- 1College of Physical Education, Shenzhen University, Shenzhen, China
- 2College of Physical Education, Southwest University, Chongqing, China
- 3Institute of Textiles and Clothing, The Hong Kong Polytechnic University, Hong Kong, China
- 4School of Physical Education and Sports Exercise, South China Normal University, Guangzhou, China
Background: Lower extremity stiffness simulates the response of the lower extremity to landing in running. However, its relationship with running economy (RE) remains unclear. This study aims to explore the relationship between lower extremity stiffness and RE.
Methods: This study utilized articles from the Web of Science, PubMed, and Scopus discussing the relationships between RE and indicators of lower extremity stiffness, namely vertical stiffness, leg stiffness, and joint stiffness. Methodological quality was assessed using the Joanna Australian Centre for Evidence-Based Care (JBI). Pearson correlation coefficients were utilized to summarize effect sizes, and meta-regression analysis was used to assess the extent of this association between speed and participant level.
Result: In total, thirteen studies involving 272 runners met the inclusion criteria and were included in this review. The quality of the thirteen studies ranged from moderate to high. The meta-analysis results showed a negative correlation between vertical stiffness (r = −0.520, 95% CI, −0.635 to −0.384, p < 0.001) and leg stiffness (r = −0.568, 95% CI, −0.723 to −0.357, p < 0.001) and RE. Additional, there was a small negative correlation between knee stiffness and RE (r = −0.290, 95% CI, −0.508 to −0.037, p = 0.025). Meta-regression results showed that the extent to which leg stiffness was negatively correlated with RE was influenced by speed (coefficient = −0.409, p = 0.020, r2 = 0.79) and participant maximal oxygen uptake (coefficient = −0.068, p = 0.010, r2 = 0.92).
Conclusion: The results of this study suggest that vertical, leg and knee stiffness were negatively correlated with RE. In addition, maximum oxygen uptake and speed will determine whether the runner can take full advantage of leg stiffness to minimize energy expenditure.
Introduction
Running is a popular sport around the world. Statistics from the US government show that more than 55.9 million people in 2017 were involved in running, jogging, and trail running (Statista, 2018). Khammassi et al. (2020) state that these regular, high-intensity physical activities offer many health benefits. In another study, Ezzatvar et al. (2022) stated that running can reduce the risk of infection and improve immune function, especially during pandemics such as the COVID-19 pandemic. Although running is considered an effective approach to maintain the health of the body, runners use it to enhance their athletic performance. There are many complex factors affecting the performance of runners. These factors include maximum oxygen uptake, lactate threshold and running economy (Joyner, 1991). Running economy (RE) seems to be the most appropriate parameter to distinguish between the endurance running performance of untrained and trained runners (Conley and Krahenbuhl, 1980; Williams and Cavanagh, 1987; Santos-Concejero et al., 2014). Running economy is the oxygen uptake at a given submaximal running speed (Conley and Krahenbuhl, 1980; Morgan and Craib, 1992; Anderson, 1996). Some studies define RE as the energy cost per unit distance (Rodrigo-Carranza et al., 2021). Running economy is one of the most important factors in determining endurance running performance (di Prampero et al., 1986).
When a person is running, bones, muscles, tendons, ligaments and other elements are usually modeled as Spring Mass Model (SMM) to support the body running forward (Blickhan, 1989; Butler et al., 2003). When a person is running, sufficient lower extremity stiffness is required to maintain motor performance. The mechanical stiffness of the lower extremity may be reflected by the vertical, leg, and joint stiffness (McMahon and Cheng, 1990; Butler et al., 2003).
Vertical stiffness describes the vertical displacement of the center of mass (COM) in response to vertical ground reaction force during a task performed in the sagittal plane (Latash and Zatsiorsky, 1993). The vertical stiffness is considered the first stiffness parameter to be measured, and the models for leg and joint stiffness were expanded (Morin et al., 2005; Brughelli and Cronin, 2008). The measurement of vertical stiffness requires the least amount of equipment in the experiment and can be obtained fast (Maloney and Fletcher, 2021). However, the calculation of vertical stiffness only attempts to model the cumulative stiffness of the lower extremity holistically and does not consider the effects of specific details. Leg stiffness characterizes the structural components of the leg, including muscles, tendons, and ligaments (Kerdok et al., 2002), which are an important component of the stretch-shortening cycle (SSC). These structural components of the leg reflect the compression of the leg spring in any plane or direction corresponding to the force (McMahon and Cheng, 1990; Hunter and Smith, 2007). During running, the leg touches the ground at a certain angle (θ). Therefore, the leg spring compression is greater than the COM displacement. Some studies have found that the leg stiffness is always less than the vertical stiffness (Farley and González, 1996; Matt; Brughelli and Cronin, 2008). Also, when the theta angle is 0 during vertical jumping, it leads to a leg stiffness that produces the same value as the vertical stiffness (Beerse and Wu, 2017). Many researchers refer to the results calculated using the equation for vertical stiffness as leg stiffness, which may cause confusion among readers about this concept (Maloney and Fletcher, 2021; Struzik et al., 2021).
The vertical stiffness and leg stiffness were calculated using the whole lower extremity as a whole SMM (Blickhan, 1989; Butler et al., 2003), and such measurements do not take into account multiple degrees of freedom of the lower extremity. Therefore, Farley and his colleagues proposed a joint stiffness calculated with the torsion spring model. First, the researchers deconstructed the hip, knee and ankle joints into three torsion springs (Farley et al., 1998). Then, the relative contribution of the stiffness of the three joints to the overall leg spring stiffness was evaluated (Farley et al., 1998). Joint stiffness describes the resistance to changes in angular displacement in flexion and rotation after applying a joint moment (Latash and Zatsiorsky, 1993; Butler et al., 2003; Brughelli and Cronin, 2008). The inverse dynamics principle obtains this net joint moment. By using a torsional spring model, joint stiffness values can be estimated for the hip, knee and ankle joints during vertical and horizontal motion. Previously, neither the vertical nor the leg stiffness could detect how each joint relative contributes to the stiffness of the whole leg. Joint stiffness in a running task can be used to consider the relative contribution of each joint to total stiffness (Butler et al., 2003; Maloney and Fletcher, 2021).
Researchers started exploring the relationship between stiffness and energy expenditure in the 1990s. However, there is still no higher quality evidence for the relationship between lower extremity stiffness and energy expenditure, and some studies have even found opposite results for each other (Tam et al., 2018; Zhang et al., 2022). Furthermore, no scholar has yet reviewed specifically the relationship between lower extremity stiffness and RE. If lower extremity stiffness is related to RE, these results may be a key indicator to evaluate the exercise performance of endurance runners. The purpose of this study was to explore whether lower extremity stiffness was associated with RE in endurance runners.
Methods
This review followed the Preferred Reporting Items for Systematic Review and Meta-Analyses (PRISMA) statement for reporting systematic reviews.
Search strategy
The literature search was conducted on three databases, PubMed, Web of Science, and Scopus. Studies published between 1980 and 2022 were screened. Journal article titles, abstracts, and keywords in each database were searched using the following terms and Boolean operators: (running economy OR energy cost OR metabolic cost OR energetics OR VO2 OR VO2max OR cost of running OR consumed oxygen OR oxygen uptake) AND (leg OR lower limb OR lower extremity OR vertical OR joint) AND (stiffness).
The study was conducted in two stages. First, two independent reviewers evaluated and included potential studies based on titles and abstracts. Second, the selected research papers were categorized as meeting the inclusion criteria (yes), likely to be included (maybe) and not meeting the inclusion criteria (no). Finally, disagreements between the two independent reviewers were discussed and resolved in a consensus session. A third reviewer was consulted where consensus could not be reached.
Inclusion criteria and data extraction
The articles included in this study met the following criteria: 1) Healthy middle-distance runners aged >18 years with a maximal oxygen uptake (VO2max) > 50 ml/kg/min. 2) The study included the observation of both RE and lower extremity stiffness metrics. 3) Correlations between lower-extremity stiffness and RE, such as Pearson’s correlation coefficient or r-square, are reported.
On the other hand, papers were excluded if they 1) were conference presentations, posters, or case studies; 2) included non-runners (e.g., athletes in basketball, and soccer); 3) did not provide data or inferred data on RE or biomechanical characteristics; and 4) did not report relevance.
After the full-text screening, a custom table was created to record basic information about the study (e.g., age, country, height, VO2max, and test speed). In addition, Pearson’s correlation coefficient (r) was extracted for the relevant stiffness parameter and energy expenditure. For studies reporting r-square, the square root of the coefficient of determination was taken by its directionality and was transformed into a correlation coefficient.
Quality evaluation
The methodological quality of each study was assessed using the Joanna Briggs Institute’s (JBI) Analytical Cross-Sectional Study Critical Assessment Tool (Moola et al., 2017). The checklist consists of ten items to evaluate the suitability of studies for inclusion in systematic reviews and meta-analyses. Two assessors assigned each study a score of 2 for “yes,” 0 for “no,” and 1 for “unclear.” Studies with a total score greater than 70% were considered to be of high quality.
Data analysis
A comprehensive meta-analysis version 3.0 (Englewood, NJ, United States) was used to analyze pooled Pearson’s correlation coefficients. A p-value of less than 0.05 was considered significantly different. The correlation coefficient was used to summarize results and report 95% confidence intervals. The heterogeneity of studies was assessed using the I2 statistic. Studies with I2 of less than 50% had poor heterogeneity, and a fixed-effects model was considered; otherwise, a random-effects model was used (Buccheri et al., 2018). According to Hopkins evaluation scheme, correlation size was judged as follows: <0.1 = trivial, 0.1–0.29 = small, 0.30–0.49 = moderate, 0.50–0.69 = large, 0.70–0.89 = very large, and ≥0.90 = extremely large (Hopkins et al., 2009). A simple meta-regression analysis was performed on the two independent variables, VO2max and test speed, to determine the variation of the moderating variables on the magnitude of the correlation. The correlation coefficients for each study were converted to Fisher’s Z-score as the dependent variable for the regression analysis. To assess the effect of individual studies on the pooled correlation coefficients and to test the robustness of the correlation between running economy and lower extremity stiffness, sensitivity analyses were performed by sequentially reducing one study per round. Publication bias was examined using funnel plots, and Duval and Tweedie’s trim and fill correction were used to address the impact of publication bias on the main meta-analysis.
Result
Study identification and characteristics
In total, 1,608 articles were obtained by searching three databases including PubMed, Web of Science, and Scopus. The Endnote software was used to remove duplicates, and 553 articles remained. In addition, papers with only titles and abstracts were filtered, and 27 articles with full texts remained. These articles were further screened. Out of the 27 articles, one longitudinal article included an intervention category, five did not include energy consumption or stiffness metrics, and eight did not include an available correlation coefficient. These articles were excluded. As a result, thirteen articles were included in our systematic review (Dalleau et al., 1998; Heise and Martin, 1998; Slawinski et al., 2008; Rabita et al., 2011; Barnes et al., 2014; Lazzer et al., 2014; Man et al., 2016; Rogers et al., 2017; Tam et al., 2018; Monte et al., 2020; Hansen et al., 2021; Li et al., 2021; Zhang et al., 2022), as shown in Figure 1.
In total, 272 runners were included in this study, 90.44% male and 9.56% female, with a mean age of 24.93 ± 6.48 years. The VO2max of the participants included in the study was 54.02–71.5 ml/kg/min. Ten studies reported correlations between vertical stiffness and RE, ten other studies reported correlations between leg stiffness and RE, and two studies reported correlations between knee stiffness and ankle stiffness and RE.
To acquire vertical, leg, and joint stiffness, the majority (n = 11) of the studies were conducted during running. Barnes and his colleagues used the peak force measured during five squat jumps divided by the vertical displacement as the “leg stiffness” (Barnes et al., 2014), which we summarized as the vertical stiffness. The approach used for measuring vertical stiffness was adopted by Rogers et al. (2017). In all studies, leg stiffness and joint stiffness were measured during running. Details of the included studies are shown in Table 1.
Quality evaluation
Table 2 shows the quality evaluation scores for each study (Table 2). The mean JBI quality rating score for the thirteen studies included in the analysis was 15.85 ± 0.86. The majority of these studies (n = 12) were of high quality. One study had a low rating because it lacked ethical consideration. A common low-scoring item in the entries was “How was the study population selected?” Given that the purpose of this review was to evaluate a simple correlation between lower extremity stiffness and RE, the sampling and selection of the study population did not affect the results of this study. In particular, most studies had clear objectives, valid and reliable data collection, and appropriate statistical methods. Therefore, no study was excluded because of methodological quality issues.
Pooled analyses
Ten studies reported Pearson correlation coefficients between vertical stiffness and RE. Heterogeneity between vertical stiffness and RE across studies was small (I2 = 20.62%). As a result, a meta-analysis was performed using a fixed effects model. The results revealed a large pooled correlation between vertical stiffness and RE (r = -0.519, 95% CI, −0.619 to −0.402, p < 0.001), as shown in Figure 2. Ten studies reported Pearson correlation coefficients between leg stiffness and RE. The test for heterogeneity between leg stiffness and RE was moderate (I2 = 67.93%). As a result, a random-effects model was adopted for meta-analysis. Pooled results showed a large pooled correlation between leg stiffness and RE (r = −0.568, p < 0.001, 95% CI, −0.723 to −0.357), as shown in Figure 3.
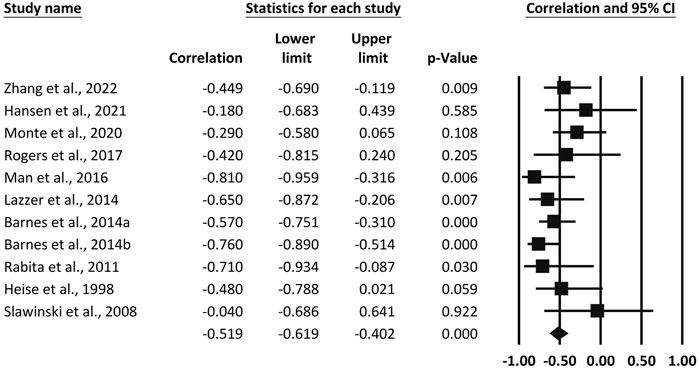
FIGURE 2. Forest plot of the correlation between vertical stiffness and running economy. The superscripted letter in column 1 refer to speed or gender items assessed in the same study.
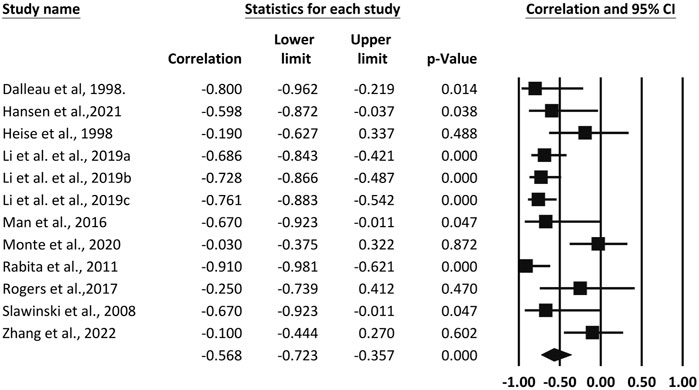
FIGURE 3. Forest plot of the correlation between leg stiffness and running economy. The superscripted letter in column 1 refer to speed or gender items assessed in the same study.
Two studies reported pooled results for knee stiffness and ankle stiffness. Pooled results with small heterogeneity showed a small negative correlation between knee stiffness and RE (r = −0.290, 95% CI, −0.508 to −0.037, p = 0.025). In contrast, ankle stiffness was not associated with RE (r = 0.0838, 95% CI, −0.716 to 0.788, p = 0.86), and interestingly, the two studies presented opposite results. As shown in Figure 4.
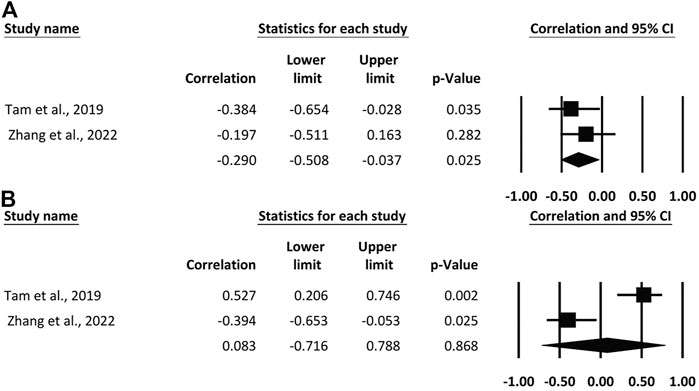
FIGURE 4. Forest plot of the correlation between knee (A) and ankle stiffness (B) and running economy.
Further one-way meta-regression analyses were performed for studies to provide clear reporting of test velocity and participant VO2max. The results showed that the correlation between vertical stiffness and RE was not affected by participant motion VO2max (coefficient = 0.003, p = 0.854) and test velocity (coefficient = 0.046, p = 0.751). However, the negative correlation between leg stiffness and RE increased with the VO2max (coefficient = −0.068, 95% CI, −0.114 to −0.022, p = 0.010, r2 = 0.92) test velocity (coefficient = −0.409, 95% CI, −0.730 to −0.089, p = 0.020, r2 = 0.79), as shown in Figure 5.
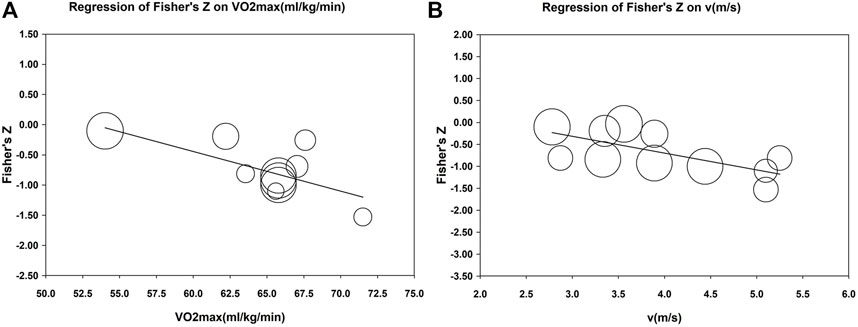
FIGURE 5. Meta-regression of VO2max (A) and test velocity (B) with correlation coefficients of leg stiffness and RE. The size of each circle is proportional to the study’s weight.
Sensitivity analyses and publication bias
We performed a sensitivity analysis with one-by-one exclusion and the estimated effects would still be within the 95% CI of the pooled results. Funnel plots of effect sizes versus standard errors were generated to determine possible publication bias. Analysis of the funnel plot revealed that the included studies were generally located on the left side of the pooled results. The funnel plot on the leg stiffness and RE correlations was adjusted using Duval and Tweedie’s trim and fill correction to produce a symmetric funnel plot around the Pearson correlation coefficient. This correction shifted the overall effect size to the right but did not change the main results, which still showed a significant trend, as shown in Figure 6.
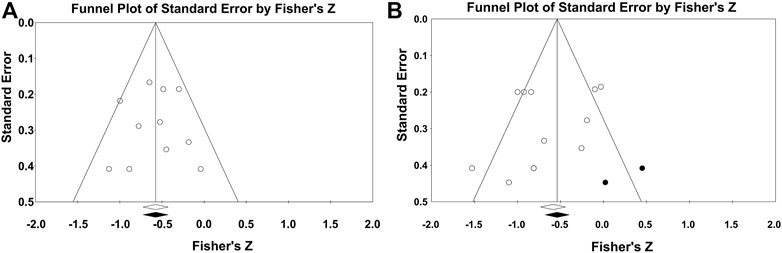
FIGURE 6. Vertical stiffness (A) and leg stiffness (B), funnel plot with running economy correlation.
Discussion
This study is the first to examine the relationship between lower extremity stiffness and RE systematically. The aim was to investigate whether lower extremity stiffness affects RE. In total, 13 articles were included in this review. The results showed a significant negative correlation between vertical, and knee stiffness and RE in endurance runners. This result suggests that vertical, leg, and knee stiffness influence RE in endurance runners. In addition, the results of the meta-regression showed that the correlation coefficient between leg stiffness and RE was related to the runner’s speed and VO2max.
Vertical stiffness and running economy
Current findings showed that vertical stiffness negatively correlated with RE during sub-maximal running. The following formula was used to calculate the vertical stiffness:
Where Fmax is the peak vertical force, and Δy is the vertical displacement of the COM from ground contact until mid-stance.
Many studies have demonstrated that increasing the peak vertical force or decreasing the COM vertical displacement during running will result in better vertical stiffness. Several studies have found that increasing running speed leads to a higher peak vertical force and a decrease in COM vertical displacement (Matt Brughelli and Cronin, 2008; Brughelli et al., 2011; Halvorsen et al., 2012). Dutto and Sam (2002) found that when the runner is exhausted, the change in vertical stiffness is primarily related to the maximum body displacement in the vertical direction during running rather than the change in peak vertical force. Other studies have also found that runners reduce energy expenditure by exhibiting less COM vertical displacement (Cavanagh et al., 1977; Anderson, 1996; Heise and Martin, 2001; Halvorsen et al., 2012). They observed that the peak vertical force decreases while the vertical stiffness increases significantly with fatigue (Morin et al., 2011; Rabita et al., 2011). This indicated that changing the COM vertical displacement affected the vertical stiffness more than changing the peak vertical force (Cavagna et al., 1988; Morin et al., 2007; Matt; Brughelli and Cronin, 2008). Meanwhile, a positive correlation was found between the vertical displacement of COM and RE (Halvorsen et al., 2012; Tartaruga et al., 2012; Folland et al., 2017). Given the absorption and release of elastic energy, greater vertical oscillation necessarily involves more work against gravity (Moore, 2016; Folland et al., 2017). The first study comparing the biomechanical characteristics of RE in runners at different levels showed that elite runners had fewer vertical oscillations, which were more symmetrical (Cavanagh et al., 1977). Farley et al. (1998) suggested that increasing vertical stiffness by reducing the COM displacement allows the spring-mass system to recoil in a shorter period, facilitating faster absorption and generation of kinetic energy during ground contact. In addition, greater vertical stiffness helped to resist flexion of the lower extremity joint during the support phase. It increased the rate of force generation during the centrifugal (centripetal) phase, which enhanced the storage and utilization of elastic energy during SSC (Matt Brughelli and Cronin, 2008).
The study found that maintaining a high whole-body vertical stiffness minimizes oxygen consumption, and smaller heterogeneity enhances the certainty of the results. Meta-regression results show that the correlation between vertical stiffness and RE is less susceptible to the effects of runner level and speed. Several studies have demonstrated that trained runners who run to exhaustion maintained initial vertical stiffness (Hunter and Smith, 2007; Morin et al., 2011) and even increased it significantly in 24-h ultra-long runs (Morin et al., 2011).
Leg stiffness and running economy
Our results found the same negative correlation between leg stiffness and RE during the submaximal running, suggesting that leg stiffness is an essential factor influencing RE. Leg stiffness is an essential parameter in regulating running mechanics, which can maintain a stable running gait in humans and animals (Seyfarth et al., 2002). The following equation can calculate the leg stiffness:
where v is running velocity and tc is contact time. Leg length is symbolized as L, which comes into contact with the ground at an angle of θ (McMahon and Cheng, 1990).
Several studies support the result of a significant negative correlation in the random effects of this study. Scholars began to focus on the possibility that RE may be related to the time course of supporting body weight, for example, ground contact time (tc). Kram et al. (1990) reported that the cost of supporting the animal’s weight and the time course of generating this force determines the cost of running. Because the longer tc allows the lower-extremities to generate propulsive force over a longer period of time when in contact with the ground, reducing energy costs (Kram and Taylor, 1990). Notably, many studies on human running showed a significant positive correlation between tc and RE (Nummela et al., 2007; Barnes et al., 2014; Di Michele and Merni, 2014; Mooses et al., 2021). This was likely due to less time required for braking to decelerate the body’s forward motion (Nummela et al., 2007; Kong and de Heer, 2008; Mooses et al., 2021). Meanwhile, a negative correlation between leg stiffness and tc was demonstrated in other studies (Morin et al., 2007; Hayes and Caplan, 2014; Santos-Concejero et al., 2014; Man et al., 2016). Morin et al. (2007) found that the change in tc can account for 90% of the change in leg stiffness. This means that reducing tc increases leg stiffness (Man et al., 2016). This may be due to the high preactivation of the calf muscles which then increases the sensitivity of muscle spindle potentiating stretch reflexes to enhance musculo-tendon stiffness and to improve the RE (Santos-Concejero et al., 2014; Mooses et al., 2021). Moreover, Moore et al. (2019) showed that the relationship between leg stiffness and RE is not linear and that there is an identifiable optimum for it, as 90% of runners can keep their optimal metabolic cost within 5% at a self-selected leg stiffness (Moore et al., 2019). Unfortunately, the optimal range of leg stiffness values for endurance runners is still unknown.
The results of the meta-regression explained the high heterogeneity of the correlation between leg stiffness and RE. The results showed that the correlation between leg stiffness and RE increased with the speed and runner VO2max. Previous studies showed that novice runners have higher oxygen consumption than trained runners, while tc is longer (Williams and Cavanagh, 1987; de Ruiter et al., 2014). Bitchell et al. (2019) state that although leg stiffness was unaffected by physiological training status, untrained runners had difficulty maintaining consistent leg stiffness during running. This variability is likely related to increased oxygen costs. Experienced and well-trained runners optimize elastic energy storage and release more rationally to minimize metabolic costs (Lussiana et al., 2019; Moore et al., 2019).
There has been no consensus whether speed affects leg stiffness in previous studies (Matt Brughelli and Cronin, 2008). Our meta-regression results confirmed that speed enhanced the correlation between leg stiffness and RE. The greater the speed, the more pronounced the favorable effect of leg stiffness on RE. Lai et al. (2014) reported that the energy stored in the elastic deformation of the tendon is better utilized at higher speeds and that the positive work done by the elastic strain energy on the tendon-muscle unit is lower at slower running speeds than at faster running speeds. The results of this study validate previous conjectures that there is indeed a “U” shaped relationship between leg stiffness and RE and that training level and running speed will determine whether a runner’s energy cost is near the bottom of the curve (Zhang et al., 2022).
Joint stiffness and running economy
The torsional spring model can be utilized to estimate the joint stiffness values of the main joints of the lower extremity during vertical and horizontal movements (Latash and Zatsiorsky, 1993). The following equation can calculate joint stiffness:
where M denotes the deformation torque and Δα is the deformation angle.
Measurement of joint stiffness, particularly of the ankle and knee joints, provides greater insight into the respective contributions of the joints to the overall stiffness of the lower extremity (Maloney and Fletcher, 2021). The combination of knee and ankle stiffness provides the best correlation for leg stiffness when exploring the variation in leg stiffness (Lorimer et al., 2018). Unfortunately, there is only two evidences supporting the relationship between joint stiffness and RE. Tam et al. (2019) found that high knee and less ankle stiffness were associated with better RE. The results of the meta-analysis showed a negative correlation between knee stiffness and RE, though it was not significant.
Joint stiffness mainly depends on the level of activation of the muscles around the joint (Farley et al., 1998). The most economical runners rely on greater muscle activation (Tam et al., 2019). Kyröläinen et al. (2001) found that muscle co-activation around the knee and ankle joints during running increases joint stiffness, which was associated with better RE. Tam et al. (2017) also showed that during the pre-activation and ground contact, rectus femoris (RD):biceps femoris (BF) co activation ratio was positively correlated with the knee, but not the ankle joint. Pre-emptive neuromuscular joint control decreases the need for corrective muscle activation at and after ground contact, where loading forces are applied to joints stiffness (Tam et al., 2019). This suggested that greater knee stiffness allowed more energy to be stored in the leg spring through co-activation of the agonist and antagonist muscles and provided coordination of the ankle and hip joints (Jin and Hahn, 2018).
Unlike walking, running requires more bracing phase energy generation (Jin and Hahn, 2018). Meanwhile, the ankle joint does more positive work in the support phase than the knee (Jin and Hahn, 2018). During landing, the knee and hip joints absorb more of the impact, which requires higher ankle stiffness and the joint muscle-tendon pull reflex, resulting in more energy demand (Jin and Hahn, 2018). Previous studies have shown that increased gastrocnemius-anterior tibialis activation is associated with high ankle stabilization stability and energy sparing (Kyröläinen et al., 2001; Fletcher and MacIntosh, 2017; Tam et al., 2017; Tam et al., 2019).
The reason for the opposing results in the two studies may stem from differences in landing patterns and running speeds (Tam et al., 2018; Zhang et al., 2022). The forefoot landing ankle stiffness used by the elite runners was lower and performed more negative work (Hamill and Gruber, 2017). This suggests that a high supple joint stiffness absorbs the energy of landing impact to a greater extent. The hindfoot landing has high ankle stiffness and performs less negative function (Hamill and Gruber, 2017). However, as speed decreases and running distance increases, some runners tend to land on their hind feet (Hasegawa et al., 2007). Additionally, Weir et al. (2020) found that habitual hindfoot runners showed a decreased ankle stiffness as the running time increased. In conclusion, the current limited evidence makes the results uncertain and future studies are necessary to address the comparison of ankle stiffness contributions at different running patterns and speeds.
Since the phase shift for the moment-displacement curve of the hip commonly exceeds 10% (Farley and Morgenroth, 1999; Kuitunen et al., 2011; Maloney and Fletcher, 2021), it is often excluded from the comparison. As a result, many studies about joint stiffness have only focused on the knee and ankle joints, and little attention has been paid to hip stiffness. Jin and Hahn (2018) observed that the hip joint plays an important role in energy absorption during the swing phase of running. However, the relationship between hip stiffness and endurance in sports performance is unknown.
Limitations and prospects
Several limitations were identified in this study. First, the number of studies included in this study was too small, and the Pearson correlation coefficient still has some limitations in expressing the relationship between lower extremity stiffness and RE. Second, the vast majority of subjects in the study were male, with only 11% of females participating. In one of the articles used in this study, women showed a greater correlation than men. Although RE may not differ by gender (Besson et al., 2022), there are differences in the biomechanics of running (Barnes et al., 2014; Besson et al., 2022). For example, women have faster stride length, shorter tc, and faster time to peak (Nelson et al., 1977; Williams et al., 1987; Besson et al., 2022). As a result, women have higher leg stiffness than men (Barnes et al., 2014). Besson et al. (2022) stated that male and female runners optimize their running patterns to suit their gender characteristics. In addition, women may have physiological and technical advantages in ultra-endurance events (Tiller et al., 2021). As a result, future researchers need to pay more attention to the relationship between lower extremity biomechanics and energy in runners of different genders.
The above conclusion are based on SMM. This simple model can reproduce the motion and forces of running remarkably well. Schroeder and Kuo (2021) proposed a running model based on SMM that combines elasticity with active actuation and passive dissipation (Actuated Spring-mass model). This model included two types of dissipation, one dissipating for collision and hysteresis losses, and the other including hysteresis to simulate imperfect energy return of tendons and other series elastic tissues (Schroeder and Kuo, 2021). However, the model is not perfect either, for example, it neglects a swing leg, whose active motion may also cost energy (Doke et al., 2005). In the future, more sophisticated models should be adopted to simulate the energy expenditure during running.
Finally, we discussed the relationship between each of the three stiffnesses and RE. A negative correlation was obtained for all except ankle stiffness. However, the consistency results may lead to the mistaken belief that they are independent and interchangeable. They act together on the body in motion and support the body mass. There is a close link between vertical, leg and joint stiffness, for example, the stiffness of the leg spring is influenced by the stiffness of the three joints (hip, knee, and ankle) (Struzik et al., 2021). Therefore, in the future, it is necessary to continue to explore the potential relationships between vertical, leg, and joint.
Conclusion
This meta-analysis found a significant negative association of vertical, leg, and knee stiffness with RE. Furthermore, the extent of this correlation was related to speed and the runner’s VO2max. This suggests that experienced runners make more rational use of elastic energy to minimize energy costs at faster speeds. Some scholars have suggested that there is a weak negative correlation between knee stiffness and oxygen consumption, but it is not clear how much ankle stiffness runners need. Many factors affect stiffness during running, and these factors are influenced by different tasks, populations, and gender. The optimal stiffness required for a runner needs to be further investigated. The results of this study may, to some extent, inform future training and research.
Data availability statement
The original contributions presented in the study are included in the article/Supplementary Material, further inquiries can be directed to the corresponding author.
Author contributions
BL and ZR designed the research. BL and WX conducted the searches and screening process. BL and WX completed the full-text screening. BL, WX, JY, and FH assessed methodological quality. BL, FY, and FH extracted the data, which were checked by JW. BL performed the statistical analysis and interpreted it. BL wrote the manuscript with critical input from ZR. ZR and QS read and approved the final manuscript.
Funding
The article was supported by National Natural Science Foundation of China (NSFC) (grant number 11002036); Research Foundation for Young Teacher of Shenzhen University (grant number QNJS0274); High-level Scientific Research Foundation for the Introduction of Talent of Shenzhen University (grant number RC00228); and Natural Science Featured Innovation Projects in Ordinary Universities in Guangdong Province (grant number 2021KTSCX297); Scientific Research Platform and Project of Colleges and Universities of Education Department of Guangdong Province (2022ZDZX 2087).
Conflict of interest
The authors declare that the research was conducted in the absence of any commercial or financial relationships that could be construed as a potential conflict of interest.
Publisher’s note
All claims expressed in this article are solely those of the authors and do not necessarily represent those of their affiliated organizations, or those of the publisher, the editors and the reviewers. Any product that may be evaluated in this article, or claim that may be made by its manufacturer, is not guaranteed or endorsed by the publisher.
References
Anderson T. (1996). Biomechanics and running economy. Sports Med. 22 (2), 76–89. doi:10.2165/00007256-199622020-00003
Barnes K. R., Mcguigan M. R., Kilding A. E. (2014). Lower-body determinants of running economy in male and female distance runners. J. Strength Cond. Res. 28 (5), 1289–1297. doi:10.1519/JSC.0000000000000267
Beerse M., Wu J. (2017). Comparison of whole-body vertical stiffness and leg stiffness during single-leg hopping in place in children and adults. J. Biomech. 56, 71–75. doi:10.1016/j.jbiomech.2017.03.001
Besson T., Macchi R., Rossi J., Morio C. Y. M., Kunimasa Y., Nicol C., et al. (2022). Sex differences in endurance running. Sports Med. 52 (6), 1235–1257. doi:10.1007/s40279-022-01651-w
Bitchell C. L., McCarthy-Ryan M., Goom T., Moore I. S. (2019). Spring-mass characteristics during human locomotion: Running experience and physiological considerations of blood lactate accumulation. Eur. J. Sport Sci. 19 (10), 1328–1335. doi:10.1080/17461391.2019.1609095
Blickhan R. (1989). The spring-mass model for running and hopping. J. Biomech. 22 (11–12), 1217–1227. doi:10.1016/0021-9290(89)90224-8
Brughelli M, Cronin J. (2008). Influence of running velocity on vertical, leg and joint stiffness : Modelling and recommendations for future research. Sports Med. 38 (8), 647–657. doi:10.2165/00007256-200838080-00003
Brughelli M., Cronin J. (2008). A review of research on the mechanical stiffness in running and jumping: Methodology and implications. Scand. J. Med. Sci. Sports 18 (4), 417–426. doi:10.1111/j.1600-0838.2008.00769.x
Brughelli M., Cronin J., Chaouachi A. (2011). Effects of running velocity on running kinetics and kinematics. J. Strength Cond. Res. 25 (4), 933–939. doi:10.1519/JSC.0b013e3181c64308
Buccheri S., Sodeck G. H., Capodanno D. (2018). Statistical primer: Methodology and reporting of meta-analyses. Eur. J. Cardiothorac. Surg. 53 (4), 708–713. doi:10.1093/ejcts/ezy004
Butler R. J., Crowell H. P., Davis I. M. (2003). Lower extremity stiffness: Implications for performance and injury. Clin. Biomech. 18 (6), 511–517. doi:10.1016/s0268-0033(03)00071-8
Cavagna G. A., Franzetti P., Heglund N. C., Willems P. (1988). The determinants of the step frequency in running, trotting and hopping in man and other vertebrates. J. Physiol. 399 (1), 81–92. doi:10.1113/jphysiol.1988.sp017069
Cavanagh P. R., Pollock M. L., Landa J. (1977). A biomechanical comparison of elite and good distance runners. Ann. N. Y. Acad. Sci. 301 (1), 328–345. doi:10.1111/j.1749-6632.1977.tb38211.x
Conley D. L., Krahenbuhl G. S. (1980). Running economy and distance running performance of highly trained athletes. Med. Sci. Sports Exerc. 12 (5), 357–360. doi:10.1249/00005768-198025000-00010
Dalleau G., Belli A., Bourdin M., Lacour J. R. (1998). The spring-mass model and the energy cost of treadmill running. Eur. J. Appl. Physiol. Occup. Physiol. 77 (3), 257–263. doi:10.1007/s004210050330
de Ruiter C. J., Verdijk P. W. L., Werker W., Zuidema M. J., de Haan A. (2014). Stride frequency in relation to oxygen consumption in experienced and novice runners. Eur. J. Sport Sci. 14 (3), 251–258. doi:10.1080/17461391.2013.783627
Di Michele R., Merni F. (2014). The concurrent effects of strike pattern and ground-contact time on running economy. J. Sci. Med. Sport 17 (4), 414–418. doi:10.1016/j.jsams.2013.05.012
di Prampero P. E., Atchou G., Brückner J.-C., Moia C. (1986). The energetics of endurance running. Eur. J. Appl. Physiol. Occup. Physiol. 55 (3), 259–266. doi:10.1007/BF02343797
Doke J., Donelan J. M., Kuo A. D. (2005). Mechanics and energetics of swinging the human leg. J. Exp. Biol. 208 (3), 439–445. doi:10.1242/jeb.01408
Dutto D. J., Smith G. A. (2002). Changes in spring-mass characteristics during treadmill running to exhaustion. Med. Sci. Sports Exerc. 34 (8), 1324–1331. doi:10.1097/00005768-200208000-00014
Farley C. T., González O. (1996). Leg stiffness and stride frequency in human running. J. Biomech. 29 (2), 181–186. doi:10.1016/0021-9290(95)00029-1
Farley C. T., Houdijk H. H. P., Van Strien C., Louie M. (1998). Mechanism of leg stiffness adjustment for hopping on surfaces of different stiffnesses. J. Appl. Physiol. 85 (3), 1044–1055. doi:10.1152/jappl.1998.85.3.1044
Farley C. T., Morgenroth D. C. (1999). Leg stiffness primarily depends on ankle stiffness during human hopping. J. Biomech. 32 (3), 267–273. doi:10.1016/S0021-9290(98)00170-5
Fletcher J. R., MacIntosh B. R. (2017). Running economy from a muscle energetics perspective. Front. Physiol. 8, 433. doi:10.3389/fphys.2017.00433
Folland J. P., Allen S. J., Black M. I., Handsaker J. C., Forrester S. E. (2017). Running technique is an important component of running economy and performance. Med. Sci. Sports Exerc. 49 (7), 1412–1423. doi:10.1249/MSS.0000000000001245
Halvorsen K., Eriksson M., Gullstrand L. (2012). Acute effects of reducing vertical displacement and step frequency on running economy. J. Strength Cond. Res. 26 (8), 2065–2070. doi:10.1519/JSC.0b013e318239f87f
Hamill J., Gruber A. H. (2017). Is changing footstrike pattern beneficial to runners? J. Sport Health Sci. 6 (2), 146–153. doi:10.1016/j.jshs.2017.02.004
Hansen C. E., Stensvig M., Wienecke J., Villa C., Lorentzen J., Rasmussen J., et al. (2021). Factors correlated with running economy among elite middle- and long-distance runners. Physiol. Rep. 9 (20), e15076. doi:10.14814/phy2.15076
Hasegawa H., Yamauchi T., Kraemer W. J. (2007). Foot strike patterns of runners at the 15-km point during an elite-level half marathon. J. Strength Cond. Res. 21 (3), 888–893. doi:10.1519/R-22096.1
Hayes P. R., Caplan N. (2014). Leg stiffness decreases during a run to exhaustion at the speed at VO2max. Eur. J. Sport Sci. 14 (6), 556–562. doi:10.1080/17461391.2013.876102
Heise G. D., Martin P. E. (2001). Are variations in running economy in humans associated with ground reaction force characteristics? Eur. J. Appl. Physiol. 84 (5), 438–442. doi:10.1007/s004210100394
Heise G. D., Martin P. E. (1998). Leg spring” characteristics and the aerobic demand of running. Med. Sci. Sports Exerc. 30 (5), 750–754. doi:10.1097/00005768-199805000-00017
Hopkins W. G., Marshall S. W., Batterham A. M., Hanin J. (2009). Progressive statistics for studies in sports medicine and exercise science. Med. Sci. Sports Exerc. 41 (1), 3–13. doi:10.1249/MSS.0b013e31818cb278
Hunter I., Smith G. A. (2007). Preferred and optimal stride frequency, stiffness and economy: Changes with fatigue during a 1-h high-intensity run. Eur. J. Appl. Physiol. 100 (6), 653–661. doi:10.1007/s00421-007-0456-1
Jin L., Hahn M. E. (2018). Modulation of lower extremity joint stiffness, work and power at different walking and running speeds. Hum. Mov. Sci. 58, 1–9. doi:10.1016/j.humov.2018.01.004
Joyner M. J. Modeling: Optimal marathon performance on the basis of physiological factors. J. Appl. Physiol(Bethesda, Md. (1991)., 70(2), 683–687. doi:10.1152/jappl.1991.70.2.683
Kerdok A. E., Biewener A. A., McMahon T. A., Weyand P. G., Herr H. M. (2002). Energetics and mechanics of human running on surfaces of different stiffnesses. J. Appl. Physiol. 92 (2), 469–478. doi:10.1152/japplphysiol.01164.2000
Khammassi M., Ouerghi N., Said M., Feki M., Khammassi Y., Pereira B., et al. (2020). Continuous moderate-intensity but not high-intensity interval training improves immune function biomarkers in healthy young men. J. Stren. Condition. Res. 34 (1), 249–256. doi:10.1519/JSC.0000000000002737
Kong P. W., de Heer H. (2008). Anthropometric, gait and strength characteristics of Kenyan distance runners. J. Sports Sci. Med. 7 (4), 499–504.
Kram R., Taylor C. R. (1990). Energetics of running: A new perspective. Nature 346 (6281), 265–267. doi:10.1038/346265a0
Kuitunen S., Ogiso K., Komi P. V. (2011). Leg and joint stiffness in human hopping. Scand. J. Med. Sci. Sports 21 (6), e159–e167. doi:10.1111/j.1600-0838.2010.01202.x
Kyröläinen H., Belli A., Komi P. V. (2001). Biomechanical factors affecting running economy. Med. Sci. Sports Exerc. 33 (8), 1330–1337. doi:10.1097/00005768-200108000-00014
Lai A., Schache A. G., Lin Y.-C., Pandy M. G. (2014). Tendon elastic strain energy in the human ankle plantar-flexors and its role with increased running speed. J. Exp. Biol. 217 (17), 3159–3168. doi:10.1242/jeb.100826
Latash M. L., Zatsiorsky V. M. (1993). Joint stiffness: Myth or reality? Hum. Mov. Sci. 12 (6), 653–692. doi:10.1016/0167-9457(93)90010-M
Lazzer S., Taboga P., Salvadego D., Rejc E., Simunic B., Narici M. V., et al. (2014). Factors affecting metabolic cost of transport during a multi-stage running race. J. Exp. Biol. 217 (5), 787–795. doi:10.1242/jeb.091645
Li F., Newton R. U., Shi Y., Sutton D., Ding H. (2021). Correlation of eccentric strength, reactive strength, and leg stiffness with running economy in well-trained distance runners. J. Strength Cond. Res. 35 (6), 1491–1499. doi:10.1519/JSC.0000000000003446
Lorimer A. V., Keogh J. W. L., Hume P. A. (2018). Using stiffness to assess injury risk: Comparison of methods for quantifying stiffness and their reliability in triathletes. PeerJ 6, e5845. doi:10.7717/peerj.5845
Lussiana T., Patoz A., Gindre C., Mourot L., Hébert-Losier K. (2019). The implications of time on the ground on running economy: Less is not always better. J. Exp. Biol. 222 (6), jeb192047. doi:10.1242/jeb.192047
Maloney S. J., Fletcher I. M. (2021). Lower limb stiffness testing in athletic performance: A critical review. Sports Biomech. 20 (1), 109–130. doi:10.1080/14763141.2018.1460395
Man H. S., Lam W. K., Lee J., Capio C. M., Leung A. K. L. (2016). Is passive metatarsophalangeal joint stiffness related to leg stiffness, vertical stiffness and running economy during sub-maximal running? Gait Posture 49, 303–308. doi:10.1016/j.gaitpost.2016.07.004
McMahon T. A., Cheng G. C. (1990). The mechanics of running: How does stiffness couple with speed? J. Biomech. 23, 65–78. doi:10.1016/0021-9290(90)90042-2
Monte A., Nardello F., Pavei G., Moro S., Festa L., Tarperi C., et al. (2020). Mechanical determinants of the energy cost of running at the half-marathon pace. J. Sports Med. Phys. Fit. 60 (2), 198–205. doi:10.23736/S0022-4707.19.09999-7
Moola S., Munn Z., Tufanaru C., Aromataris E., Sears K., Sfetcu R., et al. (2017). “Chapter 7: Systematic reviews of etiology and risk,” in Joanna briggs institute reviewer’s manual. South Australia: The Joanna Briggs Institute, 5.
Moore I. S., Ashford K. J., Cross C., Hope J., Jones H. S. R., McCarthy-Ryan M. (2019). Humans optimize ground contact time and leg stiffness to minimize the metabolic cost of running. Front. Sports Act. Living 1, 53. doi:10.3389/fspor.2019.00053
Moore I. S. (2016). Is there an economical running technique? A review of modifiable biomechanical factors affecting running economy. Sports Med. 46 (6), 793–807. doi:10.1007/s40279-016-0474-4
Mooses M., Haile D. W., Ojiambo R., Sang M., Mooses K., Lane A. R., et al. (2021). Shorter ground contact time and better running economy: Evidence from female Kenyan runners. J. Strength Cond. Res. 35 (2), 481–486. doi:10.1519/JSC.0000000000002669
Morgan D. W., Craib M. (1992). Physiological aspects of running economy. Med. Sci. Sports Exerc. 24 (4), 456–461. doi:10.1249/00005768-199204000-00011
Morin J.-B., Dalleau G., Kyröläinen H., Jeannin T., Belli A. (2005). A simple method for measuring stiffness during running. J. Appl. Biomech. 21 (2), 167–180. doi:10.1123/jab.21.2.167
Morin J.-B., Samozino P., Millet G. Y. (2011). Changes in running kinematics, kinetics, and spring-mass behavior over a 24-h run. Med. Sci. Sports Exerc. 43 (5), 829–836. doi:10.1249/MSS.0b013e3181fec518
Morin J. B., Samozino P., Zameziati K., Belli A. (2007). Effects of altered stride frequency and contact time on leg-spring behavior in human running. J. Biomech. 40 (15), 3341–3348. doi:10.1016/j.jbiomech.2007.05.001
Nelson R. C., Brooks C. M., Pike N. L. (1977). Biomechanical comparison of male and female distance runners. Ann. N. Y. Acad. Sci. 301 (1), 793–807. doi:10.1111/j.1749-6632.1977.tb38247.x
Nummela A., Keränen T., Mikkelsson L. (2007). Factors related to top running speed and economy. Int. J. Sports Med. 28 (8), 655–661. doi:10.1055/s-2007-964896
Rabita G., Slawinski J., Girard O., Bignet F., Hausswirth C. (2011). Spring-mass behavior during exhaustive run at constant velocity in elite triathletes. Med. Sci. Sports Exerc. 43 (4), 685–692. doi:10.1249/MSS.0b013e3181fb3793
Rodrigo-Carranza V., González-Mohíno F., Santos-Concejero J., González-Ravé J. M. (2021). The effects of footwear midsole longitudinal bending stiffness on running economy and ground contact biomechanics: A systematic review and meta-analysis. Eur. J. Sport Sci. 0 (0), 1508–1521. doi:10.1080/17461391.2021.1955014
Rogers S. A., Whatman C. S., Pearson S. N., Kilding A. E. (2017). Assessments of mechanical stiffness and relationships to performance determinants in middle-distance runners. Int. J. Sports Physiol. Perform. 12 (10), 1329–1334. doi:10.1123/ijspp.2016-0594
Santos-Concejero J., Tam N., Granados C., Irazusta J., Bidaurrazaga-Letona I., Zabala-Lili J., et al. (2014). Stride angle as a novel indicator of running economy in well-trained runners. J. Strength Cond. Res. 28 (7), 1889–1895. doi:10.1519/JSC.0000000000000325
Schroeder R. T., Kuo A. D. (2021). Elastic energy savings and active energy cost in a simple model of running. PLoS Comput. Biol. 17 (11), e1009608. doi:10.1371/journal.pcbi.1009608
Seyfarth A., Geyer H., Günther M., Blickhan R. (2002). A movement criterion for running. J. Biomech. 35 (5), 649–655. doi:10.1016/s0021-9290(01)00245-7
Slawinski J., Heubert R., Quievre J., Billat V., Hanon C., Hannon C. (2008). Changes in spring-mass model parameters and energy cost during track running to exhaustion. J. Strength Cond. Res. 22 (3), 930–936. doi:10.1519/JSC.0b013e31816a4475
Statista (2018). Running/jogging participants US 2006-2017, Statista. https://www.statista.com/statistics/190303/running-participants-in-the-us-since-2006/ (Accessed September 9, 2022).
Struzik A., Karamanidis K., Lorimer A., Keogh J. W. L., Gajewski J. (2021). Application of leg, vertical, and joint stiffness in running performance: A literature overview. Appl. Bionics Biomech. 2021, 1–25. doi:10.1155/2021/9914278
Tam N., Santos-Concejero J., Coetzee D. R., Noakes T. D., Tucker R. (2017). Muscle co-activation and its influence on running performance and risk of injury in elite Kenyan runners. J. Sports Sci. 35 (2), 175–181. doi:10.1080/02640414.2016.1159717
Tam N., Tucker R., Santos-Concejero J., Prins D., Lamberts R. P. (2018). Running economy: Neuromuscular and joint stiffness contributions in trained runners. Int. J. Sports Physiol. Perform. 14, 16–22. doi:10.1123/ijspp.2018-0151
Tam N., Tucker R., Santos-Concejero J., Prins D., Lamberts R. P. (2019). Running economy: Neuromuscular and joint-stiffness contributions in trained runners. Int. J. Sports Physiol. Perform. 14 (1), 16–22. doi:10.1123/ijspp.2018-0151
Tartaruga M. P., Brisswalter J., Peyré-Tartaruga L. A., Ávila A. O. V., Alberton C. L., Coertjens M., et al. (2012). The relationship between running economy and biomechanical variables in distance runners. Res. Q. Exerc. Sport 83 (3), 367–375. doi:10.1080/02701367.2012.10599870
Tiller N. B., Elliott-Sale K. J., Knechtle B., Wilson P. B., Roberts J. D., Millet G. Y. (2021). Do sex differences in Physiology confer a female advantage in ultra-endurance sport? Sports Med. 51 (5), 895–915. doi:10.1007/s40279-020-01417-2
Weir G., Willwacher S., Trudeau M. B., Wyatt H., Hamill J. (2020). The influence of prolonged running and footwear on lower extremity joint stiffness. Med. Sci. Sports Exerc. 52 (12), 2608–2614. doi:10.1249/MSS.0000000000002416
Williams K., Cavanagh P., Ziff J. (1987). Biomechanical studies of elite female distance runners. Int. J. Sports Med. 08 (2), S107–S118. doi:10.1055/s-2008-1025715
Williams K. R., Cavanagh P. R. (1987). Relationship between distance running mechanics, running economy, and performance. J. Appl. Physiol. 63 (3), 1236–1245. doi:10.1152/jappl.1987.63.3.1236
Keywords: spring, vertical stiffness, leg stiffness, joint stiffness, running economy
Citation: Liu B, Wu J, Shi Q, Hao F, Xiao W, Yu J, Yu F and Ren Z (2022) Running economy and lower extremity stiffness in endurance runners: A systematic review and meta-analysis. Front. Physiol. 13:1059221. doi: 10.3389/fphys.2022.1059221
Received: 01 October 2022; Accepted: 15 November 2022;
Published: 28 November 2022.
Edited by:
Lin Wang, Shanghai University of Sport, ChinaReviewed by:
Jianhua Wu, Georgia State University, United StatesLiang Guo, South China Normal University, China
Copyright © 2022 Liu, Wu, Shi, Hao, Xiao, Yu, Yu and Ren. This is an open-access article distributed under the terms of the Creative Commons Attribution License (CC BY). The use, distribution or reproduction in other forums is permitted, provided the original author(s) and the copyright owner(s) are credited and that the original publication in this journal is cited, in accordance with accepted academic practice. No use, distribution or reproduction is permitted which does not comply with these terms.
*Correspondence: Zhanbing Ren, cnpiQHN6dS5lZHUuY24=