi (Hemiptera: Aphididae) and their synergism to beta-cypermethrin. Acta Entomol. Sinica 59, 1151–1158.
Lu, Y., He, Y., and Gao, X. (2013). Comparative studies on acetylcholinesterase characteristics between the aphids, Sitobion avenae and Rhopalosiphum padi. J. Insect Sci. 13:9.
Lu, Y., Zheng, X., and Gao, X. (2016). Sublethal effects of imidacloprid on the fecundity, longevity, and enzyme activity of Sitobion avenae (Fabricius) and Rhopalosiphum padi (Linnaeus). Bull. Entomol. Res. 106, 551–559. doi: 10.1017/s0007485316000286
Mackenzie, T. D. B., Arju, I., Poirier, R., and Singh, M. (2018). A genetic survey of pyrethroid insecticide resistance inaphids in New Brunswick, Canada, with particular emphasis on aphids as vectors of potato virus Y. J. Econom. Entomol. 111, 1361–1368. doi: 10.1093/jee/toy035
Mao, K. K., Zhang, X. L., Ali, E., Liao, X., Jin, R. H., Ren, Z. J., et al. (2019). Characterization of nitenpyram resistance in Nilaparvata lugens (Stal). Pestic. Biochem. Physiol. 157, 26–32. doi: 10.1016/j.pestbp.2019.03.001
Miao, J., Du, Z., Wu, Y., Gong, Z., Jiang, Y., Duan, Y., et al. (2014). Sub-lethal effects of four neonicotinoid seed treatments on the demography and feeding behaviour of the wheat aphid Sitobion avenae. Pest Manag. Sci. 70, 55–59. doi: 10.1002/ps.3523
Mohammed, A., Desneux, N., Monticelli, L. S., Fan, Y., Shi, X., Guedes, R. N. C., et al. (2019). Potential for insecticide-mediated shift in ecological dominance between two competing aphid species. Chemosphere 226, 651–658. doi: 10.1016/j.chemosphere.2019.03.114
Ono, M., Swanson, J. J., Field, L. M., Devonshire, A. L., and Siegfried, B. D. (1999). Amplification and methylation of an esterase gene associated with insecticide-resistance in greenbugs, Schizaphis graminum (Rondani) (Homoptera: Aphididae). Insect Biochem. Mol. Biol. 29, 1065–1073. doi: 10.1016/s0965-1748(99)00082-x
Panini, M., Anaclerio, M., Puggioni, V., Stagnati, L., Nauen, R., and Mazzoni, E. (2015). Presence and impact of allelic variations of two alternative s-kdr mutations, M918T and M918L, in the voltage-gated sodium channel of the green peach aphid Myzus persicae. Pest Manag. Sci. 71, 878–884. doi: 10.1002/ps.3927
Puinean, A. M., Foster, S. P., Oliphant, L., Denholm, I., Field, L. M., Millar, N. S., et al. (2010). Amplification of a cytochrome P450 gene is associated with resistance to neonicotinoid insecticides in the aphid Myzus persicae. PLoS Genet. 6:e1000999. doi: 10.1371/journal.pgen.1000999
Sillapawattana, P., and Schaffer, A. (2017). Effects of imidacloprid on detoxifying enzyme glutathione S-transferase on Folsomia candida (Collembola). Environ. Sci. Poll. Res. 24, 11111–11119. doi: 10.1007/s11356-016-6686-1
Sun, Y. C., Chen, F. J., and Ge, F. (2009). Elevated CO2 changes interspecific competition among three species of wheat aphids: Sitobion avenae, Rhopalosiphum padi, and Schizaphis graminum. Environ. Entomol. 38, 26–34. doi: 10.1603/022.038.0105
Tang, Q. L., Ma, K. S., Hou, Y. M., and Gao, X. W. (2017). Monitoring insecticide resistance and diagnostics of resistance mechanisms in the green peach aphid, Myzus persicae (Sulzer) (Hemiptera: Aphididae) in China. Pestic. Biochem. Physiol. 143, 39–47. doi: 10.1016/j.pestbp.2017.09.013
Tomizawa, M., and Casida, J. E. (2003). Selective toxicity of neonicotinoids attributable to specificity of insect and mammalian nicotinic receptors. Annu. Rev. Entomol. 48, 339–364.
Ullah, S., Ejaz, M., and Ali Shad, S. (2017). Study of Synergism, Antagonism, and Resistance Mechanisms in Insecticide-Resistant Oxycarenus hyalinipennis (Hemiptera: Lygaeidae). J. Econ. Entomol. 110, 615–623. doi: 10.1093/jee/tow302
Wang, K., Zhang, M., Huang, Y., Yang, Z., Su, S., and Chen, M. (2018). Characterisation of imidacloprid resistance in the bird cherry-oat aphid, Rhopalosiphum padi, a serious pest on wheat crops. Pest Manag. Sci. 74, 1457–1465. doi: 10.1002/ps.4834
Wang, Z. Y., Yan, H. F., Yang, Y. H., and Wu, Y. D. (2010). Biotype and insecticide resistance status of the whitefly Bemisia tabaci from China. Pest Manage. Sci. 66, 1360–1366. doi: 10.1002/ps.2023
Wang, Z., Jiang, S., Mota-Sanchez, D., Wang, W., Li, X. R., Gao, Y., et al. (2019). Cytochrome P450-mediated lambda-cyhalothrin-resistance in a field strain of Helicoverpa armigera from northeast China. J. Agric. Food Chem. 67, 3546–3553. doi: 10.1021/acs.jafc.8b07308
Yan, T., Chen, H., Sun, Y., Yu, X., and Xia, L. (2016). RNA Interference of the ecdysone receptor genes EcR and USP in grain aphid (Sitobion avenae F.) affects its survival and fecundity upon feeding on wheat plants. Int. J. Mol. Sci. 17:2098. doi: 10.3390/ijms17122098
Yao, F. L., Zheng, Y., Huang, X. Y., Ding, X. L., Zhao, J. W., Desneux, N., et al. (2017). Dynamics of Bemisia tabaci biotypes and insecticide resistance in Fujian province in China during 2005-2014. Sci. Rep. 7:40803.
Zhang, J. H., Zhang, Y. X., Wang, Y. C., Yang, Y. X., Cang, X. Z., and Liu, Z. W. (2016a). Expression induction of P450 genes by imidacloprid in Nilaparvata lugens: A genome-scale analysis. Pestic. Biochem. Physiol. 132, 59–64. doi: 10.1016/j.pestbp.2015.10.016
Zhang, L. P., Lu, H., Guo, K., Yao, S. M., and Cui, F. (2017). Insecticide resistance status and detoxification enzymes of wheat aphids Sitobion avenae and Rhopalosiphum padi. Sci. China Life Sci. 60, 1–4.
Zhang, L., Shi, J., Shi, X., Liang, P., Gao, J., and Gao, X. (2010). Quantitative and qualitative changes of the carboxylesterase associated with beta-cypermethrin resistance in the housefly, Musca domestica (Diptera: Muscidae). Comp. Biochem. Physiol. Part B Biochem. Mol. Biol. 156, 6–11. doi: 10.1016/j.cbpb.2010.01.011
Zhang, X. L., Liao, X., Mao, K. K., Zhang, K. X., Wan, H., and Li, J. H. (2016b). Insecticide resistance monitoring and correlation analysis of insecticides in field populations of the brown planthopper Nilaparvata lugens (stål) in China 2012–2014. Pestic. Biochem. Physiol. 132, 13–20. doi: 10.1016/j.pestbp.2015.10.003
Zhang, Y. X., Yang, Y. X., Sun, H. H., and Liu, Z. W. (2016c). Metabolic imidacloprid resistance in the brown planthopper, Nilaparvata lugens, relies on multiple P450 enzymes. Insect Biochem. Mol. Biol. 79, 50–56. doi: 10.1016/j.ibmb.2016.10.009
Zhang, Y., Fan, J., Fu, Y., Francis, F., and Chen, J. L. (2019). Plant-mediated interactions between two cereal aphid species: Promotion of aphid performance and attraction of more parasitoids by infestation of wheat with phytotoxic aphid Schizaphis graminum. J. Agricult. Food Chem. 67, 2763–2773. doi: 10.1021/acs.jafc.8b06150
Zimmer, C. T., Garrood, W. T., Singh, K. S., Randall, E., Lueke, B., Gutbrod, O., et al. (2018). Neofunctionalization of duplicated P450 genes drives the evolution of insecticide resistance in the brown planthopper. Curr. Biol. 28, 268.e–274.e.
Keywords: imidacloprid, cytochrome P450 monooxygenase, synergist, toxicity difference, wheat aphids
Citation: Gong P, Chen D, Wang C, Li M, Li X, Zhang Y, Li X and Zhu X (2021) Susceptibility of Four Species of Aphids in Wheat to Seven Insecticides and Its Relationship to Detoxifying Enzymes. Front. Physiol. 11:623612. doi: 10.3389/fphys.2020.623612
Received: 03 November 2020; Accepted: 23 December 2020;
Published: 18 January 2021.
Edited by:
Peng He, Guizhou University, ChinaReviewed by:
Zuqing Hu, Northwest A&F University, ChinaHu Wan, Huazhong Agricultural University, China
Copyright © 2021 Gong, Chen, Wang, Li, Li, Zhang, Li and Zhu. This is an open-access article distributed under the terms of the Creative Commons Attribution License (CC BY). The use, distribution or reproduction in other forums is permitted, provided the original author(s) and the copyright owner(s) are credited and that the original publication in this journal is cited, in accordance with accepted academic practice. No use, distribution or reproduction is permitted which does not comply with these terms.
*Correspondence: Xun Zhu, emh1eHVuQGNhYXMuY24=
†These authors have contributed equally to this work