- Department of Physiology and Biochemistry, University School of Physical Education in Wrocław, Wrocław, Poland
The study was conducted to compare peak oxygen uptake (VO2peak) measured with the incremental graded test (GXT) (VO2peak) and two tests to verify maximum oxygen uptake, performed 15 min after the incremental test (VO2peak1) and on a separate day (VO2peak2). The aim was to determine which of the verification tests is more accurate and, more generally, to validate the VO2max obtained in the incremental graded test on cycle ergometer. The study involved 23 participants with varying levels of physical activity. Analysis of variance showed no statistically significant differences for repeated measurements (F = 2.28, p = 0.118, η2 = 0.12). Bland–Altman analysis revealed a small bias of the VO2peak1 results compared to the VO2peak (0.4 ml⋅min–1⋅kg–1) and VO2peak2 results compared to the VO2peak (−0.76 ml⋅min–1⋅kg–1). In isolated cases, it was observed that VO2peak1 and VO2peak2 differed by more than 5% from VO2peak. Considering the above, it can be stated that among young people, there are no statistically significant differences between the values of VO2peak measured in the following tests. However, in individual cases, the need to verify the maximum oxygen uptake is stated, but performing a second verification test on a separate day has no additional benefit.
Introduction
Maximum oxygen uptake (VO2max) is considered to be the gold standard in assessing oxygen capacity, as it reflects the efficiency of the respiratory and circulatory system and the efficiency of the muscular system in using oxygen whilst exercising (Bassett and Howley, 2000; Lucia et al., 2001; Martino et al., 2002; Joyner and Coyle, 2008). The incremental graded test (GXT) protocol is commonly used to assess the VO2max, which involves increasing the external load and continuing it until the subject reaches volitional exhaustion (Beltz et al., 2016). For years, the paradigm of the GXT was accepted and this form of VO2max testing was used. However, for several years, there has been a discussion of whether the GXT in each case allows for an accurate measurement of maximum oxygen uptake (Howley et al., 1995; Poole et al., 2008; Sánchez-Otero et al., 2014; Schaun, 2017). It was pointed out that subjects with no experience for maximal efforts and those with low motivation and low cardiorespiratory fitness may interrupt the test before reaching VO2max due to fatigue-related symptoms (Midgley et al., 2007b; Poole and Jones, 2017).
Therefore, new criteria for the accuracy of VO2max measurements have been proposed (Howley et al., 1995; Sánchez-Otero et al., 2014; Beltz et al., 2016; Schaun, 2017). It has been suggested that achieving a VO2 plateau in the final phase of the GXT is proof that a VO2max measurement is accurate (Howley et al., 1995). However, it has been documented that in many subjects (both athletes and non-athletes), it is impossible to separate the plateau phase when reaching VO2max (Lucia et al., 2006; Schaun, 2017; Hebisz et al., 2018). The other criteria for accurately measuring VO2max–analysis of peak respiratory quotient, peak heart rate (HR), and post-workout lactate concentration–have also been widely discussed (Howley et al., 1995; Duncan et al., 1997; Beltz et al., 2016). Nonetheless, their high inter-subject variability may suggest that some subjects do not satisfy mentioned criterions even if their maximum effort is made, which lowers their value. It has been also demonstrated that the criterion of achieving a VO2 plateau in the final phase of the GXT frequently does not meet the criteria for HR and lactate concentration (Poole et al., 2008). These limitations reduce the certainty that subjects performing the GXT reach their “true” VO2max.
Considering the doubts about the effectiveness of the above-mentioned criteria in verifying the accuracy of VO2max measurements, constant power verification tests were proposed (Midgley et al., 2006; Beltz et al., 2016; Poole and Jones, 2017; Schaun, 2017; Possamai et al., 2020). The idea is simply to provoke the VO2 plateau through constant-load effort performed with intensities ranging from submaximal to supramaximal effort (Barker et al., 2011; Nolan et al., 2014; Poole and Jones, 2017; Astorino and DeRevere, 2018). Usually, the verification tests are performed approximately 5–15 min after the incremental test (Schaun, 2017) and last several minutes (Barker et al., 2011; Nolan et al., 2014; Beltz et al., 2016; Schaun, 2017; Astorino and DeRevere, 2018).
On the other hand, Possamai et al. (2020) suggests that the test to verify the VO2max obtained in the GXT should be performed on a different day, assuming that the subject’s exercise tolerance/capacity is higher then and that the peak oxygen uptake (VO2peak) measured in a verification test on another day are not lower than that from a verification test performed several minutes after the GXT. However, in both verification tests they used a power output level of 100% of maximal power–as measured in a previous incremental test–which could have contributed to similar values of oxygen uptake being recorded in the tests. Moreover, their results showed that the VO2peak achieved in the verification test performed on a separate day were closer to the VO2peak of the GXT than that of a verification test done several minutes after the GXT.
More recently, in order to verify the VO2peak from the GXT, researchers proposed performing the verification test with a power level exceeding the power output of the GXT, but mainly several minutes after the GXT (Barker et al., 2011; Nolan et al., 2014; Schaun, 2017; Astorino and DeRevere, 2018). It seems that it would be worth using a higher load in the verification test performed on a separate day, as exercise tolerance is higher then.
The aim of this study was to compare the values of VO2peak obtained from the incremental test and from two verification tests completed with a power output of 110% of the peak power output reached in a previous incremental test [the first one was performed 15 min after the progressive test (Tver–1), whilst the second one was performed on a separate day (Tver–2)]. It was hypothesized that in individual cases, the verification test performed on a separate day may allow for higher VO2peak values than the incremental test and the verification test performed several minutes after the incremental test.
Materials and Methods
The study involved 23 participants: recreationally active individuals (n = 13, including 7 women and 6 men) and athletes (cyclists) (n = 10, including 4 women and 6 men). Each participant had been active recreationally or practicing sport (cyclists) for at least 3 years. The two groups, the recreationally active people and the athletes, were similar in regard to their anthropometric characteristics, whereas the parameters for physical capacity–VO2peak (p < 0.000) and power value (Pmax) (p < 0.000) differed significantly (Table 1).
The study design was approved by the institutional review board and was conducted in accordance with the ethical standards established by the Declaration of Helsinki. Written informed consent was obtained from all participants after the study details, procedures, benefits, and risks were explained.
Exercise Tests
The study consisted of three exercise tests (Figure 1). On the first day of the study, each participant performed an incremental graded test (GXT) and a verification test (Tver–1). After a 48-h break, an additional verification test (Tver–2) was performed, which was only preceded by a warm-up. The tests (GXT and Tver–1) and Tver–2 were performed at a similar time of day (±30 min). All the tests were carried out using a Lode Excalibur Sport electronically braked cycloergometer (Lode BV, Groningen, Netherlands). The tests were performed in controlled laboratory conditions at an exercise laboratory (PN-EN ISO 9001:2001 certified). One week prior to the incremental graded test, the participants were familiarized with the protocol of the test.
Incremental Exercise Test With Verification Test Performed on the Same Day
The VO2peak was determined using a continuous GXT, with a self-selected pedal rate no lower than 60 rev/min. The test started with a 40-W or 50-W load (for women and men, respectively), and it was increased by 40 W or 50 W (for women and men, respectively) every 3 min until volitional exhaustion. Heart rate was recorded with a V800 cardiofrequencimeter (Polar, Oy, Finland). The respiratory parameters were measured breath-by-breath (Quark, COSMED, Milan, Italy) and averaged over 30-s intervals. The data recording began 2 min before GXT and ended 5 min after the verification test (Tver–1). The device was calibrated with an atmospheric air and gas mixture: 5% CO2, 16% O2, and 79% N2. Oxygen uptake (VO2), exhaled carbon dioxide (VCO2), and minute pulmonary ventilation (VE) were measured. The highest VO2 recorded in the GXT was taken as the VO2peak, whilst the highest VO2 recorded in the Tver–1 was taken as the VO2peak1.
Based on the respiratory data records from the GXT, the first ventilatory threshold (VT1) was determined at the point preceding the first non-linear increase in VE⋅VO2–1 without a concomitant increase in VE⋅VCO2–1 equivalent; the second ventilatory threshold (VT2) was at the point preceding the second non-linear increase in VE⋅VO2–1 accompanied by an increase of VE⋅VCO2–1 equivalent, according to the methodology described by Davis et al. (1980) and Beaver et al. (1986).
The cycloergometer was controlled by a computer, which recorded instantaneous power and exercise time. The maximum aerobic Pmax was obtained by subtracting 0.22 W for women and 0.28 W for men for each missing second of the last performed load. After the end of the test, the subject rested for 15 min, with an active rest on a 20-W cycloergometer. Next, a 3-min, square-wave Tver–1 was performed with an intensity of 110% of Pmax with regards to Schaun (2017).
Verification Test Performed on a Different Day
The test was preceded by a 15-min warm-up consisting of 5 min of exercise at an intensity corresponding to the power achieved with the VT1, then 10 min at a power corresponding to half the distance between the VT1 and the VT2. The warm-up was followed by a 10-min passive break. Tver–2 was 3 min long and was performed at an intensity of 110% of Pmax, as determined by the results of the incremental graded test performed 2 days prior. The recording of respiratory parameters started 1 min before the verification test and ended 5 min after it was completed. The values averaged every 30 s were used in data analysis. The highest recorded oxygen uptake (from the averaging of 30-s intervals) was taken as the VO2peak in the verification test performed on a separate day (VO2peak2).
Statistical Analysis
The differences (expressed in %) between VO2peak and VO2peak1, as well as between VO2peak were calculated for each participant. The tolerance of measurement error was at 5% (Midgley et al., 2007a; Romero-Fallas et al., 2012; Hall-Lopez et al., 2015). Data normality was assessed through the Kolmogorov–Smirnov test with Lilliefors significance correction. Bland–Altman analysis was performed to determine the size of the difference shift between VO2peak and VO2peak1, as well as between VO2peak and VO2peak2. Pearson’s correlation and linear regression were performed for comparing the results of GXT and Tver–1 or Tver–2. STATISTICA 13.1 software (StatSoft Inc., Tulsa, OK, United States) was used for further statistical processing of the data. All data are reported as mean ± SD. Analysis of variance with repeated measurements and the Scheffe post hoc test were used to determine whether factors such as sex, athletic ability, or subsequent tests affected VO2peak. The results were considered statistically significant at an alpha level of p < 0.05.
Results
The GXT and Tver–2 were performed by 23 participants, while Tver–1 was performed by 21 participants (2 participants refused to perform this test because of perceived fatigue).
The analysis of the main effects showed statistically significant differences in oxygen uptake for sex (F = 25.02; p = 0.000; η2 = 0.60) and physical activity level (F = 74.24; p = 0.000; η2 = 0.81). There were no statistically significant differences for repeated measurements (F = 2.28, p = 0.118, η2 = 0.12) or mixed effects for repeated measurements and sex (F = 0.68, p = 0.516, η2 = 0.04), nor for mixed effects for repeated measurements and physical activity level (F = 0.20, p = 0.820, η2 = 0.01) (Table 2).
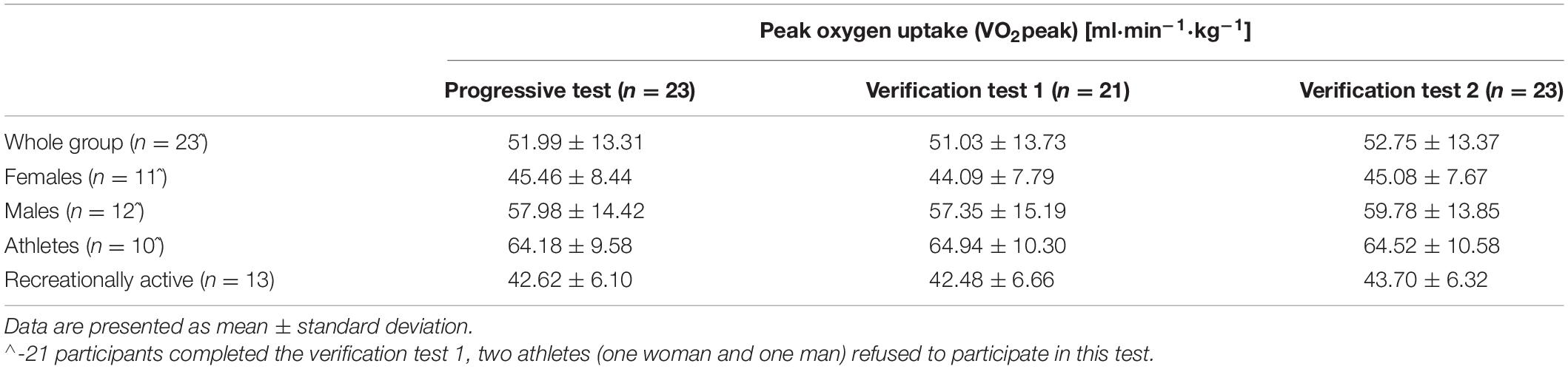
Table 2. Peak oxygen uptake value in the incremental test and in the verification tests in the entire group of subjects, as well as after dividing the group according to sex and physical activity level.
The individual analysis showed that 2 subjects in the Tver–1 and 7 subjects in the Tver–2 had a higher VO2peak by 5% than in the GXT (Table 3). Bland–Altman analysis (Figure 2) revealed a small bias of the VO2peak1 results compared to the VO2peak (0.4 ml⋅min–1⋅kg–1) and VO2peak2 results compared to the VO2peak (−0.76 ml⋅min–1⋅kg–1).
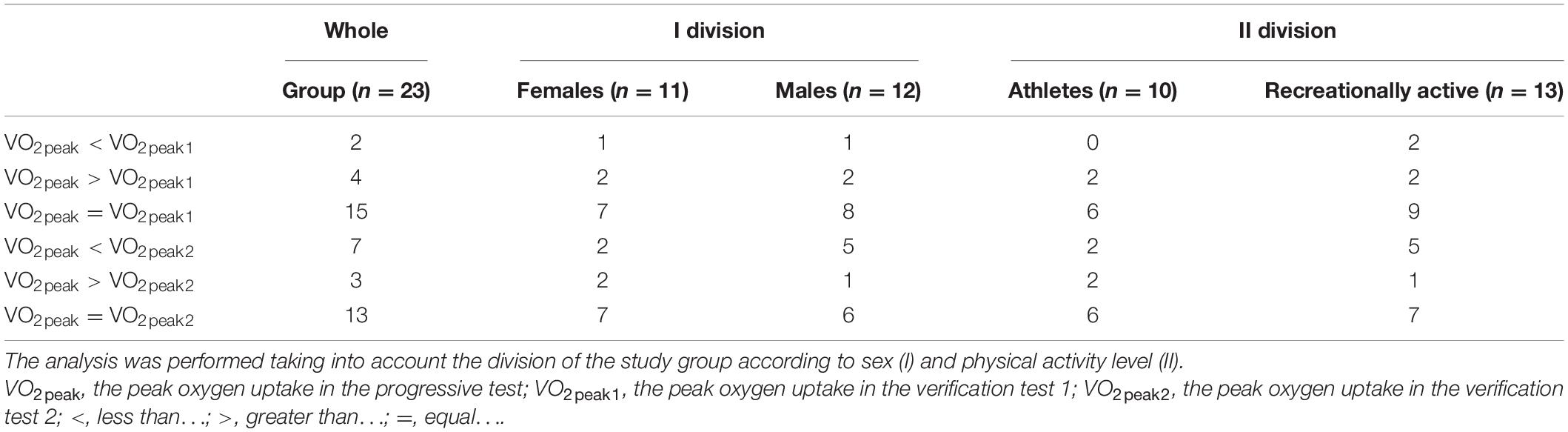
Table 3. The number of people who achieved a lower, higher or equal peak oxygen uptake in the verification tests compared to the peak oxygen uptake achieved in the progressive test.
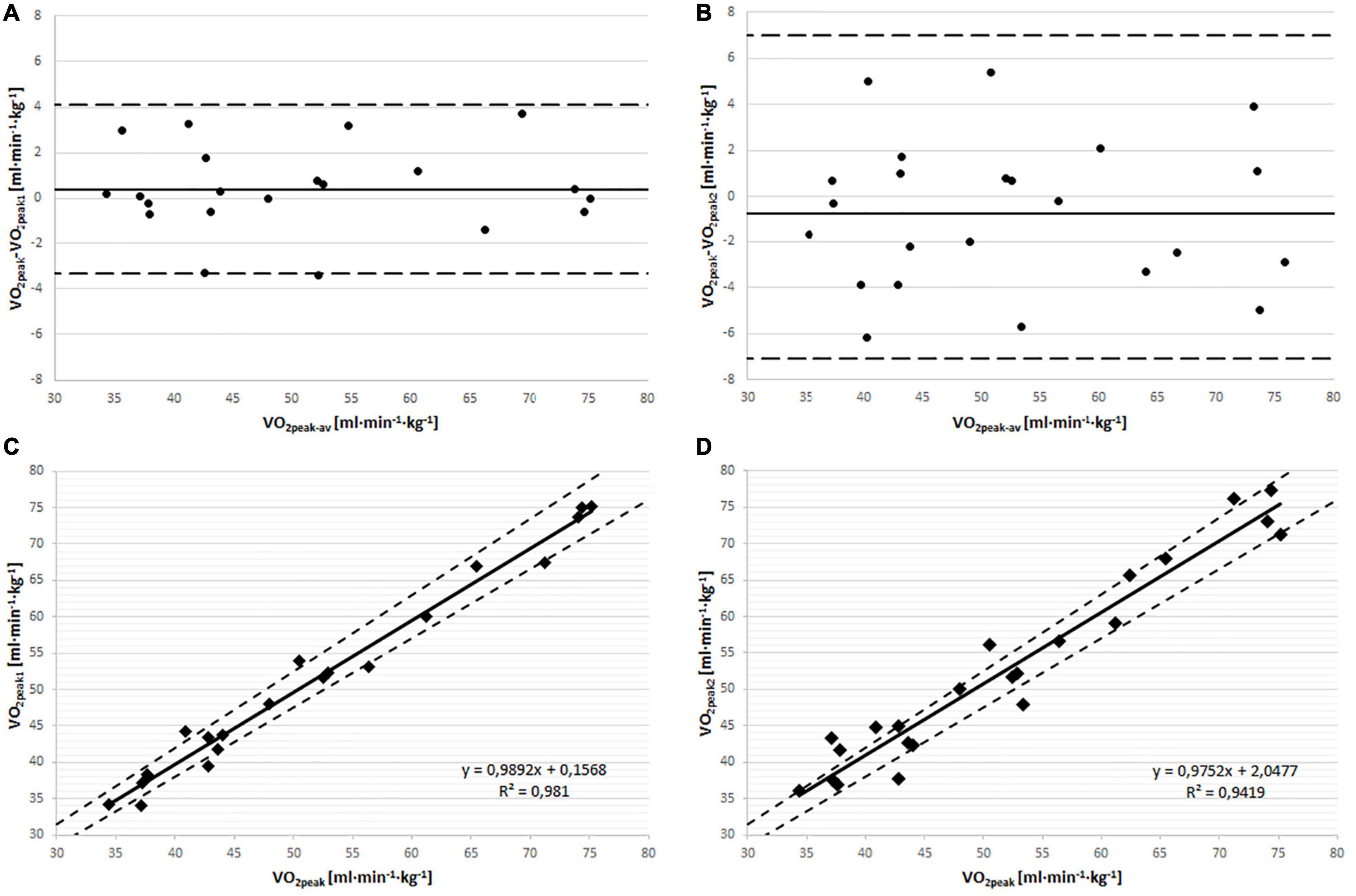
Figure 2. Bland-Altman plot showing: (A) Individual differences between the VO2peak values attained in the incremental and VO2peak1 from Tver–1 (B) individual differences between the VO2peak values attained in the incremental and VO2peak2 from Tver–2. Solid line show bias and dashed lines represent a 1.96 SD (standard deviation) for difference between peak oxygen uptakes. (C) Pearson correlation between VO2peak and VO2peak1. (D) Pearson correlation between VO2peak and VO2peak2. In (C,D) the dashed lines indicate the 5% threshold difference from VO2peak.
The raw test records that were performed in the studies described in this work are posted in the repository at https://repod.icm.edu.pl/dataset.xhtml?persistentId=doi:10.18150/HGE2PK.
Discussion
In order to assess the VO2peak, researchers traditionally use the GXT test until exhaustion. Since the primary criterion of VO2peak attainment–a VO2 plateau in exhaustion–is not always reached during the GXT, some researchers have postulated using subsequent verification tests (Niemelä et al., 1980; Midgley et al., 2007b; Poole and Jones, 2017). However, in the available literature, there are contradictory suggestions as to the need for verification tests. There are opinions that question the validity of performing tests to verify the VO2max obtained from a progressive test, due to the minimal individual differences between the results of progressive and verifying tests (Rossiter et al., 2006; Murias et al., 2018; Brito et al., 2019). Similar results, confirmed by Bland–Altman analysis, were presented by McGawley (2017) when he compared the VO2peak measured in the progressive test with the VO2peak measured in a 4-min time trial run, performed on a separate day. The data presented herein show no differences in mean VO2peak in the GXT and Tver–1 versus Tver–2 (Table 2). Bland–Altman analysis showed a small bias of VO2peak1 compared to VO2peak, as well as of VO2peak2 compared to VO2peak (Figure 2). However, several subjects (both recreationally active people and athletes) achieved higher VO2peak1 or VO2peak2 values than VO2peak. Therefore, we support the postulate of Poole and Jones (2017) about the need to perform tests verifying the values of VO2peak measured in progressive tests.
In most available literature, VO2max verifier tests are performed on the same day as the progressive test (Midgley et al., 2007b; Astorino, 2009; Kirkeberg et al., 2011; Dalleck et al., 2012; Poole and Jones, 2017; Adam et al., 2018). The factor differentiating used procedures is the time between the tests. Intervals of between 5 and 15 min have commonly been used (Midgley et al., 2007b; Poole and Jones, 2017; Adam et al., 2018), although intervals ranging from 1 to 3 min (Kirkeberg et al., 2011) to even 60–90 min (Astorino, 2009; Dalleck et al., 2012; Nolan et al., 2014) have been used for verification tests performed on the same day. Nolan et al. (2014) reported no differences in VO2peak between verification tests performed with 105% Pmax after 20- and 60-min recovery periods. Thus, 20 min of recovery may be sufficient for physically active subjects. As noted by Scharhag-Rosenberger et al. (2011), comparable VO2peak values after an incremental test and verification test followed by a 10-min break indicates that even shorter breaks can be used. The results reported by Kirkeberg et al. (2011) show that even short recovery periods of 1–3 min turned out to be sufficient among physically active people. Regardless of the intervals used between the tests, it seems that the effectiveness of the VO2max verification tests we quote above was similar. Therefore, it could be concluded that VO2peak in a verification test seems not to be affected by the exhaustion caused by the incremental test. Schaun (2017) also stated that the time elapsed between an incremental test and a verification test is not a key aspect to achieving the verification criterion. Attempts were also made to perform tests to verify VO2max on a different day than the progressive test (Scharhag-Rosenberger et al., 2011; Possamai et al., 2020; Sawyer et al., 2020). Possamai et al. (2020) found that during the verification test performed on a separate day, the exercise capacity is greater than during the verification test performed several minutes after the progressive test. Such a conclusion was formulated on the basis of a longer effort time in a verification test performed on a separate day, compared to a test performed several minutes after the progressive test. However, the greater exercise capacity described by Possamai et al. (2020) did not affect the VO2peak values, which were similar in individual tests. Scharhag-Rosenberger et al. (2011) also performed verification tests on a separate day. Based on the results of these studies, it was also considered that VO2peak in the verification test performed on a separate day does not differ significantly from VO2peak from the verification test performed several minutes after the progressive test. However, in the studies described above, verification tests were preceded by a short warm-up.
Another factor that may influence VO2peak values is the type of warm-up used before the verification test carried out on a separate day. Possamai et al. (2020) preceded the verification test with a warm-up of 6 min and measured the power at the lactate threshold, defined as the first sharp increase in lactate concentration in a progressive test. An even shorter warm-up, lasting 5 min, was used by Scharhag-Rosenberger et al. (2011) and Sawyer et al. (2020). In Scharhag-Rosenberger et al. (2011) study the warm-up was done at a speed higher than the lactate threshold speed. Also, a warm-up in the research of Sawyer et al. (2020) consisted of 5 min of exercise, however, at an intensity of 50 W (men) or 30 W (women) which is lower than those proposed by Scharhag-Rosenberger et al. (2011). Bishop (2003) stated that the optimal warm-up duration before intensive efforts with an average duration should be at least 10 min, which allows the subject to reach steady-state VO2. In our own studies, the warm-up lasted 15 min, including 5 min of VT1 effort and 10 min of effort measured halfway between VT1 and VT2. We concluded that such a warm-up, performed before the verification test on a separate day, may allow to obtain higher VO2peak values than in the above-cited works (Scharhag-Rosenberger et al., 2011; Possamai et al., 2020; Sawyer et al., 2020). This assumption was supported by the results of our own previous studies (Hebisz et al., 2017), in which we also used a long warm-up time. We then found that it is possible to achieve a higher VO2peak value even during a series of four short sprints (30-s each) in comparison to the progressive test. However, analysis of variance showed no statistically significant differences between VO2peak, VO2peak1 and VO2peak2 in the entire group of subjects. Moreover, Bland-Altman analysis revealed a bias of VO2peak1 compared to VO2peak, as well as of VO2peak2 compared to VO2peak was neglectable. Therefore, the research procedure we used produced similar statistical effects as the research results described by Scharhag-Rosenberger et al. (2011).
The possibility that the training level meets the VO2peak verification criterion was also analyzed in this study. The above-cited studies (Scharhag-Rosenberger et al., 2011; Nolan et al., 2014; Possamai et al., 2020) involved physically active people, but they were not professional athletes. Only in a review, Costa et al. (2021) stated that concordance between VO2peak level from GXT and verification tests is not affected by the cardiorespiratory level of participants. In the present study, we compared athletes with recreationally active subjects. Analysis of variance showed no mixed effects on repeated measurements and level of physical activity. Therefore, the results of the studies described in this work support Costa et al.’s (2021) suggestion that the effects of VO2max verification are not related to the level of efficiency (cardio-respiratory level).
Limitations
In our research, we compared VO2peak values achieved by cyclists and amateurs. In this way, our research complements the knowledge about the effects of verification tests, because so far there has been little information in the literature about the results of verification tests performed by athletes. On the other hand, performing analyses on a group of respondents consisting of cyclists and amateurs is a factor limiting the certainty of our conclusions, because athletes and amateurs are characterized by a different level of physical performance (muscular power, VO2peak, VO2max). Different levels of exercise tolerance in our studies may affect the high variability of the obtained results and thus may affect the results of statistical analyses.
The second factor limiting the certainty of our conclusions is the way the subjects are prepared for the verification test performed on a separate day. After warming up, and before the verification test, we used a passive break of 15 min. We decided that this way of preparing for the test is good, because in the literature there are suggestions that the type of break (active or passive) before a few minutes and intense efforts does not affect exercise capacity (McAinch et al., 2004; Fennell and Hopker, 2021). In addition, vasodilation of muscle vessels and the activity of histamine H1 and H2 receptors is high even for 90 min after exercise (Luttrell and Halliwill, 2017). However, the use of a passive break before the verification test performed on a separate day may have resulted in high variability of VO2peak–VO2peak2.
Conclusion
Among young people, there were no statistically significant differences between VO2peak measured in the progressive test and VO2peak measured in the verification tests (performed 15 min after the progressive test and performed on a separate day), in general. There are also no differences in peak oxygen consumption between the progressive test and the verification tests after dividing the group into athletes and recreationally active individuals in any of the above-mentioned groups. In individual cases, the need to verify the maximum oxygen uptake is stated, but performing a second verification test on a separate day does not bring additional benefits.
Data Availability Statement
The datasets presented in this study can be found in online repositories. The names of the repository/repositories and accession number(s) can be found below: https://repod.icm.edu.pl/dataset.xhtml?persistentId=doi:10.18150/HGE2PK.
Ethics Statement
The studies involving human participants were reviewed and approved by the Senate Research Ethics Committee at University School of Physical Education in Wrocław. The patients/participants provided their written informed consent to participate in this study.
Author Contributions
PH contributed to the study design and data collection, and drafted the manuscript. AJ contributed to the data collection and made the critical revisions to the manuscript. RH contributed to the study design and data analysis, and drafted the manuscript. All authors discussed the results, commented and edited the manuscript at all stages, approved the final version and agreed to be accountable for all aspects of the work.
Funding
This work was supported by the University School of Physical Education in Wrocław under grant number PN/BK/2020/07.
Conflict of Interest
The authors declare that the research was conducted in the absence of any commercial or financial relationships that could be construed as a potential conflict of interest.
Publisher’s Note
All claims expressed in this article are solely those of the authors and do not necessarily represent those of their affiliated organizations, or those of the publisher, the editors and the reviewers. Any product that may be evaluated in this article, or claim that may be made by its manufacturer, is not guaranteed or endorsed by the publisher.
References
Adam, J., Causer, A. J., Shute, J. K., Cummings, M. H., Shepherd, A. I., Bright, V., et al. (2018). Cardiopulmonary exercise testing with supramaximal verification produces a safe and valid assessment of VO2max in people with cystic fibrosis: a retrospective analysis. J. Appl. Physiol. 125, 1277–1283. doi: 10.1152/japplphysiol.00454.2018
Astorino, T. A. (2009). Alterations in VO2max and the VO2 plateau with manipulation of sampling interval. Clin. Physiol. Funct. Imaging. 29, 60–7. doi: 10.1111/j.1475-097X.2008.00835.x
Astorino, T. A., and DeRevere, J. (2018). Efficacy of constant load verification testing to confirm VO2 max attainment. Clin. Physiol. Funct. Imaging 38, 703–709. doi: 10.1111/cpf.12474
Barker, A. R., Williams, C. A., Jones, A. M., and Armstrong, N. (2011). Establishing maximal oxygen uptake in young people during a ramp cycle test to exhaustion. Br. J. Sports Med. 45, 498–503. doi: 10.1136/bjsm.2009.063180
Bassett, D. R., and Howley, E. T. (2000). Limiting factors for maximum oxygen uptake and determinants of endurance performance. Med. Sci. Sports Exerc. 32, 70–84.
Beaver, W. L., Wasserman, K., and Whipp, B. J. (1986). A new method for detecting anaerobic threshold by gas exchange. J. Appl. Physiol. 60, 2020–2027. doi: 10.1152/jappl.1986.60.6.2020
Beltz, N. M., Gibson, A. L., Janot, J. M., Kravitz, L., Mermier, C. M., and Dalleck, L. C. (2016). Graded exercise testing protocols for the determination of VO2max: historical perspectives, progress, and future considerations. J. Sports Med. 2016:3968393. doi: 10.1155/2016/3968393
Bishop, D. (2003). Warm up II: performance changes following active warm up and how to structure the warm up. Sports Med. 33, 483–498. doi: 10.2165/00007256-200333070-00002
Brito, J., Fernandes, L., Rebelo, A., Krustrup, P., Silva, G., and Oliveira, J. (2019). Heart rate kinetics response of pre-pubertal children during the Yo-Yo intermittent endurance test—level 1. Sports 7:65. doi: 10.3390/sports7030065
Costa, V. A. B., Midgley, A. W., Carroll, S., Astorino, T. A., de Paula, T., Farinatti, P., et al. (2021). Is a verification phase useful for confirming maximal oxygen uptake in apparently healthy adults? A systematic review and meta-analysis. PLoS One 16:e0247057. doi: 10.1371/journal.pone.0247057
Dalleck, L. C., Astorino, T. A., Erickson, R. M., McCarthy, C. M., Beadell, A. A., and Botten, B. H. (2012). Suitability of verification testing to confirm attainment of VO2max in middle-aged and older adults. Res. Sports Med. 20, 118–128. doi: 10.1080/15438627.2012.660825
Davis, J. A., Whipp, B. J., and Wasserman, K. (1980). The relation of ventilation to metabolic rate during moderate exercise in man. Eur. J. Appl. Physiol. Occup. Physiol. 44, 97–108. doi: 10.1007/BF00421087
Duncan, G. E., Howley, E. T., and Johnson, B. N. (1997). Applicability of VO2max criteria: discontinuous versus continuous protocols. Med. Sci. Sports Exerc. 29, 273–278. doi: 10.1097/00005768-199702000-00017
Fennell, C. R. J., and Hopker, J. G. (2021). The acute physiological and perceptual effects of recovery interval intensity during cycling-based high-intensity interval training. Eur. J. Appl. Physiol. 121, 425–434. doi: 10.1007/s00421-020-04535-x
Hall-Lopez, J. A., Ochoa-Martinez, P. Y., Moncada-Jimenez, J., Mendez, M. A. O., Garcia, I. M., and Garcia, M. A. M. (2015). Reliability of the maximal oxygen uptake following two consecutive trials by indirect calorimetry. Nutr. Hosp. 31, 1726–1732. doi: 10.3305/nh.2015.31.4.8487
Hebisz, P., Hebisz, R., Borkowski, J., and Zatoń, M. (2018). Time of VO2max plateau and postexercise oxygen consumption during incremental exercise testing in young mountain bike and road cyclists. Physiol. Res. 67, 711–719.
Hebisz, R., Hebisz, P., Zatoń, M., and Michalik, K. (2017). Peak oxygen uptake in a sprint interval testing protocol vs. maximal oxygen uptake in an incremental testing protocol and their relationship with cross-country mountain biking performance. Appl. Physiol. Nutr. Metab. 42, 371–376. doi: 10.1139/apnm-2016-0362
Howley, E. T., Bassett, D. R., and Welch, H. G. (1995). Criteria for maximal oxygen uptake: review and commentary. Med. Sci. Sports Exerc. 27, 1292–1301.
Joyner, M. J., and Coyle, E. F. (2008). Endurance exercise performance: the physiology of champions. J. Physiol. 586, 35–44.
Kirkeberg, J. M., Dalleck, L. C., Kamphoff, C. S., and Pettitt, R. W. (2011). Validity of 3 protocols for verifying VO2max. Int. J. Sports Med. 32, 266–270. doi: 10.1055/s-0030-1269914
Lucia, A., Hoyos, J., and Chicharro, J. L. (2001). Physiology of professional road cycling. Sports Med. 31, 325–337.
Lucia, A., Rabadan, M., Hoyos, J., Hernandez-Capilla, M., Perez, M., San Juan, A. F., et al. (2006). Frequency of the VO2max plateau phenomenon in world-class cyclists. Int. J. Sports Med. 27, 984–992. doi: 10.1055/s-2006-923833
Luttrell, M. J., and Halliwill, J. R. (2017). The intriguing role of histamine in exercise responses. Exerc. Sport. Sci. Rev. 45, 16–23. doi: 10.1249/JES.0000000000000093
Martino, M., Gledhill, N., and Jamnik, V. (2002). High VO2max with no history of training is primarily due to high blood volume. Med. Sci. Sports Exerc. 34, 966–971.
McAinch, A. J., Febbraio, M. A., Parkin, J. M., Zhao, S., Tangalakis, K., Stojanovska, L., et al. (2004). Effect of active versus passive recovery on metabolism and performance during subsequent exercise. Int. J. Sport Nutr. Exerc. Metab. 14, 185–196. doi: 10.1123/ijsnem.14.2.185
McGawley, K. (2017). The reliability and validity of a four-minute running time-trial in assessing VO2max and performance. Front. Physiol. 8:270. doi: 10.3389/fphys.2017.00270
Midgley, A. W., McNaughton, L. R., and Carroll, S. (2006). Verification phase as a useful tool in the determination of the maximal oxygen uptake of distance runners. Appl. Physiol. Nutr. Metab. 31, 541–548.
Midgley, A. W., McNaughton, L. R., and Carroll, S. (2007a). Effect of VO2 time-averaging interval on reproducibility of VO2max in healthy athletic subjects. Clin. Physiol. Funct. Imaging 27, 122–125. doi: 10.1111/j.1475-097X.2007.00725.x
Midgley, A. W., McNaughton, L. R., Polman, R., and Marchant, D. (2007b). Criteria for determination of maximal oxygen uptake: a brief critique and recommendations for future research. Sports Med. 37, 1019–1028. doi: 10.2165/00007256-200737120-00002
Murias, J. M., Pogliaghi, S., and Paterson, D. H. (2018). Measurement of a true VO2max during a ramp incremental test is not confirmed by a verification phase. Front. Physiol. 9:143. doi: 10.3389/fphys.2018.00143
Niemelä, K., Palatsi, I., Linnaluoto, M., and Takkunen, J. (1980). Criteria for maximum oxygen uptake in progressive bicycle tests. Eur. J. Appl. Physiol. Occup. Physiol. 44, 51–59. doi: 10.1007/BF00421763
Nolan, P. B., Beaven, M. L., and Dalleck, L. (2014). Comparison of intensities and rest periods for VO2max verification testing procedures. Int. J. Sports Med. 35, 1024–1029. doi: 10.1055/s-0034-1367065
Poole, D. C., and Jones, A. M. (2017). Measurement of the maximum oxygen uptake V̇O2max: V̇O2peak is no longer acceptable. J. Appl. Physiol. 122, 997–1002. doi: 10.1152/japplphysiol.01063.2016
Poole, D. C., Wilkerson, D. P., and Jones, A. M. (2008). Validity of criteria for establishing maximal O2 uptake during ramp exercise test. Eur. J. Appl. Physiol. 102, 403–410. doi: 10.1007/s00421-007-0596-3
Possamai, L. T., Campos, F. S., Salvador, P. C., de Aguiar, R. A., Guglielmo, L. G., de Lucas, R. D., et al. (2020). Similar maximal oxygen uptake assessment from a step cycling incremental test and verification tests on the same or different day. Appl. Physiol. Nutr. Metab. 45, 357–361. doi: 10.1139/apnm-2019-0405
Romero-Fallas, O., Soto-Arias, M., and Moncada-Jimenze, J. (2012). Maximal oxygen consumption in college students is reliable following four consecutive trials. J. Phys. Edu. Sport 12, 476–481. doi: 10.7522/jpes.2012.04069
Rossiter, H. B., Kowalchuk, J. M., and Whipp, B. J. (2006). A test to establish maximum O2 uptake despite no plateau in the O2 uptake response to ramp incremental exercise. J. Appl. Physiol. 100, 764–770. doi: 10.1152/japplphysiol.00932.2005
Sánchez-Otero, T., Iglesias-Soler, E., Boullosa, D. A., and Tuimil, J. L. (2014). Verification criteria for the determination of VO2max in the field. J. Strength Cond. Res. 28:35443551. doi: 10.1519/JSC.0000000000000576
Sawyer, B. J., McMahon, N., Thornhill, K. L., Baughman, B. R., Mahoney, J. M., Pattison, K. L., et al. (2020). Supra-versus submaximal cycle ergometer verification of VO2max in males and females. Sports 8:163. doi: 10.3390/sports8120163
Scharhag-Rosenberger, F., Carlsohn, A., Cassel, M., Mayer, F., and Scharhag, J. (2011). How to test maximal oxygen uptake: a study on timing and testing procedure of a supramaximal verification test. Appl. Physiol. Nutr. Metab. 36, 153–160. doi: 10.1139/H10-099
Keywords: maximum oxygen uptake, VO2 plateau, physical fitness, cycle ergometer, verification phase, incremental test
Citation: Hebisz P, Jastrzębska AD and Hebisz R (2021) Real Assessment of Maximum Oxygen Uptake as a Verification After an Incremental Test Versus Without a Test. Front. Physiol. 12:739745. doi: 10.3389/fphys.2021.739745
Received: 11 July 2021; Accepted: 07 October 2021;
Published: 28 October 2021.
Edited by:
Gary W. Mack, Brigham Young University, United StatesReviewed by:
Filipe Dinato De Lima, University Center of Brasilia, BrazilMichael E. Tschakovsky, Queen’s University, Canada
Copyright © 2021 Hebisz, Jastrzębska and Hebisz. This is an open-access article distributed under the terms of the Creative Commons Attribution License (CC BY). The use, distribution or reproduction in other forums is permitted, provided the original author(s) and the copyright owner(s) are credited and that the original publication in this journal is cited, in accordance with accepted academic practice. No use, distribution or reproduction is permitted which does not comply with these terms.
*Correspondence: Agnieszka Danuta Jastrzębska, YWduaWVzemthLmphc3RyemVic2thQGF3Zi53cm9jLnBs