- 1Department of Pulmonary and Critical Care Medicine, Shengjing Hospital of China Medical University, Shenyang, China
- 2Department of Orthopedics, First Hospital of China Medical University, Shenyang, China
Climate change, environmental pollution, and virus epidemics have sharply increased the number of patients suffering from respiratory diseases in recent years. Prolonged periods of illness and drug use increase the occurrence of complications in these patients. Osteoporosis is the common bone metabolism disease with respiratory disturbance, which affects prognosis and increases mortality of patients. The problem of osteoporosis in patients with respiratory diseases needs more attention. In this review, we concluded the characteristics of osteoporosis in some respiratory diseases including COPD, asthma, COVID-19, tuberculosis, and lung cancer. We revealed that hypoxia was the common pathogenesis of osteoporosis secondary to respiratory diseases, with malnutrition and corticosteroid abuse driving the progression of osteoporosis. Hypoxia-induced ROS accumulation and activated HIF-1α lead to attenuated osteogenesis and enhanced osteoclastogenesis in patients with respiratory diseases. Tuberculosis and cancer also invaded bone tissue and reduced bone strength by direct infiltration. For the treatment of osteoporosis in respiratory patients, oral-optimized bisphosphonates were the best treatment modality. Vitamin D was a necessary supplement, both for calcium absorption in osteogenesis and for improvement of respiratory lesions. Reasonable adjustment of the dose and course of corticosteroids according to the etiology and condition of patients is beneficial to prevent the occurrence and development of osteoporosis. Additionally, HIF-1α was a potential target for the treatment of osteoporosis in respiratory patients, which could be activated under hypoxia condition and involved in the process of bone remodeling.
Introduction
Osteoporosis is a common disease characterized by decreasing bone mass, increasing bone fragility, and weakening bone microarchitecture (Kimmel et al., 2022). Under physiological conditions, bone tissue is in a dynamic balance between the bone formation effect of osteoblasts and the bone resorption effect of osteoclasts (Teissier et al., 2022). The direct pathogenesis of osteoporosis is that the weakened bone formation is difficult to compensate for the reduced bone mass of bone resorption (Yang et al., 2022). With aging, the prevalence of osteoporosis is increasing year by year (Dong et al., 2022). Complications of osteoporosis are an important factor threatening the health of patients. The incidence of osteoporotic fractures is close to ten percent, and the reduction in life expectancy of patients with osteoporotic fractures exceeds that of cancer in some regions (Johnell and Kanis, 2006; Wang et al., 2021a). The weak awareness of people’s regular physical examination and the insidious characteristics of osteoporosis have made this phenomenon worse. Early diagnosis and prevention of osteoporosis in high-risk groups will contribute to improving this public health problem.
Osteoporosis in high-risk groups mainly includes postmenopausal women with primary osteoporosis and diabetic patients with secondary osteoporosis (Cakmak et al., 2015; Ramchand et al., 2021). Endocrinology and orthopedic doctors paid more attention to these two groups of people to improve treatment efficiency. However, another high-risk group is attracting the attention of clinicians and researchers. Bone mineral density in patients with respiratory disease was significantly lower than that in the normal population (Chiu et al., 2021; Yadav et al., 2021). Patients with severe chronic obstructive pulmonary disease (COPD) were at four times the risk to develop osteoporosis (Bitar et al., 2021). The fracture ratio increased significantly in influenza patients after discharge (Axelsson et al., 2022). Drug application in the asthma treatment process also had a potential influence on the bone mass of patients (Chalitsios et al., 2022). These clinical data indicated that the patients with respiratory diseases had a high risk of getting osteopenia and osteoporotic fracture. It is essential to monitor bone mass changes in this high-risk group to prevent the occurrence of osteoporosis complications.
Hypoxia and drug abuse were the main causes of respiratory disease-induced osteoporosis (Zhuang et al., 2020; Sood et al., 2021). In recent years, the immune response induced in infected patients by the COVID-19 epidemic is also considered to be an important factor in enhancing RANKL-mediated bone resorption (Yang et al., 2021). Air pollution, smoking, viral infections, and cancer play a role in increasing the number of people suffering from respiratory diseases. Bone quality changes and bone mass detection in this population need more attention. Osteoporosis secondary to respiratory disease is becoming a research hotspot. In this review, we aimed to conclude the pathogenesis and characteristics of osteoporosis secondary to various respiratory diseases, reveal the influence of hypoxia on bone metabolism, and provide the opinion on the bone mass maintenance in treatment.
Dyspnea and Hypoxia
Dyspnea was the common essential feature of respiratory diseases. A hypoxic state due to pulmonary ventilation and ventilation dysfunction was the fundamental cause of bone metabolism disorder in patients with respiratory diseases (Evans and Morgan, 2014).
Decreasing osteogenic marker expression could be observed under osteogenic induction in the hypoxia state (Teh et al., 2021). Hypoxia culture also promoted osteoclast differentiation by activating the NF-κB ligand and macrophage colony-stimulating factor (Kondo et al., 2021). ROS was a necessary means of hypoxia to cause pathological changes. Stress response enhancement and antioxidant function reduction occurred in cells under hypoxia conditions, leading to the production of ROS (Estrada-Cárdenas et al., 2022). The imbalance of bone metabolism caused by hypoxia was also due to the accumulation of ROS (Shao et al., 2022). ROS suppressed osteogenesis and mineralization of bone marrow mesenchymal stem cells (Huang et al., 2012). Meanwhile, ROS could initiate the mitochondrial apoptosis pathway of osteoblasts by increasing the expression of cleaved caspase-3 and the secretion of cytochrome c (Cao et al., 2015). Both activity and differentiation of osteoblasts were suppressed by ROS produced by hypoxia. As for osteoclasts, the programmed death of osteoclasts was significantly reduced under hypoxia conditions (Ni et al., 2021). Intramitochondrial ROS was associated with the differentiation of osteoclasts induced by hypoxia (Srinivasan et al., 2010). ROS participated in the autocrine process of osteoclasts by increasing the expression of cathepsin K and tartrate-resistant alkaline phosphatase to promote osteoclastogenesis (Srinivasan and Avadhani, 2007). Additionally, hypoxia led to inflammatory bone imbalance (Doi et al., 2022). Mitochondria were ineffective, and the glucose metabolism was dominated by anaerobic respiration under hypoxic conditions. The mechanism of hypoxia-induced inflammation was mainly ROS accumulation and lactate production, which were negative for bone formation (Hou et al., 2017).
HIF-1α was a necessary factor-mediated hypoxia-induced osteoporosis (Miyamoto, 2016). The effect of HIF-1α on bone metabolism was bidirectional (Song et al., 2020). On the one hand, HIF-1α assisted bone tissue had to adapt to the hypoxia microenvironment (Camacho-Cardenosa et al., 2020; Wang et al., 2022). HIF-1α overexpression was positive to prevent osteoblast apoptosis under pathological conditions (Xu et al., 2021). Activation of the HIF-1α signaling pathway contributed to bone formation (Jiang et al., 2020). On the other hand, excessive hypoxic stimulation was an activator of bone resorption. HIF-1α could increase the expression of RANKL (Zhu et al., 2019). Also, HIF-1α was associated with the activation of nuclear factor-activated T cells c1 (NFAc1) that was positive in osteoclast differentiation (Xiao et al., 2020). For treatment, intermittent hypoxic therapy could activate the adaptive process of hypoxia mediated by HIF-1α, which was helpful for the treatment of hypoxia-induced osteoporosis (Bergholt et al., 2021; Chen et al., 2021). Precisely, regulating the physiological function of HIF-1α in osteoblasts and osteoclasts might be a potential means to maintain bone homeostasis.
COPD
COPD is the respiratory disease most closely associated with the development of osteoporosis. A previous study indicated that osteopenia or osteoporosis occurred in 50–70% COPD patients (Pobeha et al., 2010). Bone mineral density decreased significantly causing the prevalence of osteoporosis or vertebral compression fracture achieving 24.6% in COPD patients (Kakoullis et al., 2022), and 43.7% COPD patients had insufficiency of vertebral deformity and soft tissue lesions (Kupeli et al., 2021). Also, restricted thoracic diastolic function in osteoporosis patients suppressed respiratory function and aggravated COPD (Chen et al., 2018a). In animal experiments, the pulmonary emphysema-mediated COPD mouse model showed an obvious decrease of bone mass, which demonstrated the direct link between COPD and osteoporosis (Tsukamoto et al., 2019).
The mechanism of osteoporosis secondary to COPD was complicated. Chronic hypoxia, malnutrition, and glucocorticoid application were thought to be triggers for decreased bone mineral density (Fouda et al., 2017; Johansen et al., 2018; Caramori et al., 2019). Based on bone metabolism monitoring in COPD patients, researchers revealed that these patients had a lower capacity for bone formation with the increasing COPD severity (Tsukamoto et al., 2020). After COPD induction, the bone mineral content and femoral strength were less (Shea et al., 19852003). Percent forced expiratory volume in 1 s (%FEV1) was moderately correlated with the osteogenic markers including alkaline phosphatase and procollagen type 1 N-terminal propeptide (P1NP). With the deterioration of lung function and exacerbations of COPD, vitamin D decreased significantly (Calle Rubio et al., 2022). Vitamin D deficiency was also a potential factor of COPD-induced osteoporosis by inhibiting the absorption of calcium to suppress bone matrix formation. Kokturk et al. (2018) concluded an effective prediction method of osteoporosis diagnosis in COPD based on these osteogenic markers and revealed that Vitamin D and osteocalcin were more sensitive indicators (Masik et al., 2021). In terms of bone resorption, a study showed that activated neutrophils increased the contents of RANKL in COPD patients’ blood and COPD patients had a higher concentration of CrossLaps, both of which represented increased bone loss (Kochetkova et al., 2002; Hu et al., 2017). The pathological state of oxidative stress and inflammation in COPD patients was the enhancer of osteoclastogenesis (Stanojkovic et al., 2013). Inflammatory mediators IL-2 and IFNγ were inversely correlated with bone metabolism in COPD (Bon et al., 2010). Skeletal muscle dysfunction mediated by inflammation was also an important factor in COPD-induced osteoporosis (Zhang and Sun, 2021). High expression of IL-6 in COPD patients disrupted muscle–bone crosstalk and inhibited the secretion of irisin from muscle, which was positive for bone formation.
Among the patients with COPD, smokers are a more noteworthy group. Lung-specific changes in smokers were associated with the morbidity and mortality of COPD-induced osteoporotic fracture (Bon et al., 2020). Smoking mediated tracheal deformity, and emphysema increased the decrease of bone mineral density (Pompe et al., 2016). Therefore, it is necessary to detect bone mass in COPD patients, especially smokers. Unfortunately, previous studies had reported the drug treatment data of many secondary osteoporosis cases but COPD (Lehouck et al., 2010; Okazaki, 2015). Osteoporosis secondary to COPD needs more attention. Effective prevention of bone loss in patients with COPD contributed to the treatment of COPD and osteoporosis.
Asthma
Asthma is an inflammatory respiratory disease characterized by airway hyperresponsiveness. Compared with COPD, asthma patients should scientifically detect the development of osteoporosis because asthma-induced dyspnea combined with osteoporosis could easily cause shock in patients. The mechanism of asthma-associated bone mass loss could be divided into asthma-induced osteoporosis and corticosteroid-induced osteoporosis, which was dominated by the latter. The direct effect of asthma leading to osteoporosis is mainly due to the occurrence of inflammation. The main pathogenesis of asthma was neutrophil-mediated inflammation. TNF-α participated in the process of neutrophil migration, which was associated with a decrease in bone mineral density (Shmarina et al., 2013). TNF-α was an inhibitor of osteogenesis and an activator of osteoclastogenesis (Han et al., 2022; Pramusita et al., 2022). TNF-α disrupted the balance of RANKL/OPG, resulting in the enhancement of bone resorption rather than formation (Wan et al., 2016). Additionally, IL-6 was also regarded as an important inflammatory factor involved in neutrophil-inflammation-mediated osteoporosis in asthma patients (Leng et al., 2005). Overexpression of IL-6 inhibited the differentiation of osteoblasts and obviously increased the expression of RANKL to increase osteoclast activity (El Kholy et al., 2018; Di Pompo et al., 2021).
Clinicians paid more attention to the problem that asthma patients were susceptible to osteoporosis and the prescription rates of bone mineral density testing and bisphosphonates therapy for osteoporosis in asthma patients were increasing (Soen et al., 2021). Enough physical activity and calcium intake were important for these patients. Meanwhile, they should rationally choose the treatment drugs according to the changes of blood and urinary calcium levels (Picado and Luengo, 1996). Bisphosphonates were the first choice for most of the asthma patients with decreased bone mass (Chalitsios et al., 2020). Vitamin D supplementation was also an effective method to prevent asthma-induced osteoporosis (Awadh et al., 2003).
Corticosteroid-Induced Osteoporosis During COPD and Asthma Treatment
Osteoporosis commonly occurred during the treatment of COPD and asthma with corticosteroids (Chen et al., 2018b; Sood et al., 2021). Oral corticosteroids increased the risk of osteoporosis by 1.56–3.38 times in patients, and the risk would increase significantly with the cumulative dose of corticosteroids (Osteoporosis and fracture risk with, 2021). Corticosteroids decreased the trabecular bone score of the lumbar spine and hip, which increased the risk of fracture (Sandru et al., 2020). Some studies revealed that in nearly 50% of patients undergoing oral corticosteroid treatment exhibited risk of fractures (Chalitsios et al., 2021a). Unexpectedly, inhaled corticosteroid therapy might not appear to increase the risk of osteoporosis (Chalitsios et al., 2021b; Grosso et al., 2021).
As for corticosteroid-induced bone loss, some prospective observational studies revealed that long-term oral corticosteroids decreased the mineralization of bone tissue (Park et al., 2015). Osteocalcin was usually lowered with corticosteroid intake (Puolijoki et al., 1992; Cesana‐Nigro et al., 2019), and biosynthesis of Collagen I was also interfered by corticosteroids (Puolijoki et al., 1996). These significantly decreasing osteogenic markers also reflected the weakness of osteoblasts with corticosteroid treatment (Brabnikova Maresova et al., 2013). At the cellular level, exogenous corticosteroid would combine with its receptor on bone tissue to impair the survival of osteoblasts and inhibit apoptosis of osteoclasts (Lee et al., 2021). For cross-talk between bone cells, a high concentration of corticosteroids increased the ratio of RANKL/OPG to enhance bone resorption. Additionally, corticosteroids mainly acted on the mitochondria of osteoblasts, leading to the accumulation of reactive oxygen radicals (ROS), and induced pathological necrosis (Wu et al., 2019a; Peng et al., 2021). Excessive ROS alternated the membrane potential of mitochondria and increased the expression of mitochondrial apoptotic proteins including Bax, caspase 9, caspase 3, and cytochrome C, which decreased the activity of osteoblasts. Corticosteroids also increased tartrate-resistant acid phosphatase from 5b to hyperactivate and prolonged the lifespan of osteoclasts (Sato et al., 2019; Chen et al., 2020). Activation of the MAPK, Akt, NF-κB, and cytoplasmic 1 signaling pathways contributed to osteoclast differentiation when corticosteroid promoted RANKL to combine with RANK (Lin et al., 2021).
Application of corticosteroid in COPD and asthma patients needed to be valued to avoid the development of osteoporosis. The guidance for corticosteroid-induced osteoporosis had been provided for groups of different ages (Weare-Regales et al., 2021). People younger than 40 years old need regular bone quality testing, and those older than 40 need bone-preserving treatment. Also, the researchers attempted to optimize the dose of corticosteroids and explored the new treatment method for asthma to avoid the side effects of traditional corticosteroid therapy (González-Barcala et al., 2021; Sood et al., 2021).
COVID-19
In past studies, scientists had suggested that the development of osteoporosis is not directly related to pneumonia. However, many studies indicated that bone metabolism disorder, bone mineral density decreasing, and bone microarchitecture destruction occurred in patients infected with SARS-CoV2 during the last 3 years of the COVID-19 pandemic (Mi et al., 2021; Tsourdi et al., 2022). COVID-19 increased the fragility of bone tissue and the risk of fractures with increasing severity (Battisti et al., 2021). Thoracic bone mineral density appeared to correlate with the mortality in COVID-19 patients (Tahtabasi et al., 2021), and the mortality also increased in vertebral fracture patients with COVID-19 (di Filippo et al., 2021). Additionally, a study from Korea reported that COVID-19 vaccination also led to some endocrine diseases like osteoporosis (Ku et al., 2021). Detecting bone mineral density and preventing bone loss would contribute to increasing therapeutic effect of COVID-19 patients.
The causes of osteoporosis induced by COVID-19 was broad including inflammatory storm, home quarantine, lack of exercise, and glucocorticoid treatment (Napoli et al., 2020). SARS-CoV-2 competed for binding to and caused the deficiency of ACE2 in mesenchymal stem cells, which increased the expression of inflammatory factor TNF-α to enhance bone resorption mediated by RANK (Tao et al., 2020). Pro-inflammatory cytokines including IL-1β and IL-2 highly expressed in COVID-19 patients also involved in the process of osteoporosis. IL-1β and IL-2 were powerful cytokines to stimulate bone resorption by upregulating RANKL (Ruscitti et al., 2015; Momiuchi et al., 2021). Other highly expressed cytokines with SARS-CoV-2 infection such as IL-8 and IL-10 were associated with bone metabolism, accelerating the process of bone loss (Amarasekara et al., 2018; Carnovali et al., 2021). Also, SARS-CoV-2 infection could induce hypoparathyroidism, resulting in the disturbance of calcium and phosphorus metabolism (Elkattawy et al., 2020). Hypocalcemia and hyperphosphatemia were negative for bone homeostasis (Gittoes et al., 2020; Cheng and Kuo, 2022). Additionally, vitamin D was a crucial factor to predict the outcomes of COVID-19 and played an important role in the correlation of COVID-19 and osteoporosis (Amrein et al., 2021; Bassatne et al., 2021). COVID-associated coagulopathy interfered the interaction of Vitamin D and platelets, which drove the imbalance of bone remodeling (Salamanna et al., 2021), and the content of circulating 250-hydroxyvitamin D was lower in COVID-19 patients, which was negative for osteogenesis (Diaz-Curiel et al., 2021). Home quarantine and lack of exercise were also catalysts for the development of osteoporosis in the elderly (Kirwan et al., 2020). Reduced physical activity and variated diet habits decreased the muscle mass and weakened the protection of bone tissue. Low energy injuries increased the ratio of fractures in elderly population (Lv et al., 2020).
Significant changes appeared in the management of osteoporosis under the COVID-19 epidemic (Kong et al., 2021; Salvio et al., 2022). Clinical data demonstrated that anti-osteoporosis treatment did not increase the infective risk, severity, and mortality of COVID-19 (Atmaca et al., 2022). Oral bisphosphonates might be a good choice for osteoporosis patients with COVID-19 due to its anti-inflammation effect (Degli Esposti et al., 2021). Vitamin D supplementation was positive to prevent the occurrence and development of osteoporosis (Raisi-Estabragh et al., 2021; Ulivieri et al., 2021). In conclusion, oral medication and self-injectable anabolic agents were the mainstay of osteoporosis treatment during the COVID-19 pandemic (Sundaresh, 2020).
Pulmonary Tuberculosis
Pulmonary tuberculosis was a potential risk for the development of osteoporosis (Choi et al., 2017). Tuberculosis patients had significantly higher odds of osteoporotic fractures (Yeh et al., 2016; Chen et al., 2017). Primary pulmonary tuberculosis mainly led to malnutrition and further induced decreased bone mass in patients (Rose et al., 1999; Ishii et al., 2007). Vitamin D deficiency played an important role in tuberculosis-induced osteoporosis (Shin et al., 2015). Vitamin D receptor was regarded as a linkage between tuberculosis and osteoporosis (Roy et al., 1999). Therapeutic method targeting Vitamin D might be effective to prevent the occurrence and deterioration of osteoporosis. Additionally, tuberculosis in bone tissue was mainly secondary to pulmonary tuberculosis. Tuberculosis disrupted the soft tissue around bone first when it invaded the skeletal system (Pattamapaspong et al., 2011). Muscle infection weakened the maintenance and prevention of bone tissue. Notably, bone tuberculosis was more likely to occur in parts with heavy load and high activity, which greatly increased the risk of fractures. In microenvironment, Mycobacterium tuberculosis could increase the ratio of RANKL/OPG to inhibit the activity of osteoblasts (Zhang et al., 2014; Zhang et al., 2015). IL-1 and TNF-α secretion induced by Mycobacterium tuberculosis also involved in the disorder of bone metabolism, leading to bone mass loss (Wright and Friedland, 2004; Yi et al., 2018). As for treatment, oral calcium and Vitamin D intake were positive for improving bone mass during tuberculosis (Shin et al., 2015).
Lung Cancer
Lung cancer was the most dangerous factor of bone mineral density changes in respiratory diseases (Savula et al., 2004). Osteopenia and osteoporosis were more likely to occur in people with lung cancer (Huang et al., 2022). Among different types of lung cancer, non-small cell lung cancer was most associated with osteoporosis, and bone metastasis was also the risk factor for its negative prognosis (Rinaldi et al., 2019).
The results obtained from the serum metabolite measurement in lung cancer patients indicated decreasing of calcium and increasing of osteocalcin, leading to bone imbalance (Dumanskiy et al., 2018). Nuclear factor kappa-B mediated the effect of bone resorption enhanced by lung cancer cells (Ma et al., 2018). The BMP2 signaling and Wnt/β-catenin pathways mediated the bone invasion of lung cancer cells (Huang et al., 2021; She et al., 2021). Secondary bone tumor eroded bone substance, disrupted bone structure, and increased the risk of fractures (Niu et al., 2022; Sethakorn et al., 2022). Additionally, microRNAs might play an important role in the development of osteoporosis secondary to lung cancer (Bellavia et al., 2019). Some microRNAs provided the implantation sites for cancer cell metastasis on bone tissue, and exosomes miR-17-5p derived from non-small cell lung cancer was determined to be positive in osteoclast differentiation by targeting PTEN (Wang et al., 2021b). Osteolytic metastasis was also a great challenge for the patients with lung cancer (Wu et al., 2021a). Serum albumin decreasing threatened the homeostasis of bone tissue in these patients (Miyashita et al., 2021).
At present, low-dose chest computed tomography was verified to be effective for the diagnosis of osteoporosis in lung cancer patients (Wu et al., 2019b; Pan et al., 2020). Based on the test results, bone density was also an independent predictor of mortality (Buckens et al., 2015). For treatment, bisphosphonates were applied to treat bone cancer metastasized from the lung together with osteoporosis but with obvious side effects (Tao et al., 2018; Mbese and Aderibigbe, 2021). Osteoporosis medications were also regarded as potentially inappropriate medications for older patients with lung cancer (Ham et al., 2022). Interestingly, some anti-osteoporosis drugs had a therapeutic effect on lung cancer, which provide a reverse view for the treatment of osteoporosis in lung cancer patients (Almutairi et al., 2019; Wu et al., 2020). Also, vitamin D supplement was positive to maintain the calcium absorption for bone matrix formation (Laskowski et al., 2016). In a word, rational and effective treatment modalities with few adverse effects need to be developed further.
Environmental Exposures to Pollutants
Environmental pollution was an important factor causing the decline of respiratory system function and leading to osteoporosis. Environmental pollutants related to the development of osteoporosis mainly included the particles with diameter <2.5 μm (PM2.5) and automobile exhaust (Chen et al., 2015; Pang et al., 2021). Previous studies reported that decreased spinal bone mineral density could be observed after exposure to PM2.5 (Ranzani et al., 2020). The calcaneus quantitative ultrasound index was lower with the increase of PM2.5 (Wu et al., 2021b). PM2.5 was positively correlated with serum RANKL and the osteoclast precursor CD14 (+) CD16 (+) monocytes (Saha et al., 2016). Additionally, PM2.5 could induce the secretion of inflammatory cytokines such as TNF-α, IL-1β, and IL-6, all of which were stimulators of bone resorption (Pang et al., 2021). Additionally, vitamin D was deficient with PM2.5 exposure (Afsar et al., 2019). Disturbance of calcium absorption without the assistance of vitamin D was not conducive to bone remodeling. The contents of automobile exhaust leading to the occurrence of osteoporosis were mainly SO2 and NO2 (Liu et al., 2021; Heo et al., 2022). Long-term exposure to SO2 and NO2 increased the risk of osteoporotic fractures (Mazzucchelli et al., 2018).
The prevention of osteoporosis induced by environmental pollutants was mainly to reduce the discharge of these pollutants. Controlling the use of energy containing sulfur and nitrogen compounds will contribute to reducing their oxidation, thereby preventing conversion to PM2.5. Bone mass monitoring is also necessary for high-risk groups living near factories or highways. Additionally, reasonable supplementation of supplements such as vitamin D and calcium tablets for these populations may be an effective measure to prevent bone loss.
Discussion
As the climate changes and the environment deteriorates, the prevalence of respiratory diseases in the elderly is increasing (Aliyu and Botai, 2018; Peng et al., 2022). In recent years, the epidemic of COVID-19 is a major challenge to the barrier of the human respiratory system (Shivshankar et al., 2022). Oxygen supplement reduction and carbon dioxide accumulation caused by gas exchange efficiency decreasing disrupted the normal physiology in the patients with decreased lung function (Williamson et al., 1993). Additionally, the treatment cycle is relatively long and the probability of radical cure is extremely low for respiratory diseases compared to other diseases. Patients with respiratory disease often require prolonged clinical intervention and lifelong maintenance with medication. Therefore, pathological changes secondary to respiratory diseases and drug side effects are problems that cannot be ignored in the process of treatment.
Skeletal health is valued in the clinical management of patients with respiratory diseases. Increased bone fragility and reduced flexibility decreased thoracic mobility, resulting in the limitation of breathing movements. The formation of this vicious circle greatly reduced the quality of life and increased the mortality rate of patients. Osteoporosis was the most common bone metabolism disease occurring in the respiratory patients. Respiratory diseases had been regarded as a high-risk factor of osteoporosis development (Leslie and Morin, 2020). In our review, we concluded several respiratory diseases that were predisposed to osteoporosis and revealed their pathogenesis. Hypoxia was the common problem. The essence of various respiratory diseases was the decline of lung function, which led to the gas exchange disorder in the lungs, leaving the body in a state of hypoxia. Hypoxia not only directly destroyed bone balance by increasing intracellular ROS but also induced inflammation, which further enhanced bone resorption (Halpin, 2007; Lin et al., 2020). Tuberculosis and tumors could erode bone tissue by metastasizing and causing bone mass loss (Coleman et al., 2014; Zeng et al., 2019). Inflammation cytokines were the accelerators for the development of osteoporosis in patients with respiratory diseases (Table 1). A variety of inflammatory factors are involved in the activation of RANKL (Figure 1). Additionally, prolonged breathing disturbances led to metabolic disorders that left patients in a state of malnutrition and affected the normal remodeling function of bone tissue (Ozcakir et al., 2020). Corticosteroid application in clinical treatment was also a non-negligible factor that threatens bone health.
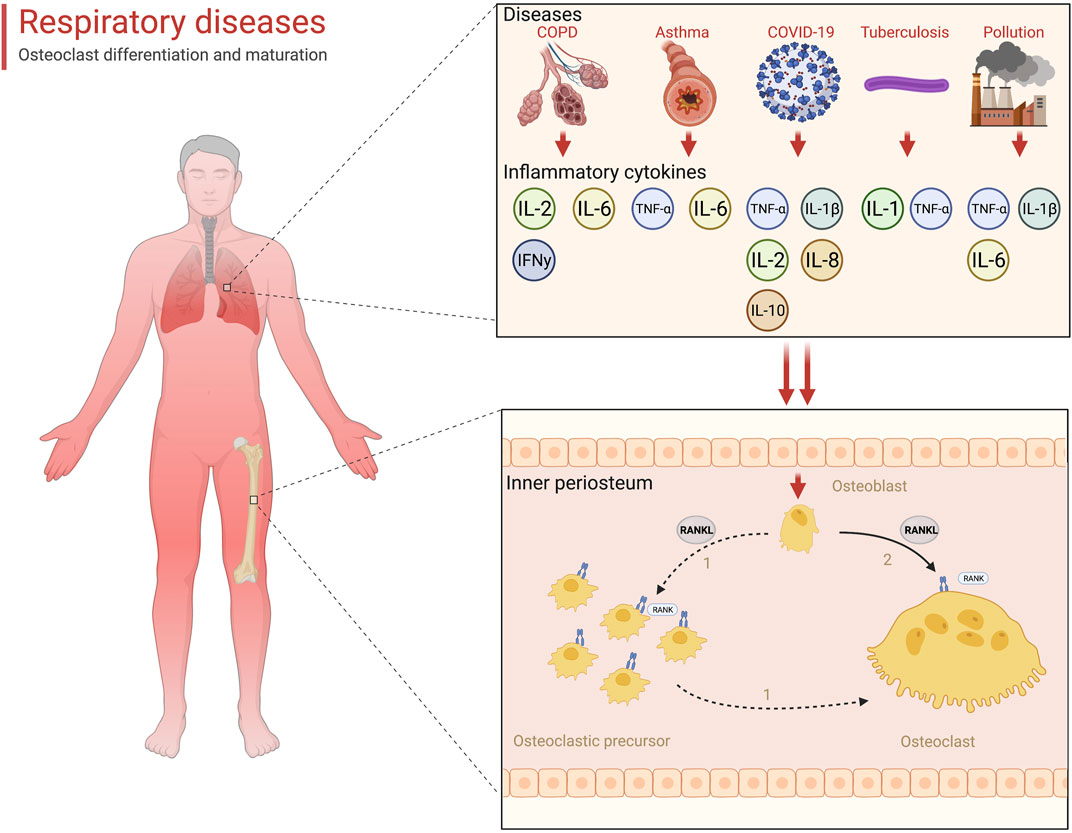
FIGURE 1. Inflammatory storm-mediated bone resorption with respiratory diseases (created with BioRender.com).
With regard to the treatment of osteoporosis in patients with respiratory diseases, clinicians had accumulated a lot of data and experience. Bisphosphonates were currently the first choice of drugs for patients with respiratory diseases complicated with osteoporosis, but side effects were also obvious. Modifications to bisphosphonates were underway to improve treatment efficacy. Rational formulation of the dose and frequency of corticosteroid use according to the characteristics and severity of diseases could help prevent the occurrence of osteoporosis. Vitamin D was an essential supplement to improve bone mass for patients with respiratory diseases. Meanwhile, HIF-1α played an important role in hypoxia-induced osteoporosis and was directly involved in the regulation of bone homeostasis, which was a potential target for the treatment of osteoporosis in respiratory patients.
Author Contributions
YM: data curation, formal analysis, methodology, and writing—original draft. SQ: investigation, methodology, software, conceptualization, and validation. RZ: funding acquisition, project administration, resources, and writing—review and editing. All authors read and approved the manuscript.
Conflict of Interest
The authors declare that the research was conducted in the absence of any commercial or financial relationships that could be construed as a potential conflict of interest.
Publisher’s Note
All claims expressed in this article are solely those of the authors and do not necessarily represent those of their affiliated organizations, or those of the publisher, the editors, and the reviewers. Any product that may be evaluated in this article, or claim that may be made by its manufacturer, is not guaranteed or endorsed by the publisher.
References
Afsar B., Elsurer Afsar R., Kanbay A., Covic A., Ortiz A., Kanbay M. (2019). Air Pollution and Kidney Disease: Review of Current Evidence. Clin. Kidney J. 12, 19–32. doi:10.1093/ckj/sfy111
Aliyu Y. A., Botai J. O. (2018). An Exposure Appraisal of Outdoor Air Pollution on the Respiratory Well-Being of a Developing City Population. J. Epidemiol. Glob. Health. 8, 91–100. doi:10.2991/j.jegh.2018.04.002
Almutairi F. M., Abd-Rabou A. A., Mohamed M. S. (2019). Raloxifene-encapsulated Hyaluronic Acid-Decorated Chitosan Nanoparticles Selectively Induce Apoptosis in Lung Cancer Cells. Bioorg. Med. Chem. 27, 1629–1638. doi:10.1016/j.bmc.2019.03.004
Amarasekara D. S., Yun H., Kim S., Lee N., Kim H., Rho J. (2018). Regulation of Osteoclast Differentiation by Cytokine Networks. Immune Netw. 18, e8. doi:10.4110/in.2018.18.e8
Amrein K., Hoffmann M., Lobmeyr E., Martucci G. (2021). Vitamin D in Critical Care: where Are We Now and what Is Next? Curr. Opin. Crit. Care 27, 378–384. doi:10.1097/mcc.0000000000000849
Atmaca A., Demirci I., Haymana C., Tasci I., Sahin I., Cakal E., et al. (2022). No Association of Anti-osteoporosis Drugs with COVID-19-Related Outcomes in Women: a Nationwide Cohort Study. Osteoporos. Int. 33, 273–282. doi:10.1007/s00198-021-06067-2
Awadh A. A., Hilleman D. E., Knezevich E., Malesker M. A., Gallagher J. C. (2003). Vitamin D Supplements: The Pharmacists' Perspective. J. Am. Pharm. Assoc. 61, e191–e201. doi:10.1016/j.japh.2021.02.002
Axelsson K. F., Litsne H., Lorentzon M. (2022). Fractures and Fall Injuries after Hospitalization for Seasonal Influenza-A National Retrospective Cohort Study. Osteoporos. Int. 33, 47–56. doi:10.1007/s00198-021-06068-1
Bassatne A., Basbous M., Chakhtoura M., El Zein O., Rahme M., El-Hajj Fuleihan G. (2021). The Link between COVID-19 and VItamin D (VIVID): A Systematic Review and Meta-Analysis. Metabolism 119, 154753. doi:10.1016/j.metabol.2021.154753
Battisti S., Napoli N., Pedone C., Lombardi M., Leanza G., Tramontana F., et al. (2021). Vertebral Fractures and Mortality Risk in Hospitalised Patients during the COVID-19 Pandemic Emergency. Endocrine 74, 461–469. doi:10.1007/s12020-021-02872-1
Bellavia D., Salamanna F., Raimondi L., De Luca A., Carina V., Costa V., et al. (2019). Deregulated miRNAs in Osteoporosis: Effects in Bone Metastasis. Cell. Mol. Life Sci. 76, 3723–3744. doi:10.1007/s00018-019-03162-w
Bergholt N. L., Demirel A., Pedersen M., Ding M., Kragstrup T. W., Andersen T., et al. (2021). Intermittent Hypoxic Therapy Inhibits Allogenic Bone-Graft Resorption by Inhibition of Osteoclastogenesis in a Mouse Model. Ijms 23, 323. doi:10.3390/ijms23010323
Bitar A., Khan A., Sulaiman S. S., Ali I. B. H. (2021). Prevalence, Risk Assessment, and Predictors of Osteoporosis Among Chronic Obstructive Pulmonary Disease Patients. J. Adv. Pharm. Technol. Res. 12, 395–401. doi:10.4103/japtr.japtr_98_21
Bon J. M., Zhang Y., Duncan S. R., Pilewski J. M., Zaldonis D., Zeevi A., et al. (2010). Plasma Inflammatory Mediators Associated with Bone Metabolism in COPD. COPD J. Chronic Obstr. Pulm. Dis. 7, 186–191. doi:10.3109/15412555.2010.482114
Bon J., Nouraie S. M., Smith K. J., Dransfield M. T., McDonald M. L., Hoffman E. A., et al. (2020). Lung‐Specific Risk Factors Associated With Incident Hip Fracture in Current and Former Smokers. J. Bone Min. Res. 35, 1952–1961. doi:10.1002/jbmr.4103
Brabnikova Maresova K., Pavelka K., Stepan J. J. (2013). Acute Effects of Glucocorticoids on Serum Markers of Osteoclasts, Osteoblasts, and Osteocytes. Calcif. Tissue Int. 92, 354–361. doi:10.1007/s00223-012-9684-4
Buckens C. F., van der Graaf Y., Verkooijen H. M., Mali W. P., Isgum I., Mol C. P., et al. (2015). Osteoporosis Markers on Low-Dose Lung Cancer Screening Chest Computed Tomography Scans Predict All-Cause Mortality. Eur. Radiol. 25, 132–139. doi:10.1007/s00330-014-3361-0
Cakmak H., Cakmak B. D., Yumru A. E., Aslan S., Coskun E. I., Karatas S., et al. (2015). The Relationships between Blood Pressure, Blood Glucose, and Bone Mineral Density in Postmenopausal Turkish Women. Tcrm 11, 1641–1648. doi:10.2147/tcrm.S95017
Calle Rubio M., Álvarez-Sala J. L., Vargas Centanaro G., Navarro A. M. H., Hermosa J. L. R. (2022). Testing for Vitamin D in High-Risk COPD in Outpatient Clinics in Spain: A Cross-Sectional Analysis of the VITADEPOC Study. Jcm 11, 1347. doi:10.3390/jcm11051347
Camacho-Cardenosa M., Quesada-Gómez J. M., Camacho-Cardenosa A., Leal A., Dorado G., Torrecillas-Baena B., et al. (2020). Effects of Normobaric Cyclic Hypoxia Exposure on Mesenchymal Stem-Cell Differentiation-Pilot Study on Bone Parameters in Elderly. Wjsc 12, 1667–1690. doi:10.4252/wjsc.v12.i12.1667
Cao B., Chai C., Zhao S. (2015). Protective Effect of Edaravone against Hypoxia-Induced Cytotoxicity in Osteoblasts MC3T3-E1 Cells. IUBMB Life 67, 928–933. doi:10.1002/iub.1436
Caramori G., Ruggeri P., Arpinelli F., Salvi L., Girbino G. (2019). Long-term Use of Inhaled Glucocorticoids in Patients with Stable Chronic Obstructive Pulmonary Disease and Risk of Bone Fractures: a Narrative Review of the Literature. Copd 14, 1085–1097. doi:10.2147/copd.S190215
Carnovali M., Valli R., Banfi G., Porta G., Mariotti M. (2021). Soybean Meal-Dependent Intestinal Inflammation Induces Different Patterns of Bone-Loss in Adult Zebrafish Scale. Biomedicines 9, 393. doi:10.3390/biomedicines9040393
Cesana‐Nigro N., Keshvari S., Barclay J. L., Sorbello J., Upham J. W., Benham H., et al. (2019). The Effect of Glucocorticoids on Thrombospondin‐1, Osteocalcin and the Thrombospondin‐1:Osteocalcin Ratio in Humans. Clin. Endocrinol. 91, 728–736. doi:10.1111/cen.14108
Chalitsios C. V., Shaw D. E., McKeever T. M. (2020). A Retrospective Database Study of Oral Corticosteroid and Bisphosphonate Prescribing Patterns in England. npj Prim. Care Respir. Med. 30, 5. doi:10.1038/s41533-020-0162-6
Chalitsios C. V., Shaw D. E., McKeever T. M. (2021). Corticosteroids and Bone Health in People with Asthma: A Systematic Review and Meta-Analysis. Respir. Med. 181, 106374. doi:10.1016/j.rmed.2021.106374
Chalitsios C. V., Shaw D. E., McKeever T. M. (2021). Risk of Osteoporosis and Fragility Fractures in Asthma Due to Oral and Inhaled Corticosteroids: Two Population-Based Nested Case-Control Studies. Thorax 76, 21–28. doi:10.1136/thoraxjnl-2020-215664
Chalitsios C. V., Shaw D. E., McKeever T. M. (2022). Risk of Subtrochanteric and Femoral Shaft Fractures Due to Bisphosphonate Therapy in Asthma: a Population-Based Nested Case-Control Study. Osteoporos. Int. 33, 931–935. doi:10.1007/s00198-021-06197-7
Chen K., Liu Y., He J., Pavlos N., Wang C., Kenny J., et al. (2020). Steroid-induced Osteonecrosis of the Femoral Head Reveals Enhanced Reactive Oxygen Species and Hyperactive Osteoclasts. Int. J. Biol. Sci. 16, 1888–1900. doi:10.7150/ijbs.40917
Chen W., Johnson K. M., FitzGerald J. M., Sadatsafavi M., Leslie W. D. (2018). Long-term Effects of Inhaled Corticosteroids on Bone Mineral Density in Older Women with Asthma or COPD: a Registry-Based Cohort Study. Arch. Osteoporos. 13, 116. doi:10.1007/s11657-018-0537-2
Chen Y.-W., Coxson H. O., Coupal T. M., Lam S., Munk P. L., Leipsic J., et al. (2018). The Contribution of Thoracic Vertebral Deformity and Arthropathy to Trunk Pain in Patients with Chronic Obstructive Pulmonary Disease (COPD). Respir. Med. 137, 115–122. doi:10.1016/j.rmed.2018.03.007
Chen Y.-Y., Feng J.-Y., Ting W.-Y., Yen Y.-F., Chuang P.-H., Pan S.-W., et al. (2017). Increased Risk of Incident Osteoporosis and Osteoporotic Fracture in Tuberculosis Patients: a Population-Based Study in a Tuberculosis-Endemic Area. Osteoporos. Int. 28, 1711–1721. doi:10.1007/s00198-017-3939-x
Chen Y. C., Hsu P. Y., Chin C. H., Hsiao C. C., Liou C. W., Wang T. Y., et al. (2021). H3K23/H3K36 Hypoacetylation and HDAC1 Up-Regulation Are Associated with Adverse Consequences in Obstructive Sleep Apnea Patients. Sci. Rep. 11, 20697. doi:10.1038/s41598-021-00052-9
Chen Z., Salam M. T., Karim R., Toledo-Corral C. M., Watanabe R. M., Xiang A. H., et al. (2015). Living Near a Freeway Is Associated with Lower Bone Mineral Density Among Mexican Americans. Osteoporos. Int. 26, 1713–1721. doi:10.1007/s00198-015-3051-z
Cheng C.-Y., Kuo Y.-J. (2022). Single-centre Cross-Sectional Study on the Impact of Cumulative Erythropoietin on Bone Mineral Density in Maintenance Dialysis Patients. BMJ Open 12, e056390. doi:10.1136/bmjopen-2021-056390
Chiu K. L., Lee C. C., Chen C. Y. (2021). Evaluating the Association of Osteoporosis with Inhaled Corticosteroid Use in Chronic Obstructive Pulmonary Disease in Taiwan. Sci. Rep. 11, 724. doi:10.1038/s41598-020-80815-y
Choi C.-J., Choi W.-S., Kim C.-M., Lee S.-Y., Kim K.-S. (2017). Risk of Sarcopenia and Osteoporosis in Male Tuberculosis Survivors: Korea National Health and Nutrition Examination Survey. Sci. Rep. 7, 13127. doi:10.1038/s41598-017-12419-y
Coleman R., Body J. J., Aapro M., Hadji P., Herrstedt J. (2014). Bone Health in Cancer Patients: ESMO Clinical Practice Guidelines. Ann. Oncol. 25 (Suppl. 3), iii124–iii137. doi:10.1093/annonc/mdu103
Degli Esposti L., Perrone V., Sangiorgi D., Andretta M., Bartolini F., Cavaliere A., et al. (2021). The Use of Oral Amino‐Bisphosphonates and Coronavirus Disease 2019 ( COVID ‐19) Outcomes. J Bone & Mineral Res 36, 2177–2183. doi:10.1002/jbmr.4419
di Filippo L., Formenti A. M., Doga M., Pedone E., Rovere-Querini P., Giustina A. (2021). Radiological Thoracic Vertebral Fractures Are Highly Prevalent in COVID-19 and Predict Disease Outcomes. J. Clin. Endocrinol. Metab. 106, e602–e614. doi:10.1210/clinem/dgaa738
Di Pompo G., Errani C., Gillies R., Mercatali L., Ibrahim T., Tamanti J., et al. (2021). Acid-Induced Inflammatory Cytokines in Osteoblasts: A Guided Path to Osteolysis in Bone Metastasis. Front. Cell Dev. Biol. 9, 678532. doi:10.3389/fcell.2021.678532
Diaz-Curiel M., Cabello A., Arboiro-Pinel R., Mansur J. L., Heili-Frades S., Mahillo-Fernandez I., et al. (2021). The Relationship between 25(OH) Vitamin D Levels and COVID-19 Onset and Disease Course in Spanish Patients. J. Steroid Biochem. Mol. Biol. 212, 105928. doi:10.1016/j.jsbmb.2021.105928
Doi K., Murata K., Ito S., Suzuki A., Terao C., Ishie S., et al. (2022). Role of Lysine‐Specific Demethylase 1 in Metabolically Integrating Osteoclast Differentiation and Inflammatory Bone Resorption Through Hypoxia‐Inducible Factor 1α and E2F1. Arthritis & Rheumatology 74, 948–960. doi:10.1002/art.42074
Dong Y., Peng R., Kang H., Song K., Guo Q., Zhao H., et al. (2022). Global Incidence, Prevalence, and Disability of Vertebral Fractures: a Systematic Analysis of the Global Burden of Disease Study 2019. Spine J. 22, 857–868. doi:10.1016/j.spinee.2021.12.007
Dumanskiy Y. V., Syniachenko O. V., Stepko P. A., Taktashov G. S., Chernyshova O. Y., Stoliarova O. Y. (2018). The State of Bone Metabolism in Lung Cancer Patients. Exp. Onc. 40, 136–139. doi:10.31768/2312-8852.2018.40(2):136-139
El Kholy K., Freire M., Chen T., Van Dyke T. E. (2018). Resolvin E1 Promotes Bone Preservation Under Inflammatory Conditions. Front. Immunol. 9, 1300. doi:10.3389/fimmu.2018.01300
Elkattawy S., Alyacoub R., Ayad S., Pandya M., Eckman A. (2020). A Novel Case of Hypoparathyroidism Secondary to SARS-CoV-2 Infection. Cureus 12, e10097. doi:10.7759/cureus.10097
Estrada-Cárdenas P., Camacho-Jiménez L., Peregrino-Uriarte A. B., Contreras-Vergara C. A., Hernandez-López J., Yepiz-Plascencia G. (2022). p53 Knock-Down and Hypoxia Affects Glutathione Peroxidase 4 Antioxidant Response in Hepatopancreas of the White Shrimp Litopenaeus Vannamei. Biochimie 199, 1–11. doi:10.1016/j.biochi.2022.03.010
Evans R. A., Morgan M. D. L. (2014). The Systemic Nature of Chronic Lung Disease. Clin. Chest Med. 35, 283–293. doi:10.1016/j.ccm.2014.02.009
Fouda M., Alhamad E., Al-Hajjaj M., Shaik S., Alboukai A., Al-Kassimi F. (2017). A Study of Chronic Obstructive Pulmonary Disease-specific Causes of Osteoporosis with Emphasis on the Emphysema Phenotype. Ann. Thorac. Med. 12, 101–106. doi:10.4103/atm.ATM_357_16
Gittoes N. J., Criseno S., Appelman-Dijkstra N. M., Bollerslev J., Canalis E., Rejnmark L., et al. (2020). ENDOCRINOLOGY IN the TIME of COVID-19: Management of Calcium Metabolic Disorders and Osteoporosis. Eur. J. Endocrinol. 183, G57–g65. doi:10.1530/eje-20-0385
González-Barcala F. J., Muñoz-Gall X., Mariscal E., García A., Yang S., van de Wetering G., et al. (2021). Cost-effectiveness Analysis of anti-IL-5 Therapies of Severe Eosinophilic Asthma in Spain. J. Med. Econ. 24, 874–882. doi:10.1080/13696998.2021.1941065
Grosso A., Cerveri I., Cazzoletti L., Zanolin M. E., Mattioli V., Piloni D., et al. (2021). Inhaled Corticosteroids and Risk of Osteoporosis in Late-Middle Age Subjects: a Multicenter European Cohort Study. Minerva Med. doi:10.23736/s0026-4806.21.07431-0
Halpin D. M. (2007). Systemic Effects of Chronic Obstructive Pulmonary Disease. Expert Rev. Respir. Med. 1, 75–84. doi:10.1586/17476348.1.1.75
Ham L., Geijteman E. C. T., Aarts M. J., Kuiper J. G., Kunst P. W. A., Raijmakers N. J. H., et al. (2022). Use of Potentially Inappropriate Medication in Older Patients with Lung Cancer at the End of Life. J. Geriatric Oncol. 13, 53–59. doi:10.1016/j.jgo.2021.07.009
Han Y., Pei D., Li W., Luo B., Jiang Q. (2022). Epigallocatechin Gallate Attenuates Tumor Necrosis Factor (TNF)-α-induced Inhibition of Osteoblastic Differentiation by Up-Regulating lncRNA TUG1 in Osteoporosis. Bioengineered 13, 8950–8961. doi:10.1080/21655979.2022.2056825
Heo S., Kim H., Kim S., Choe S.-A., Byun G., Lee J.-T., et al. (2022). Associations between Long-Term Air Pollution Exposure and Risk of Osteoporosis-Related Fracture in a Nationwide Cohort Study in South Korea. Ijerph 19, 2404. doi:10.3390/ijerph19042404
Hou K.-L., Lin S.-K., Chao L.-H., Hsiang-Hua Lai E., Chang C.-C., Shun C.-T., et al. (2017). Sirtuin 6 Suppresses Hypoxia-Induced Inflammatory Response in Human Osteoblasts via Inhibition of Reactive Oxygen Species Production and Glycolysis-A Therapeutic Implication in Inflammatory Bone Resorption. Biofactors 43, 170–180. doi:10.1002/biof.1320
Hu X., Sun Y., Xu W., Lin T., Zeng H. (2017). Expression of RANKL by Peripheral Neutrophils and its Association with Bone Mineral Density in COPD. Respirology 22, 126–132. doi:10.1111/resp.12878
Huang F., Cao Y., Wang C., Lan R., Wu B., Xie X., et al. (2021). PNMA5 Promotes Bone Metastasis of Non-small-Cell Lung Cancer as a Target of BMP2 Signaling. Front. Cell Dev. Biol. 9, 678931. doi:10.3389/fcell.2021.678931
Huang J.-F., Tan Q.-C., Bai H., Wang J., Bergman M., Wu Z. (2022). Bone Mineral Density, Osteopenia, and Osteoporosis Among U.S. Adults with Cancer. Qjm, hcac015. doi:10.1093/qjmed/hcac015
Huang Y.-C., Zhu H.-M., Cai J.-Q., Huang Y.-Z., Xu J., Zhou Y., et al. (2012). Hypoxia Inhibits the Spontaneous Calcification of Bone Marrow-Derived Mesenchymal Stem Cells. J. Cell. Biochem. 113, 1407–1415. doi:10.1002/jcb.24014
Ishii M., Yakabe M., Teramoto S., Oike Y., Ogawa S., Iijima K., et al. (2007). A 94-Year-Old Woman with Nontuberculous mycobacterium Who Developed Small Intestinal Intussusception Associated with a Percutaneous Endoscopic Jejunostomy Tube. Nippon. Ronen Igakkai Zasshi 44, 648–652. doi:10.3143/geriatrics.44.648
Jiang L., Sheng K., Wang C., Xue D., Pan Z. (2020). The Effect of MMP-2 Inhibitor 1 on Osteogenesis and Angiogenesis During Bone Regeneration. Front. Cell Dev. Biol. 8, 596783. doi:10.3389/fcell.2020.596783
Johansen N. J., Harsløf T., Hermann A. P., Andersen C. U., Hilberg O., Løkke A. (2018). Examination for Osteoporosis in Patients with Chronic Obstructive Pulmonary Disease. Ugeskr. Laeger 180, V12170934.
Johnell O., Kanis J. A. (2006). An Estimate of the Worldwide Prevalence and Disability Associated with Osteoporotic Fractures. Osteoporos. Int. 17, 1726–1733. doi:10.1007/s00198-006-0172-4
Kakoullis L., Sampsonas F., Karamouzos V., Kyriakou G., Parperis K., Papachristodoulou E., et al. (2022). The Impact of Osteoporosis and Vertebral Compression Fractures on Mortality and Association with Pulmonary Function in COPD: A Meta-Analysis. Jt. Bone Spine 89, 105249. doi:10.1016/j.jbspin.2021.105249
Kimmel D. B., Vennin S., Desyatova A., Turner J. A., Akhter M. P., Lappe J. M., et al. (2022). Bone Architecture, Bone Material Properties, and Bone Turnover in Non-osteoporotic Post-menopausal Women with Fragility Fracture. Osteoporos. Int. 33, 1125–1136. doi:10.1007/s00198-022-06308-y
Kirwan R., McCullough D., Butler T., Perez de Heredia F., Davies I. G., Stewart C. (2020). Sarcopenia during COVID-19 Lockdown Restrictions: Long-Term Health Effects of Short-Term Muscle Loss. Geroscience 42, 1547–1578. doi:10.1007/s11357-020-00272-3
Kochetkova E. A., Gel'tser B. I., Semisotova E. F., Volkova M. V. (2002). Biochemical and Immunological Markers of Osteoporosis in Patients with Chronic Obstructive Pulmonary Disease. Klin. Med. Mosk. 80, 31–35.
Kokturk N., Baha A., Oh Y.-M., Young Ju J., Jones P. W. (2018). Vitamin D Deficiency: What Does it Mean for Chronic Obstructive Pulmonary Disease (COPD)? a Compherensive Review for Pulmonologists. Clin. Respir. J. 12, 382–397. doi:10.1111/crj.12588
Kondo T., Otsuka Y., Aoki H., Goto Y., Kawaguchi Y., Waguri-Nagaya Y., et al. (2021). The Inducible Nitric Oxide Synthase Pathway Promotes Osteoclastogenesis under Hypoxic Culture Conditions. Am. J. Pathology 191, 2072–2079. doi:10.1016/j.ajpath.2021.08.014
Kong S. H., Hwang B. K., Yoon B.-H. (2021). The Impact of COVID-19 on the Optimal Management of Osteoporosis. J. Bone Metab. 28, 115–122. doi:10.11005/jbm.2021.28.2.115
Ku C. R., Jung K. Y., Ahn C. H., Moon J. S., Lee J. H., Kim E. H., et al. (2021). COVID-19 Vaccination for Endocrine Patients: A Position Statement from the Korean Endocrine Society. Endocrinol. Metab. 36, 757–765. doi:10.3803/EnM.2021.404
Kupeli A., Bulut E., Unver E., Danisan G. (2021). The Relationship between Vertebral Deformities and Thoracic Arthropathy with Back Pain in Chronic Obstructive Pulmonary Disease. Int. J. Clin. Pract. 75, e14953. doi:10.1111/ijcp.14953
Laskowski L. K., Goldfarb D. S., Howland M. A., Kavcsak K., Lugassy D. M., Smith S. W. (2016). A RANKL Wrinkle: Denosumab-Induced Hypocalcemia. J. Med. Toxicol. 12, 305–308. doi:10.1007/s13181-016-0543-y
Lee S., Krüger B. T., Ignatius A., Tuckermann J. (2021). Distinct Glucocorticoid Receptor Actions in Bone Homeostasis and Bone Diseases. Front. Endocrinol. 12, 815386. doi:10.3389/fendo.2021.815386
Lehouck A., van Remoortel H., Troosters T., Decramer M., Janssens W. (2010). BPCO et métabolisme osseux: une mise à jour clinique. Rev. Des. Mal. Respir. 27, 1231–1242. doi:10.1016/j.rmr.2010.10.007
Leng S., Xue Q.-L., Huang Y., Semba R., Chaves P., Bandeen-Roche K., et al. (2005). Total and Differential White Blood Cell Counts and Their Associations with Circulating Interleukin-6 Levels in Community-Dwelling Older Women. Journals Gerontology Ser. A Biol. Sci. Med. Sci. 60, 195–199. doi:10.1093/gerona/60.2.195
Leslie W. D., Morin S. N. (2020). New Developments in Fracture Risk Assessment for Current Osteoporosis Reports. Curr. Osteoporos. Rep. 18, 115–129. doi:10.1007/s11914-020-00590-7
Lin J., Xu R., Shen X., Jiang H., Du S. (2020). Metformin Promotes the Osseointegration of Titanium Implants under Osteoporotic Conditions by Regulating BMSCs Autophagy, and Osteogenic Differentiation. Biochem. Biophysical Res. Commun. 531, 228–235. doi:10.1016/j.bbrc.2020.06.146
Lin M.-D., Peng C.-H., Lin W.-Y., Yeh K.-T., Chen I.-H., Wu W.-T. (2021). The Molecular Etiology and Treatment of Glucocorticoid-Induced Osteoporosis. Tzu Chi Med. J. 33, 212–223. doi:10.4103/tcmj.tcmj_233_20
Liu J., Fu S., Jiang J., Tang X. (2021). Association between Outdoor Particulate Air Pollution and the Risk of Osteoporosis: a Systematic Review and Meta-Analysis. Osteoporos. Int. 32, 1911–1919. doi:10.1007/s00198-021-05961-z
Lv H., Zhang Q., Yin Y., Zhu Y., Wang J., Hou Z., et al. (2020). Epidemiologic Characteristics of Traumatic Fractures during the Outbreak of Coronavirus Disease 2019 (COVID-19) in China: A Retrospective & Comparative Multi-Center Study. Injury 51, 1698–1704. doi:10.1016/j.injury.2020.06.022
Ma G. T., Lee S. K., Park K.-K., Park J., Son S. H., Jung M., et al. (2018). Artemisinin-Daumone Hybrid Inhibits Cancer Cell-Mediated Osteolysis by Targeting Cancer Cells and Osteoclasts. Cell Physiol. Biochem. 49, 1460–1475. doi:10.1159/000493449
Masik N., Matviichuk M., Masik O. (2021). BONE FORMATION MARKERS (N-TERMINAL PROPEPTIDE TYPE I PROCOLLAGEN, OSTEOCALCIN AND VITAMIN D) AS EARLY PREDICTORS OF OSTEOPOROSIS IN PATIENTS SUFFERING FROM CHRONIC OBSTRUCTIVE LUNG DISEASE. Georgian Med. News, 64–71.
Mazzucchelli R., Crespi Villarias N., Perez Fernandez E., Durban Reguera M. L., Garcia-Vadillo A., Quiros F. J., et al. (2018). Short-term Association between Outdoor Air Pollution and Osteoporotic Hip Fracture. Osteoporos. Int. 29, 2231–2241. doi:10.1007/s00198-018-4605-7
Mbese Z., Aderibigbe B. A. (2021). Bisphosphonate-Based Conjugates and Derivatives as Potential Therapeutic Agents in Osteoporosis, Bone Cancer and Metastatic Bone Cancer. Ijms 22, 6869. doi:10.3390/ijms22136869
Mi B., Xiong Y., Zhang C., Zhou W., Chen L., Cao F., et al. (2021). SARS-CoV-2-induced Overexpression of miR-4485 Suppresses Osteogenic Differentiation and Impairs Fracture Healing. Int. J. Biol. Sci. 17, 1277–1288. doi:10.7150/ijbs.56657
Miyamoto T. (2016). Hypoxemia and Osteoporosis-Possible Roles of HIF1α on Respiratory Disease-Related Osteoporosis. Clin. Calcium 26, 1429–1435.
Miyashita H., Cruz C., Smith C. (2021). Risk Factors of Skeletal-Related Events in Patients with Bone Metastasis from Non-small Cell Lung Cancer Undergoing Treatment with Zoledronate-A Post Hoc Analysis of a Randomized Clinical Trial. Support Care Cancer 29, 1629–1633. doi:10.1007/s00520-020-05665-w
Momiuchi Y., Motomura Y., Suga E., Mizuno H., Kikuta J., Morimoto A., et al. (2021). Group 2 Innate Lymphoid Cells in Bone Marrow Regulate Osteoclastogenesis in a Reciprocal Manner via RANKL, GM-CSF and IL-13. Int. Immunol. 33, 573–585. doi:10.1093/intimm/dxab062
Napoli N., Elderkin A. L., Kiel D. P., Khosla S. (2020). Managing Fragility Fractures during the COVID-19 Pandemic. Nat. Rev. Endocrinol. 16, 467–468. doi:10.1038/s41574-020-0379-z
Ni S., Yuan Y., Qian Z., Zhong Z., Lv T., Kuang Y., et al. (2021). Hypoxia Inhibits RANKL-Induced Ferritinophagy and Protects Osteoclasts from Ferroptosis. Free Radic. Biol. Med. 169, 271–282. doi:10.1016/j.freeradbiomed.2021.04.027
Niu Y., Yang H., Yu Z., Gao C., Ji S., Yan J., et al. (2022). Intervention with the Bone-Associated Tumor Vicious Cycle through Dual-Protein Therapeutics for Treatment of Skeletal-Related Events and Bone Metastases. ACS Nano 16, 2209–2223. doi:10.1021/acsnano.1c08269
Okazaki R. (2015). Pharmacological Treatment of Other Types of Secondary Osteoporosis. Nihon Rinsho 73, 1740–1745.
Osteoporosis and Fracture Risk with Corticosteroids Used for Asthma. Drug Ther. Bull. 59 (11), 165, doi:10.1136/dtb.2021.000052 (2021).
Ozcakir S., Sigirli D., Ursavas A., Uzaslan E. (2020). COPD and Osteoporosis: Associated Factors in Patients Treated with Inhaled Corticosteroids. Copd 15, 2441–2448. doi:10.2147/copd.S274728
Pan Y., Shi D., Wang H., Chen T., Cui D., Cheng X., et al. (2020). Automatic Opportunistic Osteoporosis Screening Using Low-Dose Chest Computed Tomography Scans Obtained for Lung Cancer Screening. Eur. Radiol. 30, 4107–4116. doi:10.1007/s00330-020-06679-y
Pang K.-L., Ekeuku S. O., Chin K.-Y. (2021). Particulate Air Pollution and Osteoporosis: A Systematic Review. Rmhp 14, 2715–2732. doi:10.2147/rmhp.S316429
Park H.-W., Ge B., Tse S., Grundberg E., Pastinen T., Kelly H. W., et al. (2015). Genetic Risk Factors for Decreased Bone Mineral Accretion in Children with Asthma Receiving Multiple Oral Corticosteroid Bursts. J. Allergy Clin. Immunol. 136, 1240–1246. e1-8. doi:10.1016/j.jaci.2015.04.014
Pattamapaspong N., Muttarak M., Sivasomboon C. (2011). Tuberculosis Arthritis and Tenosynovitis. Semin. Musculoskelet. Radiol. 15, 459–469. doi:10.1055/s-0031-1293492
Peng P., Nie Z., Sun F., Peng H. (2021). Glucocorticoids Induce Femoral Head Necrosis in Rats through the ROS/JNK/c‐Jun Pathway. FEBS Open Bio 11, 312–321. doi:10.1002/2211-5463.13037
Peng W., Li H., Peng L., Wang Y., Wang W. (2022). Effects of Particulate Matter on Hospital Admissions for Respiratory Diseases: an Ecological Study Based on 12.5 Years of Time Series Data in Shanghai. Environ. Health 21, 12. doi:10.1186/s12940-021-00828-6
Picado C., Luengo M. (1996). Corticosteroid-Induced Bone Loss. Drug Saf. 15, 347–359. doi:10.2165/00002018-199615050-00005
Pobeha P., Lazúrová I., Tkácová R. (2010). Osteoporosis in Chronic Obstructive Pulmonary Disease. Vnitr Lek. 56, 1142–1149.
Pompe E., de Jong P., van Rikxoort E. M., Gallardo Estrella L., de Jong W. U., Vliegenthart R., et al. (2016). Smokers with Emphysema and Small Airway Disease on Computed Tomography Have Lower Bone Density. Copd 11, 1207–1216. doi:10.2147/copd.S103680
Pramusita A., Kitaura H., Ohori F., Noguchi T., Marahleh A., Nara Y., et al. (2022). Salt-Sensitive Hypertension Induces Osteoclastogenesis and Bone Resorption via Upregulation of Angiotensin II Type 1 Receptor Expression in Osteoblasts. Front. Cell Dev. Biol. 10, 816764. doi:10.3389/fcell.2022.816764
Puolijoki H., Liippo K., Herrala J., Salmi J., Tala E. (1992). Inhaled Beclomethasone Decreases Serum Osteocalcin in Postmenopausal Asthmatic Women. Bone 13, 285–288. doi:10.1016/8756-3282(92)90072-5
Puolijoki H., Risteli J., Herrala J., Risteli L., Liippo K. (1996). Effect of Inhaled Beclomethasone on Serum Markers of Collagen Metabolism in Postmenopausal Asthmatic Women. Respir. Med. 90, 339–342. doi:10.1016/s0954-6111(96)90129-8
Raisi-Estabragh Z., Martineau A. R., Curtis E. M., Moon R. J., Darling A., Lanham-New S., et al. (2021). Vitamin D and Coronavirus Disease 2019 (COVID-19): Rapid Evidence Review. Aging Clin. Exp. Res. 33, 2031–2041. doi:10.1007/s40520-021-01894-z
Ramchand S. K., David N. L., Lee H., Eastell R., Tsai J. N., Leder B. Z. (2021). Efficacy of Zoledronic Acid in Maintaining Areal and Volumetric Bone Density After Combined Denosumab and Teriparatide Administration: DATA‐HD Study Extension. J. Bone Min. Res. 36, 921–930. doi:10.1002/jbmr.4259
Ranzani O. T., Milà C., Kulkarni B., Kinra S., Tonne C. (2020). Association of Ambient and Household Air Pollution With Bone Mineral Content Among Adults in Peri-Urban South India. JAMA Netw. Open 3, e1918504. doi:10.1001/jamanetworkopen.2019.18504
Rinaldi S., Santoni M., Leoni G., Fiordoliva I., Marcantognini G., Meletani T., et al. (2019). The Prognostic and Predictive Role of Hyponatremia in Patients with Advanced Non-small Cell Lung Cancer (NSCLC) with Bone Metastases. Support Care Cancer 27, 1255–1261. doi:10.1007/s00520-018-4489-2
Rose M., Hildebrandt M., Schoeneich F., Danzer G., Klapp B. F. (1999). Severe Anorexia Nervosa Associated with Osteoporotic-Linked Femural Neck Fracture and Pulmonary Tuberculosis: a Case Report. Int. J. Eat. Disord. 25, 463–467. doi:10.1002/(sici)1098-108x(199905)25:4<463::aid-eat12>3.0.co;2-q
Roy S., Frodsham A., Saha B., Hazra S. K., Mascie‐Taylor C. G. N., Hill A. V. S. (1999). Association of Vitamin D Receptor Genotype with Leprosy Type. J. Infect. Dis. 179, 187–191. doi:10.1086/314536
Ruscitti P., Cipriani P., Carubbi F., Liakouli V., Zazzeroni F., Di Benedetto P., et al. (2015). The Role of IL-1βin the Bone Loss during Rheumatic Diseases. Mediat. Inflamm. 2015, 782382. doi:10.1155/2015/782382
Saha H., Mukherjee B., Bindhani B., Ray M. R. (2016). Changes in RANKL and Osteoprotegerin Expression after Chronic Exposure to Indoor Air Pollution as a Result of Cooking with Biomass Fuel. J. Appl. Toxicol. 36, 969–976. doi:10.1002/jat.3275
Salamanna F., Maglio M., Sartori M., Landini M. P., Fini M. (2021). Vitamin D and Platelets: A Menacing Duo in COVID-19 and Potential Relation to Bone Remodeling. Ijms 22, 10010. doi:10.3390/ijms221810010
Salvio G., Gianfelice C., Firmani F., Lunetti S., Ferroni R., Balercia G., et al. (2022). Remote Management of Osteoporosis in the First Wave of the COVID-19 Pandemic. Arch. Osteoporos. 17, 37. doi:10.1007/s11657-022-01069-x
Sandru F., Carsote M., Dumitrascu M. C., Albu S. E., Valea A. (2020). Glucocorticoids and Trabecular Bone Score. J. Med. Life 13, 449–453. doi:10.25122/jml-2019-0131
Sato A. Y., Cregor M., McAndrews K., Li T., Condon K. W., Plotkin L. I., et al. (2019). Glucocorticoid-Induced Bone Fragility Is Prevented in Female Mice by Blocking Pyk2/Anoikis Signaling. Endocrinology 160, 1659–1673. doi:10.1210/en.2019-00237
Savula M. M., Kravchenko N. S., Slivka IuI. (2004). Bone Mineral Density in Some Lung Diseases. Probl. Tuberk. Bolezn. Legk., 45–47.
Sethakorn N., Heninger E., Sánchez-de-Diego C., Ding A. B., Yada R. C., Kerr S. C., et al. (2022). Advancing Treatment of Bone Metastases through Novel Translational Approaches Targeting the Bone Microenvironment. Cancers 14, 757. doi:10.3390/cancers14030757
Shao J., Liu S., Zhang M., Chen S., Gan S., Chen C., et al. (2022). A Dual Role of HIF1α in Regulating Osteogenesis-Angiogenesis Coupling. Stem Cell Res. Ther. 13, 59. doi:10.1186/s13287-022-02742-1
She K., Yang W., Li M., Xiong W., Zhou M. (2021). FAIM2 Promotes Non-Small Cell Lung Cancer Cell Growth and Bone Metastasis by Activating the Wnt/β-Catenin Pathway. Front. Oncol. 11, 690142. doi:10.3389/fonc.2021.690142
Shea J. E., Miller S. C., Poole D. C., Mattson J. P. (19852003). Cortical Bone Dynamics, Strength, and Densitometry after Induction of Emphysema in Hamsters. J. Appl. Physiology 95, 631–634. doi:10.1152/japplphysiol.01049.2002
Shin M. Y., Kang Y. E., Kong S. E., Ju S. H., Back M. K., Kim K. S. (2015). A Case of Low Bone Mineral Density with Vitamin D Deficiency Due to Prolonged Lactation and Severe Malnutrition. J. Bone Metab. 22, 39–43. doi:10.11005/jbm.2015.22.1.39
Shivshankar P., Karmouty-Quintana H., Mills T., Doursout M.-F., Wang Y., Czopik A. K., et al. (2022). SARS-CoV-2 Infection: Host Response, Immunity, and Therapeutic Targets. Inflammation, 45, 1–20. doi:10.1007/s10753-022-01656-7
Shmarina G., Pukhalsky A., Petrova N., Zakharova E., Avakian L., Kapranov N., et al. (2013). TNF Gene Polymorphisms in Cystic Fibrosis Patients: Contribution to the Disease Progression. J. Transl. Med. 11, 19. doi:10.1186/1479-5876-11-19
Soen S., Kaku M., Okubo N., Touzeni S., Saito K., Kobayashi M. (2021). Epidemiology of Glucocorticoid-Induced Osteoporosis and Management of Associated Fracture Risk in Japan. J. Bone Min. Metab. 39, 1019–1030. doi:10.1007/s00774-021-01236-z
Song X., Tang Y., Zhu J., Tian Y., Song Z., Hu X., et al. (2020). HIF-1α Induces Hypoxic Apoptosis of MLO-Y4 Osteocytes via JNK/caspase-3 Pathway and the Apoptotic-Osteocyte-Mediated Osteoclastogenesis In Vitro. Tissue Cell 67, 101402. doi:10.1016/j.tice.2020.101402
Sood V., Rogers L., Khurana S. (2021). Managing Corticosteroid-Related Comorbidities in Severe Asthma. Chest 160, 1614–1623. doi:10.1016/j.chest.2021.05.021
Srinivasan S., Avadhani N. G. (2007). Hypoxia-mediated Mitochondrial Stress in RAW264.7 Cells Induces Osteoclast-like TRAP-Positive Cells. Ann. N. Y. Acad. Sci. 1117, 51–61. doi:10.1196/annals.1402.067
Srinivasan S., Koenigstein A., Joseph J., Sun L., Kalyanaraman B., Zaidi M., et al. (2010). Role of Mitochondrial Reactive Oxygen Species in Osteoclast Differentiation. Ann. N. Y. Acad. Sci. 1192, 245–252. doi:10.1111/j.1749-6632.2009.05377.x
Stanojkovic I., Kotur-Stevuljevic J., Spasic S., Milenkovic B., Vujic T., Stefanovic A., et al. (2013). Relationship between Bone Resorption, Oxidative Stress and Inflammation in Severe COPD Exacerbation. Clin. Biochem. 46, 1678–1682. doi:10.1016/j.clinbiochem.2013.08.003
Sundaresh V. (2020). Osteoporosis Management Strategies in the COVID-19 Pandemic. South Med. J. 113, 612–613. doi:10.14423/smj.0000000000001179
Tahtabasi M., Kilicaslan N., Akin Y., Karaman E., Gezer M., Icen Y. K., et al. (2021). The Prognostic Value of Vertebral Bone Density on Chest CT in Hospitalized COVID-19 Patients. J. Clin. Densitom. 24, 506–515. doi:10.1016/j.jocd.2021.07.007
Tao H., Ge G., Li W., Liang X., Wang H., Li N., et al. (2020). Dysimmunity and Inflammatory Storm: Watch Out for Bone Lesions in COVID-19 Infection. Med. Hypotheses 145, 110332. doi:10.1016/j.mehy.2020.110332
Tao M. H., Chen S., Freudenheim J. L., Cauley J. A., Johnson K. C., Mai X., et al. (2018). Oral Bisphosphonate Use and Lung Cancer Incidence Among Postmenopausal Women. Ann. Oncol. 29, 1476–1485. doi:10.1093/annonc/mdy097
Teh S. W., Koh A. E.-H., Tong J. B., Wu X., Samrot A. V., Rampal S., et al. (2021). Hypoxia in Bone and Oxygen Releasing Biomaterials in Fracture Treatments Using Mesenchymal Stem Cell Therapy: A Review. Front. Cell Dev. Biol. 9, 634131. doi:10.3389/fcell.2021.634131
Teissier T., Temkin V., Pollak R. D., Cox L. S. (2022). Crosstalk Between Senescent Bone Cells and the Bone Tissue Microenvironment Influences Bone Fragility During Chronological Age and in Diabetes. Front. Physiol. 13, 812157. doi:10.3389/fphys.2022.812157
Tsourdi E., Hofbauer L., Rauner M. (2022). The Impact of COVID-19 in Bone Metabolism: Basic and Clinical Aspects. Horm. Metab. Res. doi:10.1055/a-1825-9641
Tsukamoto M., Mori T., Nakamura E., Okada Y., Fukuda H., Yamanaka Y., et al. (2020). Chronic Obstructive Pulmonary Disease Severity in Middle-Aged and Older Men with Osteoporosis Associates with Decreased Bone Formation. Osteoporos. Sarcopenia 6, 179–184. doi:10.1016/j.afos.2020.11.003
Tsukamoto M., Mori T., Wang K.-Y., Okada Y., Fukuda H., Naito K., et al. (2019). Systemic Bone Loss, Impaired Osteogenic Activity and Type I Muscle Fiber Atrophy in Mice with Elastase-Induced Pulmonary Emphysema: Establishment of a COPD-Related Osteoporosis Mouse Model. Bone 120, 114–124. doi:10.1016/j.bone.2018.10.017
Ulivieri F. M., Banfi G., Camozzi V., Colao A., Formenti A. M., Frara S., et al. (2021). Vitamin D in the Covid-19 Era: a Review with Recommendations from a G.I.O.S.E.G. Expert Panel. Endocrine 72, 597–603. doi:10.1007/s12020-021-02749-3
Wan Q., Schoenmaker T., Jansen I. D. C., Bian Z., de Vries T. J., Everts V. (2016). Osteoblasts of Calvaria Induce Higher Numbers of Osteoclasts Than Osteoblasts from Long Bone. Bone 86, 10–21. doi:10.1016/j.bone.2016.02.010
Wang L., Yu W., Yin X., Cui L., Tang S., Jiang N., et al. (2021). Prevalence of Osteoporosis and Fracture in China. JAMA Netw. Open 4, e2121106. doi:10.1001/jamanetworkopen.2021.21106
Wang M., Zhao M., Guo Q., Lou J., Wang L. (2021). Non-small Cell Lung Cancer Cell-Derived Exosomal miR-17-5p Promotes Osteoclast Differentiation by Targeting PTEN. Exp. Cell Res. 408, 112834. doi:10.1016/j.yexcr.2021.112834
Wang X., Wei L., Li Q., Lai Y. (2022). HIF-1α Protects Osteoblasts from ROS-Induced Apoptosis. Free Radic. Res. 56, 143–153. doi:10.1080/10715762.2022.2037581
Weare-Regales N., Hudey S. N., Lockey R. F. (2021). Practical Guidance for Prevention and Management of Glucocorticoid-Induced Osteoporosis for the Allergist/Immunologist. J. Allergy Clin. Immunol. Pract. 9, 1841–1850. doi:10.1016/j.jaip.2020.12.050
Williamson J. W., Raven P. B., Foresman B. H., Whipp B. J. (1993). Evidence for an Intramuscular Ventilatory Stimulus during Dynamic Exercise in Man. Respir. Physiol. 94, 121–135. doi:10.1016/0034-5687(93)90042-9
Wright K. M., Friedland J. S. (2004). Regulation of Monocyte Chemokine and MMP-9 Secretion by Proinflammatory Cytokines in Tuberculous Osteomyelitis. J. Leukoc. Biol. 75, 1086–1092. doi:10.1189/jlb.0903433
Wu J., Guo B., Guan H., Mi F., Xu J., Li Y., et al. (2021). The Association Between Long-Term Exposure to Ambient Air Pollution and Bone Strength in China. J. Clin. Endocrinol. Metab. 106, e5097–e5108. doi:10.1210/clinem/dgab462
Wu J., Hong S., Xie X., Liu W. (2020). A Network Pharmacology-Based Study on the Anti-Lung Cancer Effect of Dipsaci Radix. Evidence-Based Complementary Altern. Med. 2020, 7424061. doi:10.1155/2020/7424061
Wu S., Pan Y., Mao Y., Chen Y., He Y. (2021). Current Progress and Mechanisms of Bone Metastasis in Lung Cancer: a Narrative Review. Transl. Lung Cancer Res. 10, 439–451. doi:10.21037/tlcr-20-835
Wu S.-C., Chen Y.-H., Peng S.-Y., Cheng M.-T., Hsu Y.-P., Huang Z.-X., et al. (2019). Different Susceptibilities of Osteoclasts and Osteoblasts to Glucocorticoid-Induced Oxidative Stress and Mitochondrial Alterations. Chin. J. Physiol. 62, 70–79. doi:10.4103/cjp.Cjp_7_19
Wu Y., Guo Z., Fu X., Wu J., Gao J., Zeng Q., et al. (2019). The Study Protocol for the China Health Big Data (China Biobank) Project. Quant. Imaging Med. Surg. 9, 1095–1102. doi:10.21037/qims.2019.06.16
Xiao C., Bai G., Du Y., Jiang H., Yu X. (2020). Association of High HIF-1α Levels in Serous Periodontitis with External Root Resorption by the NFATc1 Pathway. J. Mol. Hist. 51, 649–658. doi:10.1007/s10735-020-09911-7
Xu K., Lu C., Ren X., Wang J., Xu P., Zhang Y. (2021). Overexpression of HIF ‐1α Enhances the Protective Effect of Mitophagy on Steroid‐induced Osteocytes Apoptosis. Environ. Toxicol. 36, 2123–2137. doi:10.1002/tox.23327
Yadav R. K., Hasan M. N., Sarkar S. M., Rahman M. M., Rahman M. A., Mosharraf-Hossain A. K. (2021). Frequency of Osteoporosis Among Chronic Obstructive Pulmonary Disease Patients Attending Respiratory Medicine Out Patient Department, BSMMU. Mymensingh Med. J. 30, 1060–1066.
Yang K., Cao F., Qiu S., Jiang W., Tao L., Zhu Y. (2022). Metformin Promotes Differentiation and Attenuates H2O2-Induced Oxidative Damage of Osteoblasts via the PI3K/AKT/Nrf2/HO-1 Pathway. Front. Pharmacol. 13, 829830. doi:10.3389/fphar.2022.829830
Yang K., Wen G., Wang J., Zhou S., Da W., Meng Y., et al. (2021). Complication and Sequelae of COVID-19: What Should We Pay Attention to in the Post-Epidemic Era. Front. Immunol. 12, 711741. doi:10.3389/fimmu.2021.711741
Yeh J.-J., Wang Y.-C., Lin C.-C., Lin C.-L., Hsu W.-H. (2016). Association of Respiratory Tuberculosis with Incident Bone Fracture: Bridging the Tuberculosis Airway Infection and the Osteoporotic Bone. PLoS One 11, e0168673. doi:10.1371/journal.pone.0168673
Yi L., Li Z., Jiang H., Cao Z., Liu J., Zhang X. (2018). Gene Modification of Transforming Growth Factor β (TGF-β) and Interleukin 10 (IL-10) in Suppressing Mt Sonicate Induced Osteoclast Formation and Bone Absorption. Med. Sci. Monit. 24, 5200–5207. doi:10.12659/msm.909720
Zeng D., Yao P., Zhao H. (2019). P2X7, a Critical Regulator and Potential Target for Bone and Joint Diseases. J. Cell. Physiology 234, 2095–2103. doi:10.1002/jcp.27544
Zhang L., Sun Y. (2021). Muscle-Bone Crosstalk in Chronic Obstructive Pulmonary Disease. Front. Endocrinol. 12, 724911. doi:10.3389/fendo.2021.724911
Zhang Y., Liu X., Li K., Bai J. (2014). Effect of R-Mt-Cpn10 on Human Osteoblast Cells. Int. J. Clin. Exp. Med. 7, 2779–2786.
Zhang Y., Liu X., Li K., Bai J. (2015). Mycobacterium tuberculosis 10-kDa Co-chaperonin Regulates the Expression Levels of Receptor Activator of Nuclear Factor-κB Ligand and Osteoprotegerin in Human Osteoblasts. Exp. Ther. Med. 9, 919–924. doi:10.3892/etm.2014.2153
Zhu J., Tang Y., Wu Q., Ji Y. C., Kang F. W. (2019). Mechanism of Participation of Osteocytes in the Formation of Osteoclasts under Hypoxia. Hua Xi Kou Qiang Yi Xue Za Zhi 37, 463–468. doi:10.7518/hxkq.2019.05.002
Keywords: osteoporosis, respiratory disease, COPD, asthma, lung cancer, tuberculosis
Citation: Ma Y, Qiu S and Zhou R (2022) Osteoporosis in Patients With Respiratory Diseases. Front. Physiol. 13:939253. doi: 10.3389/fphys.2022.939253
Received: 09 May 2022; Accepted: 07 June 2022;
Published: 12 July 2022.
Edited by:
Narattaphol Charoenphandhu, Mahidol University, ThailandReviewed by:
Nattapon Panupinthu, Mahidol University, ThailandGiacomina Brunetti, University of Bari Aldo Moro, Italy
Copyright © 2022 Ma, Qiu and Zhou. This is an open-access article distributed under the terms of the Creative Commons Attribution License (CC BY). The use, distribution or reproduction in other forums is permitted, provided the original author(s) and the copyright owner(s) are credited and that the original publication in this journal is cited, in accordance with accepted academic practice. No use, distribution or reproduction is permitted which does not comply with these terms.
*Correspondence: Renyi Zhou, cnl6aG91QGNtdS5lZHUuY24=