- 1Department for Life Quality Studies University of Bologna, Bologna, Italy
- 2Sport Engineering Lab, Department Industrial Engineering, University of Rome “Tor Vergata”, Rome, Italy
- 3Department of Human Neuroscience, Faculty of Medicine and Dentistry, Sapienza University of Rome, Rome, Italy
- 4Sport and Exercise Sciences Research Unit, Department of Psychology, Educational Science and Human Movement, University of Palermo, Palermo, Italy
- 5Italian Canoe/Kayak Federation (FICK), Rome, Italy
- 6Department of Physiotherapy, Isra University, Amman, Jordan
- 7Department of Medicine Systems, University of Rome “Tor Vergata”, Rome, Italy
- 8Centre of Space Bio-Medicine, “Tor Vergata” University of Rome, Rome, Italy
- 9Department of Human Science and Promotion of Quality of Life, San Raffaele Open University of Rome, Rome, Italy
Several studies showed significant differences between bench lift exercises without investigating which is more related, in biomechanical and neuromuscular terms, to improve the sprint flatwater kayak performance. This study aims to compare the power-load and velocity-load neuromuscular parameters performed in prone bench pull (PBP), and bench press (BP) exercises to identify which of them meet the gesture specificity in sprint flatwater kayak performance. Ten elite kayakers participated in this study. Power-load, velocity-load relationships, the maximum dynamic strength, and the kayak sprint performance test were assessed. The power-load and velocity-load relationships showed significant differences between the PBP and BP for each considered load. The kayakers showed a significant correlation between maximum power performed on the PBP and the maximum velocity reached in the kayak sprint (r = 0.80, p < 0.01) and the stroke frequency (r = 0.61, p < 0.05). Conversely, the maximum power performed on the BP did not correlate with the kinematic parameters analyzed. In addition, the maximum dynamic strength in the PBP and BP did not correlate with the maximum velocity and stroke frequency. Furthermore, no significant difference was observed in both the bench exercises for the maximum dynamic strength (p > 0.05). The results of this study suggest that the maximal muscular power expressed in PBP exercise only seems to be more specific in kayak velocity performance compared with maximal dynamic strength and with all dynamic parameters recorded in the BP. This will allow coaches and trainers to use specific bench exercises for specific neuromuscular kayakers’ adaptations during the whole competitive season.
Introduction
Canoeing/kayaking is a sport where upper limbs are predominant in the propulsion phase of the boat while the action of lower limbs counteracts only the consequent kayak rotations (Mann and Kearney, 1980; Begon et al., 2010; Baker, 2012) even though in recent studies were observed a connection between improvements in lower limbs force and kayak sprint velocity (Nilsson and Rosdahl, 2016; Lum and Aziz, 2020). To maximize kayak velocity, the paddler generates high propulsive power by applying forces on the paddle blade during each stroke (Aitken and Neal, 1992; Michael et al., 2008). During the race, the kayak shows a changeable velocity (ranging from 4.63 to 5.38 m/s) generated by the paddler’s actions against the drag forces (Kendal and Sanders, 1992; Zumerchik, 1997; Gomes et al., 2015b). Therefore, to increase the kayak velocity, the paddler, dipping and pulling backward the blade (pull phase), has to produce a propulsive force more significant than the drag force (Millward, 1987; Jackson, 1995). Differently, during the aerial phase, only the drag forces (friction, form, wave) act on the kayak, decelerating it (Bonaiuto et al., 2020). Therefore, it is possible to deduce that the kayak means velocity is the consequence of the combined effects of the propulsion and the drag forces (Pendergast et al., 2005; Michael et al., 2009). In order to improve the propulsion phase useful to reduce the race time performance, the kayaker usually conditions the strength and power of upper limbs muscles through the prone bench pull (PBP) and bench press (BP) exercises (Akca and Muniroglu, 2008; García-Pallarés et al., 2009; Pearson et al., 2009; McKean and Burkett, 2010, 2014; Ualí et al., 2012; Hamano et al., 2015; Bielik et al., 2017; Bjerkefors et al., 2018; Winchcombe et al., 2019). Uali et al. (2012) reported that heavy resistance training performed in bilateral bent pull and one-arm cable row significantly correlated with the start phase of kayak sprint performances. In addition, Liow and Hopkins (2003), using both bench press and bilateral dumbbell prone lifts exercises, have shown that heavy resistance training seems to be more effective in conditioning the start phase (0–15 m) of kayak sprint performance while an explosive power training (low loads performed at high contraction velocity) could be more effective to maintain kayak velocity. However, it is necessary to consider that BP and PBP exercises present some distinctive biomechanical and neuromuscular features that make them antagonistic exercises to each other (Pearson et al., 2009; Sánchez-Medina et al., 2014). In this context, it should be more appropriate to consider these differences in specific strength and power conditioning and assessments in those sports disciplines that use upper limbs differently in pushing or pulling actions (Sánchez-Medina et al., 2014). According to these considerations, to increase the propulsive power produced by the paddler, it is necessary to condition in a dry-land environment, specific kinetic muscle chains and neuromuscular patterns using the power based-training method (Cronin et al., 2001). For that, it is essential to determine the power-load and velocity-load relationships, analyzed on bench exercises, monitoring the kinetic parameters with a dynamometer during an increasing loads test performance (Pearson et al., 2009; Sánchez-Medina et al., 2014; Sreckovic et al., 2015). Thus, this study aims to compare the power-load (p-l) and velocity-load (v-l) relationships expressed in BP and PBP exercises, verifying which of their dynamic parameters, 1RM and maximum power (Pmax), is more correlated with the maximum velocity reached during flatwater kayak performance.
Materials and methods
Participants
Ten elite male kayak athletes [age: 28.88 ± 2.26 (yrs), height: 1.85 ± 0.04 (m), weight: 84.93 ± 5.96 (kg), Body Mass Index: 24.60 ± 1.46 (kg/m2)] were involved in the study. They are members of the Italian Federation Canoe-Kayak (FICK) team with wide experience in international competitions. The subjects trained ten times a week during the study period (May), including four dry-land training and seven water sessions. The study was reviewed and approved by the Internal Research Board of “Tor Vergata” University of Rome. The subjects provided their written informed consent to participate in this study. All procedures were carried out in accordance with the Declaration of Helsinki.
Testing procedure
In order to determine 1RM, power-load, and velocity-load relationships, a linear encoder (Bosco et al., 1995) was used during the increasing load test performed on the PBP and BP exercises. Regarding the water tests, three trials of the 50 m all-out kayak sprint test (KST) were assessed to measure maximum velocity and stroke frequency. Each athlete was evaluated in five sessions (1RMPBP, 1RMBP, PBPp-l & v-l, BPp-l & v-l, and KST assessment) separated by 24 h of rest for each load test while 48 h of rest between the last load session and KST.
Power-load and velocity-load relationships
Before the strength and power tests, the athletes performed a warm-up for the upper limbs completed in 20 min (5–8 min for static/dynamic stretching and joint mobilization) and 5–12 min for shoulder circumduction and shoulder horizontal abduction and adduction).
The standard procedure to assess power-load, velocity-load relationships, and maximum strength through the 1RM were determined in the PBP and BP exercises as suggested by Sreckovic et al. (2015).
In the PBP exercise, a modified Smith machine was used (Pearson et al., 2009), where the subject was in the prone position on the bench, grabbing the barbell positioned on two fixed lateral supports. The pull phase started with both elbows in full extension. After that, it was requested to the athlete the maximum effort to reach with the barbell the lower part of the bench (the thickness of the bench from the top of the padding to the bottom of the bench was 8 cm) without lifting the chest from the horizontal plane. Furthermore, during the pulling action, the athletes were not allowed to use their lower limbs to hold on to the bench. Conversely, during the BP, the subject was supine on the bench with their head supported. The barbell initially positioned at the same chest level (resting on fixed supports) was pushed upward as fast as possible up to the maximum extension. The subjects were not allowed to bounce the barbell off the chest or lift the shoulders or trunk off the bench. Only the concentric actions (pushing for BP and pulling for PBP) were assessed in the present study with a linear encoder. During each test, the athletes observed 4 minutes of passive recovery time for each lifted load. The increasing loads’ lift was selected in 20–40–60–80–100% of 1RM for each athlete. The test of 1RM was carried out with an accuracy of 5 kg.
Kayak sprint test
Each KST trial, performed in a single session, in calm water with no influence of currents and with absent or negligible wind velocity conditions, was preceded by a standard warm-up phase where the kayaker performed 10 min of continuous paddling at moderate pace velocity followed by five trials of 50 m at increasing velocity (near to maximum velocity), observing 3 min of rest between each sprint trial.
The KST consists of three trials of 100 m each where the first 50 m were covered increasing velocity gradually up to maximum and performing the last 50 m at all-out pace velocity. Between the trials, the athletes observed 5 min of rest. The velocity was measured by the E-Kayak system (Bonaiuto et al., 2020), which was placed behind the paddler’s seat with the GPS antenna positioned over the boat to obtain the best signal strength. The best sprint performance was selected for statistical analysis.
Statistical analysis
The statistical analysis was performed using SPSS software version 20.0 (SPSS, Chicago, I). The normality of each variable was initially tested with the Shapiro Wilk test, and all the variables presented a normal distribution. Standard statistical methods were used to calculate the mean values, the standard deviations (SD), and the 95% confidence intervals for the mean (95% CI). In order to verify the correlation between maximum power, one RM, and kinematic parameters of sprint kayak (maximum velocity, stroke frequency), the Pearson product-moment correlation coefficient (r) was used. The repeated measure ANOVA (between-subjects factor) was used to evaluate the differences between BP and PBP exercises. Bonferroni corrected post-hoc analysis with paired measure was used. Statistical significance was accepted at p < 0.05.
Results
The repeated measure (between-subjects factor) showed a significant difference between the values of power and velocity in PBP and BP for loads ranging from 20 to 100% of one RM, as reported in Table 1.
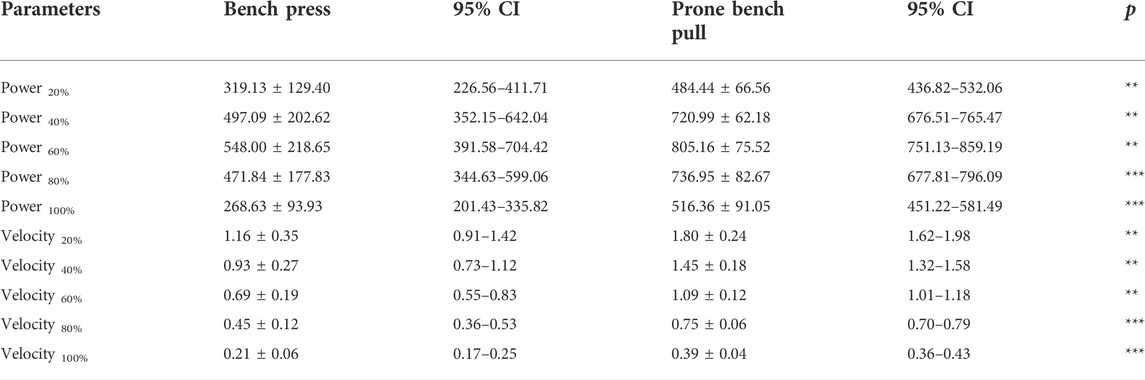
TABLE 1. Comparison between values of Power (W) and Velocity (m/s) expressed during BP and PBP. All values are mean ± SD (95% Confidence Interval). Significant difference (between subject) as reported for p < 0.05 (*), for p < 0.01 (**) and for p < 0.001 (***).
The linear regression between the muscle Pmax expressed in the PBP and the average velocity during the all-out pace 50 m KST has shown a close relationship with r = 0.80 and p < 0.01 (Figure 1; Table 2), while the correlation with stroke frequency shows an r = 0.61 and p < 0.05 (Table 2).
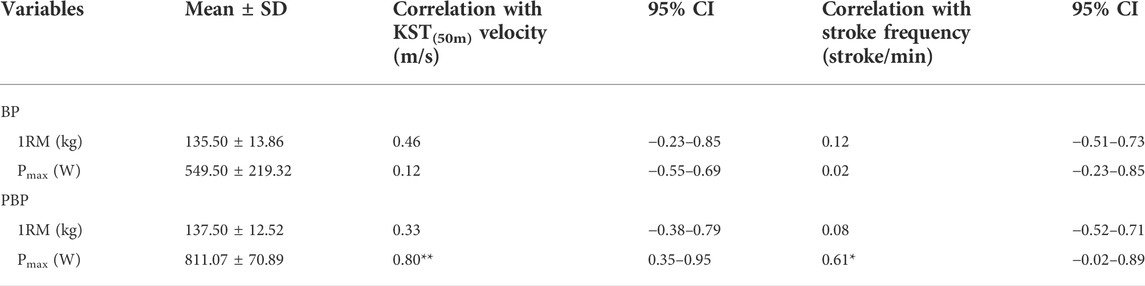
TABLE 2. Dynamic parameters (1 RM and Pmax obtained for each athlete) expressed at PBP and BP in correlation with kinematic parameters observed on KTS. All values are mean ± SD. Correlation (95% Confidence Interval) is reported p < 0.05 (*) and p < 0.01 (**).
Conversely, a poor correlation has been found between the Pmax in BP exercises and the velocity measured during KST with r = 0.12 and p = 0.74 (Figure 2; Table 2).
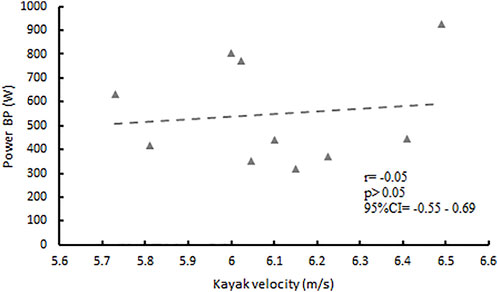
FIGURE 2. Correlation between Pmax (W) expressed at BP, and kayak velocity (m/s) reached on KTS sprint.
In contrast to the Pmax, the 1RM showed a poor or no significant correlation with the average velocity and paddle stroke frequency during the KST (Table 2).
Discussion
Both the power-load and velocity-load relationships, carried out by this study, respectively maintain the same quadratic and linear trend (Jaric, 2015; Sreckovic et al., 2015), such as those observed in the leg or arm extensors muscles during the use of isotonic (Bosco et al., 1995) or isokinetic devices (Perrine and Edgerton, 1978) or ballistic movements (Bosco and Komi, 1979; Pearson et al., 2009; Sánchez-Medina et al., 2014). In accordance with previous studies, in BP exercise the load to perform maximum power was 60% of 1RM (Izquierdo et al., 1999, 2002; Cronin et al., 2001). Conversely, in the PBP, our findings (60% of one RM) show values less than those reported in the literature (70–80% of 1RM) (Pearson et al., 2009; Sánchez-Medina et al., 2014).
The resultant curves for PBP and BP exercises show a significant difference between the kinematic and dynamic parameters for each lifted-up load considered in this study (Table 1). Probably, these differences in results could be associated with the different muscular kinetic chains involved in both bench lift test performances (Costill et al., 1976). In fact, from an anatomical point of view, the muscles involved as prime movers in the PBP exercises (i.e., latissimus dorsi, biceps brachialis, and brachialis) are composed of longer muscle fibers with a reduced angle relative to the force-generating axis (pennation angle) with a consequent faster muscle contraction than those involved as prime movers in the BP exercises (i.e., pectoralis major and triceps brachialis) which are characterized by shorter fibers and a greater pennation angles (Lieber and Fridén, 2000; Pearson et al., 2009). This allows generating more force as a consequence of slower muscle contraction velocity. Considering the muscle’s involvement during water paddle performance, Logan and Holt (1985) analyzed, in addition to the upper, lower limbs, and pelvis musculature, the detailed activation of back muscles during each subphase of the paddle stroke cycle. Subsequently, Trevithick et al. (2007) showed that the supraspinatus, the upper trapezius, the latissimus dorsi, serratus anterior, and the rhomboid major show a consistent activity pattern during kayak stroke. Moreover, it has been observed that the activity of the latissimus dorsi increases during the pull phase in water and reaches its peak during the following intermediate phase, confirming its role as a prime mover muscle during the in-water phase of the paddle stroke (Yoshio et al., 1974; Trevithick et al., 2007; Fleming et al., 2012). Based on these considerations, the PBP exercise seems more specific than BP ones relative to the technical paddle gesture (Sánchez-Medina et al., 2014).
Several studies have verified a positive correlation between strength profile and kayak performance, using isometric strength tests performed in BP and PBP and kayak ergometer performance (Lum and Aziz, 2020; Petrović et al., 2021). Nevertheless, these studies have not considered other relevant dynamic and kinematic parameters involved in both bench lift exercises and flatwater kayak performances; in contrast to the studies mentioned above, our findings have shown a poor correlation, for each bench lift exercise, between the maximum dynamic strength (1RM) and the maximum kayak velocity (Table 2). Conversely, only the PBP maximum power is significantly correlated with the maximum kayak velocity performance (r = 0.80; p < 0.01) (Figure 1) and the related stroke frequency (r = 0.61; p < 0.05) (Table 2). Probably, the differences found between this study and the previous ones (Lum and Aziz, 2020; Petrović et al., 2021) could be due to the different dry-land test protocols performed (dynamic Vs. isometric) and kayak test performance (50 m sprint flatwater kayak Vs. kayak ergometer). On the contrary, the data obtained from the BP exercise show a poor correlation (Figure 2; Table 2) not only between 1RM but also with the Pmax and the kinematic parameters of the KST analyzed, showing the scant specificity with the biomechanical parameters and the muscular kinetic chains involved during the paddle stroke.
Differently, in agreement with the principle of specificity and training monitoring (Sale and MacDougall, 1981), only the Pmax developed in the PBP exercise is coherent with the technical paddle gesture (Jackson et al., 1992; Tzabiras et al., 2010; Gomes et al., 2015a). Thus, in accordance with other factors such as paddling techniques, athlete-canoe interactions, and environmental conditions, the maximal muscle power output of the upper limbs seems to play an essential role as a limiting factor of the flatwater kayak performance.
Limitations
This study has some limitations related to the kayak sprint distance considered and, even though negligible, the exact knowledge of environmental parameters (wind velocity, water temperature, and current velocity). Furthermore, the lack of reliability data due to the trial period being too close to the start of international competitions which, given the observational nature of this study and the high reliability observed for this test in other studies, should not significantly affect the observed differences in both bench tests. Finally, only two upper limb exercises and no lower limb exercises were considered.
Conclusion
In conclusion, the PBP exercise seems to show a superior biomechanical and neuromuscular coherence with technical paddle gestures than BP.
For opposite reasons, the latter exercise is advisable as a complement to the conditioning of the antagonist’s muscles due to their synergistic role played during the aerial phase of the counter-lateral arm during the propulsive water phase of the paddling.
Future studies must investigate the effect of PBP and BP on 200–500 and 1000 m. Moreover, to understand if a power-based training rather than maximal force training before the competitions could be helpful in the kayak performance improvement.
Data availability statement
The raw data supporting the conclusion of this article will be made available by the authors, without undue reservation.
Ethics statement
The studies involving human participants were reviewed and approved by Internal Research Board of “Tor Vergata” University of Rome. The patients/participants provided their written informed consent to participate in this study.
Author contributions
CR, GA, and EP contributed to the developed designed experimental plan. CR, GG, and SL, performed and analyzed the test. GA, GG, and VB contribute to the interpretation of the data. CR, AA, and NL wrote and edited the manuscript. AB, AA, and EP assisted in drafts and final version of the manuscript. All authors read and approved the final manuscript.
Conflict of interest
The authors declare that the research was conducted in the absence of any commercial or financial relationships that could be construed as a potential conflict of interest.
Publisher’s note
All claims expressed in this article are solely those of the authors and do not necessarily represent those of their affiliated organizations, or those of the publisher, the editors and the reviewers. Any product that may be evaluated in this article, or claim that may be made by its manufacturer, is not guaranteed or endorsed by the publisher.
References
Aitken D. A., Neal R. J. (1992). An on-water analysis system for quantifying stroke force characteristics during kayak events. J. Appl. Biomechanics 8, 165–173. doi:10.1123/ijsb.8.2.165
Akca F., Muniroglu S. (2008). Anthropometric – somatotype and strength profiles and on–water performance in Turkish elite kayakers. Int. J. Appl. Sport Sci. 20, 22–34.
Baker J. (2012). “Biomechanics of paddling,” in ISBS - Conference Proceedings Archive, Melbourne, VIC, Australia, March 4, 2021. Available at: https://ojs.ub.uni-konstanz.de/cpa/article/view/5171.
Begon M., Colloud F., Sardain P. (2010). Lower limb contribution in kayak performance: Modelling, simulation and analysis. Multibody Syst. Dyn. 23, 387–400. doi:10.1007/s11044-010-9189-8
Bielik V., Lendvorskỳ L., Lengvarskỳ L., Lopata P., Petriska R., Pelikánová J., et al. (2017). Road to the olympics: Physical fitness of medalists of the canoe sprint junior European and world championship events over the past 20 years. J. Sports Med. Phys. Fit. 58, 768–777. doi:10.23736/S0022-4707.17.07380-7
Bjerkefors A., Tarassova O., Rosén J. S., Zakaria P., Arndt A. (2018). Three-dimensional kinematic analysis and power output of elite flat-water kayakers. Sports Biomech. 17, 414–427. doi:10.1080/14763141.2017.1359330
Bonaiuto V., Gatta G., Romagnoli C., Boatto P., Lanotte N., Annino G., et al. (2020). A pilot study on the e-kayak system: A wireless daq suited for performance analysis in flatwater sprint kayaks. Sensors (Basel) 20, E542. doi:10.3390/s20020542
Bosco C., Belli A., Astrua M., Tihanyi J., Pozzo R., Kellis S., et al. (1995). A dynamometer for evaluation of dynamic muscle work. Eur. J. Appl. Physiol. Occup. Physiol. 70, 379–386. doi:10.1007/bf00618487
Bosco C., Komi P. v. (1979). Potentiation of the mechanical behavior of the human skeletal muscle through prestretching. Acta Physiol. Scand. 106, 467–472. doi:10.1111/j.1748-1716.1979.tb06427.x
Costill D. L., Daniels J., Evans W., Fink W., Krahenbuhl G., Saltin B., et al. (1976). Skeletal muscle enzymes and fiber composition in male and female track athletes. J. Appl. Physiol. 40, 149–154. doi:10.1152/jappl.1976.40.2.149
Cronin J., McNair P. J., Marshall R. N. (2001). Developing explosive power: A comparison of technique and training. J. Sci. Med. Sport 4, 59–70. doi:10.1016/S1440-2440(01)80008-6
Fleming N., Donne B., Fletcher D., Mahony N. (2012). A biomechanical assessment of ergometer task specificity in elite flatwater kayakers. J. Sports Sci. Med. 11, 16–25.
García-Pallarés J., Sánchez-Medina L., Carrasco L., Díaz A., Izquierdo M. (2009). Endurance and neuromuscular changes in world-class level kayakers during a periodized training cycle. Eur. J. Appl. Physiol. 106, 629–638. doi:10.1007/s00421-009-1061-2
Gomes B. B., Conceição F. A. V., Pendergast D. R., Sanders R. H., Vaz M. A. P., Vilas-Boas J. P., et al. (2015a). Is passive drag dependent on the interaction of kayak design and paddler weight in flat-water kayaking? Sports Biomech. 14, 394–403. doi:10.1080/14763141.2015.1090475
Gomes B. B., Ramos N. V., Conceição F. A., Sanders R. H., Vaz M. A., Vilas-Boas J. P., et al. (2015b). Paddling force profiles at different stroke rates in elite sprint kayaking. J. Appl. Biomech. 31, 258–263. doi:10.1123/jab.2014-0114
Hamano S., Ochi E., Tsuchiya Y., Muramatsu E., Suzukawa K., Igawa S., et al. (2015). Relationship between performance test and body composition/physical strength characteristic in sprint canoe and kayak paddlers. Open Access J. Sports Med. 6, 191–199. doi:10.2147/OAJSM.S82295
Izquierdo Ibañez, Gorostiaga Garrues, Zúñiga Antón, GarruesM. , ZunigA A., Anton A., et al. (1999). Maximal strength and power characteristics in isometric and dynamic actions of the upper and lower extremities in middle-aged and older men: Strength and power and ageing. Acta Physiol. Scand. 167, 57–68. doi:10.1046/j.1365-201x.1999.00590.x
Izquierdo M., Häkkinen K., Gonzalez-Badillo J. J., Ibáñez J., Gorostiaga E. M. (2002). Effects of long-term training specificity on maximal strength and power of the upper and lower extremities in athletes from different sports. Eur. J. Appl. Physiol. 87, 264–271. doi:10.1007/s00421-002-0628-y
Jackson P., Locke N., Brown P. (1992). “The hydrodynamics of paddle propulsion,” in 11th Australasian Fluid Mechanics Conference, Hobart, 14-18 December 1992, 1197–1200.
Jackson P. S. (1995). Performance prediction for Olympic kayaks. J. Sports Sci. 13, 239–245. doi:10.1080/02640419508732233
Jaric S. (2015). Force-velocity relationship of muscles performing multi-joint maximum performance tasks. Int. J. Sports Med. 36, 699–704. doi:10.1055/s-0035-1547283
Kendal S. J., Sanders R. H. (1992). The technique of elite flatwater kayak paddlers using the wing paddle. J. Appl. Biomechanics 8, 233–250. doi:10.1123/ijsb.8.3.233
Lieber R. L., Fridén J. (2000). Functional and clinical significance of skeletal muscle architecture. Muscle & Nerve 23, 1647–1666. doi:10.1002/1097-4598(200011)23:11<1647:AID-MUS1>3.0.CO;2-M
Liow D. K., Hopkins W. G. (2003). Velocity specificity of weight training for kayak sprint performance. Med. Sci. Sports Exerc. 35, 1232–1237. doi:10.1249/01.MSS.0000074450.97188.CF
Logan S. M., Holt L. E. (1985). Sports Performance Series: The flatwater kayak stroke. Natl. Strength Cond. Assoc. J. 7, 4. doi:10.1519/0744-0049(1985)007<0004:tfks>2.3.co;2
Lum D., Aziz A. R. (2020). Relationship between isometric force–time characteristics and sprint kayaking performance. Int. J. Sports Physiol. Perform. 16, 474–479. doi:10.1123/ijspp.2019-0607
Mann R. V., Kearney J. T. (1980). A biomechanical analysis of the Olympic-style flatwater kayak stroke. Med. Sci. Sports Exerc. 12, 183–188. doi:10.1249/00005768-198023000-00010
McKean M. R., Burkett B. J. (2014). The influence of upper-body strength on flat-water sprint kayak performance in elite athletes. Int. J. Sports Physiol. Perform. 9, 707–714. doi:10.1123/ijspp.2013-0301
McKean M. R., Burkett B. (2010). The relationship between joint range of motion, muscular strength, and race time for sub-elite flat water kayakers. J. Sci. Med. Sport 13, 537–542. doi:10.1016/j.jsams.2009.09.003
Michael J. S., Rooney K. B., Smith R. (2008). The metabolic demands of kayaking: A review. J. Sports Sci. Med. 7, 1–7.
Michael J. S., Smith R., Rooney K. B. (2009). Determinants of kayak paddling performance. Sports Biomech. 8, 167–179. doi:10.1080/14763140902745019
Millward A. (1987). A study of the forces exerted by an oarsman and the effect on boat speed. J. Sports Sci. 5, 93–103. doi:10.1080/02640418708729769
Nilsson J. E., Rosdahl H. G. (2016). Contribution of leg-muscle forces to paddle force and kayak speed during maximal-effort flat-water paddling. Int. J. Sports Physiol. Perform. 11, 22–27. doi:10.1123/ijspp.2014-0030
Pearson S. N., Cronin J. B., Hume P. A., Slyfield D. (2009). Kinematics and kinetics of the bench-press and bench-pull exercises in a strength-trained sporting population. Sports Biomech. 8, 245–254. doi:10.1080/14763140903229484
Pendergast D., Mollendorf J., Zamparo P., Termin A., Bushnell D., Paschke D., et al. (2005). The influence of drag on human locomotion in water. Undersea Hyperb. Med. 32, 45–57.
Perrine J. J., Edgerton V. R. (1978). Muscle force-velocity and power-velocity relationships under isokinetic loading. Med. Sci. Sports 10, 159–166.
Petrović M., García Ramos A., Janićijević D., Pérez Castilla A., Knezevic O., Mirkov D., et al. (2021). Force-velocity profile of competitive kayakers: Evaluation of a novel single kayak stroke test. J. Hum. Kinet. 80, 49–59. doi:10.2478/hukin-2021-0100
Sale D., MacDougall D. (1981). Specificity in strength training: A review for the coach and athlete. Can. J. Appl. Sport Sci. 6, 87–92.
Sánchez-Medina L., González-Badillo J. J., Pérez C. E., Pallarés J. G. (2014). Velocity- and power-load relationships of the bench pull vs. Bench press exercises. Int. J. Sports Med. 35, 209–216. doi:10.1055/s-0033-1351252
Sreckovic S., Cuk I., Djuric S., Nedeljkovic A., Mirkov D., Jaric S., et al. (2015). Evaluation of force-velocity and power–velocity relationship of arm muscles. Eur. J. Appl. Physiol. 115, 1779–1787. doi:10.1007/s00421-015-3165-1
Trevithick B. A., Ginn K. A., Halaki M., Balnave R. (2007). Shoulder muscle recruitment patterns during a kayak stroke performed on a paddling ergometer. J. Electromyogr. Kinesiol. 17, 74–79. doi:10.1016/j.jelekin.2005.11.012
Tzabiras G. D., Polyzos S. P., Sfakianaki K., Diafas V., Villiotis A. D., Chrisikopoulos K., et al. (2010). Experimental and numerical study of the flow past the Olympic class K-1 flat water racing kayak at steady speed. Sport J. 13, 1–15.
Ualí I., Herrero A. J., Garatachea N., Marín P. J., Alvear-Ordenes I., García-López D., et al. (2012). Maximal strength on different resistance training rowing exercises predicts start phase performance in elite kayakers. J. Strength Cond. Res. 26, 941–946. doi:10.1519/JSC.0b013e31822e58f8
Winchcombe C. E., Binnie M. J., Doyle M. M., Hogan C., Peeling P. (2019). Development of an on-water graded exercise test for flat-water sprint kayak athletes. Int. J. Sports Physiol. Perform. 14, 1244–1249. doi:10.1123/ijspp.2018-0717
Yoshio H., Takagi K., Kumamoto M., Ito M., Ito K., Yamashita N., et al. (1974). Electromyographic study of kayak paddling in the paddling tank. Jpn. J. Phys. Educ. Health. Sport. Sci. 18, 191–198. doi:10.5432/jjpehss.kj00003392373
Keywords: optimal load, power-based training, sprint performance, propulsive force, resistance training
Citation: Romagnoli C, Gatta G, Lamouchideli N, Bianco A, Loddo S, Alashram AR, Bonaiuto V, Annino G and Padua E (2022) Specificity of weightlifting bench exercises in kayaking sprint performance: A perspective for neuromuscular training. Front. Physiol. 13:898468. doi: 10.3389/fphys.2022.898468
Received: 17 March 2022; Accepted: 01 July 2022;
Published: 22 July 2022.
Edited by:
Amador García Ramos, University of Granada, SpainReviewed by:
Dustin J. Oranchuk, Auckland University of Technology, New ZealandDanny Lum, Sport Singapore, Singapore
Copyright © 2022 Romagnoli, Gatta, Lamouchideli, Bianco, Loddo, Alashram, Bonaiuto, Annino and Padua. This is an open-access article distributed under the terms of the Creative Commons Attribution License (CC BY). The use, distribution or reproduction in other forums is permitted, provided the original author(s) and the copyright owner(s) are credited and that the original publication in this journal is cited, in accordance with accepted academic practice. No use, distribution or reproduction is permitted which does not comply with these terms.
*Correspondence: Giuseppe Annino, Z19hbm5pbm9AaG90bWFpbC5jb20=