- 1Division of Metabolism and Endocrinology, Faculty of Medicine, Saga University, Saga, Japan
- 2Japan Society for the Promotion of Science, Tokyo, Japan
- 3Fukuoka University Institute for Physical Activity, Fukuoka University, Fukuoka, Japan
- 4Faculty of Sports and Health Science, Fukuoka University, Fukuoka, Japan
- 5Liver Center, Saga University Hospital, Saga, Japan
Epidemiological evidence suggests that there is a link between diabetes and mood disorders, such as depression and anxiety. Although peripheral or central inflammation may explain this link, the molecular mechanisms are not fully understood and few effective treatments for diabetes or mood disorders are available. In the present study, we aimed to determine whether transforming growth factor (TGF)-β2, an anti-inflammatory substance, might represent a potential therapeutic agent for diabetes-related mood behaviors. TGF-β2 expression in the hippocampus is affected by anxiolytic drugs and stress exposure, it is able to cross the blood-brain barrier, and it is as an exercise-induced physiological adipokine that regulates glucose homeostasis. Therefore, we hypothesized that a chronic TGF-β2 infusion would ameliorate diabetes-related glucose intolerance and mood dysregulation. To determine the effects of the chronic administration of TGF-β2 on diabetes, we implanted osmotic pumps containing TGF-β2 into type 2 diabetic mice (db/db mice), and age-matched non-diabetic control wild type mice and db/db mice were infused with vehicle (PBS), for 12 consecutive days. To assess anxiety-like behaviors and glucose homeostasis, the mice underwent elevated plus maze testing and intraperitoneal glucose tolerance testing. Hippocampal and perigonadal visceral white adipose tissue perigonadal white adipose tissue samples were obtained 12 days later. Contrary to our hypothesis, TGF-β2 infusion had no effect on diabetes-related glucose intolerance or diabetes-related behavioral defects, such as inactivity. In db/db mice, the expression of inflammatory markers was high in pgWAT, but not in the hippocampus, and the former was ameliorated by TGF-β2 infusion. The expression of brain-derived neurotrophic factor and neuronal nitric oxide synthase, important regulators of anxiety-like behaviors, was low in db/db mice, but TGF-β2 infusion did not affect their expression. We conclude that although TGF-β2 reduces the expression of pro-inflammatory markers in the adipose tissue of diabetic mice, it does not ameliorate their obesity or mood dysregulation.
Introduction
Obesity is becoming increasingly prevalent worldwide and predisposes people toward systemic metabolic diseases, such as type 2 diabetes (T2D). T2D is associated with complications in both the peripheral and central nervous system (CNS) (McCrimmon et al., 2012). In particular, epidemiological evidence has suggested that there is a close link between T2D and mood disorders. Being overweight, even in the absence of T2D, is associated with low hippocampal volume, which is important for mood regulation (Cherbuin et al., 2015). Patients with T2D have high incidences of several anxiety and affective disorders (7–123% higher than those of healthy, community-dwelling adults) (Fisher et al., 2008), and mood disorders, such as major depressive disorder (MDD), are present in a quarter of people with T2D (Semenkovich et al., 2015). However, the mechanisms underlying diabetes-related mood behaviors are still poorly understood, because of the complex bidirectional relationship between these disorders, and there are few effective treatments (Semenkovich et al., 2015).
Animal studies have shown that db/db mice, which a model of T2D, show abnormal behaviors, including depression-like or anxiety-like behaviors (Dinel et al., 2011; Liu et al., 2017; Fourrier et al., 2019; Zhang et al., 2019). Brain-derived neurotrophic factor (BDNF) in the hippocampus is important for mood regulation (Hashimoto et al., 2004) and its expression is low in db/db mice (Dinel et al., 2011; Shima et al., 2017; Wosiski-Kuhn et al., 2018). It has been shown that hippocampal BDNF expression is regulated by molecules including neuronal nitric oxide synthase (nNOS, encoded by Nos1) in the hippocampus (Stanquini et al., 2018). The results of several previous studies have suggested that peripheral or central inflammation may explain the low BDNF expression in T2D. Chronic inflammation, involving high serum tumor necrosis factor α (TNF-α) concentrations, accompanies large subcutaneous and visceral adipocytes (Winkler et al., 2003), and is associated with insulin resistance and T2D in both humans and rodents (Coppack, 2001; Lumeng and Saltiel, 2011; Wada et al., 2013). However, in the CNS, including the hippocampus, there have been conflicting findings regarding the expression of pro-inflammatory markers, such as TNF-α and interleukin (IL)-6 in db/db and diet-induced obese mice (Lavin et al., 2009; Dinel et al., 2011; Liu et al., 2017; Fourrier et al., 2019).
Transforming growth factor (TGF)-β is a widely expressed multifunctional growth factor that is also anti-inflammatory (Yoshimura et al., 2010). Three isoforms (TGF-β1, TGF-β2, and TGF-β3) have been identified in mammals. All three are present in the CNS, and TGF-β2 is the most abundant. Notably, the mRNA expression of TGF-β2 is higher in the hippocampus than in the cortex, striatum, brain stem, and cerebellum (Unsicker et al., 1991). Hippocampal Tgfb2 is an anxiolytic drug-responsive gene (Lee et al., 2010): its expression is increased by anxiolytic drugs (Lee et al., 2010) and reduced by chronic, mild, unpredictable stress in a mouse model of depression (Grassi et al., 2017). In hippocampal neurons, TGF-β2 also acutely regulates synaptic plasticity and activates cAMP response element-binding protein (CREB) (Fukushima et al., 2007), an upstream regulator of BDNF (Conti et al., 2002). In a recent study, it was shown that TGF-β2 is a physical exercise-induced adipokine that improves the glucose homeostasis of high-fat diet (HFD)-fed obese mice (Takahashi et al., 2019). TGF-β2, but not TGF-β1, can cross the blood-brain barrier (Kastin et al., 2003; McLennan et al., 2005); thus, circulating TGF-β2 can affect the peripheral nervous system and directly access the CNS. Therefore, in the present study, we aimed to determine whether chronic TGF-β2 infusion ameliorates the glucose intolerance and behavioral deficits of db/db mice. In addition, we aimed to determine the effects of TGF-β2 infusion on hippocampal BDNF, its upstream molecules, and the expression of genes encoding pro-inflammatory proteins in db/db mice.
Materials and methods
Animals
Five-week-old male db/db (BKS.Cg-m+/+Leprdb/Jcl) and age-matched control wild type (WT) mice were purchased from CLEA (Tokyo, Japan). The number of mice used was determined by reference to previous studies (Bruchas et al., 2009; Tomiga et al., 2020). The mice were housed in an accredited animal facility that was maintained at a constant temperature (23.8 ± 0.2°C) and humidity (50.4 ± 1.9%), with a 12-h light/dark cycle, and were provided food and water ad libitum. The experiments were approved by the Saga University Animal Care and Use Committee (approval number: 1707075) and conducted in accordance with the regulations on animal experimentation at Saga University.
Osmotic pump infusion of TGF-β2 in vivo
After 1 week of acclimatization, two mice were placed into each cage, to avoid social isolation, and cage-mates were not changed during the experiment. Chronic TGF-β2 infusion was performed as previously described (Takahashi et al., 2019). Briefly, the animals were randomly allocated to three groups at 10 weeks of age: WT + vehicle; db/db + vehicle, and db/db + TGF-β2 groups. Under isoflurane anesthesia (induction: 4%, maintenance: 2%), the mice underwent surgery to implant Alzet mini-osmotic pumps (model 1002, Durect, Cupertino, CA, United States) subcutaneously. The osmotic pumps were filled with recombinant TGF-β2 (8406LC, Cell Signaling Technologies, Danvers, MA, United States) diluted in PBS, or PBS alone for the WT + vehicle and db/db + vehicle groups. For 12 consecutive days, the mice received 12 ng TGF-β2 each per hour. After euthanasia, the pumps were removed and the contents were confirmed to have been completely discharged. The dose and duration of treatment were those used in a previous study (Takahashi et al., 2019).
Behavioral testing
We assessed anxiety-like behaviors using the elevated plus maze (EPM) test, as previously described (Tomiga et al., 2021). The mice were transferred to the testing room a minimum of 60 min prior to each testing session. All the tests were performed between 11:00 and 14:00, during the light period (80–100 lux). Before each test, the testing apparatus was thoroughly cleaned with 70% (v/v) ethanol and dried to reduce olfactory cues. Briefly, the maze consisted of four arms (each arm was 30-cm long and 5-cm wide) and was placed 40 cm above the floor. Two arms contained side and end walls that were 15 cm high (the closed arms), and the other two arms had no walls (the open arms). The behavior of the mice was recorded using a video camera. The mice were placed in the center of the maze, facing an open arm, and were then allowed to explore for 5 min. Each arm entry, the resting time, and the time spent on slow and fast movement were analyzed using Smart 3.0 software (Panlab, Barcelona, Spain). The movement speed thresholds were defined as 1) rest: < 2.50 cm/s; 2) slow movement: 2.50–15.00 cm/s; 3) fast movement: >15.00 cm/s.
Glucose tolerance testing (GTT)
The mice were fasted for 15–16 h, with free access to drinking water. Baseline blood samples were collected from the tails of fully conscious mice, which were then intraperitoneally administered glucose (1 g/kg). Fifteen, 30, 60, and 120 min later, blood samples were collected from the tail and their glucose concentrations were measured using a glucose meter (Stat Strip XP3, Nipro, Osaka, Japan).
Tissue collection and weighing
The mice were euthanized at 12 weeks of age under isoflurane anesthesia. Blood samples were obtained and serum was isolated by centrifugation and frozen at −80°C until analyzed. The hippocampus, perigonadal white adipose tissue (pgWAT), and subcutaneous white adipose tissue (scWAT) were rapidly collected, and the tissues were placed in a tube containing RNA stabilization solution (Thermo Fisher Scientific, Waltham, MA, United States). The hippocampus and adipose tissue depots were weighed using an analytical balance. The mass of the tube plus the RNA stabilization solution was measured in advance, and the hippocampal mass was calculated by subtraction. Adipose tissue depots were rapidly weighed, and then portions of the pgWAT were immersed in RNA stabilization solution. After overnight incubation, the tissues were stored at −80°C for subsequent analysis. Because the mice were housed in pairs, total food intake was calculated in grams per cage per day (WT + vehicle: 6.085 ± 0.305 g/cage/day; db/db + vehicle: 11.79 ± 0.025 g/cage/day; and db/db + TGF-β2: 12.42 ± 0.29 g/cage/day.)
Serum insulin analysis
Serum insulin concentration was measured using an ELISA kit (Morinaga, Kanagawa, Japan), according to the manufacturer’s instructions.
Western blot
Protein lysates were prepared as described previously and aliquots containing 10 µg protein were separated by electrophoresis on a 14.0% (w/v) sodium dodecyl sulfate polyacrylamide gel and transferred to polyvinylidene fluoride membrane (Millipore, Billerica, MA, United States) using the semi-dry method (Tomiga et al., 2021). After transfer, Ponceau S staining (Beacle, Inc., Kyoto, Japan) was used to verify consistent loading, and the membrane was blocked with 3% (w/v) skim milk at room temperature for 1 h. The membrane was then incubated overnight at 4°C with the following primary antibodies: anti-BDNF (1:1,000; Abcam, ab108319; Cambridge, United Kingdom), which detects the precursor (proBDNF; 32 kDa) and mature (15 kDa) forms of BDNF, and anti-nNOS (#611852, BD Biosciences, San Jose, CA, United States). The membrane was then incubated with horseradish peroxidase-conjugated secondary antibodies (Vector Laboratories, Burlingame, CA, United States) for 1 h. The bound antibodies were detected using SuperSignal™ West Pico Plus Chemiluminescent Substrate (Thermo Fisher Scientific) and analyzed using the Fusion-FX7 imaging system (Vilber Lourmat, Marne-la-Vallée, France). Band densities were determined using ImageJ software (NIH, Bethesda, MD, United States) and the Ponceau S signal intensity was used as a loading control.
Gene expression analysis
Hippocampal tissue and pgWAT were homogenized using a tissue homogenizer. RNA was extracted from the samples using a FastGene RNA Basic Kit (Nippon Gene, Tokyo, Japan). and assessed for concentration and purity using a NanoDrop 2000 (Thermo Fisher Scientific). The isolated RNA was then reverse transcribed to cDNA using PrimeScript RT Master Mix (Takara Bio, Otsu, Japan). qRT-PCR analyses were then performed using the QuantStudio 3 Real-Time PCR system (Applied Biosystems, Foster City, CA, United States). The mRNA expression of Nos1a and Bdnf (coding region, exon IX) was quantified using SYBR Green Master Mix (Applied Biosystems). The brain and pgWAT target gene expression was normalized to that of Gapdh or Arbp mRNA and quantified using the ΔΔCt method. All the samples were analyzed in duplicate. The primer sequences are listed in Table 1.
Statistics
Data are presented as the mean ± standard error (SE). Statistical analyses were performed using Prism version 7.0 (GraphPad, San Diego, CA, United States). One-way ANOVA, followed by Bonferroni’s post-hoc test, was used to compare groups. Non-parametric datasets were compared using the Kruskal–Wallis test. Relationships between continuous variables were evaluated using Pearson’s product-moment correlations. p < 0.05 was considered to represent statistical significance.
Results
TGF-β2 infusion does not affect the body mass gain or glucose tolerance of db/db mice
The body and adipose tissue masses of the db/db mice were significantly higher than those of the WT mice, but their hippocampal masses were similar (Figures 1A,B). In addition, the db/db mice had high fasting serum insulin and blood glucose concentrations and lower glucose tolerance than the WT mice (Figures 1C–F). TGF-β2 administration did not affect these parameters (Figure 1).
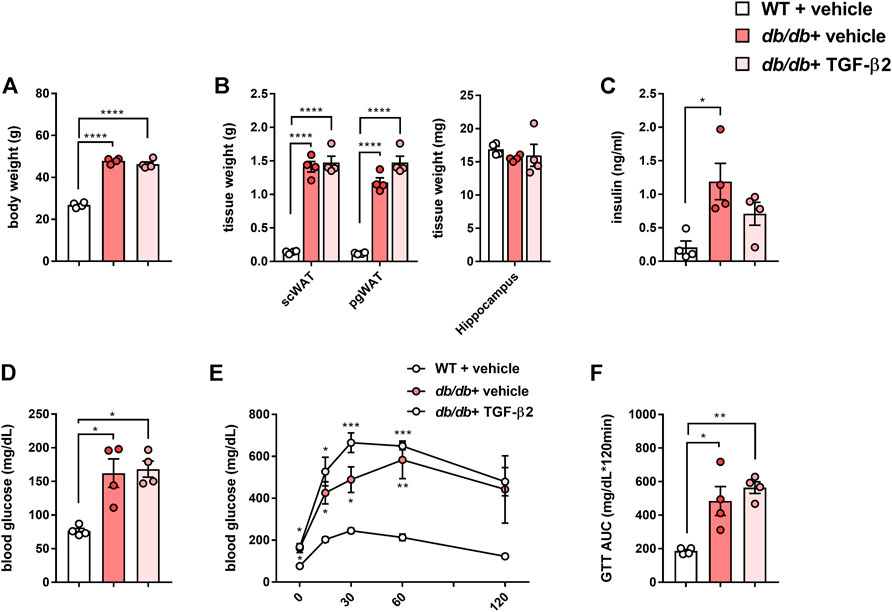
FIGURE 1. TGF-β2 infusion has no effects on the body mass gain or glucose tolerance of db/db mice (A) Body mass (B) scWAT, pgWAT, and hippocampal masses, (C) fasting serum insulin concentration (D) blood glucose concentration, (E) results of glucose tolerance testing, and (F) area under the glucose curve (AUC) for WT, db/db + vehicle, and db/db + TGF-β2 mice. Data are presented as the mean ± SE (n = 4/group). The dots represent individual data points. ****p < 0.0001; ***p < 0.001; **p < 0.01; *p < 0.05.
TGF-β2 infusion has no effect on the low activity of db/db mice
To characterize the behavior of the db/db mice, we performed EPM testing. Although there was no difference in the number of open arm entries (Figure 2A), there were significantly fewer closed arm entries (Figure 2B). This smaller number of closed arm entries implies less anxiety-like behavior, but the total number of open and closed arm entries was also lower in the db/db mice (Figure 2C). In addition, the db/db mice spent more time resting during EPM testing than the WT mice (Figure 2D). Next, we analyzed the speed of movement of the mice in both the open and closed arms. With respect to the open arms, there was no difference in the time spent on slow movement among the groups, but the db/db mice spent less time moving rapidly (Figure 2E). With respect to the closed arms, the db/db mice spent less time on both slow and fast movement (Figure 2F). With respect to the combination of the open and closed arms, the db/db mice spent less time moving both slowly and rapidly (Figure 2G). However, there were no differences in these parameters between the db/db + TGF-β2 group and the db/db + vehicle group. Thus, the low level of locomotor activity of the diabetic mice was not ameliorated by TGF-β2 infusion.
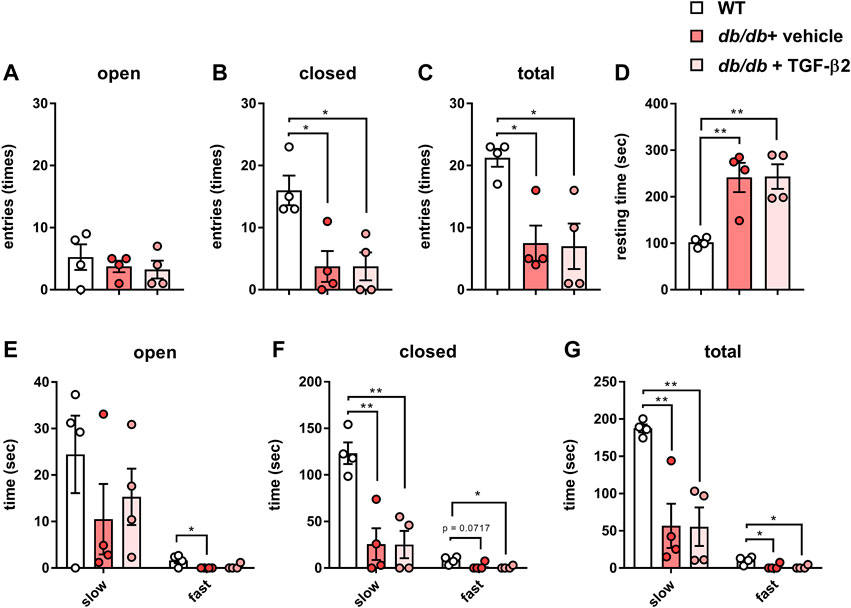
FIGURE 2. TGF-β2 infusion has no effect on the low locomotor activity of db/db mice. Numbers of (A) open, (B) closed, and (C) total arm entries (D) Resting time and time spent on slow and fast movement in the (E) open arms, (F) closed arms, and (G) both open and closed arms for the db/db + vehicle and db/db + TGF-β2 mice. Data are presented as the mean ± SE (n = 4/group). The dots represent individual data points. **p < 0.01; *p < 0.05.
TGF-β2 infusion reduces pro-inflammatory marker expression in the pgWAT but not in the hippocampus of db/db mice
In pgWAT, the expression of genes encoding pro-inflammatory molecules was significantly higher in the db/db + vehicle group than in the control group; however, this expression was lower in the db/db + TGF-β2 group (Figure 3A). There were no differences in the expression of genes encoding pro-inflammatory molecules in the hippocampus among the groups (Figure 3B). Hippocampal Tgfb2 mRNA expression was also unaffected by the db/db genotype or the TGF-β2 infusion (Figure 3C).
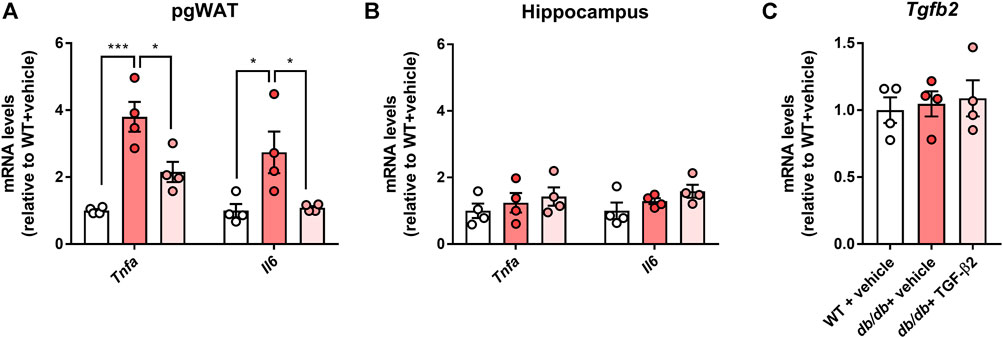
FIGURE 3. TGF-β2 infusion reduces the expression of genes encoding pro-inflammatory molecules in pgWAT, but not in the hippocampus, of db/db mice. Tnfa and Il6 mRNA expression in the (A) pgWAT and (B) hippocampus (C) Hippocampal Tgfb2 mRNA expression in WT, db/db + vehicle, and db/db + TGF-β2 mice. Data are presented as the mean ± SE (n = 4/group). The dots represent individual data points. ***p < 0.001; *p < 0.05.
TGF-β2 infusion does not alter the low BDNF and nNOS protein and mRNA expression of db/db mice
The hippocampal Bdnf mRNA expression was lower in the db/db + vehicle and db/db + TGF-β2 groups than in the WT + vehicle group (Figure 4A). The mRNA expression of the HFD-responsive and anxiety-related gene Nos1a was lower in db/db mice than in control mice, but TGF-β2 infusion had no effect on this (Figure 4A). Consistent with the mRNA data, TGF-β2 infusion did not affect the lower hippocampal BDNF and nNOS protein levels found in the db/db mice (Figures 4B,C). The expression of proBDNF in the hippocampus did not differ among the groups (Figures 4B,C). The hippocampal BDNF and nNOS protein levels negatively correlated with the pgWAT Tnfa mRNA expression (Figures 5A,B), but not with the Il6 mRNA expression (Figures 5C,D).
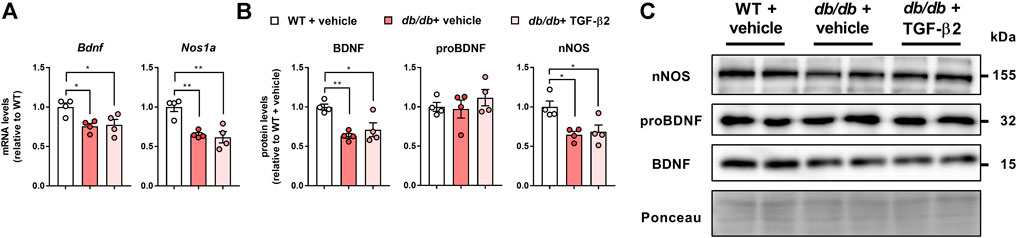
FIGURE 4. TGF-β2 infusion does not affect the low mRNA and protein expression of BDNF and nNOS in db/db mice (A) Bdnf and Nos1a mRNA expression, and (B) representative immunoblots and (C) quantification of BDNF, proBDNF, and nNOS protein levels in the hippocampi of WT, db/db + vehicle, and db/db + TGF-β2 mice. The Ponceau S signal intensity was used as a loading control for western blot analysis. Data are presented as the mean ± SE (n = 4/group). The dots represent individual data points. **p < 0.01; *p < 0.05.
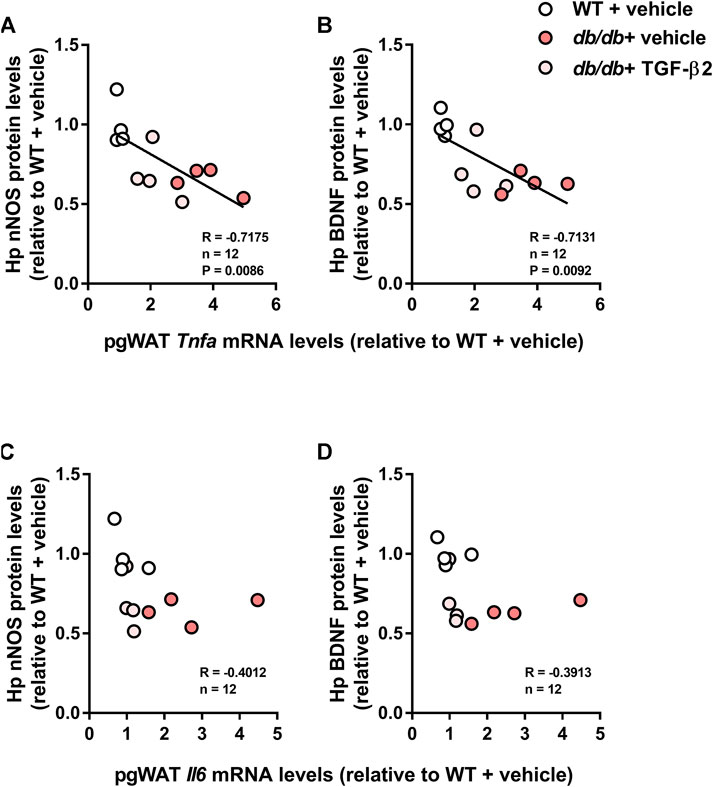
FIGURE 5. Hippocampal BDNF and nNOS protein levels correlate with pgWAT Tnfa mRNA expression, but not Il6 mRNA expression, in WT, db/db, and TGF-β2 infused db/db mice. Correlations of pgWAT Tnfa mRNA expression with (A) hippocampal nNOS and (B) BDNF protein level. Correlations of pgWAT Il6 mRNA expression with (C) hippocampal nNOS and (D) BDNF protein level in WT, db/db + vehicle, and db/db + TGF-β2 mice. The lines on the scatter plots show significant correlations (n = 4/group).
Discussion
Epidemiological studies have shown links between T2D and mood disorders (Fisher et al., 2008; McCrimmon et al., 2012; Cherbuin et al., 2015), and several studies have shown that peripheral and central inflammatory signaling may explain these links. Therefore, we aimed to determine whether the anti-inflammatory cytokine TGF-β2 would ameliorate obesity, glucose dyshomeostasis, and anxiety-like behaviors using a mouse model of T2D that was chronically infused with TGF-β2. The major findings of the study were as follows: 1) TGF-β2 ameliorated the abnormal expression of pro-inflammatory markers in adipose tissue, but had no effects on the glucose intolerance, anxiety-like behaviors, or low hippocampal BDNF and nNOS expression of db/db mice; and 2) the hippocampal BDNF and nNOS protein levels correlated with the expression of the pro-inflammatory gene Tnfa, but not Il6, in adipose tissue.
Contrary to our hypothesis, there were no marked effects of TGF-β2 infusion on the glucose tolerance of db/db mice (Figure 1). Although the effects of TGF-β2 in diabetes have been studied in the context of complications, such as retinopathy (Kita et al., 2007; Yang et al., 2010), its effects on diabetes and glucose metabolism are poorly understood. Previously, TGF-β2 treatment was found to improve the glucose tolerance and insulin sensitivity of HFD-fed obese mice (Takahashi et al., 2019), but these effects were not identified in the present study. The reason for this discrepancy is not readily apparent, but may be related to differences in the signaling pathways mediating glucose uptake in skeletal muscle in db/db and diet-induced obese mice (Halseth et al., 2002; Iglesias et al., 2002). Takahashi et al. demonstrated that TGF-β2 normalizes the infiltration of macrophages into the adipose tissue of obese mice, and similarly, we have demonstrated a TGF-β2-induced reduction in pro-inflammatory gene expression in the pgWAT of db/db mice (Figure 3A). Therefore, in severe obesity, such as that present in db/db mice, TGF-β2 treatment can reduce the degree of inflammation, but might be insufficient to improve glucose homeostasis. Ibuprofen, an anti-inflammatory drug, also has no effect on blood glucose concentration in a model of type 1 DM (Qiao et al., 2015) or on the circulating concentrations of pro-inflammatory markers, such as Il-6 and Il-1β, in db/db mice (Fourrier et al., 2019). Thus, it may be possible that the physiological effects of anti-inflammatory drugs are blunted in diabetic model rodents.
The number of closed arm entries and the total number of arm entries during EPM testing were significantly lower in db/db mice (Figure 2). In general, a reduction in the number of closed arm entries implies an amelioration of anxiety-like behaviors. However, the total number of entries was also low, which indicates lower locomotor activity, and it has been previously shown that rodents with diabetes move around less (Hussain et al., 2019). A previous methodological study showed that the total number of arm entries represents a useful index of anxiety-like behaviors (Komada et al., 2008). Thus, the present findings might imply that db/db mice have significant anxiety, similar to that described previously (Dinel et al., 2011; Fourrier et al., 2019). This conclusion is supported by the larger amount of time that db/db mice spent resting during EPM testing (Figure 2D). We found that hippocampal pro-inflammatory marker gene expression was not affected by the db/db phenotype or TGF-β2 treatment (Figure 3B). These findings suggest that central inflammation might not occur in db/db mice and might not contribute to their diabetes-related behavioral abnormalities.
Previously, it has been shown that hippocampal BDNF and nNOS affect cognitive function, including mood-related behaviors, in obesity (Molteni et al., 2002; Cai et al., 2016; Tomiga et al., 2019). In the hippocampus, because the selective inhibition of nNOS increases BDNF protein levels, nNOS is considered to be an upstream regulator of BDNF (Stanquini et al., 2018). Diabetes impairs hippocampal function (Stranahan et al., 2008). The present data suggest that BDNF and nNOS expression is low at both the mRNA and protein levels in the hippocampus of db/db mice. The low expression of BDNF in db/db mice is consistent with the results of previous studies of other mouse models of diabetes (Wosiski-Kuhn et al., 2018; Hussain et al., 2019).
BDNF is initially synthesized as a precursor, proBDNF, which is subsequently cleaved to form mature BDNF. Patients with major depressive disorders show low serum BDNF concentrations, while their serum proBDNF concentrations are high (Jiang et al., 2017). In a previous study, opposite trends were identified with respect to BDNF and proBDNF, and corticosterone was shown to increase hippocampal proBDNF expression, which negatively correlated with the number of open arm entries (Lin et al., 2022). However, we found that the proBDNF protein levels did not differ among the groups in the present study. Because the mature BDNF protein levels in the hippocampi of db/db mice were low, it is possible that the proteolytic cleavage of proBDNF is impaired in the CNS in severe obesity.
In the present study, the diabetes-related behavioral defects of the db/db mice were not ameliorated by the TGF-β2 treatment. Originally, we hypothesized that circulating TGF-β2 and endogenous Tgfb2 expression represent potential therapeutic targets for mood regulation. Previously, it was reported that circulating TGF-β2, but not TGF-β1, can cross the intact blood-brain barrier (Kastin et al., 2003; McLennan et al., 2005). TGF-β2 has been shown to positively regulate synaptic plasticity and activate CREB in hippocampal neurons (Fukushima et al., 2007). In addition, hippocampal Tgfb2 mRNA expression is upregulated by anxiolytic drugs (Lee et al., 2010) and downregulated by chronic stress (Grassi et al., 2017).
TGF-β2 expression is increased by potassium chloride-induced neuronal activity in cultured hippocampal neurons (Grassi et al., 2017). In addition, running exercise increases the circulating TGF-β2 concentration (Takahashi et al., 2019). nNOS and BDNF expression are regulated by the increase in neuronal activity that accompanies physical exercise (Zhang and Wong-Riley, 1999; Lu, 2003; Tomiga et al., 2021). Because physical exercise is commonly recommended for the management of T2D and the associated brain dysfunction, we aimed to determine whether TGF-β2 treatment would mimic the effect of exercise and its relationship with hippocampal nNOS and BDNF expression. However, consistent with the behavioral findings, TGF-β2 administration did not affect the low hippocampal nNOS and BDNF protein and mRNA expression in the db/db mice. In addition, because hippocampal Tgfb2 mRNA was not affected by TGF-β2 administration, it may be inferred that exogenous TGF-β2 does not affect neuronal activation in the hippocampus in T2D. These findings suggest that circulating TGF-β2 might not affect the hippocampal regulation of mood in db/db mice.
The low hippocampal nNOS expression was contrary to our expectation. nNOS is the major NOS isoform in the adult brain (Bredt et al., 1994) and acts as a source of nitric oxide (NO) in the CNS. NO is considered to exert neuroprotective effects at low-to-moderate concentrations, but becomes neurotoxic at high concentrations (Abbott and Nahm, 2004). Zhang et al. showed that treatment with a selective serotonin reuptake inhibitor reduced hippocampal nNOS expression, and treatment with the nNOS-selective inhibitor 7-nitroindazole reduced anxiety-like behaviors (Zhang et al., 2010). Consistent with these findings, we previously reported that HFD-induced obesity is associated with high hippocampal nNOS expression (Tomiga et al., 2017), and that the expression level correlates with body mass and visceral adipose tissue accumulation (Tomiga et al., 2019). Physical activity, such as running, which is recognized to play a role in the maintenance of mental health, increases BDNF expression and reduces hippocampal nNOS expression (Tomiga et al., 2021). Therefore, the reason for the low nNOS expression in the hippocampi of the T2D db/db mice is not readily apparent. One possible mechanism might be nNOS protein turnover. We previously reported that hippocampal Nos1 mRNA expression is low in the initial phase of HFD-induced obesity, prior to the upregulation of nNOS protein (Tomiga et al., 2019). Conversely, in the later phase of obesity, when hippocampal nNOS protein levels are high, Nos1 mRNA expression is low (Tomiga et al., 2019). Given these findings, when severe obesity develops, as in db/db mice, the low mRNA expression during the early phase of obesity might lead to low hippocampal nNOS protein levels. In addition, it has been shown that nNOS is degraded by the ubiquitin proteasome pathway (Bender et al., 2000). We can speculate that T2D might affect protein degradation pathways in the CNS and might contribute to these differences. In addition, in rodents with streptozotocin-induced type 1 diabetes, both low (Reagan and McEwen, 2002) and high (Guo et al., 2017) hippocampal nNOS levels have been identified. These findings suggest that hippocampal nNOS regulation differs according to the phase of obesity and might be not explained by differences in glucose homeostasis alone; therefore, further studies are needed.
Both the hippocampal BDNF and nNOS protein levels negatively correlated with pgWAT Tnfa mRNA expression, but not Il6 mRNA expression (Figure 5). Several previous studies have shown that anxiety-like or depression-like behaviors in db/db mice are ameliorated by the intracerebroventricular (i.c.v.) or systemic administration of a TNF-α inhibitor (Fourrier et al., 2019; Alshammari et al., 2020). Similarly, the blockade of IL-6 receptor has an antidepressant effect in rodents with social defeat stress (Zhang et al., 2017). Interestingly, although the i. c.v. administration of TNF-α provokes an anxiogenic response, IL-6 has no effects on anxiety-like behaviors (Connor et al., 1998). These findings suggest that IL-6 itself might not affect central mood regulation directly. Given these findings, it is possible that peripherally-derived TNF-α in db/db mice might contribute to central mood regulation. Although our data does not confirm a causal relationship, the correlations between pgWAT Tnfa expression and the expression of hippocampal mood regulators, such as BDNF and nNOS, are consistent with this.
There were several limitations to the present study. First, we could not confirm that TGF-β2 had direct effects in the CNS in the db/db mice. McLennan et al. have shown that the entry of acutely administered TGF-β2 reaches a peak after 10 min in both the circulation and the brain, after which the concentration of TGF-β2 in the brain remains stable for at least 45 min (McLennan et al., 2005). We confirmed an anti-inflammatory effect of TGF-β2 in the pgWAT of db/db mice, which implies that the TGF-β2 entered the circulation at least. However, given that above findings, and although they provide indirect evidence, it can be inferred that TGF-β2 enters the CNS. Second, we did not include a TGF-β2-treated WT group. Therefore, it is unclear whether the effects of TGF-β2 are specific to the diabetic condition or whether it would have similar effects in normal animals. These limitations will be addressed in future studies.
In conclusion, in the present study, we have demonstrated behavioral defects, including in the resting time and movement speed of db/db mice. These behaviors and the low hippocampal BDNF and nNOS expression of db/db mice are not improved by chronic TGF-β2 infusion. In addition, the high body and adipose tissue masses and glucose intolerance of db/db mice are unaffected by chronic TGF-β2 infusion, and the adipose tissue pro-inflammatory marker gene expression of db/db mice is comparable to that of non-diabetic WT mice. These data suggest that although TGF-β2 ameliorates inflammation in T2D, it would not have a therapeutic effect in patients with T2D-induced mood alteration.
Data availability statement
The original contributions presented in the study are included in the article/supplementary material, further inquiries can be directed to the corresponding author.
Ethics statement
The animal study was reviewed and approved by Saga University Animal Care and Use Committee.
Author contributions
YT, YH, KA, and HT conceived and designed the study. YT and HT conducted the experiments, and analyzed and interpreted the data. YT wrote the first draft of the manuscript. All the authors contributed to manuscript revision, and read and approved the submitted version.
Funding
This work was supported by the Japanese Society for the Promotion of Science KAKENHI (grant numbers 20K19502 and 20J00439), and the Nakatomi Foundation.
Acknowledgments
The quantitative RT-PCR, ELISA, and western blotting analyses were conducted at the Analytical Research Center for Experimental Sciences, Saga University. We thank Mark Cleasby, PhD from Edanz (https://jp.edanz.com/ac) for editing a draft of this manuscript.
Conflict of interest
The authors declare that the research was conducted in the absence of any commercial or financial relationships that could be construed as a potential conflict of interest.
Publisher’s note
All claims expressed in this article are solely those of the authors and do not necessarily represent those of their affiliated organizations, or those of the publisher, the editors and the reviewers. Any product that may be evaluated in this article, or claim that may be made by its manufacturer, is not guaranteed or endorsed by the publisher.
References
Abbott L. C., Nahm S. S. (2004). Neuronal nitric oxide synthase expression in cerebellar mutant mice. Cerebellum 3, 141–151. doi:10.1080/14734220410031927
Alshammari M. A., Khan M. R., Majid Mahmood H., Alshehri A. O., Alasmari F. F., Alqahtani F. M., et al. (2020). Systemic TNF-α blockade attenuates anxiety and depressive-like behaviors in db/db mice through downregulation of inflammatory signaling in peripheral immune cells. Saudi Pharm. J. 28, 621–629. doi:10.1016/j.jsps.2020.04.001
Bender A. T., Demady D. R., Osawa Y. (2000). Ubiquitination of neuronal nitric-oxide synthase in vitro and in vivo. J. Biol. Chem. 275, 17407–17411. doi:10.1074/jbc.M000155200
Bredt D. S., Snyder S. H., Hwang P. M. (1994). Transient nitric oxide synthase neurons in embryonic cerebral cortical plate, sensory ganglia, and olfactory epithelium. Neuron 13, 301–313. doi:10.1016/0896-6273(94)90348-4
Bruchas M. R., Land B. B., Lemos J. C., Chavkin C. (2009). CRF1-R activation of the dynorphin/kappa opioid system in the mouse basolateral amygdala mediates anxiety-like behavior. PLoS One 4, e8528–21. doi:10.1371/journal.pone.0008528
Cai M., Wang H., Li J. J., Zhang Y. L., Xin L., Li F., et al. (2016). The signaling mechanisms of hippocampal endoplasmic reticulum stress affecting neuronal plasticity-related protein levels in high fat diet-induced obese rats and the regulation of aerobic exercise. Brain Behav. Immun. 57, 347–359. doi:10.1016/j.bbi.2016.05.010
Cherbuin N., Fraser M., Sachdev P., Anstey K. J. (2015). Being overweight is associated with hippocampal atrophy : the PATH through life study. Int. J. Obes. 39, 1509–1514. doi:10.1038/ijo.2015.106
Connor T. J., Song C., Leonard B. E., Merali Z., Anisman H. (1998). An assessment of the effects of central interleukin-1beta, -2, -6, and tumor necrosis factor-alpha administration on some behavioural, neurochemical, endocrine and immune parameters in the rat. Neuroscience 84, 923–933. doi:10.1016/S0306-4522(97)00533-2
Conti A. C., Cryan J. F., Dalvi A., Lucki I., Blendy J. A. (2002). cAMP response element-binding protein is essential for the upregulation of brain-derived neurotrophic factor transcription, but not the behavioral or endocrine responses to antidepressant drugs. J. Neurosci. 22, 3262–3268. doi:10.1523/JNEUROSCI.22-08-03262.2002
Coppack S. W. (2001). Pro-inflammatory cytokines and adipose tissue. Proc. Nutr. Soc. 60, 349–356. doi:10.1079/pns2001110
Dinel A. L., André C., Aubert A., Ferreira G., Layé S., Castanon N. (2011). Cognitive and emotional alterations are related to hippocampal inflammation in a mouse model of metabolic syndrome. PLoS One 6, e24325. doi:10.1371/journal.pone.0024325
Fisher L., Skaff M. M., Mullan J. T., Arean P., Glasgow R., Masharani U. (2008). A longitudinal study of affective and anxiety disorders, depressive affect and diabetes distress in adults with type 2 diabetes. Diabet. Med. 25, 1096–1101. doi:10.1111/j.1464-5491.2008.02533.x
Fourrier C., Bosch-Bouju C., Boursereau R., Sauvant J., Aubert A., Capuron L., et al. (2019). Brain tumor necrosis factor-α mediates anxiety-like behavior in a mouse model of severe obesity. Brain Behav. Immun. 77, 25–36. doi:10.1016/j.bbi.2018.11.316
Fukushima T., Liu R.-Y., Byrne J. H. (2007). Transforming growth factor-beta2 modulates synaptic efficacy and plasticity and induces phosphorylation of CREB in hippocampal neurons. Hippocampus 17, 5–9. doi:10.1002/hipo.20243
Grassi D., Franz H., Vezzali R., Bovio P., Heidrich S., Dehghanian F., et al. (2017). Neuronal activity, TGFβ-signaling and unpredictable chronic stress modulate transcription of Gadd45 family members and DNA methylation in the hippocampus. Cereb. Cortex 27, 4166–4181. doi:10.1093/cercor/bhx095
Guo F., Yue H., Wang L., Ding C., Wu L., Wu Y., et al. (2017). Vitamin D supplement ameliorates hippocampal metabolism in diabetic rats. Biochem. Biophys. Res. Commun. 490, 239–246. doi:10.1016/j.bbrc.2017.06.028
Halseth A. E., Ensor N. J., White T. A., Ross S. A., Gulve E. A. (2002). Acute and chronic treatment of ob/ob and db/db mice with AICAR decreases blood glucose concentrations. Biochem. Biophys. Res. Commun. 294, 798–805. doi:10.1016/S0006-291X(02)00557-0
Hashimoto K., Shimizu E., Iyo M. (2004). Critical role of brain-derived neurotrophic factor in mood disorders. Brain Res. Brain Res. Rev. 45, 104–114. doi:10.1016/j.brainresrev.2004.02.003
Hussain Y., Jain S. K., Samaiya P. K. (2019). Short-term westernized (HFFD) diet fed in adolescent rats: Effect on glucose homeostasis, hippocampal insulin signaling, apoptosis and related cognitive and recognition memory function. Behav. Brain Res. 361, 113–121. doi:10.1016/j.bbr.2018.12.042
Iglesias M. A., Ye J. M., Frangioudakis G., Saha A. K., Tomas E., Ruderman N. B., et al. (2002). AICAR administration causes an apparent enhancement of muscle and liver insulin action in insulin-resistant high-fat-fed rats. Diabetes 51, 2886–2894. doi:10.2337/diabetes.51.10.2886
Jiang H., Chen S., Li C., Lu N., Yue Y., Yin Y., et al. (2017). The serum protein levels of the tPA-BDNF pathway are implicated in depression and antidepressant treatment. Transl. Psychiatry 7, e1079–5. doi:10.1038/tp.2017.43
Kastin A. J., Akerstrom V., Pan W. (2003). Circulating TGF-beta1 does not cross the intact blood-brain barrier. J. Mol. Neurosci. 21, 43–48. doi:10.1385/JMN:21:1:43
Kita T., Hata Y., Kano K., Miura M., Nakao S., Noda Y., et al. (2007). Transforming growth factor-beta2 and connective tissue growth factor in proliferative vitreoretinal diseases: possible involvement of hyalocytes and therapeutic potential of rho kinase inhibitor. Diabetes 56, 231–238. doi:10.2337/db06-0581
Komada M., Takao K., Miyakawa T. (2008). Elevated plus maze for mice. J. Vis. Exp. (22), e1088. doi:10.3791/1088
Lavin D. N., Joesting J. J., Chiu G. S., Moon M. L., Meng J., Dilger R. N., et al. (2009). Fasting induces an anti-inflammatory effect on the neuroimmune system which a high-fat diet prevents. Obesity 19, 1586–1594. doi:10.1038/oby.2011.73
Lee J. H., Ko E., Kim Y. E., Min J. Y., Liu J., Kim Y., et al. (2010). Gene expression profile analysis of genes in rat hippocampus from antidepressant treated rats using DNA microarray. BMC Neurosci. 11, 152. doi:10.1186/1471-2202-11-152
Lin L., Herselman M. F., Zhou X. F., Bobrovskaya L. (2022). Effects of corticosterone on BDNF expression and mood behaviours in mice. Physiol. Behav. 247, 113721. doi:10.1016/j.physbeh.2022.113721
Liu W., Liu J., Xia J., Xue X., Wang H., Qi Z., et al. (2017). Leptin receptor knockout-induced depression-like behaviors and attenuated antidepressant effects of exercise are associated with STAT3/SOCS3 signaling. Brain Behav. Immun. 61, 297–305. doi:10.1016/j.bbi.2017.01.001
Lu B. (2003). BDNF and activity-dependent synaptic modulation. Learn. Mem. 10, 86–98. doi:10.1101/lm.54603
Lumeng C. N., Saltiel A. R. (2011). Inflammatory links between obesity and metabolic disease. J. Clin. Invest. 121, 2111–2117. doi:10.1172/JCI57132
McCrimmon R. J., Ryan C. M., Frier B. M. (2012). Diabetes and cognitive dysfunction. Lancet 379, 2291–2299. doi:10.1016/S0140-6736(12)60360-2
McLennan I. S., Weible M. W., Hendry I. A., Koishi K. (2005). Transport of transforming growth factor-β2 across the blood-brain barrier. Neuropharmacology 48, 274–282. doi:10.1016/j.neuropharm.2004.10.005
Molteni R., Barnard R. J., Ying Z., Roberts C. K., Gómez-Pinilla F. (2002). A high-fat, refined sugar diet reduces hippocampal brain-derived neurotrophic factor, neuronal plasticity, and learning. Neuroscience 112, 803–814. doi:10.1016/S0306-4522(02)00123-9
Qiao W., Wang C., Chen B., Zhang F., Liu Y., Lu Q., et al. (2015). Ibuprofen attenuates cardiac fibrosis in streptozotocin-induced diabetic rats. Cardiology 131, 97–106. doi:10.1159/000375362
Reagan L. P., McEwen B. S. (2002). Diabetes, but not stress, reduces neuronal nitric oxide synthase expression in rat hippocampus: Implications for hippocampal synaptic plasticity. Neuroreport 13, 1801–1804. doi:10.1097/00001756-200210070-00022
Semenkovich K., Brown M. E., Svrakic D. M., Lustman P. J. (2015).Depression in type 2 diabetes mellitus : Prevalence , impact , and treatment. Drugs 75, 577–587. doi:10.1007/s40265-015-0347-4
Shima T., Matsui T., Jesmin S., Okamoto M., Soya M., Inoue K., et al. (2017). Moderate exercise ameliorates dysregulated hippocampal glycometabolism and memory function in a rat model of type 2 diabetes. Diabetologia 60, 597–606. doi:10.1007/s00125-016-4164-4
Stanquini L. A., Biojone C., Guimarães F. S., Joca S. R. (2018). Repeated treatment with nitric oxide synthase inhibitor attenuates learned helplessness development in rats and increases hippocampal BDNF expression. Acta Neuropsychiatr. 30, 127–136. doi:10.1017/neu.2017.28
Stranahan A. M., Arumugam T. V., Cutler R. G., Lee K., Egan J. M., Mattson M. P. (2008). Diabetes impairs hippocampal function through glucocorticoid-mediated effects on new and mature neurons. Nat. Neurosci. 11, 309–317. doi:10.1038/nn2055
Takahashi H., Alves C. R. R., Stanford K. I., Middelbeek R. J. W., Nigro P., Ryan R. E., et al. (2019). TGF-β2 is an exercise-induced adipokine that regulates glucose and fatty acid metabolism. Nat. Metab. 1, 291–303. doi:10.1038/s42255-018-0030-7
Tomiga Y., Yoshimura S., Ito A., Nakashima S., Kawanaka K., Uehara Y., et al. (2017). Exercise training rescues high fat diet-induced neuronal nitric oxide synthase expression in the hippocampus and cerebral cortex of mice. Nitric Oxide 66, 71–77. doi:10.1016/j.niox.2017.03.002
Tomiga Y., Yoshimura S., Ra S.-G., Takahashi Y., Goto R., Kugimoto I., et al. (2019). Anxiety-like behaviors and hippocampal nNOS in response to diet-induced obesity combined with exercise. J. Physiol. Sci. 69, 711–722. doi:10.1007/s12576-019-00686-5
Tomiga Y., Sakai K., Nakashima S., Uehara Y., Kawanaka K., Higaki Y. (2020). Effects of inosine monophosphate and exercise training on neuronal nitric oxide synthase in the mouse brain. Neurosci. Lett. 734, 135083. doi:10.1016/j.neulet.2020.135083
Tomiga Y., Sakai K., Ra S.-G., Kusano M., Ito A., Uehara Y., et al. (2021). Short-term running exercise alters DNA methylation patterns in neuronal nitric oxide synthase and brain-derived neurotrophic factor genes in the mouse hippocampus and reduces anxiety-like behaviors. FASEB J. 35, e21767. doi:10.1096/fj.202100630R
Unsicker K., Flanders K. C., Cissel D. S., Lafyatis R., Sporn M. B. (1991). Transforming growth factor beta isoforms in the adult rat central and peripheral nervous system. Neuroscience 44, 613–625. doi:10.1016/0306-4522(91)90082-y
Wada T., Onogi Y., Kimura Y., Nakano T., Fusanobori H., Ishii Y., et al. (2013). Cilostazol ameliorates systemic insulin resistance in diabetic db/db mice by suppressing chronic inflammation in adipose tissue via modulation of both adipocyte and macrophage functions. Eur. J. Pharmacol. 707, 120–129. doi:10.1016/j.ejphar.2013.03.016
Winkler G., Kiss S., Keszthelyi L., Sápi Z., Öry I., Salamon F., et al. (2003). Expression of tumor necrosis factor (TNF)-α protein in the subcutaneous and visceral adipose tissue in correlation with adipocyte cell volume, serum TNF-α, soluble serum TNF-receptor-2 concentrations and C-peptide level. Eur. J. Endocrinol. 149, 129–135. doi:10.1530/eje.0.1490129
Wosiski-Kuhn M., Bota M., Snider C. A., Wilson S. P., Venkataraju K. U., Osten P., et al. (2018). Hippocampal brain-derived neurotrophic factor determines recruitment of anatomically connected networks after stress in diabetic mice. Hippocampus 28, 900–912. doi:10.1002/hipo.23018
Yang H., Huang Y., Chen X., Liu J., Lu Y., Bu L., et al. (2010). The role of CTGF in the diabetic rat retina and its relationship with VEGF and TGF-β2, elucidated by treatment with CTGFsiRNA. Acta Ophthalmol. 88, 652–659. doi:10.1111/j.1755-3768.2009.01641.x
Yoshimura A., Wakabayashi Y., Mori T. (2010). Cellular and molecular basis for the regulation of inflammation by TGF-beta. J. Biochem. 147, 781–792. doi:10.1093/jb/mvq043
Zhang C., Wong-Riley M. (1999). Expression and regulation of NMDA receptor subunit R1 and neuronal nitric oxide synthase in cortical neuronal cultures: Correlation with cytochrome oxidase. J. Neurocytol. 28, 525–539. doi:10.1023/A:1007053204929
Zhang J., Huang X.-Y., Ye M., Luo C., Wu H.-Y., Hu Y., et al. (2010). Neuronal nitric oxide synthase alteration accounts for the role of 5-HT1A receptor in modulating anxiety-related behaviors. J. Neurosci. 30, 2433–2441. doi:10.1523/JNEUROSCI.5880-09.2010
Zhang J. C., Yao W., Dong C., Yang C., Ren Q., Ma M., et al. (2017). Blockade of interleukin-6 receptor in the periphery promotes rapid and sustained antidepressant actions: a possible role of gut-microbiota-brain axis. Transl. Psychiatry 7, e1138. doi:10.1038/tp.2017.112
Keywords: type 2 diabetes, BDNF (brain derived neurotrophic factor), hippocampus, TGF-β2, anxiety
Citation: Tomiga Y, Higaki Y, Anzai K and Takahashi H (2022) Behavioral defects and downregulation of hippocampal BDNF and nNOS expression in db/db mice did not improved by chronic TGF-β2 treatment. Front. Physiol. 13:969480. doi: 10.3389/fphys.2022.969480
Received: 15 June 2022; Accepted: 26 July 2022;
Published: 25 August 2022.
Edited by:
Ovidiu Constantin Baltatu, Anhembi Morumbi University, BrazilReviewed by:
Yanxia Lu, Shandong University, ChinaMaria Joana Guimarães Pinto, University of Coimbra, Portugal
Copyright © 2022 Tomiga, Higaki, Anzai and Takahashi. This is an open-access article distributed under the terms of the Creative Commons Attribution License (CC BY). The use, distribution or reproduction in other forums is permitted, provided the original author(s) and the copyright owner(s) are credited and that the original publication in this journal is cited, in accordance with accepted academic practice. No use, distribution or reproduction is permitted which does not comply with these terms.
*Correspondence: Yuki Tomiga, dG9taWdhMDUwN0BnbWFpbC5jb20=