- 1Department of Sport and Sport Science, University of Freiburg, Freiburg, Germany
- 2Praxisklinik Rennbahn, Muttenz, Switzerland
- 3Australasian Kidney Trials Network, Centre for Health Services Research, Faculty of Medicine, University of Queensland, Brisbane, QLD, Australia
- 4CRI, Collagen Research Institute, Kiel, Germany
- 5Medical Faculty Mannheim, University of Heidelberg, Heidelberg, Germany
- 6Department of Sports Science and Clinical Biomechanics, Research Unit for Muscle Physiology and Biomechanics, University of Southern Denmark, Odense, Denmark
- 7Geriatric Research Unit, Department of Geriatric and Palliative Medicine, Copenhagen University Hospital Bispebjerg and Frederiksberg, Copenhagen, Denmark
- 8Geriatric Research Unit, Department of Medicine, Copenhagen University Hospital Herlev and Gentofte, Copenhagen, Denmark
- 9Department of Nutritional Science, Institute for Nutrition, Exercise and Health, University of Vienna, Vienna, Austria
- 10Centre for Sports Science and University Sports, Institute for Nutrition, Exercise and Health, University of Vienna, Vienna, Austria
Previous evidence suggests that resistance training in combination with specific collagen peptides (CP) improves adaptive responses of the muscular apparatus. Although beneficial effects have been repeatedly demonstrated, the underlying mechanisms are not well understood. Therefore, the primary objective of the present randomized trial was to elucidate differences in gene expression pathways related to skeletal muscle signal transduction following acute high-load resistance exercise with and without CP intake. Recreationally active male participants were equally randomized to high-load leg extension exercise in combination with 15 g CP or placebo (PLA) supplementation. Muscle biopsies from the vastus lateralis muscle were obtained at baseline as well as 1, 4 and 24 h post exercise to investigate gene expression using next generation sequencing analysis. Several important anabolic pathways including PI3K-Akt and MAPK pathways were significantly upregulated at 1 and 4 h post-exercise. Significant between-group differences for both pathways were identified at the 4 h time point demonstrating a more pronounced effect after CP intake. Gene expression related to the mTOR pathway demonstrated a higher visual increase in the CP group compared to PLA by trend, but failed to achieve statistically significant group differences. The current findings revealed a significantly higher upregulation of key anabolic pathways (PI3K-Akt, MAPK) in human skeletal muscle 4 h following an acute resistance training combined with intake of 15 g of specific collagen peptides compared to placebo. Further investigations should examine potential relationships between upregulated gene expression and changes in myofibrillar protein synthesis as well as potential long-term effects on anabolic pathways on the protein level.
Introduction
Human skeletal muscle mass accounts for up to 35% of total body weight (Janssen et al., 2000) and serves vital functions such as generating force and thus enabling locomotion. Additionally, skeletal muscle metabolism comprises numerous metabolic processes including energy storage (Hultman et al., 1971), energy consumption and endocrine functioning (Hoffmann and Weigert, 2017). Thus, the preservation or even increase of skeletal muscle mass and strength are of fundamental importance to sustain and improve many physiological functions including a healthy aging process with the longest possible maintenance of activities of daily living (Wang et al., 2020). Resistance exercise in combination with protein ingestion is known to be a potent stimulator of skeletal muscle protein synthesis and the timely coordinated combination of both has been demonstrated to augment net muscle protein synthesis (Burd et al., 2009; Wirth et al., 2020).
Previous research indicated that the beneficial effects of protein supplementation (in particular whey protein (Moore et al., 2011; Areta et al., 2013; Morton et al., 2018; Hamarsland et al., 2019)) on muscle protein synthesis are predominantly mediated by the essential amino acid leucine, which augments intramuscular muscle protein synthesis via the mechanistic target of rapamycin (mTOR) complex and its downstream regulators (Drummond and Rasmussen, 2008; Moberg et al., 2016). Additionally, via exercise-induced growth factor secretion, the stimulation of the PI3k-Akt pathway has repeatedly been shown to induce protein synthesis via activation of its downstream targets (Bodine et al., 2001). Independently of mTOR activity, resistance exercise can also activate the mitogen-activated protein kinase (MAPK) pathway. Previous researchers (Moore et al., 2011) speculated that MAPK might facilitate the stimulation of myofibrillar protein synthesis by regulating proteins which mediate the initiation and elongation stages of mRNA translation (Wang et al., 2001; Roux et al., 2007).
Based on clinical and preclinical studies on the acute and longitudinal muscular responses to resistance exercise and protein consumption, whey protein (Moore et al., 2011; Areta et al., 2013; Hamarsland et al., 2019), soy (Darren et al., 2006; Tang et al., 2009), casein (Tipton et al., 2004; Tang et al., 2009) or collagen peptides (Jendricke et al., 2019; Kirmse et al., 2019; Zdzieblik et al., 2021) have been receiving increased attention over the past 2 decades.
Collagen peptides (CP) in combination with resistance training have previously been shown to improve muscle function and increase fat-free mass beyond the levels of resistance training alone (Zdzieblik et al., 2015; Jendricke et al., 2019; Kirmse et al., 2019), although some studies found less pronounced effects on muscle protein synthesis (Oikawa et al., 2020) and muscle mass compared to whey protein (Jacinto et al., 2022). In fact, however, Jacinto et al. (2022) did not find significant group differences with regard to performance parameters, which is practically relevant for the effectiveness of training. To date, little is known about the underlying mechanisms on a cellular level responsible for these observations. While CPs are rich in amino acids such as proline, glycine and arginine (Zdzieblik et al., 2015), the amount of e.g., leucine is low, which might lead to the conclusion about limited anabolic potential. Yet, in vitro data by Kitakaze et al. (2016) have indicated that the collagen derived dipeptide hydroxyprolyl-glycine promotes activation of the PI3K-Akt-mTOR signaling pathway. In a recent clinical investigation, Oertzen-Hagemann and others (2019) analyzed the skeletal muscle proteome before and after 12 weeks of resistance training in combination with CP supplementation. The authors demonstrated that anabolic signaling pathways were upregulated with CP combined with resistance training, as indicated by a higher upregulation of the MAPK pathway in response to CP supplementation compared to placebo intake (Oertzen-Hagemann et al., 2019).
Based on these findings (Kitakaze et al., 2016; Oertzen-Hagemann et al., 2019), the present study aimed to investigate the acute effects of CP in combination with resistance exercise on gene expression in pathways known to be activated in muscular remodeling, such as MAPK, mTOR and PI3K-Akt. Specifically, this study examined acute changes in gene expression in relation to a single high-load resistance exercise. Investigating these short-term responses is crucial for understanding basic physiological mechanisms of CP action in combination with resistance exercise.
Materials and Methods
Participants
In total, n = 30 male participants were included in this randomized-controlled double-blinded study. To decrease variability in anabolic responses, strict inclusion and exclusion criteria were utilized. Participant age was limited to between 18 and 29 years. All participants were currently recreationally active (∼150 min/wk of moderate or 75 min/wk of vigorous exercise (e.g., team sports, cycling)). Additionally, participants’ BMI was within 18.5 kg/m2 and 25 kg/m2. Participants diagnosed with any acute or chronic diseases, cardiac conditions, or metabolic disorders were excluded from the study.
The local ethics committee approved all procedures of this study, which all adhered with the Declaration of Helsinki. All participants were informed about the study procedures and any potential risks involved in the study and provided written informed consent (DRKS00027112).
Study Design
A randomized, controlled, double-blinded design was implemented to investigate the effects of CP ingestion combined with acute resistance exercise on skeletal muscle anabolic gene expression.
One week before the acute exercise trial, participants visited the laboratory and underwent a preliminary screening, which included a survey of the complete medical history and a general medical examination to ensure compliance with the study-specific inclusion criteria. After the assessment of eligibility, participants were randomly allocated into two groups either supplemented with CP or placebo. A random number generator was used for allocation sequence generation. All outcome assessors, statistical analysis group, as well as trainers and participants were blinded to group assignments.
One-Repetition Maximum Assessment
One week prior to the exercise trial participants visited the laboratory and completed a familiarization session with the knee extensor training device (FREI AG, Kirchzarten, Germany). Additionally, a one-repetition maximum (1RM) assessment of the right knee extensors was completed. Before conducting the 1RM test, a warm-up consisting of two sets of ten repetitions at submaximal load followed by two additional sets of three to five repetitions were completed (Baechle and Earle, 2000). During all warm-up and testing procedures, repetitions were performed with a full range of motion from 90° knee flexion to full extension (180°). After warm-up, participants selected a weight close to their perceived maximum and performed one repetition. Weight was increased by 5–10% until the participants were not able to lift the weight through the full range of motion (Baechle and Earle, 2000; Patterson and Ferguson, 2011). Each attempt was followed by a rest period of 4 min to assure recovery and the final 1RM was achieved within five attempts.
Experimental Protocol
After an overnight fast of 10–12 h, participants arrived at the laboratory between 7 and 8 am. To eliminate any bias of prior physical activity, all participants were instructed to refrain from any exercise 48 h prior to the trial and avoid certain means of transportation on their way to the laboratory (e.g., bicycle commute). After a resting period of 30 min, a muscle biopsy was obtained from the m. vastus lateralis (VL) muscle of the right leg (more details provided below). 60 min following this biopsy a high-load (80% 1RM) resistance exercise bout was completed (details provided below). Immediately after exercise participants consumed a beverage containing 250 ml of water and either 15 g of CP (Bodybalance®, Gelita AG, Eberbach, Germany) or PLA (silicon dioxide). CP and PLA products were similar in taste and appearance. Details on the specific CP amino acid composition are reported in (Table 1). Following the temporal dynamics of the uptake of collagen peptides (∼1–2 h after ingestion) (Shigemura et al., 2014), three additional biopsies were taken 1, 4 and 24 h after completion of the exercise bout (Figure 1). These time points have been frequently used in this context to assess intramuscular signaling cascades following exercise and nutritional interventions (Areta et al., 2013; Camera et al., 2015; Jackman et al., 2017). Between the first three biopsies, participants stayed at the laboratory and were instructed to rest.
Exercise Protocol
The exercise session was designed in accordance with protocols reported in previous studies investigating the acute effect of protein supplementation on muscle protein synthesis (Burd et al., 2010; Areta et al., 2013). All exercise was conducted using the FREI AG (Kirchzarten, Germany) knee extension machine. After a warm-up of two sets of five repetitions with 50 and 60% 1RM (Areta et al., 2013), four sets of 10 repetitions at 80% 1RM were completed with the right leg. Each set was separated by a 2-min resting period (Burd et al., 2010). The range of motion reached from 90° knee flexion to full extension (180°). The duration of the exercise session was ∼7.5 min.
Diet
Individual dietary intake was recorded by all participants for 72 h before the experimental trial as previously described (Burd et al., 2010). Further, all participants were instructed to abstain from alcohol and caffeine for 48 h before the experiment (Camera et al., 2015). The subjects were instructed to maintain their habitual dietary intake until the evening prior to the experimental trial. At this point in time, participants were provided with pre-packed meals, i.e., dinner on the night before the first biopsy as well as lunch and dinner between the 4 and 24 h biopsy. The meals were designed to meet protein guidelines of 0.4 g per kg body weight per meal for resistance-trained athletes (Schoenfeld and Aragon, 2018) corresponding to 1.2–1.4 g per kg bodyweight per day (Tang et al., 2009; Konig et al., 2020), and complying with caloric requirements estimated using the Benedict Harris equation (PAL 1,6) (Moore et al., 2009). Consequently, baseline biopsies as well as 24 h biopsies were obtained following an overnight fasting (Hulmi et al., 2009).
Muscle Biopsy Sampling
Muscle biopsies were obtained from the VL of the right leg using a 5-mm Bergström needle with manual suction following local anesthesia (2% Xylocitin) (Nielsen et al., 2012; Suetta et al., 2013). The location of all incision sites was randomized, and all incisions were 2–3 cm apart arranged in a parallelogram shape at the mid-portion of the VL muscle. After collection of the muscle tissue, the material was cleaned if there was excess blood using blotting paper and frozen in liquid nitrogen. Subsequently, all samples were stored at −80°C for later analysis (Nielsen et al., 2012; Suetta et al., 2013).
RNA Sequencing
RNA was prepared using Trizol (Gibco) followed by additional purification using the RNeasy Mini Kit (Qiagen). RNA quality was checked with the Agilent 2,100 Bioanalyzer and the RNA 6000 Nano Kit (Agilent, Waldbronn). Samples with RNA integrity number (RIN) above 9.5 were used for RNA sequencing. The sequencing work was performed by BGI Tech Solutions Co. (Hong Kong, China). RNA sequencing was performed with BGISEQ-500, a new desktop sequencer developed by BGI. Using DNA nanoball and combinational probe anchor synthesis developed from Complete Genomics™ sequencing technologies, it generates short reads at a large scale. The RNA sequencing was performed with 50 M reads paired end. The raw and normalized data are deposited in the Gene Expression Omnibus database (http://www.ncbi.nlm.nih.gov/geo/; accession No. GSE195585).
Statistical Analysis
Most of the statistical evaluations of the pre-to-post exercise changes in mRNA expression were completed with R and bioconductor using the NGS analysis plackage systempipe R (Backman and Girke, 2016). Quality control of raw sequencing reads was accomplished using FastQC (Babraham Bioinformatics). Low-quality reads were removed with trim_galore (version 0.6.4). The resulting reads were aligned to human genome version GRCh38. p13 from GeneCode and counted using kallisto version 0.46.1 (Bray et al., 2016). The count data was transformed to log2-counts per million (logCPM) using the voom-function from the limma package (Ritchie et al., 2015). The comparison of changes between CP and placebo was performed with the linear regression method using the limma package in R for every timepoint (1, 4, 24 h). A false positive rate of α = 0.05 with FDR correction was taken as the level of significance. Colored KEGG plots were made with the pathview package in R (Luo and Brouwer, 2013).
The pathway analysis was made with fgsea package (Sergushichev, 2016) and the enrichment browser package (Geistlinger et al., 2016) in R using the pathway information from KEGG database (https://www.genome.jp/kegg/pathway.html). The GSEA (gene set enrichment analysis) is a method to identify enriched pathways with up- or downregulated genes. Within this approach, enrichment scores (ES) are calculated, which reflect the degree to which a pathway is enriched with upregulated (positive ES) or downregulated (negative ES) genes (https://www.gsea-msigdb.org/gsea). This corresponds to up- or downregulated pathways. Normalizing the ES [normalized ES (NES)] by the mean of gene ES of all dataset permutations allows comparisons across gene sets. The gene pathway approach is of superior interpretational value compared to single gene expression analysis, since only the upregulation of a whole pathway (and thus the concert of upregulated single genes) facilitates specific functional consequences. As described above, the pathway composition is based on the KEGG database.
Results
All participants successfully completed all aspects of the study (n = 15 CP, n = 15 PLA). There were no differences between groups for any anthropometric or exercise related measurements (Table 2).
Gene Expression Analysis
Across groups, the signaling pathways that were most upregulated demonstrated peak changes in mRNA expression at the 4 h timepoint. This is in accordance with previous studies investigating the effects of acute resistance exercise on gene expression (Oertzen-Hagemann et al., 2019). Overall, at this timepoint a total of 30 signaling pathways were found to be significantly upregulated (Figure 2).
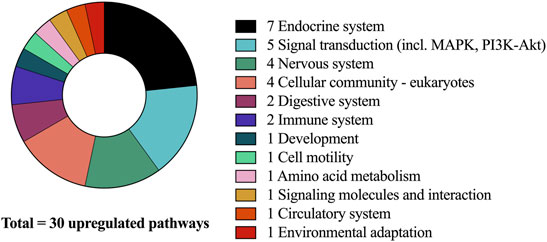
FIGURE 2. Upregulated pathways after CP supplementation compared to PLA at 4-h and associated sub-categories.
PI3K-Akt
Compared to baseline, the PI3K-Akt-Pathway was significantly enriched with upregulated genes at 1 h for both CP and PLA (pFDR < 0.05). At the 4 h timepoint, significant differences compared to baseline were observed for CP only (pFDR < 0.05). These differences were significantly larger compared to PLA with normalized enrichment scores of 1.67 for CP and 1.35 for PLA. No significant differences between CP and PLA were observed at the 24 h timepoint (Figure 3A).
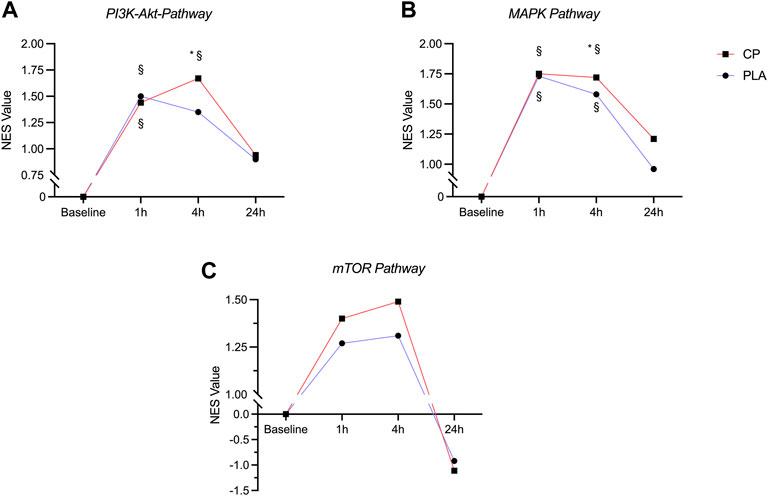
FIGURE 3. Time course of the normalized enrichment scores (NES) in the PI3K-Akt-Pathway (A), MAPK-Pathway (B) and mTOR pathway (C) in the collagen peptide (CP, n = 15) and placebo (PLA, n = 15) group. § = significantly different from baseline (pFDR < 0.05), * = significantly different from PLA (pFDR < 0.05).
MAPK
A significant upregulation of gene expression in the MAPK pathway was observed at 1 and 4 h post-exercise for both CP and PLA compared to baseline (pFDR < 0.05). Significant group differences were observed at 4 h in favor of CP (pFDR < 0.05). No significant upregulation of the MAPK gene expression pathway was seen after 24 h (Figure 3B).
mTOR
Compared to baseline, the mTOR pathway was descriptively enriched with upregulated genes at 1 and 4 h post exercise for both CP and PLA, however after correction for multiple testing these changes did not reach statistical significance for neither CP nor PLA (pFDR = 0.347) (Figure 3C).
Discussion
The main purpose of the present study was to systematically investigate changes in gene expression following an acute bout of high-load resistance exercise combined with specific collagen peptides compared to placebo. This acute study design was chosen, since it best reflects the temporal dynamics of gene expression. The main findings indicated a significantly upregulated gene expression in key myocellular pathways (PI3K-Akt, MAPK) involved in protein synthesis and muscle hypertrophy following the ingestion of collagen peptides compared to placebo.
The PI3K-Akt pathway can be activated by several precursors including IGF-1 and regulates multiple intracellular processes including muscle hypertrophy (Glass, 2010) and collagen synthesis (Yokoyama et al., 2012). As a serine-threonine protein kinase, Akt is capable of inducing myofibrillar protein synthesis and inhibit the upregulation of muscle atrophy markers such as MuRF-1 or Atrogin-1 via phosphorylation of FOXO (Glass, 2010). In addition to the inhibition of atrophy-related markers, PI3K-Akt has been demonstrated to block myostatin and thus stimulate differentiation and skeletal protein synthesis via distinct physiological mechanisms.
Previous research shows that the phosphorylation levels of Akt and PI3K-Akt are significantly elevated following an acute bout of resistance exercise (Dreyer et al., 2008; Camera et al., 2010; Horii et al., 2020) and that this response can be augmented by protein supplementation (Dreyer et al., 2008; Camera et al., 2015; Lane et al., 2017). Besides being stimulated by whey protein (Lane et al., 2017), Akt protein has recently been shown to be upregulated following the ingestion of CP in combination with chronic resistance exercise training (12 weeks) (Oertzen-Hagemann et al., 2019). The results of the present study complement these previous findings and demonstrated that PI3K and Akt mRNA are significantly upregulated following an acute bout of high-load exercise when ingesting a CP supplement compared to placebo. After inspection of relevant genes which might be involved in PI3K-Akt pathway upregulation following CP, angiopoietin-1 seems to play key roles as important activators of the PI3k signaling (Figure 4). In previous animal models, McClung et al. (2015) demonstrated that muscle cell derived angiopoietin-1 is involved in promoting myoblast differentiation and myotube formation. These findings are supported by studies from Mofarrahi et al. (2015) confirming strong positive associations of angiopoietin-1 and muscle regeneration following fiber injury. Interestingly, previous findings point towards the notion that the PI3K-Akt pathway plays an essential role in the expression of type I collagen induced by TGF-beta (Yokoyama et al., 2012). Although speculative, it might be assumed that the improvement in extracellular connective tissue might help to facilitate an enhanced contractile force transmission from the muscle to tendons or bones (Holwerda and van Loon, 2021). Indeed, such improvements in muscle strength and force have repeatedly been demonstrated in previous experiments following prolonged CP intake in combination with resistance training (Zdzieblik et al., 2015; Jendricke et al., 2019).
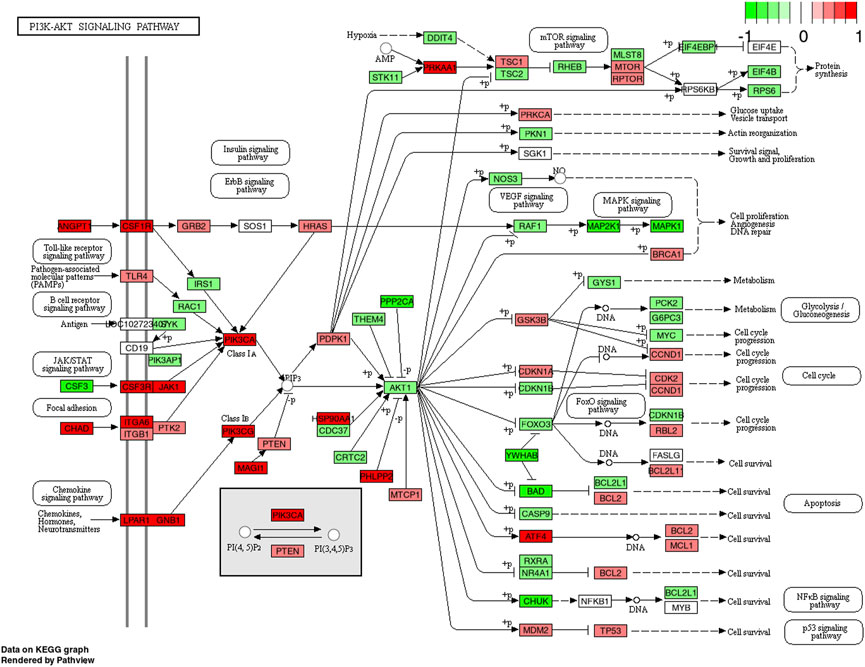
FIGURE 4. KEGG pathway of the PI3K-Akt signaling pathway with individual genes at 4 h comparing CP (collagen peptides) and PLA (placebo). Color label indicates genes that are up (red) or down (green) regulated compared to PLA. Dark coloring = p-value equal or below 0.05, light coloring = p-value higher than 0.05.
The mechanistic target of rapamycin (mTORC1) signaling pathway is generally considered as an important regulator of translation initiation (Bodine et al., 2001; Hoppeler, 2016). Besides the stimulatory effects of resistance exercise on the mTOR pathway (Drummond et al., 2009; Dreyer et al., 2010), numerous studies have also found favorable effects of protein supplementation manifested as an augmentation of mTOR phosphorylation and activation (Farnfield et al., 2009; Farnfield et al., 2012; Kakigi et al., 2014). Despite a visual increase, the present mRNA expression data demonstrated similar findings to these previous results, however, no statistical group by time effects were identified. A potential explanation for these results may lay within the temporal upregulation of the investigated pathways. Previous researchers confirmed that Akt acts as a precursor of mTOR by phosphorylating (and thus inhibiting) TSC2, a known suppressor of mTOR (Inoki et al., 2002). Given the present upregulation of PI3K-Akt gene expression at 4 h post exercise combined with CP intake, it may be speculated that mTOR upregulation peaked between 4 and 24 h and was thus missed due to the specific timing of sampling in the current study. Additionally, it could be speculated that the previously observed effects of longitudinal CP supplementation combined with resistance training on fat-free mass (Zdzieblik et al., 2015; Jendricke et al., 2019; Kirmse et al., 2019; Jendricke et al., 2020; Zdzieblik et al., 2021) can be explained via the stimulation of pathways other than the mTOR pathway (e.g., MAPK). The hypothesis of a potential convergence of mTOR and MAPK pathways to promote enhancements in anabolic signaling has been formulated by previous authors (Burd et al., 2010) although this aspect warrants further investigation. The exact mechanisms by which CP induce upregulations in gene expression remain largely unknown (Figure 5). Within the physiological chain of how upregulated gene expression pathways might facilitate physiologically relevant effects, Kitakaze et al. (2016) showed an increased upregulation of ribosomal S6 kinase which might facilitate increases in mRNA translation via eukaryotic initiation factor 3. Further studies are, however, needed to elucidate the physiological mechanisms in this field.
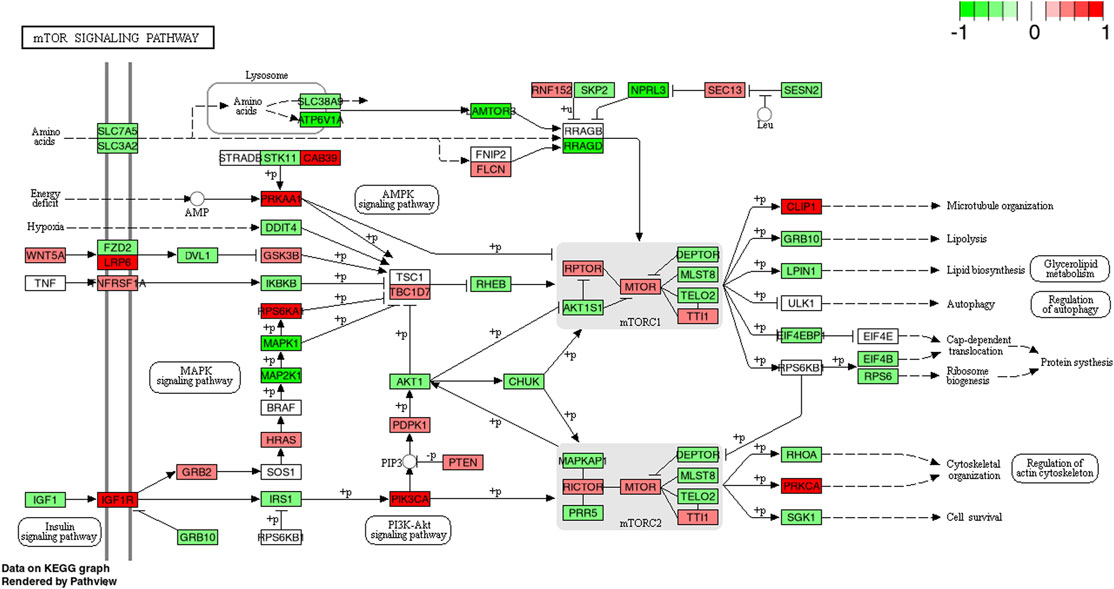
FIGURE 5. KEGG pathway of the mTOR signaling pathway with individual genes at 4 h comparing CP (collagen peptides) and PLA (placebo). Color label indicates genes that are up (red) or down (green) regulated compared to PLA. Dark coloring = p-value equal or below 0.05, light coloring = p-value higher than 0.05.
The MAPK-pathway has been shown to be involved in myofibrillar muscle protein synthesis as well as indirectly in collagen synthesis (Kimoto et al., 2004). A study by Holm et al. (2009) has shown that several steps in the MAPK pathway such as ERK1/2 or p38 are upregulated after exercise in an intensity-dependent manner and may therefore be reflective of the magnitude of mechanical stress applied to the muscle fiber (Martineau and Gardiner, 2001). Besides exercise-induced stimulation of the MAPK pathway (Williamson et al., 2003; Tannerstedt et al., 2009) there is conflicting evidence regarding the effects of protein feeding. In an early study by Karlsson et al. (2004) the authors found that the intake of branched-chained amino acids did not significantly augment the phosphorylation of several proteins found within the MAPK pathway (e.g., ERK1/2, p38) compared to resistance exercise alone. This is supported by a study from Moore and others (2011) indicating no effect of protein feeding alone but only when exercise was performed. Conversely, following a long-term trial with 12-weeks of resistance training and concomitant ingestion of CP, Oertzen-Hagemann et al. (2019) performed muscle proteome analyses and found that the MAPK pathway was overrepresented in the CP group compared to PLA. Although changes in muscle proteome and gene expression are not necessarily inter-related, these findings were confirmed by the present data, showing a significantly higher upregulation of MAPK mRNA in CP compared to PLA at 4 h post-exercise.
Interestingly, besides angiopoietin-1, upregulations of the MAPK pathway following CP ingestion seem to be driven by TGF-β-1 (Figure 6). TGF-β-1 is a multifunctional cytokine being involved in various cellular processes including type I collagen expression (Ismaeel et al., 2019) or myofibroblast transdifferentiation (Fan et al., 1999). In an earlier study by Heinemeier et al. (2003), the authors conclude that TGF-β-1 may play an important role in collagen formation after mechanical loading of tendon structures. As the PI3K-Akt and MAPK pathways are strongly inter-related, functional effects of extracellular matrix might facilitate optimized contractile force transmissions seen with long-term CP supplementation (Zdzieblik et al., 2015; Jendricke et al., 2019). This link, however, needs to be confirmed in future studies.
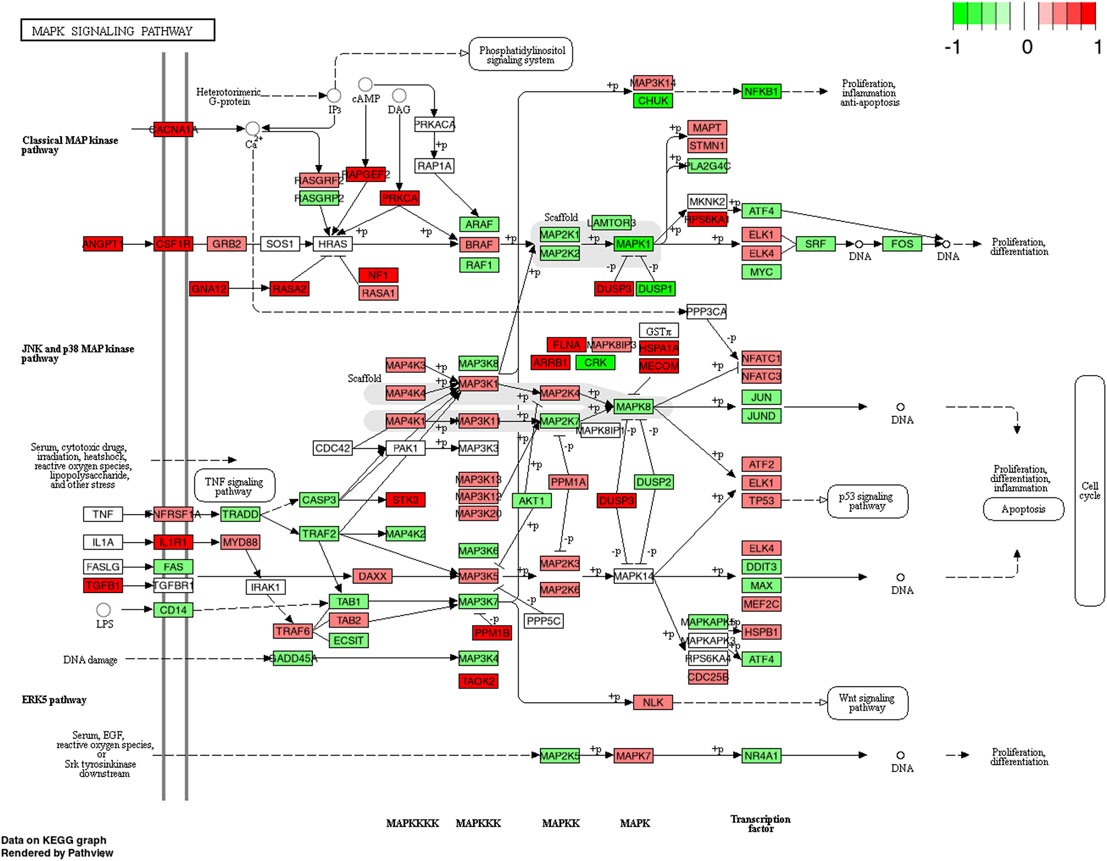
FIGURE 6. KEGG pathway of the MAPK signaling pathway with individual genes at 4 h comparing CP (collagen peptides) and PLA (placebo). Color label indicates genes that are up (red) or down (green) regulated compared to PLA. Dark coloring = p-value equal or below 0.05, light coloring = p-value higher than 0.05.
Although speculative, it may be argued that MAPK might be involved in mediating the effects on fat-free mass previously reported following 12 weeks of combined resistance training and CP supplementation (Zdzieblik et al., 2015; Jendricke et al., 2019; Jendricke et al., 2020; Zdzieblik et al., 2021). Such changes in fat-free mass might be explained by increases in either contractile or non-contractile elements. Generally, it must be mentioned that most studies focusing on intramuscular signaling following resistance exercise and protein supplementation investigated changes on the protein level. Therefore, further studies are needed which investigate the link between upregulated gene expression and myocellular as well as ECM protein synthesis. Conclusively, it needs to be mentioned that the findings from the present study are valid for this specific collagen peptide composition but might not necessarily be transferred to other collagen peptide ingredients.
Perspectives
The main objective was to examine important gene expression pathways involved in muscular remodeling. The choice of these specific pathways was based on previous experiments (Oertzen-Hagemann et al., 2019) highlighting increased upregulations of proteins associated with protein metabolism and contractile fibers. It is important to note that the anabolic response to nutrition and exercise is highly dynamic and the observed acute changes are not necessarily predictive of long-term adaptations. Moreover, the current study investigated young and recreationally active males and the acute responses in healthy individuals cannot readily be transferred to responses in clinical cohorts. Nevertheless, this might also be of high clinical relevance for patients suffering from sarcopenia or diseases associated with muscular atrophy. To obtain a high level of standardization, individuals in this study were fasted (except CP and PLA intake) between the baseline and 4 h biopsy to exclude other mediating factors resulting from additional nutritional protein sources which might influence the results obtained. Conclusively, future studies are needed which validate these findings in further study populations including gender, age and training status as well as long-term effects including pre-exercise meals.
Conclusion
The present study provides evidence for an increased upregulation of gene expression in the PI3K-Akt and MAPK pathways at 4 h following acute high-load resistance exercise combined with ingestion of CP compared to PLA. These results demonstrate for the first time that CP directly increase gene expression signaling of anabolic pathways in human skeletal muscle in response to an acute bout of resistance exercise. However, further studies, are needed to investigate the effects of combined resistance exercise and CP supplementation on PI3K-Akt and MAPK post-translational activation or miRNA regulation.
Data Availability Statement
The datasets presented in this study can be found in online repositories. The names of the repository/repositories and accession number(s) can be found below: https://www.ncbi.nlm.nih.gov/geo/, GSE195585.
Ethics Statement
The studies involving human participants were reviewed and approved by the Ethics Committee Freiburg. The patients/participants provided their written informed consent to participate in this study.
Author Contributions
CC, SJ, AM, NG, PA, JN, UF, CS, AG, and DK conceived and designed the experiments; CC, SJ, AM, AH, EV, SG, and DK performed the experiments; CC, SJ, CS, NG, and DK analyzed the experiments; CC, SJ, AM, SO, NG, PA, JN, UF, CS, AG, and DK were involved in data interpretation. CC and DK wrote the manuscript. All authors revised the manuscript, approved the final version and are accountable for all aspects of the work. All persons designated as authors qualify for authorship, and all those who qualify for authorship are listed.
Funding
Part of the costs was paid by Gelita AG, Uferstraße 7, Eberbach, Germany.
Conflict of Interest
SO is coinventor of patents concerning the use of collagen peptides.
The remaining authors declare that the research was conducted in the absence of any commercial or financial relationships that could be construed as a potential conflict of interest.
Publisher’s Note
All claims expressed in this article are solely those of the authors and do not necessarily represent those of their affiliated organizations, or those of the publisher, the editors and the reviewers. Any product that may be evaluated in this article, or claim that may be made by its manufacturer, is not guaranteed or endorsed by the publisher.
Acknowledgments
We thank all participants who volunteered for this study.
Abbreviations
1RM, One-repetition maximum; CP, Collagen peptides; ES, Enrichment score; FOXO, Forkhead-Box-Protein; GSEA, Gene set enrichment analysisl; MAPK, Mitogen-activated protein kinase; mTOR, Mechanistic target of rapamycin; MuRF1, Muscle RING-finger protein-1; NES, Normalized enrichment score; VL, Vastus lateralis muscle.
References
Areta J. L., Burke L. M., Ross M. L., Camera D. M., West D. W. D., Broad E. M., et al. (2013). Timing and Distribution of Protein Ingestion during Prolonged Recovery from Resistance Exercise Alters Myofibrillar Protein Synthesis. J. Physiol. 591, 2319–2331. doi:10.1113/jphysiol.2012.244897
Backman T. W. H., Girke T. (2016). systemPipeR: NGS Workflow and Report Generation Environment. BMC Bioinformatics 17, 388. doi:10.1186/s12859-016-1241-0
Baechle T. R., Earle R. W. (2000). Essentials of Strength Training and Conditioning. Champaign: Human Kinetics.
Bodine S. C., Stitt T. N., Gonzalez M., Kline W. O., Stover G. L., Bauerlein R., et al. (2001). Akt/mTOR Pathway Is a Crucial Regulator of Skeletal Muscle Hypertrophy and Can Prevent Muscle Atrophy In Vivo. Nat. Cel Biol. 3, 1014–1019. doi:10.1038/ncb1101-1014
Bray N. L., Pimentel H., Melsted P., Pachter L. (2016). Near-optimal Probabilistic RNA-Seq Quantification. Nat. Biotechnol. 34, 525–527. doi:10.1038/nbt.3519
Burd N. A., Tang J. E., Moore D. R., Phillips S. M. (2009). Exercise Training and Protein Metabolism: Influences of Contraction, Protein Intake, and Sex-Based Differences. J. Appl. Physiol. (1985) 106, 1692–1701. doi:10.1152/japplphysiol.91351.2008
Burd N. A., Holwerda A. M., Selby K. C., West D. W. D., Staples A. W., Cain N. E., et al. (2010). Resistance Exercise Volume Affects Myofibrillar Protein Synthesis and Anabolic Signalling Molecule Phosphorylation in Young Men. J. Physiol. 588, 3119–3130. doi:10.1113/jphysiol.2010.192856
Camera D. M., Edge J., Short M. J., Hawley J. A., Coffey V. G. (2010). Early Time Course of Akt Phosphorylation after Endurance and Resistance Exercise. Med. Sci. Sports Exerc. 42, 1843–1852. doi:10.1249/MSS.0b013e3181d964e4
Camera D. M., West D. W. D., Phillips S. M., Rerecich T., Stellingwerff T., Hawley J. A., et al. (2015). Protein Ingestion Increases Myofibrillar Protein Synthesis after Concurrent Exercise. Med. Sci. Sports Exerc. 47, 82–91. doi:10.1249/mss.0000000000000390
Darren G. C., Natalie C. B., Smith-Palmer T., Darren G. B. (2006). Effect of Whey and Soy Protein Supplementation Combined with Resistance Training in Young Adults. Int. J. Sport Nutr. Exerc. Metab. 16, 233–244. doi:10.1123/ijsnem.16.3.233
Dreyer H. C., Drummond M. J., Pennings B., Fujita S., Glynn E. L., Chinkes D. L., et al. (2008). Leucine-enriched Essential Amino Acid and Carbohydrate Ingestion Following Resistance Exercise Enhances mTOR Signaling and Protein Synthesis in Human Muscle. Am. J. Physiol. Endocrinol. Metab. 294, E392–E400. doi:10.1152/ajpendo.00582.2007
Dreyer H. C., Fujita S., Glynn E. L., Drummond M. J., Volpi E., Rasmussen B. B. (2010). Resistance Exercise Increases Leg Muscle Protein Synthesis and mTOR Signalling Independent of Sex. Acta Physiol. 199, 71–81. doi:10.1111/j.1748-1716.2010.02074.x
Drummond M. J., Rasmussen B. B. (2008). Leucine-enriched Nutrients and the Regulation of Mammalian Target of Rapamycin Signalling and Human Skeletal Muscle Protein Synthesis. Curr. Opin. Clin. Nutr. Metab. Care 11, 222–226. doi:10.1097/mco.0b013e3282fa17fb
Drummond M. J., Dreyer H. C., Fry C. S., Glynn E. L., Rasmussen B. B. (2009). Nutritional and Contractile Regulation of Human Skeletal Muscle Protein Synthesis and mTORC1 Signaling. J. Appl. Physiol. (1985) 106, 1374–1384. doi:10.1152/japplphysiol.91397.2008
Fan J.-M., Ng Y.-Y., Hill P. A., Nikolic-Paterson D. J., Mu W., Atkins R. C., et al. (1999). Transforming Growth Factor-β Regulates Tubular Epithelial-Myofibroblast Transdifferentiation In Vitro. Kidney Int. 56, 1455–1467. doi:10.1046/j.1523-1755.1999.00656.x
Farnfield M., Carey K., Gran P., Trenerry M., Cameron-Smith D. (2009). Whey Protein Ingestion Activates mTOR-dependent Signalling after Resistance Exercise in Young Men: a Double-Blinded Randomized Controlled Trial. Nutrients 1, 263–275. doi:10.3390/nu1020263
Farnfield M. M., Breen L., Carey K. A., Garnham A., Cameron-Smith D. (2012). Activation of mTOR Signalling in Young and Old Human Skeletal Muscle in Response to Combined Resistance Exercise and Whey Protein Ingestion. Appl. Physiol. Nutr. Metab. 37, 21–30. doi:10.1139/h11-132
Geistlinger L., Csaba G., Zimmer R. (2016). Bioconductor's EnrichmentBrowser: Seamless Navigation through Combined Results of Set- & Network-Based Enrichment Analysis. BMC Bioinformatics 17, 45. doi:10.1186/s12859-016-0884-1
Glass D. J. (2010). PI3 Kinase Regulation of Skeletal Muscle Hypertrophy and Atrophy. Curr. Top. Microbiol. Immunol. 346, 267–278. doi:10.1007/82_2010_78
Hamarsland H., Aas S. N., Nordengen A. L., Holte K., Garthe I., Paulsen G., et al. (2019). Native Whey Induces Similar Post Exercise Muscle Anabolic Responses as Regular Whey, Despite Greater Leucinemia, in Elderly Individuals. J. Nutr. Health Aging 23, 42–50. doi:10.1007/s12603-018-1105-6
Heinemeier K., Langberg H., Olesen J. L., Kjaer M. (2003). Role of TGF-β1 in Relation to Exercise-Induced Type I Collagen Synthesis in Human Tendinous Tissue. J. Appl. Physiol. 95, 2390–2397. doi:10.1152/japplphysiol.00403.2003
Hoffmann C., Weigert C. (2017). Skeletal Muscle as an Endocrine Organ: The Role of Myokines in Exercise Adaptations. Cold Spring Harb. Perspect. Med. 7, a029793. doi:10.1101/cshperspect.a029793
Holm L., Van Hall G., Rose A. J., Miller B. F., Doessing S., Richter E. A., et al. (2009). Contraction Intensity and Feeding Affect Collagen and Myofibrillar Protein Synthesis Rates Differently in Human Skeletal Muscle. Am. J. Physiol. Endocrinol. Metab. 298, E257–E269. doi:10.1152/ajpendo.00609.2009
Holwerda A. M., van Loon L. J. C. (2021). The Impact of Collagen Protein Ingestion on Musculoskeletal Connective Tissue Remodeling: a Narrative Review. Nutr. Rev., nuab083. doi:10.1093/nutrit/nuab083
Hoppeler H. (2016). Molecular Networks in Skeletal Muscle Plasticity. J. Exp. Biol. 219, 205–213. doi:10.1242/jeb.128207
Horii N., Hasegawa N., Fujie S., Uchida M., Iemitsu M. (2020). Resistance Exercise‐induced Increase in Muscle 5α‐dihydrotestosterone Contributes to the Activation of Muscle Akt/mTOR/p70S6K‐ and Akt/AS160/GLUT4‐signaling Pathways in Type 2 Diabetic Rats. FASEB J. 34, 11047–11057. doi:10.1096/fj.201903223rr
Hulmi J. J., Tannerstedt J., Selänne H., Kainulainen H., Kovanen V., Mero A. A. (2009). Resistance Exercise with Whey Protein Ingestion Affects mTOR Signaling Pathway and Myostatin in Men. J. Appl. Physiol. (1985) 106, 1720–1729. doi:10.1152/japplphysiol.00087.2009
Hultman E., Bergström J., Roch-Norlund A. E. (1971). “Glycogen Storage in Human Skeletal Muscle,” in Muscle Metabolism during Exercise: Proceedings of a Karolinska Institutet Symposium Held in Stockholm, Sweden, September 6–9, 1970 Honorary Guest: E Hohwü Christensen. Editors B. Pernow, and B. Saltin (Boston, MA: Springer US), 273–288. doi:10.1007/978-1-4613-4609-8_24
Inoki K., Li Y., Zhu T., Wu J., Guan K.-L. (2002). TSC2 Is Phosphorylated and Inhibited by Akt and Suppresses mTOR Signalling. Nat. Cel Biol. 4, 648–657. doi:10.1038/ncb839
Ismaeel A., Kim J.-S., Kirk J. S., Smith R. S., Bohannon W. T., Koutakis P. (2019). Role of Transforming Growth Factor-β in Skeletal Muscle Fibrosis: A Review. Int. J. Mol. Sci. 20, 2446. doi:10.3390/ijms20102446
Jacinto J. L., Nunes J. P., Gorissen S. H. M., Capel D. M. G., Bernardes A. G., Ribeiro A. S., et al. (2022). Whey Protein Supplementation Is Superior to Leucine-Matched Collagen Peptides to Increase Muscle Thickness during a 10-Week Resistance Training Program in Untrained Young Adults. Int. J. Sport Nutr. Exerc. Metab., 1–11. doi:10.1123/ijsnem.2021-0265
Jackman S. R., Witard O. C., Philp A., Wallis G. A., Baar K., Tipton K. D. (2017). Branched-Chain Amino Acid Ingestion Stimulates Muscle Myofibrillar Protein Synthesis Following Resistance Exercise in Humans. Front. Physiol. 8, 390. doi:10.3389/fphys.2017.00390
Janssen I., Heymsfield S. B., Wang Z., Ross R. (2000). Skeletal Muscle Mass and Distribution in 468 Men and Women Aged 18-88 Yr. J. Appl. Physiol. (1985) 89, 81–88. doi:10.1152/jappl.2000.89.1.81
Jendricke P., Centner C., Zdzieblik D., Gollhofer A., König D. (2019). Specific Collagen Peptides in Combination with Resistance Training Improve Body Composition and Regional Muscle Strength in Premenopausal Women: A Randomized Controlled Trial. Nutrients 11, 892. doi:10.3390/nu11040892
Jendricke P., Kohl J., Centner C., Gollhofer A., König D. (2020). Influence of Specific Collagen Peptides and Concurrent Training on Cardiometabolic Parameters and Performance Indices in Women: A Randomized Controlled Trial. Front. Nutr. 7, 580918. doi:10.3389/fnut.2020.580918
Kakigi R., Yoshihara T., Ozaki H., Ogura Y., Ichinoseki-Sekine N., Kobayashi H., et al. (2014). Whey Protein Intake after Resistance Exercise Activates mTOR Signaling in a Dose-dependent Manner in Human Skeletal Muscle. Eur. J. Appl. Physiol. 114, 735–742. doi:10.1007/s00421-013-2812-7
Karlsson H. K. R., Nilsson P.-A., Nilsson J., Chibalin A. V., Zierath J. R., Blomstrand E. (2004). Branched-chain Amino Acids Increase p70S6k Phosphorylation in Human Skeletal Muscle after Resistance Exercise. Am. J. Physiol. Endocrinol. Metab. 287, E1–E7. doi:10.1152/ajpendo.00430.2003
Kimoto K., Nakatsuka K., Matsuo N., Yoshioka H. (2004). p38 MAPK Mediates the Expression of Type I Collagen Induced by TGF-β2 in Human Retinal Pigment Epithelial Cells ARPE-19. Invest. Ophthalmol. Vis. Sci. 45, 2431–2437. doi:10.1167/iovs.03-1276
Kirmse M., Oertzen-Hagemann V., de Marées M., Bloch W., Platen P. (2019). Prolonged Collagen Peptide Supplementation and Resistance Exercise Training Affects Body Composition in Recreationally Active Men. Nutrients 11, 1154. doi:10.3390/nu11051154
Kitakaze T., Sakamoto T., Kitano T., Inoue N., Sugihara F., Harada N., et al. (2016). The Collagen Derived Dipeptide Hydroxyprolyl-glycine Promotes C2C12 Myoblast Differentiation and Myotube Hypertrophy. Biochem. Biophys. Res. Commun. 478, 1292–1297. doi:10.1016/j.bbrc.2016.08.114
Konig D., Carlsohn A., Braun H., Grosshauser M., Lampen A., Mosler S., et al. (2020). Proteins in Sports Nutrition. Position of the Working Group Sports Nutrition of the German Nutrition Society (DGE). Ernahrungs Umschau 67, M406–M413. doi:10.5960/dzsm.2020.450
Lane M., Herda T., Fry A., Cooper M., Andre M., Gallagher P. (2017). Endocrine Responses and Acute mTOR Pathway Phosphorylation to Resistance Exercise with Leucine and Whey. Biol. Sport 2, 197–203. doi:10.5114/biolsport.2017.65339
Luo W., Brouwer C. (2013). Pathview: an R/Bioconductor Package for Pathway-Based Data Integration and Visualization. Bioinformatics 29, 1830–1831. doi:10.1093/bioinformatics/btt285
Martineau L. C., Gardiner P. F. (2001). Insight into Skeletal Muscle Mechanotransduction: MAPK Activation Is Quantitatively Related to Tension. J. Appl. Physiol. (1985) 91, 693–702. doi:10.1152/jappl.2001.91.2.693
McClung J. M., Reinardy J. L., Mueller S. B., Mccord T. J., Kontos C. D., Brown D. A., et al. (2015). Muscle Cell Derived Angiopoietin-1 Contributes to Both Myogenesis and Angiogenesis in the Ischemic Environment. Front. Physiol. 6, 161. doi:10.3389/fphys.2015.00161
Moberg M., Apró W., Ekblom B., Van Hall G., Holmberg H.-C., Blomstrand E. (2016). Activation of mTORC1 by Leucine Is Potentiated by Branched-Chain Amino Acids and Even More So by Essential Amino Acids Following Resistance Exercise. Am. J. Physiol. Cell Physiol. 310, C874–C884. doi:10.1152/ajpcell.00374.2015
Mofarrahi M., Mcclung J. M., Kontos C. D., Davis E. C., Tappuni B., Moroz N., et al. (2015). Angiopoietin-1 Enhances Skeletal Muscle Regeneration in Mice. Am. J. Physiol. Regulatory Integr. Comp. Physiol. 308, R576–R589. doi:10.1152/ajpregu.00267.2014
Moore D. R., Robinson M. J., Fry J. L., Tang J. E., Glover E. I., Wilkinson S. B., et al. (2009). Ingested Protein Dose Response of Muscle and Albumin Protein Synthesis after Resistance Exercise in Young Men. Am. J. Clin. Nutr. 89, 161–168. doi:10.3945/ajcn.2008.26401
Moore D. R., Atherton P. J., Rennie M. J., Tarnopolsky M. A., Phillips S. M. (2011). Resistance Exercise Enhances mTOR and MAPK Signalling in Human Muscle over that Seen at Rest after Bolus Protein Ingestion. Acta Physiol. (Oxf) 201, 365–372. doi:10.1111/j.1748-1716.2010.02187.x
Morton R. W., Murphy K. T., Mckellar S. R., Schoenfeld B. J., Henselmans M., Helms E., et al. (2018). A Systematic Review, Meta-Analysis and Meta-Regression of the Effect of Protein Supplementation on Resistance Training-Induced Gains in Muscle Mass and Strength in Healthy Adults. Br. J. Sports Med. 52, 376–384. doi:10.1136/bjsports-2017-097608
Nielsen J. L., Aagaard P., Bech R. D., Nygaard T., Hvid L. G., Wernbom M., et al. (2012). Proliferation of Myogenic Stem Cells in Human Skeletal Muscle in Response to Low-Load Resistance Training with Blood Flow Restriction. J. Physiol. 590, 4351–4361. doi:10.1113/jphysiol.2012.237008
Oertzen-Hagemann V., Kirmse M., Eggers B., Pfeiffer K., Marcus K., de Marées M., et al. (2019). Effects of 12 Weeks of Hypertrophy Resistance Exercise Training Combined with Collagen Peptide Supplementation on the Skeletal Muscle Proteome in Recreationally Active Men. Nutrients 11, 1072. doi:10.3390/nu11051072
Oikawa S. Y., Kamal M. J., Webb E. K., Mcglory C., Baker S. K., Phillips S. M. (2020). Whey Protein but Not Collagen Peptides Stimulate Acute and Longer-Term Muscle Protein Synthesis with and without Resistance Exercise in Healthy Older Women: a Randomized Controlled Trial. Am. J. Clin. Nutr. 111, 708–718. doi:10.1093/ajcn/nqz332
Patterson S. D., Ferguson R. A. (2011). Enhancing Strength and Postocclusive Calf Blood Flow in Older People with Training with Blood-Flow Restriction. J. Aging Phys. Act 19, 201–213. doi:10.1123/japa.19.3.201
Ritchie M. E., Phipson B., Wu D., Hu Y., Law C. W., Shi W., et al. (2015). Limma powers Differential Expression Analyses for RNA-Sequencing and Microarray Studies. Nucleic Acids Res. 43, e47. doi:10.1093/nar/gkv007
Roux P. P., Shahbazian D., Vu H., Holz M. K., Cohen M. S., Taunton J., et al. (2007). RAS/ERK Signaling Promotes Site-specific Ribosomal Protein S6 Phosphorylation via RSK and Stimulates Cap-dependent Translation. J. Biol. Chem. 282, 14056–14064. doi:10.1074/jbc.m700906200
Schoenfeld B. J., Aragon A. A. (2018). How Much Protein Can the Body Use in a Single Meal for Muscle-Building? Implications for Daily Protein Distribution. J. Int. Soc. Sports Nutr. 15, 10. doi:10.1186/s12970-018-0215-1
Sergushichev A. A. (2016). An Algorithm for Fast Preranked Gene Set Enrichment Analysis Using Cumulative Statistic Calculation. bioRxiv, 060012. doi:10.1101/060012
Shigemura Y., Kubomura D., Sato Y., Sato K. (2014). Dose-dependent Changes in the Levels of Free and Peptide Forms of Hydroxyproline in Human Plasma after Collagen Hydrolysate Ingestion. Food Chem. 159, 328–332. doi:10.1016/j.foodchem.2014.02.091
Suetta C., Frandsen U., Mackey A. L., Jensen L., Hvid L. G., Bayer M. L., et al. (2013). Ageing Is Associated with Diminished Muscle Re-growth and Myogenic Precursor Cell Expansion Early after Immobility-Induced Atrophy in Human Skeletal Muscle. J. Physiol. 591, 3789–3804. doi:10.1113/jphysiol.2013.257121
Tang J. E., Moore D. R., Kujbida G. W., Tarnopolsky M. A., Phillips S. M. (2009). Ingestion of Whey Hydrolysate, Casein, or Soy Protein Isolate: Effects on Mixed Muscle Protein Synthesis at Rest and Following Resistance Exercise in Young Men. J. Appl. Physiol. (1985) 107, 987–992. doi:10.1152/japplphysiol.00076.2009
Tannerstedt J., Apró W., Blomstrand E. (2009). Maximal Lengthening Contractions Induce Different Signaling Responses in the Type I and Type II Fibers of Human Skeletal Muscle. J. Appl. Physiol. (1985) 106, 1412–1418. doi:10.1152/japplphysiol.91243.2008
Tipton K. D., Elliott T. A., Cree M. G., Wolf S. E., Sanford A. P., Wolfe R. R. (2004). Ingestion of Casein and Whey Proteins Result in Muscle Anabolism after Resistance Exercise. Med. Sci. Sports Exerc. 36, 2073–2081. doi:10.1249/01.mss.0000147582.99810.c5
Wang X., Li W., Williams M., Terada N., Alessi D. R., Proud C. G. (2001). Regulation of Elongation Factor 2 Kinase by p90RSK1 and P70 S6 Kinase. EMBO J. 20, 4370–4379. doi:10.1093/emboj/20.16.4370
Wang D. X. M., Yao J., Zirek Y., Reijnierse E. M., Maier A. B. (2020). Muscle Mass, Strength, and Physical Performance Predicting Activities of Daily Living: a Meta‐analysis. J. Cachexia Sarcopenia Muscle 11, 3–25. doi:10.1002/jcsm.12502
Williamson D., Gallagher P., Harber M., Hollon C., Trappe S. (2003). Mitogen-activated Protein Kinase (MAPK) Pathway Activation: Effects of Age and Acute Exercise on Human Skeletal Muscle. J. Physiol. 547, 977–987. doi:10.1113/jphysiol.2002.036673
Wirth J., Hillesheim E., Brennan L. (2020). The Role of Protein Intake and its Timing on Body Composition and Muscle Function in Healthy Adults: A Systematic Review and Meta-Analysis of Randomized Controlled Trials. J. Nutr. 150, 1443–1460. doi:10.1093/jn/nxaa049
Yokoyama K., Kimoto K., Itoh Y., Nakatsuka K., Matsuo N., Yoshioka H., et al. (2012). The PI3K/Akt Pathway Mediates the Expression of Type I Collagen Induced by TGF-β2 in Human Retinal Pigment Epithelial Cells. Graefes Arch. Clin. Exp. Ophthalmol. 250, 15–23. doi:10.1007/s00417-011-1766-x
Zdzieblik D., Oesser S., Baumstark M. W., Gollhofer A., König D. (2015). Collagen Peptide Supplementation in Combination with Resistance Training Improves Body Composition and Increases Muscle Strength in Elderly Sarcopenic Men: a Randomised Controlled Trial. Br. J. Nutr. 114, 1237–1245. doi:10.1017/s0007114515002810
Keywords: gene expression, pathway analysis, collagen peptides, resistance exercise, KEGG enrichment analysis
Citation: Centner C, Jerger S, Mallard A, Herrmann A, Varfolomeeva E, Gollhofer S, Oesser S, Sticht C, Gretz N, Aagaard P, Nielsen JL, Frandsen U, Suetta C, Gollhofer A and König D (2022) Supplementation of Specific Collagen Peptides Following High-Load Resistance Exercise Upregulates Gene Expression in Pathways Involved in Skeletal Muscle Signal Transduction. Front. Physiol. 13:838004. doi: 10.3389/fphys.2022.838004
Received: 17 December 2021; Accepted: 22 March 2022;
Published: 05 April 2022.
Edited by:
Katsuhiko Suzuki, Waseda University, JapanReviewed by:
Eva Blomstrand, Swedish School of Sport and Health Sciences, SwedenWilhelm Bloch, German Sport University Cologne, Germany
Copyright © 2022 Centner, Jerger, Mallard, Herrmann, Varfolomeeva, Gollhofer, Oesser, Sticht, Gretz, Aagaard, Nielsen, Frandsen, Suetta, Gollhofer and König. This is an open-access article distributed under the terms of the Creative Commons Attribution License (CC BY). The use, distribution or reproduction in other forums is permitted, provided the original author(s) and the copyright owner(s) are credited and that the original publication in this journal is cited, in accordance with accepted academic practice. No use, distribution or reproduction is permitted which does not comply with these terms.
*Correspondence: Christoph Centner, Q2hyaXN0b3BoLmNlbnRuZXJAc3BvcnQudW5pLWZyZWlidXJnLmRl