- 1Departamento de Fisioterapia, Facultad de Ciencias de la Salud, Centro Superior de Estudios Universitarios La Salle, Universidad Autónoma de Madrid, Madrid, Spain
- 2Breatherapy Research Group, Instituto de Neurociencias y Ciencias del Movimiento (INCIMOV), Centro Superior de Estudios Universitarios La Salle, Universidad Autónoma de Madrid, Madrid, Spain
- 3Sports Training Laboratory, Faculty of Sports Sciences, Universidad de Castilla-La Mancha, Toledo, Spain
- 4Sport, Physical Education and Health Sciences, University of Edinburgh, Edinburgh, United Kingdom
- 5Facultad de Ciencias de la Vida y de la Naturaleza, Universidad Nebrija, Madrid, Spain
Background Trained breath-hold divers have shown physiological adaptations that might improve athletes’ aerobic and anaerobic performance.
Objective This study aimed to systematically review the scientific literature and perform a meta-analysis to assess the effects of voluntary apnoea training on markers of anaerobic and aerobic performance, such as blood lactate and VO2max.
Methods A literature search on three databases (Web of Science, PubMed and SCOPUS) was conducted in March 2022. The inclusion criteria were 1) peer-reviewed journal publication; 2) clinical trials; 3) healthy humans; 4) effects of apnoea training; 5) variables included markers of aerobic or anaerobic performance, such as lactate and VO2max.
Results 545 manuscripts were identified following database examination. Only seven studies met the inclusion criteria and were, therefore, included in the meta-analysis. 126 participants were allocated to either voluntary apnoea training (ApT; n = 64) or normal breathing (NB; n = 63). Meta-analysis on the included studies demonstrated that ApT increased the peak blood lactate concentration more than NB (MD = 1.89 mmol*L−1 [95% CI 1.05, 2.73], z = 4.40, p < 0.0001). In contrast, there were no statistically significant effects of ApT on VO2max (MD = 0.89 ml*kg−1*min−1 [95% CI −1.23, 3.01], z = 0.82, p = 0.41).
Conclusion ApT might be an alternative strategy to enhace anaerobic performance associated with increased maximum blood lactate; however, we did not find evidence of ApT effects on physiological aerobic markers, such as VO2max.
Systematic Review Registration: [PRISMA], identifier [registration number].
Key points
- Results suggest that apnoea training might increase peak blood lactate concentration, potentially related to improved performance in sports requiring high anaerobic capacity, assuming an increased lactate tolerance. Further evidence is required to analyze potential benefits of apnoea training on maximal aerobic capacity.
- It is possible to generate severe hypoxaemia, increased lactate concentration and a greater glycolytic activity without being placed in a hypoxic environment, by using apnoea training at low lung volumes, eliciting faster hypoxaemia.
- Repeated apnoea training seems to be the most optimal method for use in athletes unfamiliar with apnoea training, as it is necessary to train an individual’s psychological tolerance of maximal apnoea.
Introduction
In a breath-hold exercise, a succession of mechanisms elicit a range of physiological responses. First, even in dry conditions, the so-called diving reflex (Costalat, 2015) triggers a bradycardia powerful enough to nullify the exercise-induced increase in Heart Rate (HR) produced by breath-hold diving exercise (Breskovic, 2011). The diving response also elicits a centralization of blood flow by increased peripheral vasoconstriction (Andersson, 2009), as a consequence of hypoxaemia (Lindholm et al., 2007), whilst trying to maintain continuous gas exchange (O2/CO2) between the alveoli, blood and the energy-demanding cell. Redistribution of the cardiac output better maintains the blood flow to organs sensitive to hypoxia, such as the brain and heart, leaving peripheral tissues without sufficient oxygen to metabolize lactate. The increasing accumulation of blood lactate and concomitant progressive acidification is associated with peripherical fatigue in these muscles (Brooks et al., 2022); thus, after a maximal apnoea, PO2 may be reduced to as low as 17–22 mmHg while PCO2 may be increased to 55–80 mmHg if breath-hold occurs during physical exercise (Schagatay, 2010; Bain et al., 2016). During apnea training the exposure to hypoxia is short and intermittent but much more intense than that suffered by climbers or pilots (Benedek, 2002; Truszczynski et al., 2009); for instance, during a maximal apnea a trained diver could reach as low as 30% oxygen saturation (de Asís Fernández et al., 2019). These combined disturbances in arterial gases trigger a splenic contraction, increasing the levels of circulating red cells in the blood (Richardson, 2009).
Apnoea training has been demonstrated to induce beneficial physiological adaptations that help trained breath-hold divers (BHDs), as well as habitual diving populations, to withstand extreme hypoxaemic/hypercapnic conditions. BHDs are known to employ acute increases in lung volume through the thoracic stretching and glossopharyngeal insufflation (Lindholm, 2005) in order to increase gas storage capacity, i.e., increasing oxygen delivery and also allowing more CO2 to be transferred to the lungs during exercice (Schagatay, 2012). Indeed, a previous study has shown that apnoea training increases lung volume and the maximal aerobic capacity (VO2max) of swimmers (Lemaitre, 2009). Apnoea training might stimulate anaerobic glycolysis to compensate for the reduction in aerobic metabolism in working muscles, resulting in increased lactate production during breath-hold exercice whilst cycling (Kume, 2013). Furthemore, the overall CO2 storage in BHDs has been estimated to be twice that of non-divers (Ferretti, 2001). Thus, the increased total Haemoglobin (Hb) resulting from apnoea training noted above might increase buffering capacity for CO2.
Previous reviews suggested that breath-hold divers display beneficial adaptations primarily as a consequence of exposure to training-induced stimuli (Elia et al., 2021), that might contribute to improved sports performance (Lemaître et al., 2010). These adaptations have led researchers to explore the possible use of apnoea training beyond freediving as a means of enhancing performance markers such as: 100, 200 and 400 m swimming time trial (Stavrou et al., 2015; Woorons et al., 2016); 3 km cycling time trial (Bouten et al., 2022); or repeated sprint ability (RSA) (Trincat et al., 2017; Fornasier-Santos et al., 2018; Woorons et al., 2019), defined as an important attribute for athletes competing in high-intensity intermittent sports, such as soccer, basketball, handball, tennis, etc (Bishop and Spencer, 2004).
In line with this ideology, the present article aimed to systematically review the scientific literature and perform a meta-analysis to assess the effects of voluntary apnoea training on markers of aerobic and anaerobic performance, such as maximal aerobic capacity (VO2max) and peak blood lactate concentration ([La]peak).
Methods
A systematic review of the literature was performed according to the Preferred Reporting Items for Systematic Reviews and Meta-Analyses (PRISMA) guidelines (Page and Moher, 2017).
Search strategy
A literature search was conducted on March 2022 by two independent reviewers (DS and JG-R) for the following databases: Web of Science, PubMed and SCOPUS.
Title, abstract and keyword search fields were searched using the following search strategy: “TITLE”: (apnea OR apnoea OR breath hold diving OR free diving OR hypoventilation) NOT (sleep apnea OR sleep apnoea OR obesity hypoventilation syndrome) AND “TITLE, ABSTRACT AND KEYWORDS”: (maximal aerobic capacity OR lactate).
Identification, screening, eligibility assessments and inclusion of studies were performed independently by two reviewers (DS and JG-R) with disagreement settled by a third independent reviewer (FD-F). All records of the literature search were examined by title and abstract to exclude irrelevant records. Studies were selected following the eligibility criteria. Data collected included the author and publication year, sample characteristics, description of intervention and results. If insufficient information was reported, the authors were contacted to confirm additional information about the included studies. Reference lists of selected manuscripts were also screened for any further eligible manuscripts.
Inclusion criteria
The inclusion criteria were 1) peer-reviewed journal publication; 2) clinical trials; 3) healthy humans; 4) short-term (≤6 weeks), medium-term (7–23 weeks) or long-term (≥24 weeks) effects of apnoea training (Ashton et al., 2020), excluding acute warm-up effects of apnoea; 5) variables included markers of aerobic or anaerobic performance, such as blood lactate and VO2max.
Risk of bias in individual studies
A risk of bias assessment was performed using the Cochrane risk-of-bias appraisal tool (Cochrane Cochrane Handbook for Systematic Reviews of Interventions, 2022). The extracted information enabled appraisal of freedom from risk of bias: “Yes” (low risk), “Unclear” (uncertain risk) or “No” (high risk). We applied this approach to each of seven domains: sequence generation (domain I), allocation concealment used to implement the random sequence (II), blinding of participants and study personnel (IIIa), blinding of outcome assessors (IIIb), incomplete outcome data (IV), selective outcome reporting (V), and other sources of bias (VI). Two authors (DS and FG-M) independently reviewed and scored each study, with disagreement settled by a third independent reviewer (F-DF). Kappa index was calculated to analyze the reliability between the two reviewers before contact with the third reviewer.
Quality assesment
Strength of the evidence included in this review was determined using the Grading of Recommendations Assessment, Development and Evaluation (GRADE) scale, which shows the overall certainty of the evidence for the outcome being reviewed (Guyatt et al., 2011). The GRADE scale assesses five factors concerning: risk of bias, inconsistency (calculated heterogeneity), indirectness (evidence addresses review question), imprecision (width of confidence intervals [CIs]) and publication bias (funnel plots). These factors lead to a reported score of: “High,” very confident that the true effect lies close to that of the estimate of the effect; “Moderate,” moderately confident in the effect estímate and the true effect is likely to be close to the estimate of the effect but there is a possibility that it is substantially different; “Low,” confidence in the effect estimate is limited and the true effect may be substantially different from the estimate of the effect; or “Very Low,” very little confidence in the effect estímate and the true effect is likely to be substantially different from the estimate of effect.
Statistical considerations
Meta-analytical procedures were applied to evaluate possible effects of apnoea training (ApT) on the variables studied. Standardised mean difference (SMD) or mean difference (MD) with 95% Confidence Intervals (CI) between ApT and normal breathing (NB) were calculated using a random effects model. It was necessary to contact the authors for further data. Significance for overall effect was set at p < 0.05. Heterogeneity of the analysed studies was assessed using an I-squared (I2) test. Significance level of I2 test was set at p < 0.05. I2 represents the proportion of effects that are due to heterogeneity as opposed to chance (Liberati et al., 2009). Thresholds for low, moderate, and high levels of heterogeneity correspond to I2 values of 25, 50, and 75%, respectively. Within the controlled trial studies, a positive effect indicates a larger improvement in the ApT when compared to the NB on VO2max or [La]peak, while a negative effect means the opposite. Funnel plots (Egger’s test was not possible) were used to assess publication bias (Sterne and Egger, 2001). All statistical analyses were performed using RevMan 5.3.2 (Nordic Cochrane Centre).
Results
Final study selection
538 potential manuscripts were identified following database examination (Figure 1). Snowballing generated seven additional potential manuscripts. After removal of duplicates and elimination of papers based on title and abstract screening, 192 studies remained. Only seven out of 192 studies met the inclusion criteria and were, therefore, included in the meta-analysis (Figure 1).
Level of evidence and quality of the studies
Using the Kappa index, the overall agreement rate between the two extractors of information prior to correcting discrepant items was 0.841. Risk of bias was identified in several studies, in terms of: lack of sample size estimation; low details on random sequence generation; or, lack of clinical trial registration.
The quality assesment of evidence was judged to be moderate for those variables that were significantly enhanced by ApT over NB. Evidence for other variables was judged to be low quality, indicating that further evidence is required before potential benefits can be excluded. Quality of evidence according to the GRADE system is summarised in Table 1.
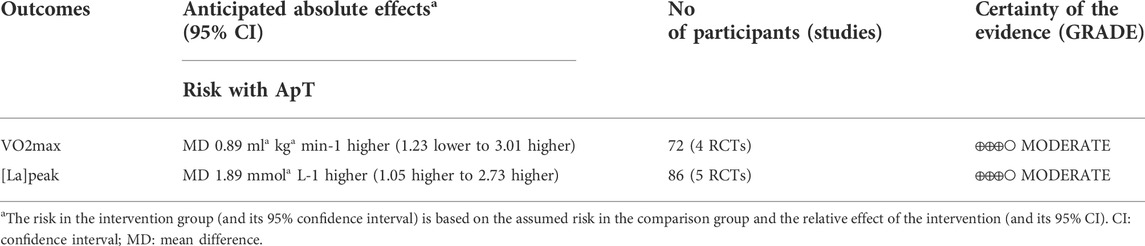
TABLE 1. Grading of Recommendations Assessment, Development, and Evaluation (GRADE) summary of finding.
Characteristics of the participants
Table 2 shows the participant characteristics of the seven studies included in this meta-analysis (total sample size of 127 participants, age 27 ± 9 years old) (Woorons, 2008; Woorons et al., 2019, 2016; Trincat et al., 2017; Fernandez et al., 2018; Fornasier-Santos et al., 2018; Bouten et al., 2022). The total pool of participants was allocated to either ApT (n = 64) or NB (n = 63). Several different types of recreational athletes were enrolled in the studies, including cyclists (Woorons et al., 2019), rugby-players (Fornasier-Santos et al., 2018), breath-hold divers (Fernandez et al., 2018), triathletes (Woorons et al., 2016), swimmers (Trincat et al., 2017), and runners (Woorons, 2008).
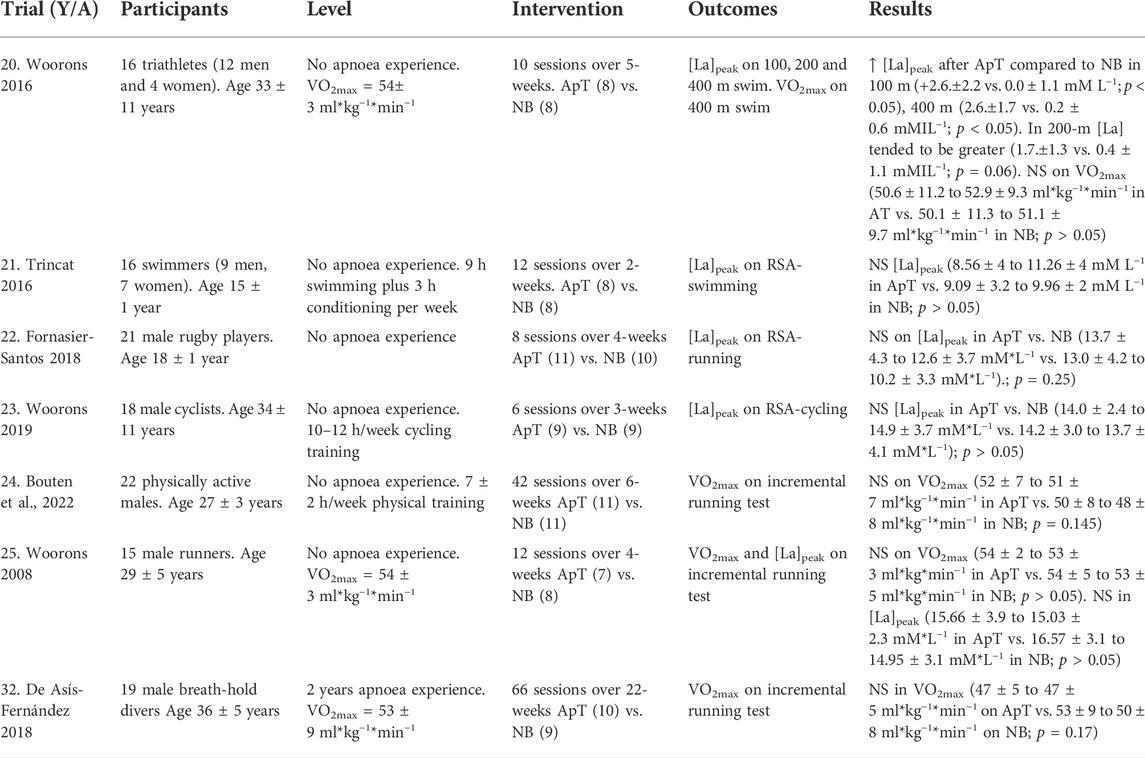
TABLE 2. Characteristics of apnoea training (AT) and normal breathing (NB) of included studies. [La]peak peak blood lactate concentration, VO2max maximal aerobic capacity, NS not statistically significant.
Characteristics of the studies selected
The studies included in the review were published between 2008 and 2022. Mean intervention duration was 8 weeks (range 2–22 weeks). Mean training frequency was 3.6 times per week (range 2–7 times per week). The training stimulus of ApT and NB was recorded as overall training stimulus which consists of: multiplying the Rating of Perceived Exertion (RPE) of the global session by its duration (Woorons, 2008) in four studies (Woorons et al., 2019, 2016; Trincat et al., 2017; Fornasier-Santos et al., 2018); as % oxygen saturation (SpO2) in four studies (Woorons et al., 2019, 2016; Trincat et al., 2017; Fornasier-Santos et al., 2018); as RPE in four studies (Woorons et al., 2019, 2016; Trincat et al., 2017; Fernandez et al., 2018); as HR in three studies (Woorons et al., 2016; Trincat et al., 2017; Fornasier-Santos et al., 2018) and as [La]peak in three studies (Woorons et al., 2016; Trincat et al., 2017; Fornasier-Santos et al., 2018). Results consistently confirmed that oxygen saturation was lower in ApT than NB, but that each of the four papers reported these data in different ways. Tables 2, 3 show the characteristics of the apnoea training interventions for the seven studies included in this review.
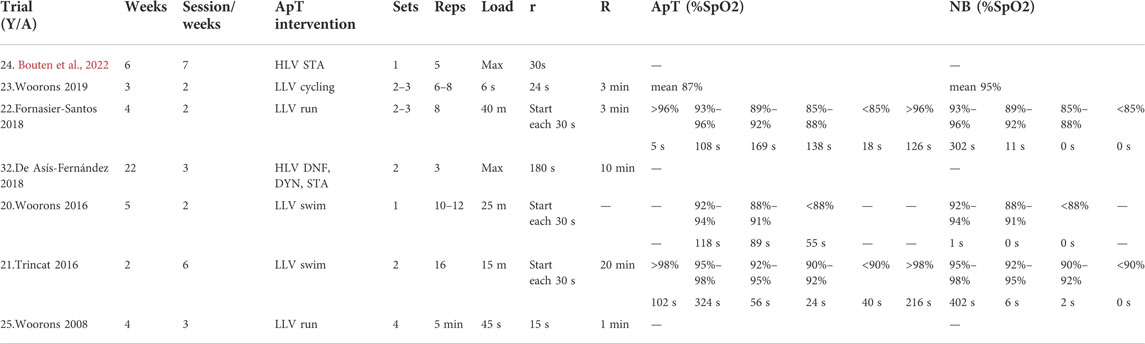
TABLE 3. Apnoea training interventions of included studies. DNF dynamic apnoea no fins DYN dynamic apnoea with fins, HLVDNF, DYN, STA dynamic apnoea no fins, dynamic apnoea fins and static apnoea at high lung volumes, LLVcycling apnoea cycling at low lung volumes, LLVrun apnoea running at low lung volumes, LLVswim apnoea swimming at low lung volumes, r recovery between repetitions, R recovery between sets.
Anaerobic exercise performance
A total of five studies analyzed the effects of ApT on anaerobic exercise performance (Woorons, 2008; Woorons et al., 2019, 2016; Trincat et al., 2017; Fornasier-Santos et al., 2018), with [La]peak the only variable reported. The overall quality of evidence was judged to be moderate evidence for [La]peak (Table 1).
The [La]peak increased to a greater extent following ApT when compared to NB (MD = 1.89 mmol*L−1 [95% CI 1.05, 2.73], Z = 4.40, p < 0.0001). The I2 test showed a non-significant heterogeneity among the included studies (I2 = 0%, p = 0.83). These results are displayed in Figure 2A.
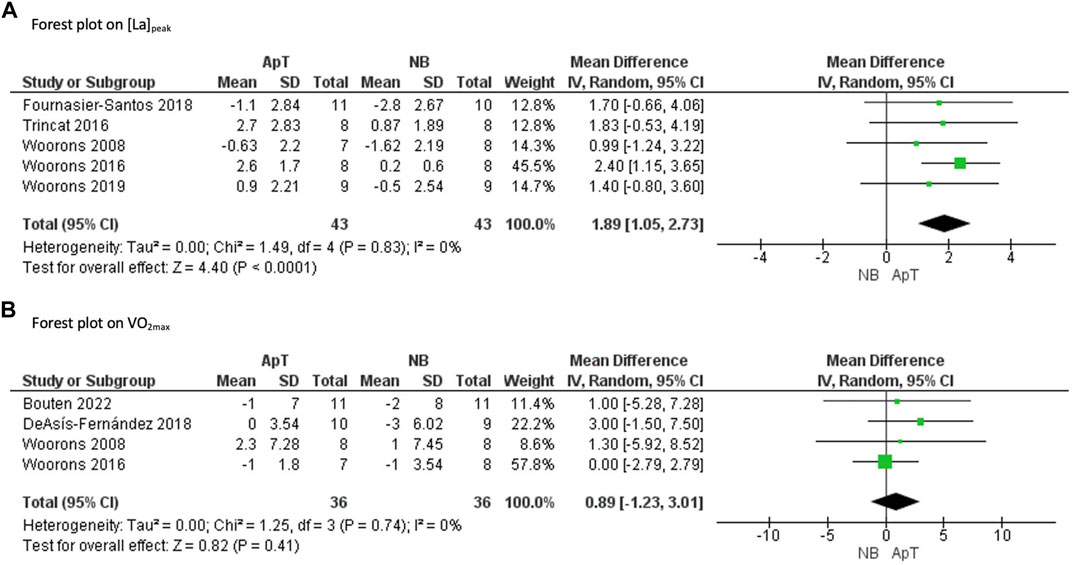
FIGURE 2. Forest plot of the effects of apnoea training (ApT) and Normal Breathing (NB) on [La]peak peak blood lactate concentration (A) and VO2max maximal aerobic capacity (B). CI confidence interval, SD standard deviation, IV weighted mean difference.
Aerobic exercise performance
A total of four studies analyzed the effects of ApT on aerobic exercise performance (Woorons, 2008; Woorons et al., 2016; Fernandez et al., 2018; Bouten et al., 2022), with VO2max the only variable reported. The overall quality of evidence was judged to be moderate evidence on VO2max (Table 1).
Results show that there were no differences in changes to VO2max following ApT compared with NB (MD = 0.89 ml*kg*min−1 [95% CI −1.23, 3.01], Z = 0.82, p = 0.41). The I2 test showed a non-significant heterogeneity among the included studies (I2 = 0%, p = 0.74). These results are displayed in Figure 2B.
Discussion
This is the first review to systematically analyze the longitudinal effects of apnoea training on aerobic and anaerobic performance. The main finding of this systematic review was that longitudinal (2–22 weeks) apnoea training (ApT) increases peak blood lactate concentration to a greater extent than control interventions using normal breathing (NB) in recreational athletes. However, we found no significant increase for maximal aerobic capacity after longitudinal ApT.
In terms of rationale for aerobic performance, cross-sectional data show that trained breath-hold divers exhibit higher lung and splenic volumes (Schagatay, 2012), greater oxygen and carbon dioxide stores in tissues (Liner, 1994), intensified oxygen conserving mechanisms (Costalat, 2015), and blunted ventilatory chemosensitivity (Roecker et al., 2014) compared with non-diving controls. However, the lack of controlled clinical trials previously made it difficult to evaluate the strength of supporting evidence. Although these studies reported some promising data suggesting potential beneficial effects of ApT for exercise performance, methodological limitations must be considered. The physiological adaptations acquired by the BHDs goes beyond an isolated breath-hold stimulus (i.e. stretching, endurance, inspiratory muscle training, swimming, glossopharyngeal insufflation, etc.), so such other interventions are likely confound the effectiveness of ApT. As the effects mentioned above are advantageous in several sports, we hypothesised that ApT may be an effective alternative to hypobaric or normobaric hypoxia to increase aerobic and/or anaerobic performance.
Oxygen supply and transport capacity to the working mucles are important determinants of aerobic performance (Brugniaux, 2006), usually measured by VO2max. Since most of the oxygen in the blood is bound to Hb, this oxygen transport capacity of the body is largely determined by the amount of Hb. Previous studies (Joulia, 2002; Prommer, 2007; Richardson, 2008) have shown a marked correlation between total blood hemoglobin (Hb) and VO2max, furthermore showing that lowering Hb (e.g. after blood donation) decreases oxygen uptake, whereas increasing Hb enhances VO2max. In their study, Schmidt and Prommer (Prommer, 2007) have shown an increase of 1 g in Hb corresponding to an increase of 4 ml kg−1 min−1 in VO2max. In this context, ApT has been proposed as a new hypoxic training method, since serum erythropoietin (EPO) levels increase in a dose-dependent manner in response to reductions in blood oxygenation (Levine, 1997). Hypoxic training effects depend mainly on the conditions of the hypoxia exposure—mainly the severity of hypoxia and length of exposure (de Asís Fernández et al., 2019). Accordingly, a variety of methods have been developed to augment endogenous EPO. For example, the ‘‘live high, train low” method is widely used by endurance athletes (Levine, 1997), consisting of combining resting exposure to hypoxia with normoxic training. Nevertheless, it is possible to generate hypoxaemia without the many challenges of being placed in a hypoxic environment, ie by voluntarily reducing the breathing frequency. It should be noted that hypoxia during ApT can be severe, although it is only for a very short time, far shorter than more chronic exposures to hypobaric hypoxia such as seen in mountaineers (Benedek, 2002).
In the studies included in this review (Woorons, 2008; Woorons et al., 2019, 2016; Trincat et al., 2017; Fernandez et al., 2018; Fornasier-Santos et al., 2018; Bouten et al., 2022), it was reported that athletes could train under hypoxic conditions at sea level through apnoea training at low lung volumes (LLV, i.e., “end-expiratory” breath-hold). During such exercise with ApT at LLV, arterial oxygen saturation dropped to severe hypoxaemia. Conversely, when athletes applied ApT at higher lung volumes (–HLV, i.e., “end-inspiratory” breath-hold) no hypoxic effect occurred.
In addition, the hypoxic stimulus increases long-term splenic volume and contractility, increasing the red blood cell reservoir in hypoxic situations, thus acutely improving oxygen delivery in these conditions. Such an effect would be an effective warm-up to enhance red blood cell volume, however these improvements disappears within 10 min after the last apnoea (Schagatay et al., 2005), which may limit application for longer lasting activities.
Another factor that will influence aerobic performance will be respiratory function. Trained BHDs typically exhibit high lung volumes (Andersson, 2004; Schagatay, 2012; Fernandez et al., 2018), range 5.9–7.3 L of VC, that are higher than in those unaccustomed to ApT. Usually, BHDs training includes concomitant training such as stretching, aerobic training, glossopharyngeal insufflation, or inspiratory muscle training. Such training has shown positive effects on increasing VC (Enright and Unnithan, 2011; Khosravi et al., 2013), but it is not possible to differentiate the effect of ApT on VC through such observational studies. Based on our findings, it is recommended that future studies evaluating the effect of ApT on VC distinguish the effects produced on BHDs (near their physiological limit) from participants without apnoea experience, who would have a greater scope for improvement.
Previous reviews consulted (Lemaitre, 2009; Schagatay, 2010; Elia et al., 2021) all agree that ApT might be an effective alternative to increase hematocrit, EPO, Hb and lung volume; all of them predictors of aerobic fitness. However, in stark contrast, the randomised control trials included in this review (Woorons, 2008; Woorons et al., 2016; Fernandez et al., 2018; Bouten et al., 2022) suggest no effects of ApT on aerobic performance measured by VO2max. It is of course acknowledged that there are many other parameters of endurance performance, beyond VO2max, that are arguably more ecologically valid and these were not assessed in the reported studies. Furthermore, factors such as accelerated recovery processes or muscle buffering capacity could also be important for performance (Bishop et al., 2004) and again were not assessed.
Accordingly, the meta-analysis results might suggest that improvements in anaerobic capacity may be more likely to have influenced the greater improvements in performance following ApT (Stavrou et al., 2015), though other factors cannot yet be excluded. A total of five clinical trials analyzed the effects of ApT on anaerobic exercise performance (Woorons, 2008; Woorons et al., 2019, 2016; Trincat et al., 2017; Fornasier-Santos et al., 2018). These studies showed that ApT seems to be advantageous for improving anaerobic glycolysis. For instance, results showed improved performance of short actions at maximal or near to maximal intensity, separated by short recovery periods and for a relatively long period of time (between 1 and 4 h), which are reflective of sport-specific demands for athletes competing in high-intensity intermittent sports, such as soccer, basketball, handball, tennis, etc (Bishop et al., 2001, p. 2004). During such repeated sprints, the majority of ATP resynthesis comes from anaerobic metabolism, but with progressive sprint number there is a decline in energy provided by the anaerobic system and consequently, despite increased aerobic contribution, muscle power cannot be maintained, with a concomittant decline in performance (Gaitanos et al., 1993).
The meta-analysis revealed increases in [La]peak, without benefits for aerobic markers, supporting the underpinning mechanisms are more related to improvements in anaerobic capacity. Such mechanisms may include increases in glycogen and phosphocreatine availability, enzymatic activity, buffering capacity, or even improved “tolerance” of the exercise-induced acidosis (Bishop et al., 2011, p. 2011). Training adaptations of anaerobic glycolysis may include both increased maximum rates of lactate production and improved muscle-buffering capacity which leads to increase lactate clearance and minimize pH disturbance in muscle (Sharp et al., 1986). The improved [La]peak supports this, but cannot differentiate effects further as net blood lactate values reflect the continuous balance of muscle lactate production and clearance (Brooks, 2018) and both processes may have been affected.
It is necessary to acknowledge several limitations to this systematic review. These include the wide variety of different protocols for apnoea training interventions, such as differences in biomechanics (run, cycling, and swim); training volume and frequency; lung volume (low or high); apnoea duration (6 s to maximal); and recovery (4 s–20 min). For future perspectives, it is recommended that researchers carry-out clinical trials analysing isolated apnoea training effects to find the optimal characteristics of the training method (e.g., training volume, lung volume, apnoea duration or recovery time), as well as using more ecologically valid markers of aerobic performance.
Practical applications
This study highlights the importance that ApT might be an effective intervention to enhace anaerobic performance by increasing peak blood lactate concentration in athletes who practice high-intensity intermittent sports such as, racquet sports, mixed martial arts, or team sports. It may also be of interest for high-intensity continuous sports such as sprinting in swimming, athletics, or cycling.
A great variability has been found in the training methods, for example in the duration of apnoea efforts. Some training methods use maximal apnoeas with long rest periods (Fernandez et al., 2018) while other studies use short-repeated apnoeas with short rest periods (Fornasier-Santos et al., 2018). Repeated apnoea training seems to be the most optimal method for use in athletes unfamiliar with apnoea training, as it is necessary to train an individual’s psychological tolerance of maximal apnoea.
It is possible to generate severe hypoxaemia, increased blood lactate concentration and a greater glycolytic activity without the costs and challenges of being placed in a hypoxic environment, by using apnoea training at low lung volumes, eliciting faster hypoxaemia.
Apnoea training, as with blood flow restriction training, could be a suitable intervention when athletes are injured and not training, or doing rehabilitation with limited high-intensity work.
Conclusion
The meta-analysis findings of improved [La]peak, without benefits for aerobic markers, suggests that the underpinning mechanisms may lie more with improvements in anaerobic capacity. Such improvements may include increases in glycogen and phosphocreatine availability, increased enzymatic activity, buffering capacity, or even improved “tolerance” of the exercise-induced acidosis.
Data availability statement
The original contributions presented in the study are included in the article/supplementary material, further inquiries can be directed to the corresponding author.
Author contributions
JG-R, FG-M, DS, AT and FD-F conceptualized the review and criteria, and, FG-M, DS and FD-F completed the screening and data extraction of data within this manuscript. All authors created the tables and figures. All authors contributed to the writing of the manuscript. All authors read and approved the final manuscript.
Conflict of interest
The authors declare that the research was conducted in the absence of any commercial or financial relationships that could be construed as a potential conflict of interest.
Publisher’s note
All claims expressed in this article are solely those of the authors and do not necessarily represent those of their affiliated organizations, or those of the publisher, the editors and the reviewers. Any product that may be evaluated in this article, or claim that may be made by its manufacturer, is not guaranteed or endorsed by the publisher.
References
Andersson J., Liner M. H., Fredsted A., Schagatay E. K. A. (2004). Cardiovascular and respiratory responses to apneas with and without face immersion in exercising humans. J. Appl. Physiol. 96, 1005–1010. doi:10.1152/japplphysiol.01057.2002
Andersson J., Schagatay E. (2009). Repeated apneas do not affect the hypercapnic ventilatory response in the short term. Eur. J. Appl. Physiol. 105, 569–574. doi:10.1007/s00421-008-0936-y
Ashton R. E., Tew G. A., Aning J. J., Gilbert S. E., Lewis L., Saxton J. M. (2020). Effects of short-term, medium-term and long-term resistance exercise training on cardiometabolic health outcomes in adults: Systematic review with meta-analysis. Br. J. Sports Med. 54, 341–348. doi:10.1136/bjsports-2017-098970
Bain A. R., Ainslie P. N., Hoiland R. L., Barak O. F., Cavar M., Drvis I., et al. (2016). Cerebral oxidative metabolism is decreased with extreme apnoea in humans; impact of hypercapnia. J. Physiol. 594, 5317–5328. doi:10.1113/JP272404
Benedek K., Keri S., Grosz A., Totka Z., Toth E., Benedek G. (2002). Short-term hypobaric hypoxia enhances visual contrast sensitivity. NeuroReport 13, 1063–1066. doi:10.1097/00001756-200206120-00017
Bishop D., Edge J., Goodman C. (2004). Muscle buffer capacity and aerobic fitness are associated with repeated-sprint ability in women. Eur. J. Appl. Physiol. 92, 540–547. doi:10.1007/s00421-004-1150-1
Bishop D., Girard O., Mendez-Villanueva A. (2011). Repeated-sprint ability - part II: Recommendations for training. Sports Med. 41, 741–756. doi:10.2165/11590560-000000000-00000
Bishop D., Spencer M. (2004). Determinants of repeated-sprint ability in well-trained team-sport athletes and endurance-trained athletes. J. Sports Med. Phys. Fit. 44, 1
Bishop D., Spencer M., Duffield R., Lawrence S. (2001). The validity of a repeated sprint ability test. J. Sci. Med. Sport 4, 19–29. doi:10.1016/S1440-2440(01)80004-9
Bouten J., Debusschere J., Lootens L., Declercq L., Van Eenoo P., Boone J., et al. (2022). Six weeks of static apnea training does not affect Hbmass and exercise performance. J. Appl. Physiol. 132, 673–681. doi:10.1152/japplphysiol.00770.2021
Breskovic T., Uglesic L., Zubin P., Kuch B., Kraljevic J., Zanchi J., et al. (2011). Cardiovascular changes during underwater static and dynamic breath-hold dives in trained divers. J. Appl. Physiol. 111, 673–678. doi:10.1152/japplphysiol.00209.2011
Brooks G. A., Arevalo J. A., Osmond A. D., Leija R. G., Curl C. C., Tovar A. P. (2022). Lactate in contemporary biology: A phoenix risen. J. Physiol. 600, 1229–1251. doi:10.1113/JP280955
Brooks G. A. (2018). The science and translation of lactate shuttle theory. Cell Metab. 27, 757–785. doi:10.1016/j.cmet.2018.03.008
Brugniaux J. V., Schmitt L., Robach P., Nicolet G., Fouillot J. P., Moutereau S., et al. (2006). Eighteen days of “living high, training low” stimulate erythropoiesis and enhance aerobic performance in elite middle-distance runners. J. Appl. Physiol. 100, 203–211. doi:10.1152/japplphysiol.00808.2005
Cochrane Handbook for Systematic Reviews of Interventions (2022). Version 6.3. Part 2: Core methods. Chapter 8: Assessing risk of bias in included studies. Cochrane Database Syst. Rev. Available at: https://training.cochrane.org/handbook/current/chapter-08.
Costalat G., Pichon A., Joulia F., Lemaitre F. (2015). Modeling the diving bradycardia: Toward an “oxygen-conserving breaking point”. Eur. J. Appl. Physiol. 115, 1475–1484. doi:10.1007/s00421-015-3129-5
de Asís Fernández F., González-Mohino F., González-Ravé J. M. (2019). Assessment of sensory sensitivity through critical flicker fusion frequency thresholds after a maximum voluntary apnoea. Diving Hyperb. Med. 49, 186–191. doi:10.28920/dhm49.3.186-191
Elia A., Gennser M., Harlow P. S., Lees M. J. (2021). Physiology, pathophysiology and (mal)adaptations to chronic apnoeic training: A state-of-the-art review. Eur. J. Appl. Physiol. 121, 1543–1566. doi:10.1007/s00421-021-04664-x
Enright S. J., Unnithan V. B. (2011). Effect of inspiratory muscle training intensities on pulmonary function and work capacity in people who are healthy: A randomized controlled trial. Phys. Ther. 91, 894–905. doi:10.2522/ptj.20090413
Fernandez F. A., Martin-Martin R., Garcia-Camacha I., Juarez D., Fidel P., Gonzalez-Rave J. M. (2018). Medium term effects of physical conditioning on breath-hold diving performance. Respir. Physiol. Neurobiol. 259, 70–74. doi:10.1016/j.resp.2018.07.013
Ferretti G. (2001). Extreme human breath-hold diving. Eur. J. Appl. Physiol. 84, 254–271. doi:10.1007/s004210000377
Fornasier-Santos C., Millet G. P., Woorons X. (2018). Repeated-sprint training in hypoxia induced by voluntary hypoventilation improves running repeated-sprint ability in rugby players. Eur. J. Sport Sci. 18, 504–512. doi:10.1080/17461391.2018.1431312
Gaitanos G. C., Williams C., Boobis L. H., Brooks S. (1993). Human muscle metabolism during intermittent maximal exercise. J. Appl. Physiol. 75, 712–719. doi:10.1152/jappl.1993.75.2.712
Guyatt G. H., Oxman A. D., Vist G., Kunz R., Brozek J., Alonso-Coello P., et al. (2011). GRADE guidelines: 4. Rating the quality of evidence - study limitations (risk of bias). J. Clin. Epidemiol. 64, 407–415. doi:10.1016/j.jclinepi.2010.07.017
Joulia F., Steinberg J. G., Wolff F., Gavarry O., Jammes Y. (2002). Reduced oxidative stress and blood lactic acidosis in trained breath-hold human divers. Respir. Physiol. Neurobiol. 133, 121–130. doi:10.1016/s1569-9048(02)00133-7
Khosravi M., Tayebi S. M., Safari H. (2013). Single and concurrent effects of endurance and resistance training on pulmonary function. Iran. J. Basic Med. Sci. 16, 628
Kume D., AkahoShi S., Song J., YamagaTa T., WakimoTo T., NagaoM. , et al. (2013). Intermittent breath holding during moderate bicycle exercise provokes consistent changes in muscle oxygenation and greater blood lactate response. J. Sports Med. Phys. Fit. 53, 327
Lemaître F., Joulia F., Chollet D. (2010). Apnea: A new training method in sport? Med. Hypotheses 74, 413–415. doi:10.1016/j.mehy.2009.09.051
Lemaitre F., Seifert L., Polin D., Juge J., Tourny-Chollet C., Chollet D. (2009). Apnea training effects on swimming coordination. J. Strength Cond. Res. 23, 1909–1914. doi:10.1519/JSC.0b013e3181b073a8
Levine B. D., Stray-Gundersen J. (1997). Living high-training low”: Effect of moderate-altitude acclimatization with low-altitude training on performance. J. Appl. Physiol. 83, 102–112. doi:10.1152/jappl.1997.83.1.102
Liberati A., Altman D. G., Tetzlaff J., Mulrow C., Gøtzsche P. C., Ioannidis J. P. A., et al. (2009). The PRISMA statement for reporting systematic reviews and meta-analyses of studies that evaluate health care interventions: Explanation and elaboration. PLoS Med. 6, e1000100. doi:10.1371/journal.pmed.1000100
Lindholm P., Blogg S. L., Gennser M. (2007). Pulse oximetry to detect hypoxemia during apnea: Comparison of finger and ear probes. Aviat. Space Environ. Med. 78, 770
Lindholm P., Nyren S. (2005). Studies on inspiratory and expiratory glossopharyngeal breathing in breath-hold divers employing magnetic resonance imaging and spirometry. Eur. J. Appl. Physiol. 94, 646–651. doi:10.1007/s00421-005-1358-8
Liner M., Linnarsson D. (1994). Tissue oxygen and carbon dioxide stores and breath-hold diving in humans. J. Appl. Physiol. 77, 542–547. doi:10.1152/jappl.1994.77.2.542
Page M. J., Moher D. (2017). Evaluations of the uptake and impact of the preferred reporting items for systematic reviews and meta-analyses (PRISMA) statement and extensions: A scoping review. Syst. Rev. 6, 263. doi:10.1186/s13643-017-0663-8
Prommer N., Heinicke K., Viola T., Cajigal J., Behn C., Schmidt W. F. J. (2007). Long-term intermittent hypoxia increases O2-transport capacity but not VO2max. High. Alt. Med. Biol. 8, 225–235. doi:10.1089/ham.2007.8309
Richardson M., de Bruijn R., Schagatay E. (2009). Hypoxia augments apnea-induced increase in hemoglobin concentration and hematocrit. Eur. J. Appl. Physiol. 105, 63–68. doi:10.1007/s00421-008-0873-9
Richardson M., Lodin A., Reimers J., Schagatay E. (2008). Short-term effects of normobaric hypoxia on the human spleen. Eur. J. Appl. Physiol. 104, 395–399. doi:10.1007/s00421-007-0623-4
Roecker K., Metzger J., Scholz T., Tetzlaff K., Sorichter S., Walterspacher S. (2014). Modified ventilatory response characteristics to exercise in breath-hold divers. Int. J. Sports Physiol. Perform. 9, 757–765. doi:10.1123/ijspp.2013-0308
Schagatay E., Haughey H., Reimers J. (2005). Speed of spleen volume changes evoked by serial apneas. Eur. J. Appl. Physiol. 93, 447–452. doi:10.1007/s00421-004-1224-0
Schagatay E. (2010). Predicting performance in competitive apnea diving, part II: Dynamic apnoea. Diving Hyperb. Med. 40, 11
Schagatay E., Richardson M. X., Lodin-Sundstrom A. (2012). Size matters: Spleen and lung volumes predict performance in human apneic divers. Front. Physiol. 3, 173. doi:10.3389/fphys.2012.00173
Sharp R. L., Costill D. L., Fink W. J., King D. S. (1986). Effects of eight weeks of bicycle ergometer sprint training on human muscle buffer capacity. Int. J. Sports Med. 7, 13–17. doi:10.1055/s-2008-1025727
Stavrou V., Toubekis A. G., Karetsi E. (2015). Changes in respiratory parameters and fin-swimming performance following a 16-week training period with intermittent breath holding. J. Hum. Kinet. 49, 89–98. doi:10.1515/hukin-2015-0111
Sterne J. A. C., Egger M. (2001). Funnel plots for detecting bias in meta-analysis: Guidelines on choice of axis. J. Clin. Epidemiol. 54, 1046–1055. doi:10.1016/S0895-4356(01)00377-8
Trincat L., Woorons X., Millet G. P. (2017). Repeated-sprint training in hypoxia induced by voluntary hypoventilation in swimming. Int. J. Sports Physiol. Perform. 12, 329–335. doi:10.1123/ijspp.2015-0674
Truszczynski O., Wojtkowiak M., Biernacki M., Kowalczuk K. (2009). The effect of hypoxia on the critical flicker fusion threshold in pilots. Int. J. Occup. Med. Environ. Health 22, 13–18. doi:10.2478/v10001-009-0002-y
Woorons X., Bourdillon N., Vandewalle H., Lamberto C., Mollard P., Richalet J. P., et al. (2010). Exercise with hypoventilation induces lower muscle oxygenation and higher blood lactate concentration: Role of hypoxia and hypercapnia. Eur. J. Appl. Physiol. 110, 367–377. doi:10.1007/s00421-010-1512-9
Woorons X., Millet G. P., Mucci P. (2019). Physiological adaptations to repeated sprint training in hypoxia induced by voluntary hypoventilation at low lung volume. Eur. J. Appl. Physiol. 119, 1959–1970. doi:10.1007/s00421-019-04184-9
Woorons X., Mollard P., Pichon A., Duvallet A., Richalet J. P., Lamberto C. (2008). Effects of a 4-week training with voluntary hypoventilation carried out at low pulmonary volumes. Respir. Physiol. Neurobiol. 160, 123S1569–130. doi:10.1016/j.resp.2007.09.010
Keywords: breath-hold, sports performance, lactate, VO2max, endurance
Citation: de Asís-Fernández F, Sereno D, Turner AP, González-Mohíno F and González-Ravé JM (2022) Effects of apnoea training on aerobic and anaerobic performance: A systematic review and meta-analysis. Front. Physiol. 13:964144. doi: 10.3389/fphys.2022.964144
Received: 08 June 2022; Accepted: 15 August 2022;
Published: 27 September 2022.
Edited by:
Jan Jacek Kaczor, University of Gdansk, PolandReviewed by:
Troy J. Cross, The University of Sydney, AustraliaDanilo Cialoni, Dan Europe Foundation, Italy
Copyright © 2022 de Asís-Fernández, Sereno, Turner, González-Mohíno and González-Ravé. This is an open-access article distributed under the terms of the Creative Commons Attribution License (CC BY). The use, distribution or reproduction in other forums is permitted, provided the original author(s) and the copyright owner(s) are credited and that the original publication in this journal is cited, in accordance with accepted academic practice. No use, distribution or reproduction is permitted which does not comply with these terms.
*Correspondence: Fernando González-Mohíno, ZmVybmFuZG8uZ21heW9yYWxhc0B1Y2xtLmVz