- 1Urology Surgery, Yueyang Hospital of Integrated Traditional Chinese and Western Medicine Hospital, Shanghai University of Traditional Chinese Medicine, Shanghai, China
- 2Nephrology, Shuguang Hospital, Shanghai University of Traditional Chinese Medicine, Shanghai, China
- 3Urology Surgery, Shanghai Seventh People's Hospital, Shanghai, China
Background: Renal ischemia-reperfusion (I/R) injury is one of the major causes related to acute kidney damage. Melatonin has been shown as a powerful antioxidant, with many animal experiments have been designed to evaluate the therapeutic effect of it to renal I/R injury.
Objectives: This systematic review aimed to assess the therapeutic effect of melatonin for renal I/R injury in animal models.
Methods and Results: The PubMed, Web of Science, Embase, and Science Direct were searched for animal experiments applying melatonin to treat renal I/R injury to February 2021. Thirty-one studies were included. The pooled analysis showed a greater reduction of blood urea nitrogen (BUN) (21 studies, weighted mean difference (WMD) = −30.00 [−42.09 to −17.91], p < 0.00001), and serum creatinine (SCr) (20 studies, WMD = −0.91 [−1.17 to −0.66], p < 0.00001) treated with melatonin. Subgroup analysis suggested that multiple administration could reduce the BUN compared with control. Malondialdehyde and myeloperoxidase were significantly reduced, meanwhile, melatonin significantly improved the activity of glutathione, as well as superoxide dismutase. The possible mechanism for melatonin to treat renal I/R injury is inhibiting endoplasmic reticulum stress, apoptosis, inflammation, autophagy, and fibrillation in AKI to chronic kidney disease.
Conclusions: From the available data of small animal studies, this systematic review demonstrated that melatonin could improve renal function and antioxidative effects to cure renal I/R injury through, then multiple administration of melatonin might be more appropriate. Nonetheless, extensive basic experiments are need to study the mechanism of melatonin, then well-designed randomized controlled trials to explore the protective effect of melatonin.
Introduction
Acute kidney injury (AKI) is defined as a sequence of clinical syndromes, refers to the sudden and continuous damage of renal function, which is manifested by a sharp reduction of glomerular filtration rate, with decreased urine output and azotemia (Levey and James, 2017). It is becoming increasingly clear that AKI is a non-negligible factor from chronic kidney disease (CKD) to chronic renal failure (Chawla and Kimmel, 2012). It was reported that 35 and 71% of AKI patients had incomplete recovery of renal function (Basile et al., 2001). Renal transplantation, systemic hypotension, hypovolemic shock, cardiovascular surgery, and partial nephrectomy as well as many other clinical conditions can lead to renal ischemia-reperfusion (I/R). As the most common cause related to AKI, renal I/R injury is an important factor leading to high mortality in intensive care units (Bansal et al., 2018; Smith et al., 2019). It would also cause the increase of antibodies, and the increased antibodies may impair renal transplantation, so as to explain the pathophysiological relationship between delayed renal allograft function and rejection in renal transplant recipients (Fuquay et al., 2013).
In recent years, researchers have deeply explored the changing signal pathways related to I/R, then put forward various mechanisms to explain the renal tissue damage. A reduced blood supply can lead to a low energy condition, causing loss of cell polarity and disorganization of organelles, especially mitochondrion, in the ischemia stage of renal I/R injury. Then subsequent reperfusion induces cause accumulation of a large number of reactive oxygen species (ROS) leading to oxidative stress, as well as mitochondrial and microvascular dysfunction, leading to more ROS production. ROS is generally considered as a key factor in renal I/R-induced injury. The release and accumulation of ROS activate a series of signaling pathways, including inflammation, apoptosis, autophagy, and endoplasmic reticulum (ER) stress in kidney (Inagi, 2009). The formation of the fibrotic tissues is the final manifestation from AKI to CKD situation, such as glomerulosclerosis, tubular atrophy, as well as renal interstitial fibrosis, which are characterized by fibroblasts activation and macrophage infiltrates (Webster et al., 2017).
Melatonin is one of the hormones secreted by the pineal gland that can construct circadian rhythm to regulate sleep and enhance immune function (Patel et al., 2020). Because of its high lipophilicity, melatonin can easily reach the subcellular structure through the cell membrane (Reiter et al., 2001). Melatonin has been shown as an excellent antioxidant due to the powerful ability to reduce ROS (Zhang and Zhang, 2014). This characteristic offers melatonin the ability to protect DNA against oxidative stress. In addition, melatonin also shows strong anti apoptotic and anti-inflammatory effects and other significant cytoprotective effects (Carrascal et al., 2018; Mortezaee et al., 2019; Xia et al., 2019). At present, many animal experimental studies related to the therapeutic action of melatonin for renal I/R injury have been published, however, there is still no final conclusion and whether it is due to its antioxidant properties. Also, a randomized controlled trial using melatonin on renal I/R injury in transplant patients was published in 2019, as only 20 patients were enrolled in each group, thus there were still doubts about its effect (Panah et al., 2019).
Systematic review is a secondary study that attempts to collect all evidence, use methodological methods to minimize the risk of bias and better evaluate the treatment effect in combine with individual studies. Therefore, we systematically reviewed and meta-analyzed the therapeutic action of melatonin in renal I/R injury rat models, and the mechanism of the renal protective effect of melatonin was also discussed.
Methods
Search Methods
A systematic literature search was performed in PubMed, Web of Science, Embase, and Science Direct from their inception date to February 2021. Keywords included “ischemia/reperfusion injury,” “ischemia-reperfusion injury,” “I/R injury,” “melatonin,” “N-acetyl-5-methoxytryptamine,” and “MT,” “MLT.” All object types were limited to animals, and there are no restrictions on language.
Study Selection
Two investigators (DRL and YM) screened the titles and abstracts of studies identified for this review independently. Download the full text of the screened articles to determine whether they meet the predetermined inclusion and exclusion criteria. Agreement of the study selection was determined using the quadratic-weighted kappa value (Kw). The result was accepted if the Kw value was >0.75. Disagreements were resolved through discussion with the third reviewers (YP).
Eligibility Criteria
Types of Studies
The controlled studies that evaluating melatonin administrated in vivo to renal I/R injury in experimental animals were included. Only in vitro experiments, case reports, and case series studies were ruled out. The study had no restrictions on language, publication date, as well as publication status.
Types of Animals
The study included experimental animals of any species, week age, as well as gender to induce renal I/R injury. The time of ischemia and reperfusion was not limited. Kidney transplantation, folic acid, and genetically modified induced AKI models were excluded.
Types of Intervention
Any types of melatonin that were compared to empty control or placebo were included. Dosage, dosage form, dissolvent, administration route, as well as melatonin administration time or duration were not limited. Placebo controls were not limited to physiological saline, absolute ethanol, or DMSO.
Type of Outcome
Serum creatinine (SCr) as well as blood urea nitrogen (BUN) that evaluated the renal function were considered as primary outcomes, ignoring the different detection methods. The biochemical indexes related to oxidative stress were secondary outcomes, including superoxide dismutase (SOD), malondialdehyde (MDA), myeloperoxidase (MPO), as well as glutathione (GSH). These tests were the most frequently used indicators of oxidative stress. The detection methods of MDA, MPO, SOD, and GSH and their corresponding numerical units are not limited.
Data Extraction
Data was extracted from the included studies independently by two reviewers (DRL and LTY), agreement of the data was determined using the quadratic-weighted kappa value (Kw). The result was accepted if the Kw value was >0.75. Disagreements were resolved through discussion with the third reviewers (YP). The following relevant information of each study was extracted in Table 1: (1) study's characteristics (i.e., first author's name, publication year, country), (2) animals (i.e., number of included animals, strain, gender, weight/week age), (3) I/R injury (i.e., kidney excised or not, duration of ischemia, left or right, unilateral or bilateral), (4) interventions (i.e., group, dosage and time, administration route, timing, and duration), and (5) data about BUN, SCr, MDA, MPO, SOD, and GSH. The mean results, standard deviation (SD), as well as the sample size of animals in each group were collected.
If any data were only displayed by graphs, the GetData Graph Digitizer 2.24 was adopted to estimate the results. Besides, in order to avoid human error, two reviewers (DRL and LTY) independently extracted relevant data from the papers. If the error was within the acceptable range (error ≤ 1% average data), the average data of two were used. Otherwise, the third investigator (MY) would extract the data again, and take the average of two data which were more close.
Assessment of the Risk of Bias
The methodological quality was assessed by using the CAMARADES 10-item checklist: (1) peer-reviewed journal; (2) temperature control; (3) animals were randomly allocated; (4) blind established model; (5) blinded outcome assessment; (6) anesthetics used without marked intrinsic neuroprotective properties; (7) animal model (diabetic, advanced age or hypertensive); (8) calculation of sample size; (9) statement of compliance with animal welfare regulations; (10) possible conflicts of interest (Macleod et al., 2004).
Two reviewers (DRL and LTY) assessed the risk of bias. Bias was marked as high or low risk, as well as “unclear” indicated that the risk of bias was unclear. The symbol “+” was used to marked low risk, and it was also recorded as the point of quality score. Agreement over the quality assessment was determined using the Kw. The Kw value >0.75 was accepted. Disagreements were resolved through discussion with the third reviewers (YP).
Statistical Analysis
If at least three studies reported the results of the same outcome, the data were summarized, thus we evaluated six outcomes separately (BUN, SCr, MDA, MPO, SOD, and GSH). First, we conducted a meta-analysis for studies comparing melatonin group to control group. The Review Manager 5.3 software was adopted to analyzed data. The results in this report were described as weighted mean difference (WMD) of measurements with same unit or standardized mean difference (SMD) using different units. We analyzed the pooled data to evaluate the therapeutic action of melatonin. The heterogeneity of included studies was evaluated by I2. The fixed effect models were adopted, if the heterogeneity was not obvious (i.e., p > 0.1; I2 ≤ 50%); when p ≤ 0.1; I2 > 50%, the source of heterogeneity was tried to detect by sensitivity analysis. Otherwise, random effect models were used (Higgins and Green, 2011).
Subgroup analysis was conducted between different ischemia duration (≤ 45 min, or > 45 min), times of administration (single or multiple), unilateral or bilateral I/R injury, dosage (< 10, 10, or > 10 mg/kg), administration time (before ischemia, or after reperfusion), and risk of bias (< 4, or ≥ 4). If we include at least 10 studies in a meta-analysis related to primary outcomes, funnel plots were used to test the potential risk of publication bias (Higgins and Green, 2011).
Results
Selection of Studies
The process of inclusion and exclusion is shown in Figure 1. Two hundred seventeen publications were identified after search. After removing duplicates and performing title and abstract screening, 35 papers were selected for full-text screening, and 31 met the inclusion criteria (Sener et al., 2002; Kunduzova et al., 2003; Sahna et al., 2003; Rodríguez-Reynoso et al., 2004; Aktoz et al., 2007; Kurcer et al., 2007a,b; Fadillioglu et al., 2008; Ersoz et al., 2009; Sinanoglu et al., 2012; Ahmadiasl et al., 2013, 2014a,b; Sezgin et al., 2013; Cetin et al., 2014; Sehajpal et al., 2014; Hadj Ayed Tka et al., 2015; Oguz et al., 2015; Yilmaz et al., 2015; Yip et al., 2015; Banaei et al., 2016a,b; Chang et al., 2016; Souza et al., 2018; Chen et al., 2019a,b; Shi et al., 2019; M El Agaty and Ibrahim Ahmed, 2020; Wang et al., 2020; Yang et al., 2020; Zahran et al., 2020). Of the four publications excluded at the full-text level, two did not conduct renal I/R injury to AKI (Li et al., 2009; Zhu et al., 2017), and the other two used melatonin combined with other treatments (mesenchymal stem cell-derived exosomes, or nitric oxide synthase inhibitor) (Deniz et al., 2006; Alzahrani, 2019). The Kw value of the 2 reviewers was 0.915, after discussion with the third review author, the differences were resolved by consensus.
Characteristics of the Studies Included
The majority of these studies were conducted in Turkey (n = 12), while the remaining in China (n = 6), Iran (n = 5), Egypt (n = 2), Brazil (n = 1), USA (n = 1), India (n = 1), France (n = 1), Mexico (n = 1), and Tunisia (n = 1) (Table 2). A unilateral nephrectomy was performed before contralateral renal I/R injury in 12 studies (Sener et al., 2002; Sahna et al., 2003; Rodríguez-Reynoso et al., 2004; Kurcer et al., 2007a; Sinanoglu et al., 2012; Ahmadiasl et al., 2013, 2014a,b; Sezgin et al., 2013; Oguz et al., 2015; Banaei et al., 2016a,b). Most of the studies conducted unilateral renal I/R injury, except 10 studies performed bilateral renal I/R injury (Ersoz et al., 2009; Sehajpal et al., 2014; Hadj Ayed Tka et al., 2015; Yip et al., 2015; Chen et al., 2019a,b; Shi et al., 2019; M El Agaty and Ibrahim Ahmed, 2020; Wang et al., 2020; Zahran et al., 2020). The duration of ischemia ranged from 25 to 75 min, then the reperfusion from 1 to 72 h. The sample size of a single group ranged between 5 and 12. The melatonin administration was < 10 mg/kg in eight studies (Kunduzova et al., 2003; Sahna et al., 2003; Kurcer et al., 2007b; Fadillioglu et al., 2008; Cetin et al., 2014; Sehajpal et al., 2014; Yilmaz et al., 2015; Wang et al., 2020), 10 mg/kg in 16 studies (Sener et al., 2002; Rodríguez-Reynoso et al., 2004; Aktoz et al., 2007; Kurcer et al., 2007a; Ersoz et al., 2009; Sinanoglu et al., 2012; Ahmadiasl et al., 2013, 2014a,b; Sezgin et al., 2013; Sehajpal et al., 2014; Oguz et al., 2015; Banaei et al., 2016a,b; Souza et al., 2018; Shi et al., 2019), and > 10 mg/kg in eight studies (Hadj Ayed Tka et al., 2015; Yip et al., 2015; Chang et al., 2016; Chen et al., 2019a,b; M El Agaty and Ibrahim Ahmed, 2020; Yang et al., 2020; Zahran et al., 2020). Most studies took melatonin intraperitoneal injection, except one with subcutaneous injection (Sener et al., 2002), one with intravenous injection (Kunduzova et al., 2003), and another one with oral administration (M El Agaty and Ibrahim Ahmed, 2020). Single-dose administration was conducted in 18 studies (Sener et al., 2002; Kunduzova et al., 2003; Sahna et al., 2003; Rodríguez-Reynoso et al., 2004; Kurcer et al., 2007a; Ersoz et al., 2009; Ahmadiasl et al., 2013, 2014a,b; Cetin et al., 2014; Sehajpal et al., 2014; Hadj Ayed Tka et al., 2015; Oguz et al., 2015; Banaei et al., 2016a,b; Souza et al., 2018; Wang et al., 2020; Zahran et al., 2020), while the others used multiple-dose administration (Deniz et al., 2006; Aktoz et al., 2007; Kurcer et al., 2007b; Fadillioglu et al., 2008; Sinanoglu et al., 2012; Sezgin et al., 2013; Yilmaz et al., 2015; Yip et al., 2015; Chang et al., 2016; Chen et al., 2019a,b; Shi et al., 2019; Yang et al., 2020). Two independent reviewers assessed the data, with a Kw of 0.817. Consensus was reached on 100% of the occasions when the reviewers initially disagreed with the third reviewer.
Risk of Bias Within Studies
The Kw value of the 2 reviewers was 0.903, consensus was reached on 100% of the occasions, and the risk of bias was in Table 2. The overall quality of research methodology is good, as 21 (80.65%) out of these 31 studies were labeled as high quality. All of them were peer reviewed publication, and applied appropriate animal model, and anesthetic without significant intrinsic vascular protection activity, but none of them reported sample size calculation, and only 10 studies did not report control of temperature (Sener et al., 2002; Kunduzova et al., 2003; Sinanoglu et al., 2012; Sehajpal et al., 2014; Yilmaz et al., 2015; Chen et al., 2019b; M El Agaty and Ibrahim Ahmed, 2020; Wang et al., 2020; Yang et al., 2020; Zahran et al., 2020). Randomization was conducted in 14 studies (Aktoz et al., 2007; Kurcer et al., 2007b; Fadillioglu et al., 2008; Ersoz et al., 2009; Sinanoglu et al., 2012; Sezgin et al., 2013; Yilmaz et al., 2015; Yip et al., 2015; Souza et al., 2018; Chen et al., 2019a,b; Shi et al., 2019; M El Agaty and Ibrahim Ahmed, 2020; Yang et al., 2020), then no study reported blinded induction of model, and blinded assessment of the outcome was applied in 15 studies (Sahna et al., 2003; Ersoz et al., 2009; Sinanoglu et al., 2012; Ahmadiasl et al., 2013, 2014a,b; Cetin et al., 2014; Yilmaz et al., 2015; Yip et al., 2015; Banaei et al., 2016a; Chang et al., 2016; Souza et al., 2018; Shi et al., 2019; Yang et al., 2020). Most of the studies reported compliance with animal welfare regulations (Sahna et al., 2003; Wang et al., 2020), while 10 studies reported potential conflicts of interest and study funding (Sener et al., 2002; Rodríguez-Reynoso et al., 2004; Aktoz et al., 2007; Ersoz et al., 2009; Ahmadiasl et al., 2013; Sehajpal et al., 2014; Yilmaz et al., 2015; Yip et al., 2015; Banaei et al., 2016a,b; Wang et al., 2020).
Bun
The BUN was reported in 21 studies, then animals treated with melatonin showed a greater reduction in BUN level compared with controls (21 studies, n = 324; WMD = −30.00; 95% CI = −42.09 to −17.91; p < 0.00001; Figure 2), with random-effects model. Subgroup analysis suggested that multiple administration of melatonin could not reduce the BUN level in renal I/R injury model compared with controls (11 studies, n = 160; WMD = −26.51; 95% CI = −36.86 to 16.15; p < 0.00001; Table 3), while single administration was the opposite (10 studies, n = 164; WMD = −28.92; 95% CI = −61.27 to 3.43; p = 0.08; Table 3). The other subgroup analysis found that the reduction of BUN level did not differ between different ischemia duration (≤ 45 min, or > 45 min), unilateral or bilateral I/R injury, dosage (< 10, 10, or > 10 mg/kg), administration time (before ischemia, or after reperfusion), or risk of bias (< 4, or ≥ 4). The subgroup analysis also concluded that enhancing the dosage of melatonin did not improve the protective effect of BUN compared to dosage of 10 mg/kg (10 studies, n = 148; WMD = −38.07; 95% CI = −70.19 to −5.94; p = 0.01; Table 3), and > 10 mg/kg (6 studies, n = 92; WMD = −15.25; 95% CI = −22.76 to −7.74; p < 0.0001; Table 3).
SCr
The pooled results from 20 studies showed a significant reduction in SCr level with melatonin treatment (20 studies, n = 288; WMD = −0.91; 95% CI = −1.17 to −0.66; p < 0.00001; Figure 3), with random-effects model. Subgroup analysis suggested that the reduction of SCr level did not differ between different ischemia duration (≤ 45 min, or > 45 min), times of administration (single or multiple), unilateral or bilateral I/R injury, dosage (< 10, 10, or > 10 mg/kg), administration time (before ischemia, or after reperfusion), and risk of bias (< 4, or ≥ 4). The subgroup analysis also concluded that enhancing the dosage of melatonin did not improve the protective effect of SCr compared to dosage of 10 mg/kg (7 studies, n = 122; WMD = −1.18; 95% CI = −1.85 to −0.51; p = 0.0006; Table 4), and > 10 mg/kg (6 studies, n = 94; WMD = −0.57; 95% CI = −0.80 to −0.34; p < 0.00001; Table 4).
Outcome Related to Oxidative Stress
Antioxidative effects were measured in most of the studies, except 9 studies (Kurcer et al., 2007b; Sinanoglu et al., 2012; Oguz et al., 2015; Yilmaz et al., 2015; Banaei et al., 2016b; Chang et al., 2016; Chen et al., 2019a,b; Wang et al., 2020). The MDA level (20 studies, n = 332; SMD = −2.76; 95% CI = −3.52 to −2.01; p < 0.00001; Figure 4) and the MPO level (6 studies, n = 88; SMD = −3.78; 95% CI = −6.60 to −0.95; p = 0.009; Figure 5) were significantly reduced after melatonin administration, while the SOD level (10 studies, n = 152; SMD = 1.79; 95% CI, 0.54 to 3.05; P = 0.005; Figure 6), and GSH level (6 studies, n = 98; SMD = 2.58; 95% CI, 1.33–3.82; P < 0.0001; Figure 7) were increased compared with control.
Publication Bias
The funnel plots of BUN and SCr were asymmetrical, hinting at a high risk of publication bias (Figures 8A,B).
Discussion
Summary of Evidence
This is the first preclinical meta-analysis of melatonin in the treatment of renal I/R injury. Thirty-one studies compared melatonin to placebo controls were enrolled. The overall quality of all the included studies was good, as there were 21 (80.65%) out of 31 studies were considered high quality.
Our findings indicated that melatonin can significantly improve renal function (BUN and SCr) and reduces oxidative stress (decreasing MDA and MPO, increasing SOD and GSH) of renal post-I/R injury in animals studies. The subgroup analysis also concluded that 10 mg/kg might be the appropriate dosage of melatonin for renal post-I/R injury, while enhancing the dosage of melatonin did not improve the therapeutic action of melatonin for renal I/R injury, and multiple administration might be more effective. Otherwise, the marked benefits of melatonin were not affected by either the duration of ischemia, duration of reperfusion, unilateral or bilateral I/R injury, timing of administration, or methodological quality.
The Possible Mechanism for the Effect of Melatonin in Renal I/R Injury
At present, there are various mechanisms to explain the origin of tissue damage. An important initiating step in renal I/R injury is the uncontrolled ROS production during reperfusion (Kim et al., 2010). From here, some injury cascades are activated, including loss of endothelial function and programmed leukocyte death (such as apoptosis and autophagy). The activation of the innate and acquired immune system can be achieved through the risk or injury related molecular patterns associated with toll like receptor (TLR) binding and the activation of complement system, resulting in the occurrence of fibrosis associated with chronic transplantation dysfunction (Ben Mosbah et al., 2010). While in this situation, melatonin can function as an antioxidant stress molecule could attenuate apoptosis, autophagy, inflammation, and fibrillation in renal I/R injury (Table 5, Figure 9).
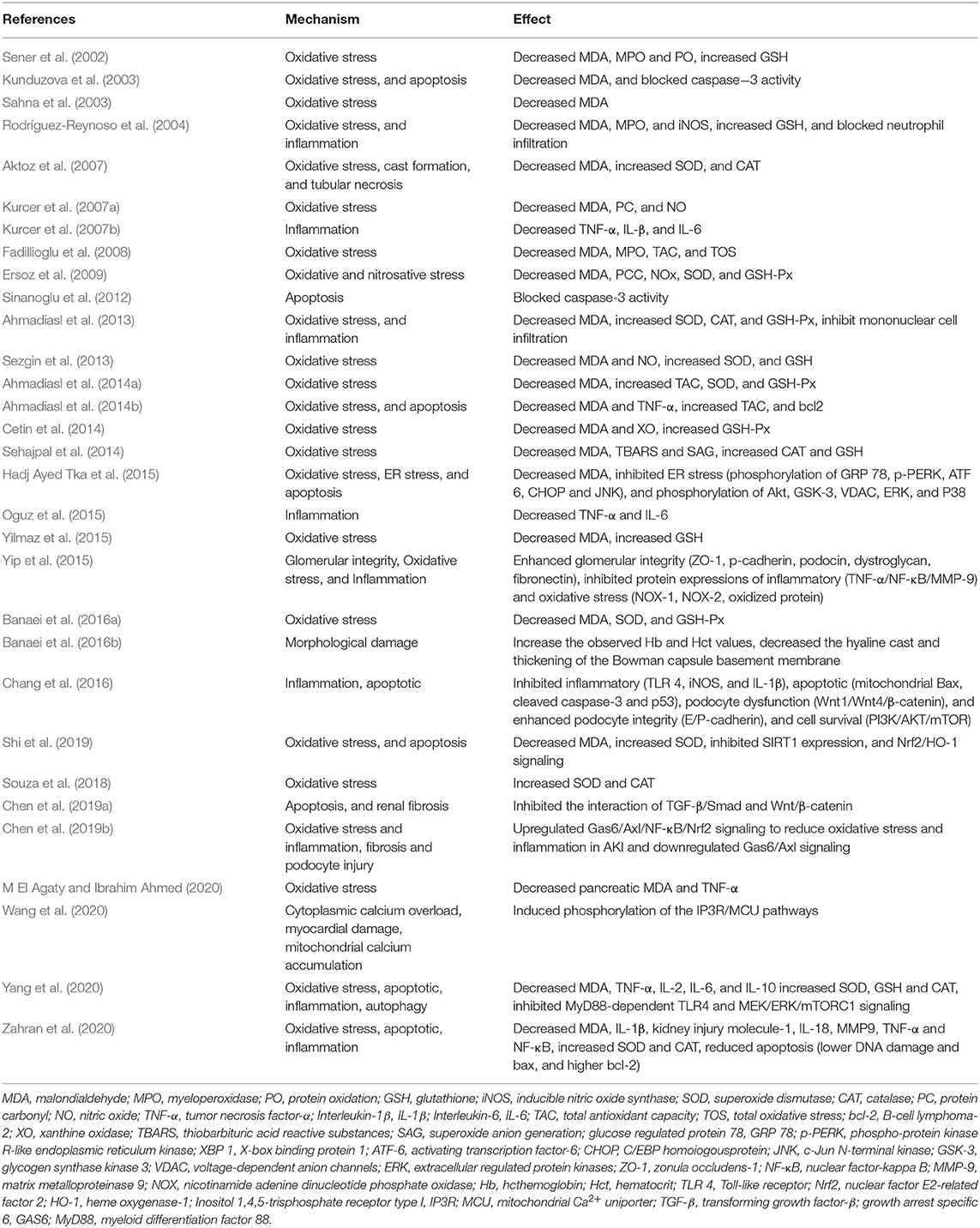
Table 5. The proposed molecular and cellular mechanism of the protective effect of melatonin for renal I/R injury.
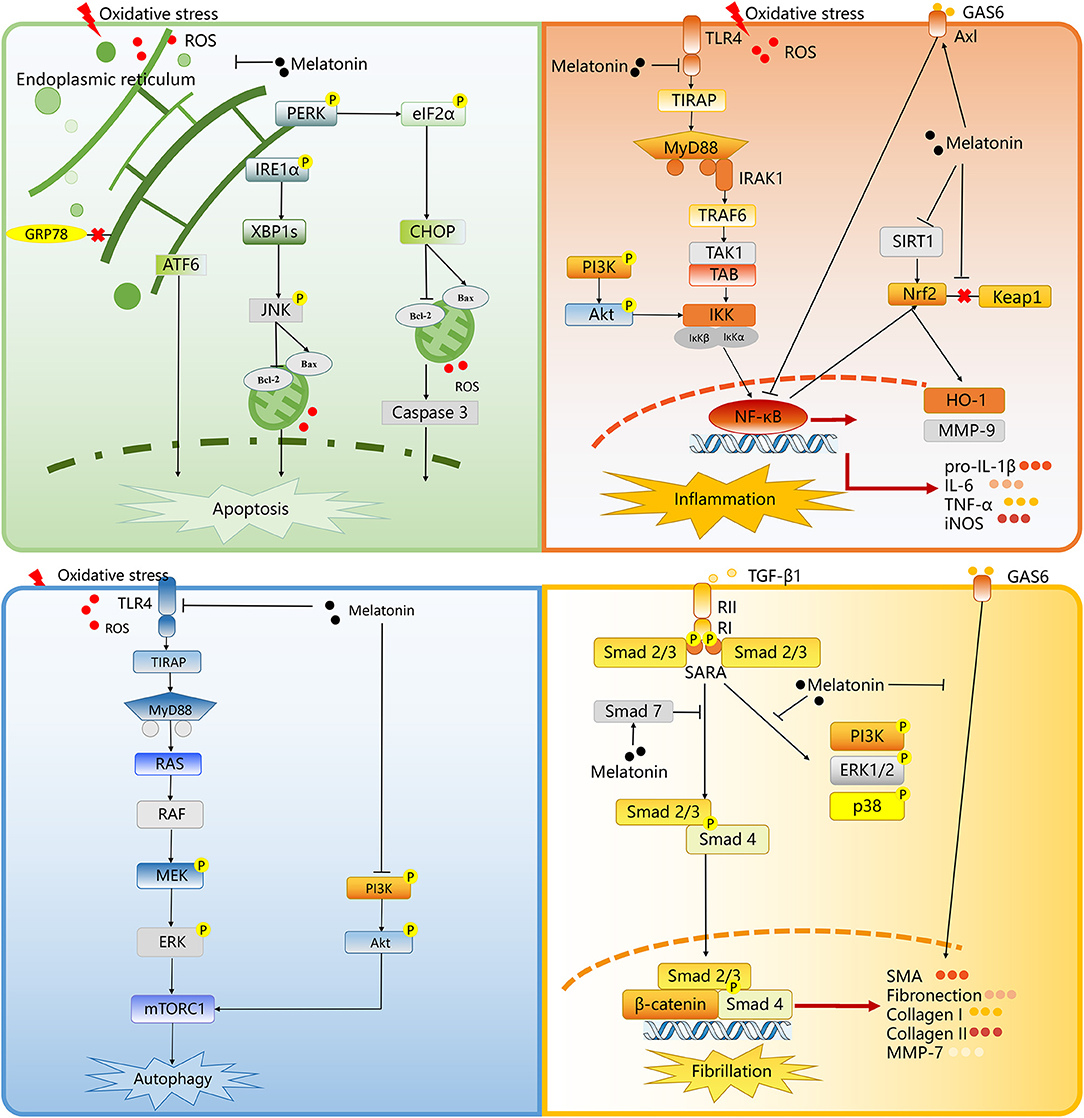
Figure 9. The proposed molecular and cellular mechanism of the protective effect of melatonin for renal I/R injury. The figure was created based on the data of the studies. ROS, reactive oxygen species; PERK, protein kinase RNA-like endoplasmic reticulum kinase; eIF2a, eukaryotic translation initiation factor 2 a; CHOP, C/EBP homologous protein; IRE1a, inositolrequiring enzyme 1 a; XBP1s, X-box binding protein 1; ATF6, activating transcription factor 6; JNK, c-Jun NH2-terminal kinase; Bcl-2, B cell lymphoma-2; Bax, BCL-2 associated X; GRP78, glucose regulated protein 78; TLR4, Toll-like receptor 4; MyD88, myeloid differentiation 88; IRAK1, interleukin-1 receptor-associated kinases; TRAF6, tumor necrosis factor receptor-associated factor 6; TAK1, transforming growth factor beta-activated kinase1; IKK, IkappaB kinase; PI3K, phosphatidylinositol 3-kinase; NF-κB, noncanonical nuclear factor-kappaB; HO-1, heme oxygenase 1; SIRT1, Sirtuin-1; MMP9, matrix metalloproteinase 9; GAS6, growth arrest-specific 6; Keap1, kelch-like ECH-associated protein 1; Nrf2, nuclear factor (erythroid-derived 2) factor 2; IL-6, interleukin 6; pro-IL-1ß, pro-interleukin-1beta; TNF-a, tumor necrosis factor-alpha; iNOS, inducible nitric oxide synthase; RAS, viral oncogene homolog; MEK, mitogen-activated protein kinase; ERK, extracellular regulated protein kinases; mTORC1, mammalian target of rapamycin complex 1; RII, transforming growth factor-ß receptor II; RI, transforming growth factor-ß receptor I; SARA, Smad anchor for receptor activation; TGF-ß1, transforming growth factor-ß; SMA, smooth muscle actin; MMP7, matrix metalloproteinase 7.
Protective Effect of Melatonin Related to Apoptosis
Oxidative stress and other states may cause ER stress. Under the condition of ER stress, ER chaperone, glucose-regulated protein (GRP) 78, leaving from ER membrane, and combining the free misfolding proteins, results in the activation of protein kinase-(PKR-) like ER kinase (PERK), inositol-requiring enzyme 1, and activating transcription factor 6, so as to reconstruct ER homeostasis (Ben Mosbah et al., 2010). Hadj Ayed Tka et al. revealed that melatonin treatment could inhibit ER stress in an experimental model of renal I/R injury (Hadj Ayed Tka et al., 2015). Melatonin inhibits ER stress by suppressing cell apoptosis. Melatonin can play a protective role by inhibiting phosphorylated c-Jun NH2 terminal kinase (JNK), as well as C/EBP homologous protein (CHOP). The CHOP and JNK activation, as downstream events of ER stress, can cause mitochondrial mediated apoptosis by promoting Bax aggregation in mitochondria (Yang et al., 2014; Yan et al., 2018).
Protective Effect of Melatonin Related to Inflammation
The TLRs act critical roles in the pathogen immune response, and TLR4 is an important regulator related to the inflammatory reaction in I/R injury (Zhang et al., 2016). The myeloid differentiation primary response 88 (MyD88) pathways is the TLR4-induced downstream pathways, then the former pathway promotes the expression of inflammation-associated genes by activating the transcription factors, nuclear factor (NF)-κB. It regulates the expression of a variety of inflammatory factors, such as interleukin (IL)-1β, and IL-6 (Su et al., 2019). Chang et al. and Yang et al. showed that melatonin could down-regulate the pathway of TLR4-MyD88-NF-κB and reduced the deliver of IL-6, IL-1β, as well as tumor necrosis factor (TNF)-α, eventually leading to the reduction of inflammation in the injured area (Chang et al., 2016; Yang et al., 2020). Otherwise, the activation of NF-κB is also enhanced according to growth arrest-specific 6 (Gas6)/Axl. In the early stage of I/R injury, Gas6/Axl inhibits its downstream mediator nuclear factor-erythroid-2-related factor 2 (Nrf2) and NF-κB to attenuate inflammation and oxidative stress after I/R in multiple organs (Llacuna et al., 2010; Ruiz et al., 2013). Oxidative stress and inflammation are closely related, as they jointly construct a vicious circle, that is, oxidative stress causes inflammation, which leading to the recruitment and activation of immunocytes. On the other hand, inflammation promotes oxidative stress by activating leukocytes and resident cells to produce ROS and reactive nitrogen species (He et al., 2017). Chen DQ et al., indicated that melatonin could upregulate Gas6/Axl to down-regulate NF-κB/Nrf2 signaling to reduce oxidative stress, as well as inflammation in the renal I/R injury early stage (Chen et al., 2019b).
Protective Effect of Melatonin Related to Autophagy
Autophagy plays a cardinal contribution in renal I/R injury. Injurious autophagy may lead to the release of excessive ROS and pro apoptotic factors, which ultimately cause cell death (Kurusu and Kuchitsu, 2017; Liu et al., 2019). Yang et al. suggested that a major pathway for melatonin-induced autophagy in I/R injury involves activating the TLR4-MyD88 signaling pathway (Yang et al., 2020). Mitogen-activated protein kinases (MAPK) pathways is actived by the MyD88 pathway (Liu et al., 2018). Mammalian target of rapamycin complex 1 (mTORC1), a convergence point for many upstream pathways, including MAPKs, regulates autophagy. Yang et al. revealed that melatonin-induced autophagy among renal I/R mice was dependent on downregulation of mTORC1 by its upstream activator, the TLR4/MyD88 (Yang et al., 2020).
Otherwise, it is known that phosphatidylinositol-3-kinase (PI3K)/AKT/mTOR pathway perform a core effect to regulate cell survival, as well as cell growth, and proliferation (Hassan et al., 2013). Another important finding of Chang YC was that PI3K/AKT/mTORC1 signaling pathway was significantly upregulated in the I/R kidney, while melatonin can significantly inhibit this signal pathway (Chang et al., 2016). It was claimed that the activation of PI3K/AKT/mTOR signaling under the I/R condition is the internal response of tissue or organs to I/R (i.e., the more serious, the stonger response), and melatonin could reverse this situation (Chang et al., 2016).
Protective Effect of Melatonin Related to Fibrillation
Fibrillation plays a leading role in renal I/R injury to accelerate kidney damage from AKI to CKD condition. The Wnt/β-catenin, as well as transforming growth factor-β (TGF-β)/Smad pathways perform important effects in the fibrosis of CKD (Yang et al., 2010; Meng et al., 2015). Under damage conditions, Wnt/β-catenin signaling is activated (Chen et al., 2017; Wang et al., 2017), and its sustained activation can promote renal fibrosis to induce the transition from AKI to CKD (Xiao et al., 2016). Fibrotic, as well as profibrotic genes, including twist, snail1, fibroblast-specific protein 1, and plasminogen activator inhibitor-1, are the downstream molecules related to Wnt/β-catenin signaling. TGF-β1 phosphorylate Smad2 and Smad3 to activate receptors through the combination with its adaptor, Smad anchor with its receptors TGF-β receptor I and II (Wang et al., 2017). The phosphorylated Smad2 and Smad3 pass through Wnt/β-catenin signaling to regulate the expression the profibrotic factor. Smad4 is also critical to TGF-β1-mediated fibrogenesis (Morishita et al., 2014), while Smad7, as the negative regulation factor of the TGF-β/Smad pathway, could inhibit phosphorylate Smad2 and Smad3 (Meng et al., 2012). Chen et al. demonstrated that melatonin performed extensive inhibitory effects on the TGF-β/Smad and Wnt/β-catenin pathways to prevent the AKI from progressing to CKD (Chen et al., 2019b).
Otherwise, in AKI to CKD condition, Gas6/Axl mentioned earlier serves as a profibrotic route and accelerates fibrogenesis (Bárcena et al., 2015). Then, melatonin could downregulate Gas6/Axl signaling pathway to prevent the fibrosis of the kidney (Chen et al., 2019b).
Advantage and Limitation of This Review
Combining the advantages of systematic review and traditional review, the study pooled the previous data to show that melatonin is a promising drug for renal I/R injury, and summarized the mechanism of melatonin. A deeper understanding of the mechanism of melatonin to treat renal I/R injury would not only help to explore the pathological mechanisms during renal I/R injury, but also contribute to the investigation of new effective drugs for further development and related translational research.
Inevitably, the article also has some limitations. First, since we cannot obtain the data of individual animals, the study can only be meta-analyzed at the overall level of each study (based on mean and standard deviation). The renal I/R injury models used of each study are different, different ischemia times for instance. The simple hybrid method is not the most appropriate method, but we can't analyze all the inconsistencies hierarchically. Then, the funnel chart is asymmetric, so the potential publication deviation cannot be ignored, which may affect the accuracy of the results.
Conclusion
This systematic review demonstrated that melatonin could improve renal function and antioxidative effects in renal I/R injury from the available data of small animal studies. A possible mechanism is that it can reduce ROS, thereby inhibiting apoptosis, inflammation, autophagy, and finally stopping fibrillation in AKI to CKD situation. Melatonin could be a promising drug to treat renal I/R injury. Nonetheless, extensive animal experimental studies are need to explore the mechanism of melatonin, and well-designed randomized controlled trials to explore the protective effect of melatonin.
Data Availability Statement
The original contributions presented in the study are included in the article/supplementary material, further inquiries can be directed to the corresponding author/s.
Author Contributions
The study was conceived by Rl-D, G-cQ, and YP. R-lD, T-yL, JT, X-hH, J-mM, Y-qS, and W-jZ developed the eligibility criteria, search strategy, risk of bias assessment strategy, and data extraction plan with guidance from G-cQ and YP. Rl-D wrote the manuscript. All authors contributed to the article and approved the submitted version.
Funding
This work was funded by National Natural Science Foundation of China (No. 81904070), Leading talents plan of TCM of Shanghai (No. ZY-2018-2020 - RCPY - 1017), and supported by Shanghai Key TCM specialty training program.
Conflict of Interest
The authors declare that the research was conducted in the absence of any commercial or financial relationships that could be construed as a potential conflict of interest.
Publisher's Note
All claims expressed in this article are solely those of the authors and do not necessarily represent those of their affiliated organizations, or those of the publisher, the editors and the reviewers. Any product that may be evaluated in this article, or claim that may be made by its manufacturer, is not guaranteed or endorsed by the publisher.
References
Ahmadiasl, N., Banaei, S., Alihemati, A., Baradaran, B., and Azimian, E. (2014a). Effect of a combined treatment with erythropoietin and melatonin on renal ischemia reperfusion injury in male rats. Clin. Exp. Nephrol. 18, 855–864. doi: 10.1007/s10157-014-0937-6
Ahmadiasl, N., Banaei, S., and Alihemmati, A. (2013). Combination antioxidant effect of erythropoietin and melatonin on renal ischemia-reperfusion injury in rats. Iran. J. Basic Med. Sci. 16, 1209–1216
Ahmadiasl, N., Banaei, S., Alihemmati, A., Baradaran, B., and Azimian, E. (2014b). The anti-inflammatory effect of erythropoietin and melatonin on renal ischemia reperfusion injury in male rats. Adv. Pharm. Bull. 4, 49–54. doi: 10.5681/apb.2014.008
Aktoz, T., Aydogdu, N., Alagol, B., Yalcin, O., Huseyinova, G., and Atakan, I. H. (2007). The protective effects of melatonin and vitamin E against renal ischemia-reperfusion injury in rats. Ren. Fail. 29, 535–542. doi: 10.1080/08860220701391738
Alzahrani, F. A. (2019). Melatonin improves therapeutic potential of mesenchymal stem cells-derived exosomes against renal ischemia-reperfusion injury in rats. Am. J. Transl. Res. 11, 2887–2907.
Banaei, S., Ahmadiasl, N., and Alihemmati, A. (2016a). Combination anti-apoptotic effect of erythropoietin and melatonin on ischemia reperfusion-induced renal injury in rats. Acta Med. Iran. 54, 624–630.
Banaei, S., Ahmadiasl, N., and Alihemmati, A. (2016b). Comparison of the protective effects of erythropoietin and melatonin on renal ischemia-reperfusion injury. Trauma Mon. 21:e23005. doi: 10.5812/traumamon.23005
Bansal, N., Matheny, M. E., Greevy, R. A. Jr., Eden, S. K., Perkins, A. M., Parr, S. K., et al. (2018). Acute kidney injury and risk of incident heart failure among US veterans. Am. J. Kidney Dis. 71, 236–245. doi: 10.1053/j.ajkd.2017.08.027
Bárcena, C., Stefanovic, M., Tutusaus, A., Joannas, L., Menéndez, A., García-Ruiz, C., et al. (2015). Gas6/Axl pathway is activated in chronic liver disease and its targeting reduces fibrosis via hepatic stellate cell inactivation. J. Hepatol. 63, 670–678. doi: 10.1016/j.jhep.2015.04.013
Basile, D. P., Donohoe, D., Roethe, K., and Osborn, J. L. (2001). Renal ischemic injury results in permanent damage to peritubular capillaries and influences long-term function. Am. J. Physiol. Renal Physiol. 281, F887–899. doi: 10.1152/ajprenal.00050.2001
Ben Mosbah, I., Alfany-Fernández, I., Martel, C., Zaouali, M. A., Bintanel-Morcillo, M., Rimola, A., et al. (2010). Endoplasmic reticulum stress inhibition protects steatotic and non-steatotic livers in partial hepatectomy under ischemia-reperfusion. Cell Death Dis. 1:e52. doi: 10.1038/cddis.2010.29
Carrascal, L., Nunez-Abades, P., Ayala, A., and Cano, M. (2018). Role of melatonin in the inflammatory process and its therapeutic potential. Curr. Pharm. Des. 24, 1563–1588. doi: 10.2174/1381612824666180426112832
Cetin, N., Suleyman, H., Sener, E., Demirci, E., Gundogdu, C., and Akcay, F. (2014). The prevention of ischemia/reperfusion induced oxidative damage by venous blood in rabbit kidneys monitored with biochemical, histopatological and immunohistochemical analysis. J. Physiol. Pharmacol. 65, 383–392.
Chang, Y. C., Hsu, S. Y., Yang, C. C., Sung, P. H., Chen, Y. L., Huang, T. H., et al. (2016). Enhanced protection against renal ischemia-reperfusion injury with combined melatonin and exendin-4 in a rodent model. Exp. Biol. Med. 241, 1588–1602. doi: 10.1177/1535370216642528
Chawla, L. S., and Kimmel, P. L. (2012). Acute kidney injury and chronic kidney disease: an integrated clinical syndrome. Kidney Int. 82, 516–524. doi: 10.1038/ki.2012.208
Chen, D. Q., Cao, G., Chen, H., Liu, D., Su, W., Yu, X. Y., et al. (2017). Gene and protein expressions and metabolomics exhibit activated redox signaling and wnt/β-catenin pathway are associated with metabolite dysfunction in patients with chronic kidney disease. Redox Biol. 12, 505–521. doi: 10.1016/j.redox.2017.03.017
Chen, D. Q., Cao, G., Zhao, H., Chen, L., Yang, T., Wang, M., et al. (2019a). Combined melatonin and poricoic acid A inhibits renal fibrosis through modulating the interaction of Smad3 and β-catenin pathway in AKI-to-CKD continuum. Ther. Adv. Chronic Dis. 10:2040622319869116. doi: 10.1177/2040622319869116
Chen, D. Q., Feng, Y. L., Chen, L., Liu, J. R., Wang, M., Vaziri, N. D., et al. (2019b). Poricoic acid A enhances melatonin inhibition of AKI-to-CKD transition by regulating Gas6/AxlNFκB/Nrf2 axis. Free Radic. Biol. Med. 134, 484–497. doi: 10.1016/j.freeradbiomed.2019.01.046
Deniz, E., Colakoglu, N., Sari, A., Sonmez, M. F., Tugrul, I., Oktar, S., et al. (2006). Melatonin attenuates renal ischemia-reperfusion injury in nitric oxide synthase inhibited rats. Acta Histochem. 108, 303–309. doi: 10.1016/j.acthis.2006.04.002
Ersoz, N., Guven, A., Cayci, T., Uysal, B., Turk, E., Oztas, E., et al. (2009). Comparison of the efficacy of melatonin and 1400W on renal ischemia/reperfusion injury: a role for inhibiting iNOS. Ren. Fail. 31, 704–710. doi: 10.3109/08860220903085989
Fadillioglu, E., Kurcer, Z., Parlakpinar, H., Iraz, M., and Gursul, C. (2008). Melatonin treatment against remote organ injury induced by renal ischemia reperfusion injury in diabetes mellitus. Arch. Pharm. Res. 31, 705–712. doi: 10.1007/s12272-001-1216-3
Fuquay, R., Renner, B., Kulik, L., McCullough, J. W., Amura, C., Strassheim, D., et al. (2013). Renal ischemia-reperfusion injury amplifies the humoral immune response. J. Am. Soc. Nephrol. 24, 1063–1072. doi: 10.1681/ASN.2012060560
Hadj Ayed Tka, K., Mahfoudh Boussaid, A., Zaouali, M. A., Kammoun, R., Bejaoui, M., Ghoul Mazgar, S., et al. (2015). Melatonin modulates endoplasmic reticulum stress and Akt/GSK3-beta signaling pathway in a rat model of renal warm ischemia reperfusion. Anal. Cell. Pathol. 2015:635172. doi: 10.1155/2015/635172
Hassan, B., Akcakanat, A., Holder, A. M., and Meric-Bernstam, F. (2013). Targeting the PI3-kinase/Akt/mTOR signaling pathway. Surg. Oncol. Clin. N. Am. 22, 641–664. doi: 10.1016/j.soc.2013.06.008
He, L., Wei, Q., Liu, J., Yi, M., Liu, Y., Liu, H., et al. (2017). AKI on CKD: heightened injury, suppressed repair, and the underlying mechanisms. Kidney Int. 92, 1071–1083. doi: 10.1016/j.kint.2017.06.030
Higgins, J. P. T., and Green, S. E. (2011). Cochrane Handbook for Systematic Reviews of Interventions Version 5.1.0 [updated March 2011]. The Cochrane Collaboration. Available online at: https://training.cochrane.org/handbook
Inagi, R. (2009). Endoplasmic reticulum stress in the kidney as a novel mediator of kidney injury. Nephron Exp. Nephrol. 112, e1–9. doi: 10.1159/000210573
Kim, J., Jang, H. S., and Park, K. M. (2010). Reactive oxygen species generated by renal ischemia and reperfusion trigger protection against subsequent renal ischemia and reperfusion injury in mice. Am. J. Physiol. Renal Physiol. 298, F158–166. doi: 10.1152/ajprenal.00474.2009
Kunduzova, O. R., Escourrou, G., Seguelas, M. H., Delagrange, P., De La Farge, F., Cambon, C., et al. (2003). Prevention of apoptotic and necrotic cell death, caspase-3 activation, and renal dysfunction by melatonin after ischemia/reperfusion. FASEB J. 17, 872–874. doi: 10.1096/fj.02-0504fje
Kurcer, Z., Oguz, E., Ozbilge, H., Baba, F., Aksoy, N., Celik, H., et al. (2007a). Melatonin protects from ischemia/reperfusion-induced renal injury in rats: this effect is not mediated by proinflammatory cytokines. J. Pineal Res. 43, 172–178. doi: 10.1111/j.1600-079X.2007.00459.x
Kurcer, Z., Parlakpinar, H., Vardi, N., Tasdemir, S., Iraz, M., and Fadillioglu, E., (2007b) Protective effects of chronic melatonin treatment against renal ischemia/reperfusion injury in streptozotocin-induced diabetic rats. Exp. Clin. Endocrinol. diabet. 115, 365–371. doi: 10.1055/s-2007-971056.
Kurusu, T., and Kuchitsu, K. (2017). Autophagy, programmed cell death and reactive oxygen species in sexual reproduction in plants. J. Plant Res. 130, 491–499. doi: 10.1007/s10265-017-0934-4
Levey, A. S., and James, M. T. (2017). Acute kidney injury. Ann. Internal Med. 167, Itc66–itc80. doi: 10.7326/AITC201711070
Li, Z., Nickkholgh, A., Yi, X., Bruns, H., Gross, M. L., Hoffmann, K., et al. (2009). Melatonin protects kidney grafts from ischemia/reperfusion injury through inhibition of NF-kB and apoptosis after experimental kidney transplantation. J. Pineal Res. 46, 365–372. doi: 10.1111/j.1600-079X.2009.00672.x
Liu, H., Dong, J., Song, S., Zhao, Y., Wang, J., Fu, Z., et al. (2019). Spermidine ameliorates liver ischaemia-reperfusion injury through the regulation of autophagy by the AMPK-mTOR-ULK1 signalling pathway. Biochem. Biophys. Res. Commun. 519, 227–233. doi: 10.1016/j.bbrc.2019.08.162
Liu, H., Zhou, K., Liao, L., Zhang, T., Yang, M., and Sun, C. (2018). Lipoxin A4 receptor agonist BML-111 induces autophagy in alveolar macrophages and protects from acute lung injury by activating MAPK signaling. Respir. Res. 19:243. doi: 10.1186/s12931-018-0937-2
Llacuna, L., Bárcena, C., Bellido-Martín, L., Fernández, L., Stefanovic, M., Marí, M., et al. (2010). Growth arrest-specific protein 6 is hepatoprotective against murine ischemia/reperfusion injury. Hepatology. 52, 1371–1379. doi: 10.1002/hep.23833
M El Agaty, S., and Ibrahim Ahmed, A (2020). Pathophysiological and immunohistochemical analysis of pancreas after renal ischemia/reperfusion injury: protective role of melatonin. Arch. Physiol. Biochem. 126, 264–275. doi: 10.1080/13813455.2018.1517182
Macleod, M. R., O'Collins, T., Howells, D. W., and Donnan, G. A. (2004). Pooling of animal experimental data reveals influence of study design and publication bias. Stroke 35, 1203–1208. doi: 10.1161/01.STR.0000125719.25853.20
Meng, X. M., Huang, X. R., Xiao, J., Chung, A. C., Qin, W., Chen, H. Y., et al. (2012). Disruption of Smad4 impairs TGF-β/Smad3 and Smad7 transcriptional regulation during renal inflammation and fibrosis in vivo and in vitro. Kidney Int. 81, 266–279. doi: 10.1038/ki.2011.327
Meng, X. M., Tang, P. M., Li, J., and Lan, H. Y. (2015). TGF-β/Smad signaling in renal fibrosis. Front. Physiol. 6:82. doi: 10.3389/fphys.2015.00082
Morishita, Y., Yoshizawa, H., Watanabe, M., Ishibashi, K., Muto, S., Kusano, E., et al. (2014). siRNAs targeted to Smad4 prevent renal fibrosis in vivo. Sci. Rep. 4:6424. doi: 10.1038/srep06424
Mortezaee, K., Najafi, M., Farhood, B., Ahmadi, A., Potes, Y., Shabeeb, D., et al. (2019). Modulation of apoptosis by melatonin for improving cancer treatment efficiency: an updated review. Life Sci. 228, 228–241. doi: 10.1016/j.lfs.2019.05.009
Oguz, E., Yilmaz, Z., Ozbilge, H., Baba, F., Tabur, S., Yerer, M. B., et al. (2015). Effects of melatonin on the serum levels of pro-inflammatory cytokines and tissue injury after renal ischemia reperfusion in rats. Ren. Fail. 37, 318–322. doi: 10.3109/0886022X.2014.991263
Panah, F., Ghorbanihaghjo, A., Argani, H., Haiaty, S., Rashtchizadeh, N., Hosseini, L., et al. (2019). The effect of oral melatonin on renal ischemia-reperfusion injury in transplant patients: a double-blind, randomized controlled trial. Transpl. Immunol. 57:101241. doi: 10.1016/j.trim.2019.101241
Patel, S., Rahmani, B., Gandhi, J., Seyam, O., Joshi, G., Reid, I., et al. (2020). Revisiting the pineal gland: a review of calcification, masses, precocious puberty, and melatonin functions. Int. J. Neurosci. 130, 464–475. doi: 10.1080/00207454.2019.1692838
Reiter, R. J., Tan, D. X., Manchester, L. C., and Qi, W. (2001). Biochemical reactivity of melatonin with reactive oxygen and nitrogen species: a review of the evidence. Cell Biochem. Biophys. 34, 237–256. doi: 10.1385/CBB:34:2:237
Rodríguez-Reynoso, S., Leal, C., Portilla-de Buen, E., Castillo, J. C., and Ramos-Solano, F. (2004). Melatonin ameliorates renal ischemia/reperfusion injury. J. Surg. Res. 116, 242–247. doi: 10.1016/j.jss.2003.10.002
Ruiz, S., Pergola, P. E., Zager, R. A., and Vaziri, N. D. (2013). Targeting the transcription factor Nrf2 to ameliorate oxidative stress and inflammation in chronic kidney disease. Kidney Int. 83, 1029–1041. doi: 10.1038/ki.2012.439
Sahna, E., Parlakpinar, H., Ozturk, F., Cigremis, Y., and Acet, A. (2003). The protective effects of physiological and pharmacological concentrations of melatonin on renal ischemia-reperfusion injury in rats. Urol. Res. 31, 188–193. doi: 10.1007/s00240-003-0314-5
Sehajpal, J., Kaur, T., Bhatti, R., and Singh, A. P. (2014). Role of progesterone in melatonin-mediated protection against acute kidney injury. J. Surg. Res. 191, 441–447. doi: 10.1016/j.jss.2014.04.025
Sener, G., Sehirli, A. O., Keyer-Uysal, M., Arbak, S., Ersoy, Y., and Yegen, B. C. (2002). The protective effect of melatonin on renal ischemia-reperfusion injury in the rat. J. Pineal Res. 32, 120–126. doi: 10.1034/j.1600-079x.2002.1848.x
Sezgin, G., Oztürk, G., Güney, S., Sinanoglu, O., and Tunçdemir, M. (2013). Protective effect of melatonin and 1,25-dihydroxyvitamin D3 on renal ischemia-reperfusion injury in rats. Ren. Fail. 35, 374–379. doi: 10.3109/0886022X.2012.760409
Shi, S., Lei, S., and Tang, C. (2019). Melatonin attenuates acute kidney ischemia/reperfusion injury in diabetic rats by activation of the SIRT1/Nrf2/HO-1 signaling pathway. Biosci. Rep. 39:BSR20181614. doi: 10.1042/BSR20181614
Sinanoglu, O., Sezgin, G., Ozturk, G., Tuncdemir, M., Guney, S., Aksungar, F. B., et al. (2012). Melatonin with 1,25-dihydroxyvitamin D3 protects against apoptotic ischemia-reperfusion injury in the rat kidney. Ren. Fail. 34, 1021–1026. doi: 10.3109/0886022X.2012.700887
Smith, S. F., Hosgood, S. A., and Nicholson, M. L. (2019). Ischemia-reperfusion injury in renal transplantation: 3 key signaling pathways in tubular epithelial cells. Kidney Int. 95, 50–56. doi: 10.1016/j.kint.2018.10.009
Souza, P. C., Santos, E. B. D., Motta, G. L., Bona, S. R., Schaefer, P. G., Campagnol, D., et al. (2018). Combined effects of melatonin and topical hypothermia on renal ischemia-reperfusion injury in rats. Acta Cirurgica Brasileira 33, 197–206. doi: 10.1590/s0102-865020180030000001
Su, S., Zhang, P., Zhang, Q., and Yin, Z. (2019). GSK-3β inhibitor induces expression of the TLR4/MyD88/NF-κB signaling pathway to protect against renal ischemia-reperfusion injury during rat kidney transplantation. Inflammation 42, 2105–2118. doi: 10.1007/s10753-019-01074-2
Wang, J., Toan, S., Li, R., and Zhou, H. (2020). Melatonin fine-tunes intracellular calcium signals and eliminates myocardial damage through the IP3R/MCU pathways in cardiorenal syndrome type 3. Biochem. Pharmacol. 174:113832. doi: 10.1016/j.bcp.2020.113832
Wang, M., Chen, D. Q., Wang, M. C., Chen, H., Chen, L., Liu, D., et al. (2017). Poricoic acid ZA, a novel RAS inhibitor, attenuates tubulo-interstitial fibrosis and podocyte injury by inhibiting TGF-β/Smad signaling pathway. Phytomedicine 36, 243–253. doi: 10.1016/j.phymed.2017.10.008
Webster, A. C., Nagler, E. V., Morton, R. L., and Masson, P. (2017). Chronic kidney disease. Lancet 389, 1238–1252. doi: 10.1016/S0140-6736(16)32064-5
Xia, Y., Chen, S., Zeng, S., Zhao, Y., Zhu, C., Deng, B., et al. (2019). Melatonin in macrophage biology: current understanding and future perspectives. J. Pineal Res. 66:e12547. doi: 10.1111/jpi.12547
Xiao, L., Zhou, D., Tan, R. J., Fu, H., Zhou, L., Hou, F. F., et al. (2016). Sustained activation of Wnt/β-Catenin signaling drives AKI to CKD progression. J. Am. Soc. Nephrol. 27, 1727–1740. doi: 10.1681/ASN.2015040449
Yan, M., Shu, S., Guo, C., Tang, C., and Dong, Z. (2018). Endoplasmic reticulum stress in ischemic and nephrotoxic acute kidney injury. Ann. Med. 50, 381–390. doi: 10.1080/07853890.2018.1489142
Yang, F., Huang, X. R., Chung, A. C., Hou, C. C., Lai, K. N., and Lan, H. Y. (2010). Essential role for Smad3 in angiotensin II-induced tubular epithelial-mesenchymal transition. J. Pathol. 221, 390–401. doi: 10.1002/path.2721
Yang, J., Liu, H., Han, S., Fu, Z., Wang, J., Chen, Y., et al. (2020). Melatonin pretreatment alleviates renal ischemia-reperfusion injury by promoting autophagic flux via TLR4/MyD88/MEK/ERK/mTORC1 signaling. FASEB J. 34, 12324–12337. doi: 10.1096/fj.202001252R
Yang, J. R., Yao, F. H., Zhang, J. G., Ji, Z. Y., Li, K. L., Zhan, J., et al. (2014). Ischemia-reperfusion induces renal tubule pyroptosis via the CHOP-caspase-11 pathway. Am. J. Physiol. Renal Physiol. 306, F75–84. doi: 10.1152/ajprenal.00117.2013
Yilmaz, M., Mogulkoc, R., and Baltaci, A. K. (2015). Effect of three-week zinc and melatonin supplementation on the oxidant-antioxidant system in experimental renal ischemia-reperfusion in rats. Acta Clin. Croat. 54, 395–401.
Yip, H. K., Yang, C. C., Chen, K. H., Huang, T. H., Chen, Y. L., Zhen, Y. Y., et al. (2015). Combined melatonin and exendin-4 therapy preserves renal ultrastructural integrity after ischemia-reperfusion injury in the male rat. J. Pineal Res. 59, 434–447. doi: 10.1111/jpi.12273
Zahran, R., Ghozy, A., Elkholy, S. S., El-Taweel, F., and El-Magd, M. A. (2020). Combination therapy with melatonin, stem cells and extracellular vesicles is effective in limiting renal ischemia-reperfusion injury in a rat model. Int. J. Urol. 27, 1039–1049. doi: 10.1111/iju.14345
Zhang, H. M., and Zhang, Y. (2014). Melatonin: a well-documented antioxidant with conditional pro-oxidant actions. J. Pineal Res. 57, 131–146. doi: 10.1111/jpi.12162
Zhang, J., Xia, J., Zhang, Y., Xiao, F., Wang, J., Gao, H., et al. (2016). HMGB1-TLR4 signaling participates in renal ischemia reperfusion injury and could be attenuated by dexamethasone-mediated inhibition of the ERK/NF-κB pathway. Am. J. Transl. Res. 8, 4054–4067.
Zhu, F., Chong Lee Shin, O. L., Xu, H., Zhao, Z., Pei, G., Hu, Z., et al. (2017). Melatonin promoted renal regeneration in folic acid-induced acute kidney injury via inhibiting nucleocytoplasmic translocation of HMGB1 in tubular epithelial cells. Am. J. Transl. Res. 9, 1694–1707. doi: 10.1093/ndt/gfx142.SP182
Keywords: renal ischemia-reperfusion injury, melatonin, systematic review, reactive oxygen species, meta-analysis
Citation: Dun R-l, Lan T-y, Tsai J, Mao J-m, Shao Y-q, Hu X-h, Zhu W-j, Qi G-c and Peng Y (2022) Protective Effect of Melatonin for Renal Ischemia-Reperfusion Injury: A Systematic Review and Meta-Analysis. Front. Physiol. 12:791036. doi: 10.3389/fphys.2021.791036
Received: 04 November 2021; Accepted: 16 December 2021;
Published: 13 January 2022.
Edited by:
Adriana Castello Costa Girardi, University of São Paulo, BrazilReviewed by:
Abdelaziz M. Hussein, Mansoura University, EgyptChunling Li, Sun Yat-sen University, China
Copyright © 2022 Dun, Lan, Tsai, Mao, Shao, Hu, Zhu, Qi and Peng. This is an open-access article distributed under the terms of the Creative Commons Attribution License (CC BY). The use, distribution or reproduction in other forums is permitted, provided the original author(s) and the copyright owner(s) are credited and that the original publication in this journal is cited, in accordance with accepted academic practice. No use, distribution or reproduction is permitted which does not comply with these terms.
*Correspondence: Yu Peng, Nzc2NTc2MDIwQHFxLmNvbQ==
†These authors have contributed equally to this work and share first authorship