- Animal Nutrition Institute, Feed Engineering Research Centre of Sichuan Province, Sichuan Agricultural University, Chengdu, China
The objective of this study was to compare the bioavailability of zinc (Zn) from zinc-glycine (Zn-Gly) and zinc-methionine (Zn-Met) as compared with zinc sulfate (ZnSO4) used as a standard in broilers. A total of 1,200 one-day-old male broilers (Cobb 500) were randomly allotted to one of 10 treatments with eight replicate cages of 15 birds each. The broilers were fed a corn-soybean meal basal diet (containing 26.46 mg Zn/kg; control) or the basal diet added with 40, 80, and 120 mg Zn/kg as Zn-Gly, Zn-Met, or ZnSO4 for 14 days. The relative bioavailability value (RBV) was calculated based on multiple linear regression slope ratios of Zn concentrations in tibia and pancreas, pancreas metallothionein (MT) concentration, and pancreas MT mRNA abundance on added Zn intake. When comparing the control with all Zn-supplemented treatments, Zn addition did not significantly affect average feed intake and bodyweight gain during days 1–14 (p > 0.10). However, Zn concentrations in the tibia, pancreas, and liver and pancreas MT concentration and MT mRNA abundance increased in all Zn-supplemented treatments compared with the control (p < 0.05), and these indices increased linearly (p < 0.001) with increasing added Zn levels on days 7 and 14. The RBV of Zn as Zn-Met was similar to that as Zn-Gly or ZnSO4 (p > 0.40) on days 7 and 14, based on tibia and pancreas Zn. In contrast, on days 7 and 14, the RBVs of Zn were in the following order: Zn-Met > Zn-Gly > ZnSO4 (p < 0.05), based on pancreas MT concentration. The bioavailable Zn from Zn-Met was 1.20 or 1.25 times that from Zn-Gly on day 7 or 14, respectively, evaluated by pancreas MT content. The RBV of Zn as Zn-Met was similar to that as Zn-Gly or ZnSO4 on day 7, whereas it was higher than that as Zn-Gly or ZnSO4 on day 14, based on pancreas MT mRNA abundance. In conclusion, Zn-Met had higher bioavailable Zn than Zn-Gly for the starter broilers fed with the corn-soybean meal diet, using pancreas MT concentration as the response criterion.
Introduction
Zinc (Zn) is one of the essential trace minerals for the growth and development of broilers (Baltaci et al., 2018). It plays an important role in protein and DNA synthesis and the metabolism of lipids and carbohydrates because Zn ion is an essential cofactor of some metalloenzymes involved in these biological processes (McCall et al., 2000). The National Research Council (1994) reported the zn requirement of the starter broiler was 40 mg/kg in the purified or semipurified diet based on the growth performance of broilers. However, when the corn-soybean meal is used, the Zn requirement is 60 mg of Zn/kg of diet for the starter broiler assessed by the tibia Zn content (Wedekind et al., 1992). Huang et al. (2007) also found that the optimal Zn requirement of chicks from hatch to 21 days of age was 59.15 mg/kg for pancreas Zn and 61.70 mg/kg for bone Zn in a corn-soybean meal diet; however, the optimal dietary Zn level of the starter broilers was 84 mg/kg assessed by the serum 5′-nucleotidase activity and pancreas Zn transporter 2 mRNA level. Hence, the differences in the Zn requirement for the starter broiler partly lie in the differences in the basal diet and the choice of responsive parameters.
Recently, considerable attention has been focused on the bioavailability of Zn from different supplemental Zn sources for broiler chickens. Supplemental Zn must be used in broiler diets to optimize the biological responses of Zn because the Zn concentration in the natural feedstuff ingredients does not meet the requirement of broiler chickens (Shao et al., 2019). The growth performance and Zn content in different tissues are the biomarkers to assess the Zn bioavailability from different Zn sources when the dietary Zn level is below the Zn requirement of chickens (Wedekind and Baker, 1990; Batal et al., 2001). Bioavailability of Zn from Zn-methionine chelate compared with Zn sulfate (ZnSO4; set as 100%) are 124% and 177%, respectively, assessed by weight gain and tibia Zn of broilers (Wedekind et al., 1992). A disadvantage of growth rate assays lies in the fact that the method requires the use of semipurified diets, which may yield results not entirely applicable when practical diets containing natural ingredients are fed. Star et al. (2012) reported that the relative bioavailability of one Zn-proteinate complex was 1.64 compared with zinc sulfate as a reference zinc source (1.00) using tibia Zn content as a response parameter. However, the growth performance is not sensitive to evaluate the Zn bioavailability since it can be affected by many nutrient factors in practical diets (Wedekind et al., 1992; Sandoval et al., 1997). Recent studies have demonstrated that the activities and transcriptional expression of Zn regulatory enzymes, such as metallothionein (MT), are sensitive responsive indicators to evaluate the available Zn from different Zn sources in animals (Cousins et al., 2003; Huang et al., 2009; Suo et al., 2015).
The chemical form of supplemental Zn source affects the Zn bioavailability. Zn is added to broiler diets usually as inorganic feed-grade ZnSO4, Zn oxide, Zn-amino acid chelate, or Zn-proteinate complex. Organic Zn sources have been used increasingly due to their potentially high Zn bioavailability, although there are some conflicting findings (Kidd et al., 1996; Huang et al., 2009; Salim et al., 2010; Star et al., 2012). It is well established that an inorganic metal salt has a different absorption level than a metal-amino acid chelate (Ashmead, 2012). Even among metal-amino acid chelates, absorption rates vary according to the ligand, stability constants, molecular weights, etc. (Ashmead, 2012). Huang et al. (2009) found that the Zn bioavailability from Zn-amino acid or Zn-proteinate complex was closely related to their chelation strength, the value representing stability constant, in starter broilers. However, little research has been carried out to compare the Zn bioavailability from different Zn-amino acid chelates in broilers under the same conditions. The objective of this study was to compare the Zn bioavailability from Zn-glycine (Zn-Gly) and Zn-methionine chelate (Zn-Met) as compared with ZnSO4 used as a standard in the corn-soybean meal basal diet for the starter broiler chickens.
Materials and methods
Animals and diets
A total of 1,200 one-day-old male broilers (Cobb 500) were randomly allotted to one of 10 treatments with eight replicate cages of 15 chicks each. Dietary treatments included the corn-soybean meal basal diet (control) and the basal diet supplemented with 40, 80, or 120 mg Zn/kg from ZnSO4, Zn-Gly, or Zn-Met. The basal diet (Table 1) was formulated to meet or exceed the requirements of starter broilers (day 1–14) as recommended by the Feeding Standard of Chicken (Ministry of Agriculture of the People’s Republic of China, 2004), except for Zn. The basal diet contained 26.46 mg Zn/kg by analysis. Supplemental ZnSO4 was provided as ZnSO4•7H2O (reagent grade, purity 99.95%), purchased from Chendu Xilong Biochemical Company (Chengdu, China). The Zn-Gly and Zn-Met chelates contained 19.00% and 23.75% Zn, respectively, purchased from Sichuan Chelota Group (Mianyang, China). In these chelates, the glycine or methionine molecule is bound with Zn2+ by oxygen and nitrogen atoms (Chang et al., 2022; Wang et al., 2022). In the basal diet, calcium carbonate and dicalcium phosphate (Chendu Xilong Biochemical Company, Chengdu, China) were reagent-grade, and the purity of these compounds was more than 99.00%. Each Zn source was premixed with maize starch to the same weight and then was added to the respective diet based on its analyzed Zn concentration. Variable amounts of glycine and methionine were added to the respective experimental diets according to the amounts of glycine and methionine from supplemental Zn-glycine and Zn-methionine chelates so as to balance the glycine and methionine in each experimental diet. Dietary Zn concentrations are presented in Table 2. Drinking water contained 30.2 μg Zn/L.
The broiler chickens were fed different treatment diets for 14 days. All diets were offered in a mash form. All birds were housed in electrically heated, thermostatically controlled cages (100 × 100 × 50 cm) with a 16-h light/8-h dark photocycle. The chicks were allowed ad libitum access to the experimental diets and water. The ambient temperature was gradually decreased by 0.5°C per day from 32°C on day 1 to 25°C on day 14, and the relative humidity of the house was kept at 60%.
Growth performance
On day 1, the body weight (BW) of birds was measured, and on days 7 and 14, the BW and feed consumption were measured for each replicate cage after 8-h fasting. Mortality was recorded during the experimental period. The body weight gain (BWG), average feed intake (AFI), and the ratio of feed consumption and BWG (FCR) were calculated during days 1–7, 8–14, and 1–14.
Sample collection
On days 7 and 14, two chicks were selected from each replicate cage according to average body weight and were sacrificed by cervical dislocation after 12-h fasting. The pancreas, left lobe of the liver, and the left tibia were excised and frozen at −20°C for Zn or MT protein analysis. Another subsample of the pancreas was frozen in liquid nitrogen for MT mRNA analysis.
Zn concentrations in different tissues
The concentrations of Zn in the diets and tissues were determined by flame atomic absorption spectrometry (ContrAA700, Jena Company, Germany) as described by Zhao et al. (2019). Approximately 1.0 g of the pancreas or liver or 0.5 g of diet samples was digested with 10 ml of the mixed acid of HNO3 and HF (4:1, v:v) using a microwave digestion system (MARS6, CEM Corporation, Matthews, NC, United States). The digestion procedure was as follows: the temperature was increased to 120°C within 5 min and maintained for 10 min and then increased up to 180°C within the next 5 min and maintained for 90 min. After digestion, the digestion tubes were taken out, and the mixture acid was evaporated to 0.5 ml at 160°C using a heater (Model BHW-09Y, Botonyc, Beijing, China). After cooling, the digested solution was diluted to 50 ml volume with 0.5% nitric acid for Zn analysis. The frozen tibia samples were boiled for 10 min in deionized water, and all soft tissues were removed. The tibia samples were then dried at 105°C and ashed at 550°C for 8 h in a muffle furnace. The tibia ash was dissolved in 0.5% nitric acid for Zn analysis. For quality control, a standard reference of wheat powder purchased from National Institute of Standards and Technology (Beijing, China) was included in each batch of analysis to verify the Zn analysis validation.
Pancreas MT concentration
The concentration of MT in pancreas was determined using a chicken MT ELISA kit (Nanjing Jiancheng Bioengineering Institute, Nanjing, China) following the manufacturer’s instructions. Briefly, approximately 0.2 g of the pancreas was transferred to a 5-ml tube containing 2 ml of lysis buffer (50 mM Tris-Cl, 1 mM EDTA, and 1 mM β-mercaptoethanol, pH 7.5). The pancreas samples were homogenized on ice with a homogenizer (Bio-Gen PRO200 Homogenizer, Pro Scientific Co., Oxford, CT, United States) for 30 s at 300 × rpm. This was repeated in 30 s pulses alternating with 30 s on ice twice. The lysates were then centrifuged at 13,000 × g at 4°C for 30 min, and the supernatant was collected as sample solution for determining the concentrations of MT and total protein. For the MT analysis, 50 μl of the MT standard solution or sample solution was incubated for 2 h at room temperature in each well (containing the immobilized murine monoclonal antibody against chicken MT). A 50 μl volume of the antibody–enzyme (horseradish peroxidase) conjugate solution was then incubated in the wells at 37°C for 30 min. Between the aforementioned steps, the plate was emptied and washed three times with a washing buffer. After drying, 50 μl volume of substrate mixture tetramethyl benzidine was pipetted into each well. The enzymatic reaction took place for 10 min at 37°C and was then stopped by adding 50 μl of a 2 M H2SO4 solution. Using a blank well as a reference (zero optical density), the optical density of each well under 450 nm wavelength was measured. Protein concentration was quantified with Bradford reagent (Sigma-Aldrich, United States) using an Infinite M200Pro multimode plate reader and Magellan software (Tecan, United States).
Pancreas MT mRNA abundance
Total RNA was extracted from frozen pancreas samples using RNAiso plus reagent (Takara, Dalian, China). The quality of extracted RNA was detected by 1% agarose gel electrophoresis. The purity and concentration of RNA were determined using a nucleic acid protein analyzer (NanoDrop 2000, Thermo Scientific, United States). First-strand cDNA was synthesized from 200 ng of total RNA with a PrimeScript TM RT reagent kit (Takara, Dalian, China). The transcription procedure was as follows: 37°C for 120 min and 85°C for 5 min. Real-time PCR was performed in duplicate in 20 μl system that contained 10 μl SYBR Green PCR mix (TaKaRa, China), 2 μl of diluted cDNA, 0.8 μl of 10 μM each primer, and 6.4 μl of PCR-grade water using a 7,900 Fluorescence quantitative PCR (Applied Biosystems, United States). The primer sequences were as follows: MT (forward) 5′-AAGGGCTGTGTCTGCAAGG-3′, (reverse) 5′-CTTCATCGGTATGGAAGGTAC-3’; and β-actin (forward) 5′- GAGAAATTGTGCGTGACATCA -3′, (reverse) 5′-CCTGAACCTCTCATTGCCA-3’. Real-time PCR reaction condition was in the following: 95°C for 30 s and 40 cycles of 95°C for 5 s plus 60°C for 34 s. The dissolution procedure was as follows: 95°C for 15 s, 60°C for1 min, and 95°C for 15 s. The β-actin was selected as the reference gene (Bai et al., 2020), and the MT mRNA expression was calculated using the 2−ΔΔCt method (Livak and Schmittgen, 2001).
Statistical analysis
Data were statistically analyzed using the general linear model procedure using SAS 9.2 software (SAS Institute Inc., Cary, NC, United States). To compare all Zn-supplemented treatments with the control, the data were analyzed using the one-way single degree of freedom contrast method (Suo et al., 2015). The data of all treatments except for the control were analyzed by two-way ANOVA, and the statistical model included Zn source, added Zn level, and their interaction. Linear responses of Zn concentrations in the tibia, liver, and pancreas, pancreas MT content, and pancreas MT mRNA abundance to the graded supplemental Zn level were evaluated using the orthogonal polynomial coding in the CONTRAST statement. Relative bioavailability value (RBV) of Zn was determined using Zn sulfate as a standard by means of multiple linear regression and slope ratio methodology (Suo et al., 2015). The liver Zn concentration was not significantly correlated to supplemental Zn intake for broilers on days 7 and 14, and it was consequently not used to calculate RBV estimate. Standard errors were calculated for each regression coefficient as described by Littell et al. (1997). The replicate cage was served as the experimental unit. Significant differences among means were tested by the least square difference method. For all statistical analyses, significance was declared at p < 0.05, and tendency was declared at 0.05 < p < 0.10.
Results
Growth performance
The effects of supplemental Zn source and Zn level on the growth performance of broilers are shown in Table 3. Overall, Zn supplementation decreased (p < 0.05) the AFI and FCR but did not significantly affect the BWG of broilers during days 1–7 compared with the control (p > 0.1). In all Zn-added treatments, Zn-Met and Zn-Gly addition significantly increased (p < 0.05) the BWG compared with ZnSO4 during days 1–7, whereas no significant difference was observed between the Zn-Met and Zn-Gly treatments. Meanwhile, they also tended to increase the BW on day 7 compared with the ZnSO4 treatment (p = 0.05). Irrespective of added Zn source, addition of 80 mg Zn/kg tended to increase the AFI compared with 40 mg Zn/kg treatment (p = 0.05). For ZnSO4 treatments, addition of 80 or 120 mg Zn/kg increased FCR compared with 40 mg Zn/kg addition group (p < 0.04). The addition of 80 mg Zn/kg also increased FCR compared with the 40 mg Zn/kg addition group for the Zn-Met treatment (p = 0.04), while addition of 120 mg Zn/kg decreased it compared with the 80 mg Zn/kg addition group (p = 0.03). However, added Zn level did not significantly affect FCR for the Zn-Gly treatment during days 1–7 (p > 0.4), and these ratios were lower than that in 80 or 120 mg Zn/kg as the ZnSO4 addition group (p < 0.05).
Dietary Zn addition, as a whole, had no significant effects on the BW of broilers on day 14, and the AFI, BWG, and FCR (p > 0.15) compared with the control during days 8–14. In all Zn-added treatments, the BW on day 14 and BWG during days 8–14 were higher in the Zn-Gly treatment than those in the Zn-Met treatment (p < 0.05), which were higher than those in the ZnSO4 treatment (p < 0.05). The Zn-Met and Zn-Gly treatments also had higher AFI during days 8–14 compared with the ZnSO4 treatment (p < 0.05). However, added Zn level and the interaction between added Zn source and Zn level had no significant effect on the AFI, BWG, and FCR during days 8–14 (p > 0.15).
In the overall period (days 1–14), dietary Zn addition, as a whole, had no significant effect on the AFI, BWG, and FCR (p > 0.10) compared with the control. In all Zn-added treatments, addition of Zn-Met or Zn-Gly increased (p < 0.05) the AFI and BWG compared with the ZnSO4 treatment, and the BWG was higher in the Zn-Gly treatment than in the Zn-Met treatment (p < 0.05) during days 1–14. No significant difference in FCR was observed among three added-Zn sources for 40 or120 mg Zn/kg treatments (p > 0.10), whereas this ratio was higher in the Zn-Met group than in the Zn-Gly or ZnSO4 group when 80 mg Zn/kg was given (p < 0.05).
Zn concentrations in different tissues
The effects of supplemental Zn source and Zn level on the Zn content in different tissues of broilers are shown in Table 4. Overall, Zn supplementation significantly increased (p < 0.05) Zn concentrations in the liver, tibia, and pancreas of broilers on days 7 and 14 compared with the control. In all Zn-added groups, Zn-Met addition increased the tibia Zn level compared with the Zn-Gly or ZnSO4 treatment on day 7 (p < 0.05), whereas Zn sources did not affect it on day 14. Supplemental Zn sources also did not affect the Zn concentrations in the liver and pancreas on days 7 and 14 (p > 0.10). However, supplemental Zn level significantly affected Zn concentration in different tissues of broilers on days 7 and 14, except for pancreas Zn on day 7. Addition of 120 mg Zn/kg increased the liver Zn concentration compared with 40 or 80 mg/kg Zn addition treatments (p < 0.05). Likewise, the tibia Zn concentration increased significantly (p < 0.05) with increasing supplemental Zn level from 40 to 120 mg/kg Zn on day 7. On day 14, the concentrations of Zn in the liver, tibia, and pancreas increased linearly with increasing supplemental Zn level (p < 0.001). However, dietary supplemental Zn source and Zn level did not have interactive effect on the Zn content in the liver, tibia, and pancreas of broilers on days 7 and 14.
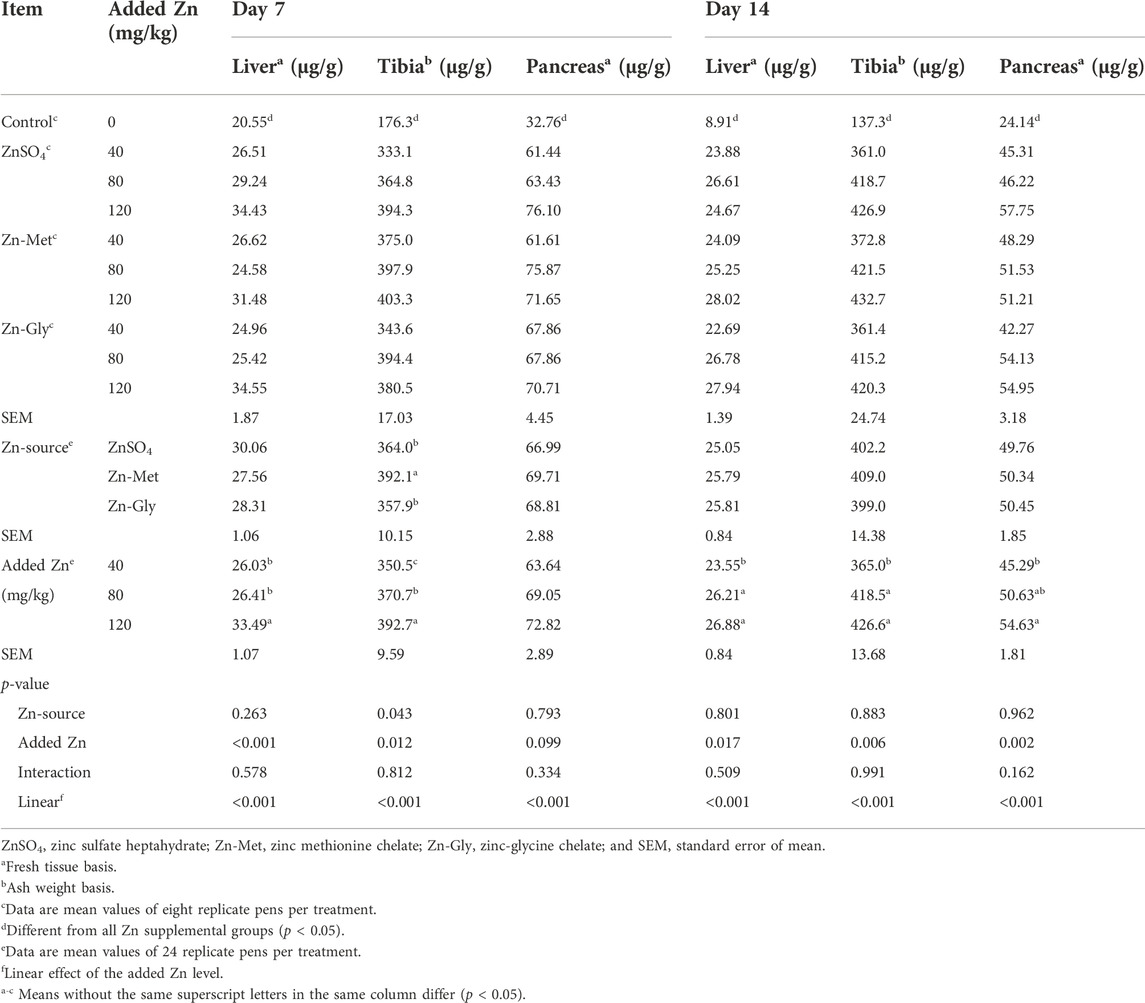
TABLE 4. Effects of supplemental zinc source and zinc level on zinc concentration in different tissues of broilers.
Pancreas MT expression
The effects of supplemental Zn source and Zn level on MT concentration and MT mRNA abundance in the pancreas of broilers are shown in Table 5. Overall, Zn supplementation significantly increased (p < 0.05) the MT protein concentration and MT mRNA abundance in the pancreas of broilers on days 7 and 14. In all Zn-added groups, the pancreas MT protein level was higher in the Zn-Met treatment than that in the ZnSO4 treatment (p < 0.05) on day 7, and it was intermediate for the Zn-Gly treatment. Added Zn source did not significantly affect the MT mRNA abundance in the pancreas of broilers on day 7 (p > 0.05). The pancreas MT concentration and MT mRNA abundance increased linearly with increasing supplemental Zn level (p < 0.05) by tendency analysis, whereas the difference in pancreas MT mRNA abundance between 40 and 80 mg/kg Zn addition treatments did not attain the significant level (p > 0.05).
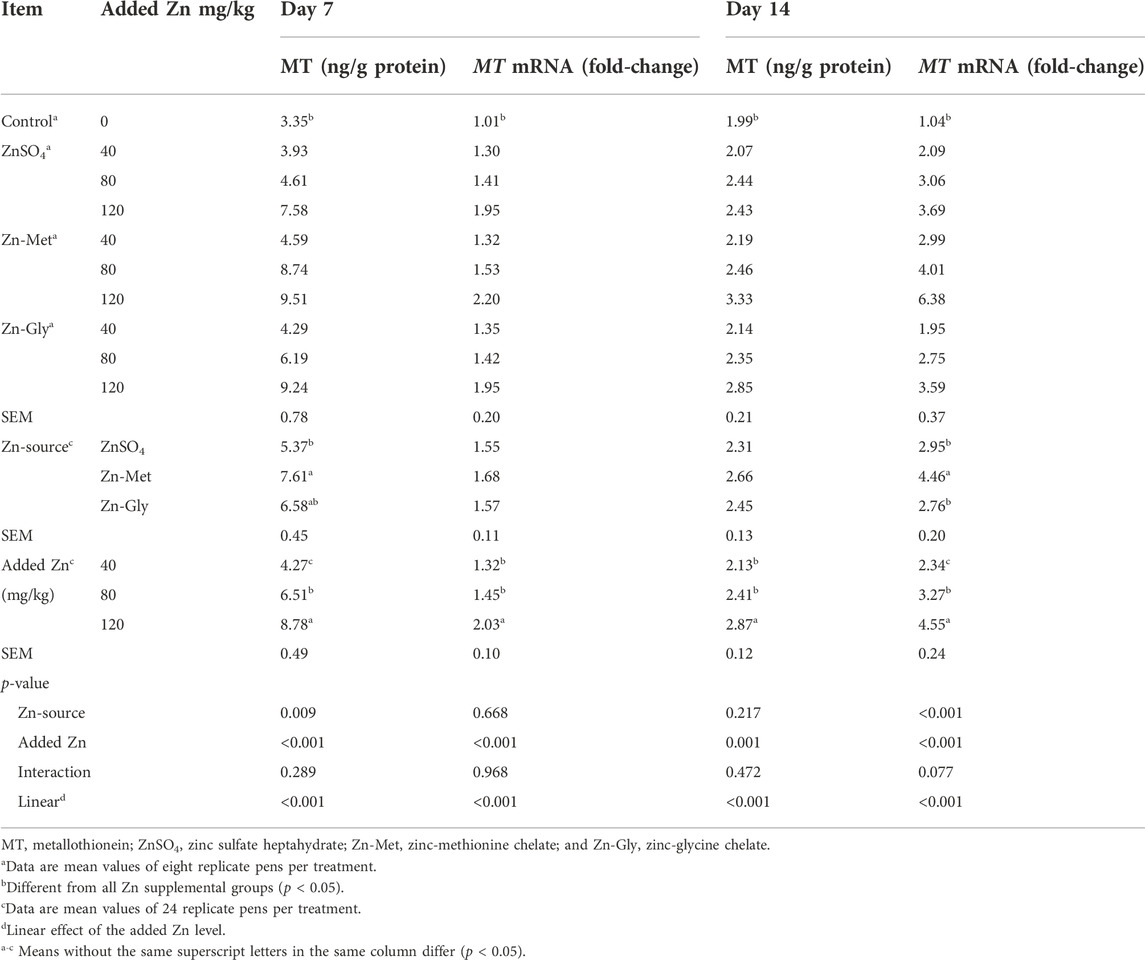
TABLE 5. Effects of supplemental Zn source and zinc level on the concentration and mRNA abundance of metallothionine in the pancreas of broilers.
On day 14, the Zn-Met treatment increased pancreas MT mRNA abundance compared with the Zn-Gly or ZnSO4 treatment (p < 0.001), whereas added Zn sources did not significantly affect the pancreas MT concentration (p > 0.10). The pancreas MT concentration and MT mRNA abundance increased linearly with increasing supplemental Zn level (p < 0.05), while no significant difference in pancreas MT concentration between 40 and 80 mg Zn/kg addition treatments on day 14 was observed.
Relative bioavailability of Zn from Zn-Gly or Zn-Met chelate
Different biological indices were regressed on the supplemental Zn intake and significant multiple linear regression relationships were observed (p < 0.001) for the tibia and pancreas Zn concentrations, pancreas MT content, and pancreas MT mRNA abundance in broilers at 7 and 14 days of age (Table 6). Thus, the RBV was calculated using ZnSO4 as a standard (100%) by means of slope ratio methodology and the results are shown in Table 7. On day 7, no significant differences in the RBV were observed among three supplemental Zn sources, assessed by tibia Zn, pancreas Zn, and pancreas MT mRNA (p > 0.40), whereas it was in the following order: Zn-Met > Zn-Gly > ZnSO4 (p < 0.05), assessed by the pancreas MT content. The bioavailable Zn from Zn-Met or Zn-Gly was 1.73 or 1.44 times that from ZnSO4, respectively, and the bioavailable Zn from Zn-Met was 1.20 times that from Zn-Gly. On day 14, no significant differences were also observed in the RBV of Zn among three supplemental Zn sources based on tibia Zn and pancreas Zn (p > 0.46). However, the RBV of Zn was in the order Zn-Met > Zn-Gly > ZnSO4 (p < 0.05), assessed by the pancreas MT content. The bioavailable Zn from Zn-Met or Zn-Gly was 1.75 or 1.39 times that from ZnSO4, respectively, and the bioavailable Zn from Zn-Met was 1.25 times that from Zn-Gly. The RBV of Zn were significantly higher for Zn-Met than that for Zn-Gly or ZnSO4 (p < 0.05) based on the pancreas MT mRNA expression, and there was no significant difference between Zn-Gly and Zn-Met (p > 0.10).
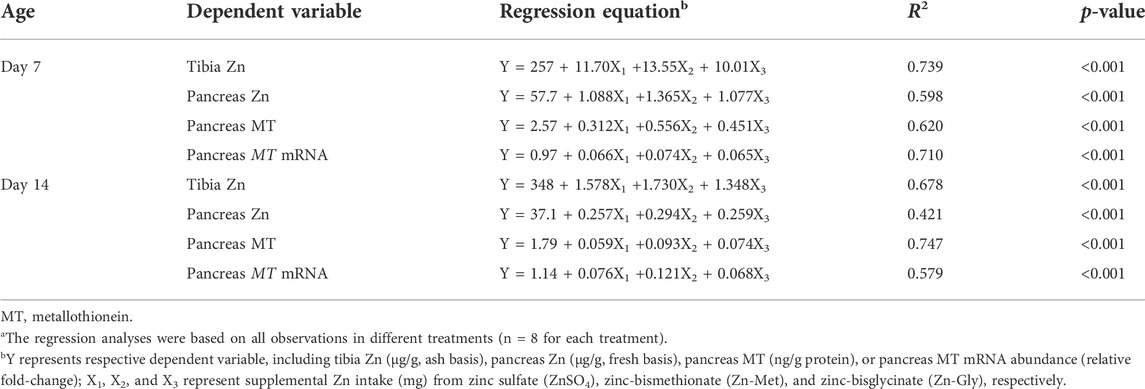
TABLE 6. Multiple linear equation of different Zn sourcesa.
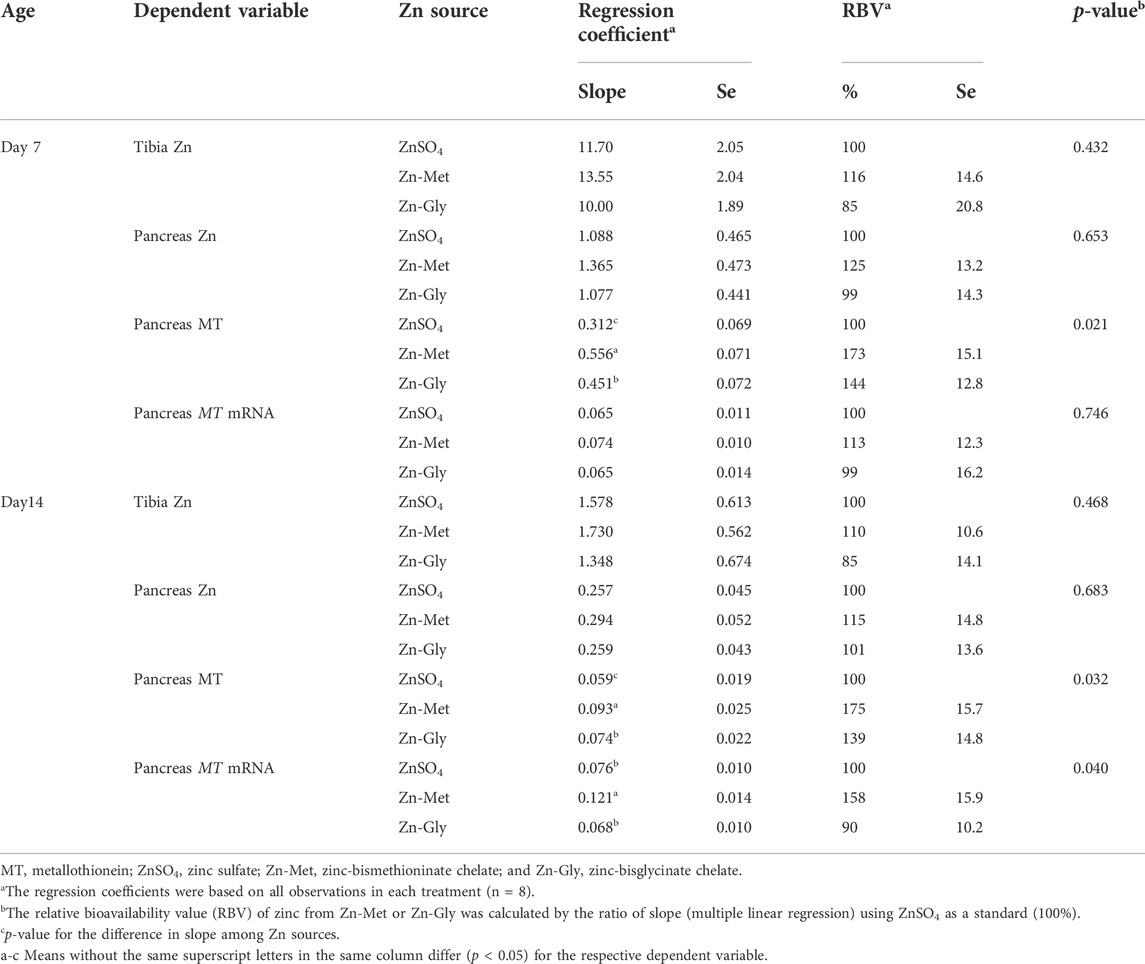
TABLE 7. Relative bioavailability values (RBVs) of zinc based on the multiple regression of different biological indices on supplemental zinc intake.
Discussion
The term “bioavailability” is defined as the degree to which an ingested nutrient in a particular source is absorbed in a form that can be utilized in metabolism by animal (Ammerman et al., 1995). The growth performance can be used as a response biomarker to assess the RBV of Zn for broilers when dietary graded Zn levels are below their requirement in the purified diet (Wedekind and Baker, 1990; Wedekind et al., 1992). However, in this study, all Zn-supplemented treatments did not increase the BWG of starter broilers during days 1–14 compared with the control, and no significant differences occurred in AFI and BWG among the Zn-supplemented treatments. These results indicated that the addition of 40 mg/kg Zn in the corn-soybean meal diet is sufficient for the growth of starter broilers (Huang et al., 2007; Bao et al., 2009). No negative effect of the control diet on the BWG compared with the Zn-supplemented treatments presented herein might be due to the increase of feed intake during days 1–7. Our result of no increase in the growth performance with increasing dietary Zn level proved that it is not a suitable biomarker to assess the RBV of Zn for the broilers when dietary Zn level is higher than their requirement (Wedekind et al., 1992) because the growth performance of chicks can be affected by many dietary factors other than Zn (Moran, 2017). In addition, our study found that Zn-Gly and Zn-Met increased the BWG and AFI compared with ZnSO4 during days 8–14. This finding might be due to the important role of Zn in the appetite regulation for broilers (Dewar et al., 1982). The increase of the BWG in the Zn-Gly treatment compared with the Zn-Met treatment might be attributed to the dissociated glycine from Zn-Gly chelate at intestinal mucosal barrier (Ammerman et al., 1995), which could improve mucosal absorption function in the small intestine of poultry (Ospina-Rojas et al., 2013; Moran, 2017).
The Zn concentrations in different tissues, such as the tibia, liver, pancreas, kidney, and intestinal mucosa, have been used as the biological criterion for RBV assays with the poultry (Sandoval et al., 1997; Cao et al., 2000; Huang et al., 2009). In the present study, we only chose the Zn concentration in the tibia, liver, and pancreas of broilers to determine for the RBV assay, because the mucosal Zn accumulation only reflects the status of Zn absorption and homeostasis (Cao et al., 2000) and uptake of Zn by kidney is affected by the feed intake for broilers (Sandoval et al., 1997; Sandovel et al., 1999). Our results demonstrated that the tibia and pancreas accumulated more Zn than the liver, and the pancreas Zn level was higher than that of tibia Zn if on the wet-tissue basis, which were consistent with the previous findings (Sandoval et al., 1997; Sandovel et al., 1998). Moreover, we did not find the differences in pancreas Zn and liver Zn on day 7 and Zn concentrations in the tibia, pancreas, and liver on day 14 among Zn-Met, Zn-Gly, and ZnSO4 treatments. Our study also found that the RBV of Zn as Zn-Met was comparable with that as Zn-Gly or ZnSO4 evaluated by tibia and pancreas Zn levels. In agreement, Suo et al. (2015) reported that the RBV of Zn from Zn-Met was just as bioavailable as that from ZnSO4 based on multiple linear regression ratio of pancreas or tibia Zn on dietary Zn intake. Similarly, the estimated RBV of Zn from a certain Zn-amino acid chelate did not differ that from ZnSO4 based on tibia Zn (Cao et al., 2000; Huang et al., 2009). However, the RBV of Zn from Zn-Met was higher in the purified (crystalline amino acid) or semipurified (soy isolate) diets compared to that from ZnSO4 in broilers given the Zn level was far below the requirement (Wedekind et al., 1992). The discrepancies in these findings might be due to the differences in the composition of the basal diet and supplemental Zn level.
MT plays a crucial role in the cellular Zn homeostasis because it exhibits high affinity binding to Zn ion in cytoplasm (Kimura and Kambe, 2016). Zn intake has been shown to induce tissue MT synthesis (Kimura and Itoh, 2008). The present results found that the concentration and mRNA abundance of pancreas MT increased linearly as dietary supplemental Zn level increased. In agreement, pancreas MT protein or MT mRNA expression increased linearly in chicks with increasing dietary Zn level up to subtherapeutic level (1,000 or 1,200 mg/kg) for chicks (Sandovel et al., 1998). These results confirmed that pancreas MT expression can be used as a biomarker to assess the RBV of Zn for broiler chickens feeding excess dietary Zn (Sandoval et al., 1997; Sandovel et al., 1998). In the present study, the results of multiple regression slope ratio of the pancreas MT concentration on dietary supplemental Zn intake showed the RBV of Zn was in the following order: Zn-Met > Zn-Gly > ZnSO4. However, Zn-Met and other unknown Zn-amino acid complexes had bioavailability values similar to that of ZnSO4 for chicks with dietary supplementation of 200–600 mg/kg Zn (Cao et al., 2000). Cao et al. (2002) reported that the RBV of Zn from Zn-Met was 0.78–0.91 of Zn-acetate standard (1.00) for starter broilers given 30–90 mg Zn/kg diet, based on the intestinal mucosal MT. Huang et al. (2009) also found that the unknown Zn-amino acid chelate had a comparable RBV of Zn relative to ZnSO4 assessed by pancreas MT. The differences in the standard Zn source, the selected specific tissue for the MT determination, and the ligands of Zn-amino acid chelate might explain the discrepancies in these studies. In addition, the responses of pancreas MT to dietary supplemental Zn intake presented herein were inconsistent with the aforementioned findings of the RBV based on tissue Zn accumulation, which might be due to the fact that the response of MT is more sensitive to the Zn uptake than tissue Zn accumulation (Baltaci et al., 2018; Zhao et al., 2019). These results indicated the pancreas MT concentration was more suitable to evaluate the RBV of Zn from different sources for starter broilers than the tissue Zn accumulation.
Several studies found that tissue MT mRNA could be used as a response criterion to evaluate the RBV of Zn from different Zn sources in chickens (Cao et al., 2002; Huang et al., 2009). In the present study, we found that the RBV of Zn from Zn-Met did not differ from that as Zn-Gly or ZnSO4 based on pancreas MT mRNA abundance on day 7, and it was lower assessed by pancreas MT mRNA abundance than pancreas MT concentration. The results were not in line with the previous finding that the MT mRNA abundance in pancreas was more sensitive in reflecting differences in bioavailability among organic Zn sources than the MT concentration in pancreas (Huang et al., 2009). There were two potential reasons to explain these discrepancies. First, the accumulation of MT mRNA may be partly regulated by MT mRNA stability, which relates to the concentrations of metals (Zn and Cd) and inflammation factors (De et al., 1991; Cousins and Lee-Ambrose, 1992). Second, the increase of MT protein can cause the decrease of the free Zn ion, resulting in the downregulation of MT transcription (Kimura and Itoh, 2008). Additionally, we found that the RBV of Zn from Zn-Met or Zn-Gly compared with ZnSO4 used as a standard on day 7 was similar to that on day 14, based on the pancreas MT concentration. However, based on the pancreas MT mRNA abundance, the RBV of Zn from Zn-Met or Zn-Gly on day 7 differed from that on day 14. These results indicated that the pancreas MT concentration was more stable to evaluate the RBV of Zn as compared with the pancreas MT mRNA abundance. Future work should focus on the potential mechanisms underlying the inconsistent expression of MT mRNA and protein in the pancreas of broilers for the Zn bioavailability assay.
Conclusion
The Zn concentrations in the tibia, pancreas, and liver, pancreas MT content, and pancreas MT mRNA abundance of starter broilers (d 1–14) increased linearly with increasing supplemental Zn from 40 to 120 mg/kg for the corn-soybean meal basal diet. Zn-Met had similar bioavailable Zn to Zn-Gly, based on pancreas or tibia Zn, whereas it had higher levels of bioavailable Zn than Zn-Gly, using the pancreas MT concentration as a response biomarker, for the starter broilers.
Data availability statement
The original contributions presented in the study are included in the article/supplementary material; further inquiries can be directed to the corresponding author.
Ethics statement
The animal study was reviewed and approved by Institutional Animal Care and Use Committee of Sichuan Agricultural University.
Author contributions
XC and CH contributed to investigation, methodology, writing—original draft preparation, and editing. KZ, JW, and XD contributed to software and investigation. QZ, HP, JB, LL, and YX contributed to investigation. SB contributed to project administration, funding acquisition, and writing—review and editing. All authors have read and approved the submitted version of the manuscript.
Funding
This work was supported by National Key Research and Development Program of China (Beijing, China; grant number 2021YFD1300203) and Science and Technology Support Project of Ya’an City (Ya’an, China; grant number 2022YA0003-02).
Conflict of interest
The authors declare that the research was conducted in the absence of any commercial or financial relationships that could be construed as a potential conflict of interest.
Publisher’s note
All claims expressed in this article are solely those of the authors and do not necessarily represent those of their affiliated organizations, or those of the publisher, the editors, and the reviewers. Any product that may be evaluated in this article, or claim that may be made by its manufacturer, is not guaranteed or endorsed by the publisher.
References
Ammerman C. B., Baker D. H., Lewis A. J. (1995). Bioavailability of nutrients for animals: Amino acids, minerals, and vitamins. San Diego: Academic Press.
Ashmead H. D. (2012). Amino acid chelation in human and animal nutrition. Boca Raton: CRC Press, 81–152.
Bai S., Peng J., Zhang K., Ding X., Wang J., Zeng Q., et al. (2020). Effects of dietary iron on manganese utilization in broilers fed with corn-soybean meal diet. Biol. Trace Elem. Res. 194, 514–524. doi:10.1007/s12011-019-01780-w
Baltaci A. K., Yuce K., Mogulkoc R. (2018). Zinc metabolism and metallothioneins. Biol. Trace Elem. Res. 183, 22–31. doi:10.1007/s12011-017-1119-7
Bao Y. M., Choct M., Iji P. A., Bruerton K. (2009). Optimal dietary inclusion of organically complexed zinc for broiler chickens. Br. Poult. Sci. 50, 95–102. doi:10.1080/00071660802590377
Batal A. B., Parr T. M., Baker D. H. (2001). Zinc bioavailability in tetrabasic zinc chloride and the dietary zinc requirement of young chicks fed a soy concentrate diet. Poult. Sci. 80, 87–90. doi:10.1093/ps/80.1.87
Cao J., Henry P. R., Davis S. R., Cousins R. J., Littell R. C., Miles R. D., et al. (2002). Relative bioavailability of organic zinc sources based on tissue zinc and metallothionein in chicks fed conventional dietary zinc concentrations. Anim. Feed Sci. Technol. 101, 161–170. doi:10.1016/s0377-8401(02)00051-2
Cao J., Henry P. R., Guo R., Holwerda R. A., Toth J. P., Littell R. C., et al. (2000). Chemical characteristics and relative bioavailability of supplemental organic zinc sources for poultry and ruminants. J. Anim. Sci. 78, 2039–2054. doi:10.2527/2000.7882039x
Chang Y., Tang H., Zhang Z., Yang T., Wu B., Zhao H., et al. (2022). Zinc methionine improves the growth performance of meat ducks by enhancing the antioxidant capacity and intestinal barrier function. Front. Vet. Sci. 9, 774160. doi:10.3389/fvets.2022.774160
Cousins R. J., Blanchard R. K., Popp M. P., Liu L., Cao J., Moore J. B., et al. (2003). A global view of the selectivity of zinc deprivation and excess on genes expressed in human THP-1 mononuclear cells. Proc. Natl. Acad. Sci. U. S. A. 100, 6952–6957. doi:10.1073/pnas.0732111100
Cousins R. J., Lee-Ambrose L. M. (1992). Nuclear zinc uptake and interactions and metallothionein gene expression are influenced by dietary zinc in rats. J. Nutr. 122, 56–64. doi:10.1093/jn/122.1.56
De S. K., Enders G. C., Andrews G. K. (1991). Metallothionein mRNA stability in chicken and mouse cells. Biochim. Biophys. Acta 1090 (2), 223–229. doi:10.1016/0167-4781(91)90105-u
Dewar W. A., Sibbald I., Wight P. (1982). The contribution of anorexia to reduced growth in zinc-deficient chickens. Br. Poult. Sci. 23, 129–134. doi:10.1080/00071688208447939
Huang Y. L., Lu L., Li S. F., Luo X. G., Liu B. (2009). Relative bioavailabilities of organic zinc sources with different chelation strengths for broilers fed a conventional corn-soybean meal diet. J. Anim. Sci. 87, 2038–2046. doi:10.2527/jas.2008-1212
Huang Y. L., Lu L., Luo X. G., Liu B. (2007). An optimal dietary zinc level of broiler chicks fed a corn-soybean meal diet. Poult. Sci. 86, 2582–2589. doi:10.3382/ps.2007-00088
Kidd M. T., Ferket P. R., Qureshi M. A. (1996). Zinc metabolism with special reference to its role in immunity. World's. Poult. Sci. J. 52, 309–324. doi:10.1079/WPS19960022
Kimura T., Itoh N. (2008). Function of metallothionein in gene expression and signal transduction: Newly found protective role of metallothionein. J. Health Sci. 54, 251–260. doi:10.1248/jhs.54.251
Kimura T., Kambe T. (2016). The functions of metallothionein and ZIP and ZnT transporters: An overview and perspective. Int. J. Mol. Sci. 17, 336. doi:10.3390/ijms17030336
Littell R. C., Henry P. R., Lewis A. J., Ammerman C. B. (1997). Estimation of relative bioavailability of nutrients using SAS procedures. J. Anim. Sci. 75, 2672–2683. doi:10.2527/1997.75102672x
Livak K. J., Schmittgen T. D. (2001). Analysis of relative gene expression data using real-time quantitative PCR and the 2(-Delta Delta C(T)) method. Methods 25, 402–408. doi:10.1006/meth.2001.1262
McCall K. A., Huang C., Fierke C. A. (2000). Function and mechanism of zinc metalloenzymes. J. Nutr. 130, 1437S–1446S. doi:10.1093/jn/130.5.1437s
Ministry of Agricultural of the People’s Republic of China (2004). Feeding standard of chicken. Beijing: Chinese Agricultural Press.
Moran E. T. (2017). Nutrients central to maintaining intestinal absorptive efficiency and barrier integrity with fowl. Poult. Sci. 96, 1348–1363. doi:10.3382/ps/pew337
National Research Council (1994). Nutrient requirements of poultry. 9th ed. Washington: National Academy Press.
Ospina-Rojas I. C., Murakami A. E., Oliveira C. A. L., Guerra A. F. Q. G. (2013). Supplemental glycine and threonine effects on performance, intestinal mucosa development, and nutrient utilization of growing broiler chickens. Poult. Sci. 92, 2724–2731. doi:10.3382/ps.2013-03171
Salim H. M., Lee H. R., Jo C., Lee S. K., Lee B. D. (2010). Effect of sources and levels of zinc on the tissue mineral concentration and carcass quality of broilers. Avian Biol. Res. 3, 23–29. doi:10.3184/175815510X12636595095213
Sandoval M., Henry P. R., Ammerman C. B., Miles R. D., Littell R. C. (1997). Relative bioavailability of supplemental inorganic zinc sources for chicks. J. Anim. Sci. 75, 3195–3205. doi:10.2527/1997.75123195x
Sandovel M., Henry P. R., Littell R. C., Miles R. D., Ammerman C. B. (1999). Effect of dietary zinc source and method of oral administration on performance and tissue trace mineral concentration ofbroiler chicks. J. Anim. Sci. 77, 1788–1799. doi:10.2527/1999.7771788x
Sandovel M., Henry P. R., Luo X. G., Littell R. C., Miles R. D., Ammerman C. B. (1998). Performance and tissue zinc and metallothionein accumulation in chicks fed a high dietary level of zinc. Poult. Sci. 77, 1354–1363. doi:10.1093/ps/77.9.1354
Shao Y. X., Zhang L. Y., Ma X. L., Wang L. Z., Liu D. Y., Lu L., et al. (2019). A survey on distribution of zinc contents in feedstuffs for livestock and poultry in China. Sci. Agric. Sin. 52, 2002–2010. doi:10.3864/j.issn.0578-1752.2019.11.015
Star L., van der Klis J. D., Rapp C., Ward T. L. (2012). Bioavailability of organic and inorganic zinc sources in male broilers. Poult. Sci. 91, 3115–3120. doi:10.3382/ps.2012-02314
Suo H., Lu L., Zhang L., Zhang X., Li H., Lu Y., et al. (2015). Relative bioavailability of zinc-methionine chelate for broilers fed a conventional corn-soybean meal diet. Biol. Trace Elem. Res. 165, 206–213. doi:10.1007/s12011-015-0252-4
Wang L., Wang Z., Luo P., Bai S., Chen Y., Chen W. (2022). Dietary zinc glycine supplementation improves tibia quality of meat ducks by modulating the intestinal barrier and bone resorption. Biol. Trace Elem. Res. doi:10.1007/s12011-022-03207-5
Wedekind K. J., Baker D. H. (1990). Zinc bioavailability in feed-grade sources of zinc. J. Anim. Sci. 68, 684–689. doi:10.2527/1990.683684x
Wedekind K. J., Hortin A. E., Baker D. H. (1992). Methodology for assessing zinc bioavailability: Efficacy estimates for zinc-methionine, zinc sulfate, and zinc oxide. J. Anim. Sci. 70, 178–187. doi:10.2527/1992.701178x
Keywords: organic zinc, bioavailability, pancreas metallothionein, zinc accumulation, chicken
Citation: Chen X, He C, Zhang K, Wang J, Ding X, Zeng Q, Peng H, Bai J, Lv L, Xuan Y and Bai S (2022) Comparison of zinc bioavailability in zinc-glycine and zinc-methionine chelates for broilers fed with a corn-soybean meal diet. Front. Physiol. 13:983954. doi: 10.3389/fphys.2022.983954
Received: 01 July 2022; Accepted: 02 November 2022;
Published: 17 November 2022.
Edited by:
Federico Sirri, University of Bologna, ItalyReviewed by:
Monika Proszkowiec-Weglarz, Agricultural Research Service (USDA), United StatesGiorgio Brugaletta, University of Bologna, Italy
Copyright © 2022 Chen, He, Zhang, Wang, Ding, Zeng, Peng, Bai, Lv, Xuan and Bai. This is an open-access article distributed under the terms of the Creative Commons Attribution License (CC BY). The use, distribution or reproduction in other forums is permitted, provided the original author(s) and the copyright owner(s) are credited and that the original publication in this journal is cited, in accordance with accepted academic practice. No use, distribution or reproduction is permitted which does not comply with these terms.
*Correspondence: Shiping Bai, c2hpcGluZ2JhaUBzaWNhdS5lZHUuY24=
†These authors have contributed equally to this work