- 1Olympic Training Center of Berlin, Berlin, Germany
- 2Max-Delbrück-Center for Molecular Medicine in the Helmholtz Association (MDC), Berlin, Germany
Elite athletes are regularly exposed to high and repetitive mechanical stresses and impacts, resulting in high injury rates. The consequences of injury can range from time lost from training and competition to chronic physical and psychological burden, with no guarantee that the athlete will return to preinjury levels of sport activity and performance. Prominent predictors include load management and previous injury, highlighting the importance of the postinjury period for effective return to sport (RTS). Currently, there is conflicting information on how to choose and assess the best reentry strategy. Treating RTS as a continuum, with controlled progression of training load and complexity, seems to provide benefits in this process. Furthermore, objectivity has been identified as a critical factor in improving the effectiveness of RTS. We propose that assessments derived from biomechanical measurements in functional settings can provide the objectivity needed for regular biofeedback cycles. These cycles should aim to identify weaknesses, customize the load, and inform on the status of RTS progress. This approach emphasizes individualization as the primary determinant of RTS and provides a solid foundation for achieving it.
1 Introduction
Elite athletes are exposed to repeated mechanical stress and high levels of impact on their bodies, both during training and competition. One of the highest injury rates in professional sport is encountered in elite athletics in particular. A recent survey of retired Olympians ranks athletics as one of the five sports with the highest lifetime prevalence of injury (Palmer et al., 2021). Similarly, a 1-year prospective study on an elite cohort in athletics that included all injuries revealed a staggering annual injury incidence of 68%, half of which (51%) were classified as severe (i.e., causing a period of absence from normal training for more than 3 weeks) (Jacobsson et al., 2013). Another study reported a similar incident rate (65%) (Edouard et al., 2022). Injuries in athletics often occur during competition. Between 2007 and 2012, one in every twelve registered athletes suffered an injury (categorized as resulting to time-loss) at international athletics championships (Alonso et al., 2012; Feddermann-Demont et al., 2014). Further, these injuries take a significant amount of time to heal, often sidelining athletes for weeks or even months and adversely affect the careers of elite athletes (Palmer et al., 2021).
Poor load management is often cited as the culprit of high injury rates, yet previous injury is also an important predictor (Jacobsson et al., 2013; Soligard et al., 2016). While the first received a lot of scientific attention in recent years (Kuipers and Keizer, 1988; Meeuwisse, 1994; Drew and Finch, 2016; Schwellnus et al., 2016; Soligard et al., 2016; Jones et al., 2017; Eckard et al., 2018), the latter is inherently more complicated to objectively assess and quantify, for instance due to different locations of injuries, variegated causality mechanisms and rehabilitation practices, and so on. After an injury, the athlete is eventually called to reenter the sporting activity and the decision-making process at this stage is extremely strenuous. In fact, in the 24 months after anterior cruciate ligament reconstruction, athletes had a greater risk to suffer a subsequent injury compared with young athletes without a history of anterior cruciate ligament injuries (Paterno et al., 2014). Consensus regarding the period after the typical medical treatment is scarce and generalized advice is typically convoluted (Ardern et al., 2016a). To make matters more complex, there is strong evidence for heterogeneity in the responsiveness to physical activity (Bouchard and Rankinen, 2001), which can be attributed to innate and acquired characteristics simultaneously (Ross et al., 2019). This interindividual variability in response to a prescribed post-injury training program necessitates a way to track these responses and raises questions about existing methods.
In addition to the difficulties in choosing and assessing the best reentry strategy, there is no guarantee that the athlete will return to preinjury levels of sport activity and performance. For instance, following anterior cruciate ligament reconstruction surgery, 81% of people returned to any sport, 65% returned to their preinjury level of sport and only 55% returned to competitive level sport after surgery (Ardern et al., 2014). Return to the preinjury level of sport following the same procedure is 83% among elite athletes (Lai et al., 2018). The consequences can also be chronic and/or affect mental health. One-third of a high sample survey on retired elite athletes reported pain and functional limitations that were present up to the time of survey due to those injuries, while depression was more prevalent in those who have sustained a significant injury (Palmer et al., 2021). Thus, it is essential that these decisions are based on the best available evidence and are made in the athlete’s best interests. Not only do these decisions affect an athlete’s ability to compete at the highest levels, but they also have significant implications for an athlete’s long-term health and wellbeing.
The purpose of this article is to 1) give an overview of the injury landscape in elite sports, 2) present the current state of the return to sport (RTS) complexity, 3) examine different frameworks of RTS, 4) offer perspectives on the increasingly important role of objective assessments in the field and v) consider future directions. The article skews towards athletics, but the considerations presented thereafter might be relevant to most elite sports.
2 Location and mechanisms of injury
In a survey sample of 3,357 retired Olympians of all sports, two-thirds of them reported significant injuries in their careers, with the knee, lumbar spine, and shoulder/clavicle being the most commonly injured areas (Palmer et al., 2021). The anatomical site of injury (e.g., bone stress injuries) can influence rehabilitation timelines and the risk of complication (Hoenig et al., 2023). A study that examined injuries during the 2016 summer Olympics found that the most commonly injured anatomical locations were, in order of more to less prevalent, the knee, thigh, ankle, face and lower leg (Soligard et al., 2017). In elite athletics, most of the time-loss injuries in competition (2007–2012) affected the lower extremity (87.1%), followed by the upper extremity (6.1%) and the trunk (5.9%). The thigh was the most commonly injured body part (34.5%), followed by the lower leg (14.6%), foot (9.8%), and knee (9.6%) (Feddermann-Demont et al., 2014).
In general, the causes behind injuries in elite athletes are multifactorial and often include a combination of intrinsic (e.g., muscle weakness or previous injuries) and extrinsic (e.g., equipment, field conditions, training volume, etc.) factors (Meeuwisse, 1994; Meeuwisse et al., 2007). However, adaptations occurring within the context of a specific sport alter the injury risk and affect the aetiology in a dynamic, recursive fashion (Meeuwisse et al., 2007). Overtraining and overuse have long been identified as a major drive for injury in elite athletes (Kuipers and Keizer, 1988; Meeuwisse, 1994; Schwellnus et al., 2016; Jones et al., 2017; Eckard et al., 2018), especially in disciplines of the summer Olympics (Palmer et al., 2021). In a prospective report from British elite athletics, the proportion of overuse injuries was approximately 70% of all injuries, while in a study of Swedish elite athletics, the percentage was around 96% (Jacobsson et al., 2013; Kelly et al., 2022). The discrepancy may be explained by differences in overuse and acute injury definitions in the two studies. Inconsistencies within the literature regarding the use of various terminologies such as training load, fatigue, injury and illness are limiting our ability to develop a complete model of association (Jones et al., 2017). Here, we use the term “load” as “the sport and non-sport burden as a stimulus that is applied to a human biological system” (Soligard et al., 2016). There is strong evidence for a load-injury relationship in tactical and elite athlete populations, with higher emphasis on the overall amount of load and less on its frequency of application (Eckard et al., 2018). Acute changes in the training program have also been shown to be a common mechanism of injury (Jones et al., 2017). Predicting injury events would be of immense value in elite sports, and several indicators have been examined. The most prominent include the self-reported perceived exertion questionnaire and the “acute to chronic load ratio”. With both methods it is possible to obtain good association and, thus, prediction of injury risk (Gabbett et al., 2016; Eckard et al., 2018).
Previous injuries are also an important predictor of follow-up injuries, highlighting the importance of the rehabilitation and RTS periods, since it has been shown that the variability of RTS duration and responsiveness to therapy can affect possible reinjury risk (Meeuwisse, 1994; Meeuwisse et al., 2007). During the first 9 months after anterior cruciate ligament reconstruction surgery, a later RTS has shown to be associated with a lower reinjury rate (Grindem et al., 2016). Remarkably, for every 1-month delay in return to sport, the reinjury rate was reduced by 51% (Grindem et al., 2016). However, after the 9-month mark, time was no longer a predictor of injury (Grindem et al., 2016) making it unusable for determining readiness to RTS. Further, we know that the individual’s psychology and the sociocultural substrate affect injury risk, response, and recovery and both should also be accounted for (Galambos et al., 2005; Wiese-Bjornstal, 2010). These aspects add to the overall complexity and make it difficult to build appropriate predictive models.
3 Return to sport: definitions and consensus
Concepts such as return to preinjury levels in terms of training and competition participation after injury are naturally difficult to consistently define. The terms RTS, return to play, return to performance and others have been often used interchangeably to describe several stages of reconditioning following injury (Doege et al., 2021). Most studies use the term RTS when the athlete competes again in a game, while other variants refer to it as returning to training and/or explicitly defined competition levels and objectives (Doege et al., 2021). Unfortunately, this lack of consensus can lead to inaccurate comparisons, making it difficult to manage patient expectations and recovery (Kyritsis et al., 2016; Wiggins et al., 2016; Doege et al., 2021).
Returning to preinjury levels of performance is the primary measure of success from the athlete’s standpoint (Palmer et al., 2021). Historically, RTS has often been considered a single end point, reached when the athlete returns to competition or game. However, it is recently recognized as a more complex and gradual process starting when the athlete return to training and up to the point of return to previous levels of performance (Ardern et al., 2016a; Snyders et al., 2023). This can be seen as a continuum that is paralleled with recovery and rehabilitation (Ardern et al., 2016a), and in which the effects of injury and their alleviation should be actively incorporated to the ongoing training.
A recent consensus statement described the RTS continuum and identified three elements or stages: 1) return to participation, such as modified training, including ongoing rehabilitation, but not been able to return to competitive sport; 2) RTS, characterized by returning back to the same sport, but not returning back to previous levels of performance; 3) return to performance, describing the resumption of sport performing at or above his or her preinjury level (Ardern et al., 2016a). A similar, slightly different model suggested four distinct phases of RTS progression including: 1) on-field rehabilitation; 2) return to training; 3) return to competitive match play; 4) return to performance (Buckthorpe et al., 2019). Recognizing RTS as a continuum provides several advantages, primarily by allowing the RTS stakeholders to better understand and frame this process correctly. Identifying and achieving the milestones described above can then be used as is or adapted to suit individual cases and needs. Using the term RTS in the context of a continuum, or “RTS continuum” as an umbrella term, could provide much needed consistency in the literature. Here, we have chosen the first option, “RTS”, in line with recent literature (Ardern et al., 2016a; Doege et al., 2021).
4 Return to sport frameworks
RTS is influenced by a multitude of physical and non-physical factors. Conceptualisation of the connectivity between different factors are one important way to empower and inform the team around the athlete of what influences RTS (Ardern et al., 2016a). The development of such frameworks helps to understand and guide the RTS process by promoting consistency in decision making and minimizing risk.
The three-step decision-based model was introduced in 2010 (Creighton et al., 2010) and improved upon later, with the Strategic Assessment of Risk and Risk Tolerance (StARRT) (Shrier, 2015). This framework helps to estimate the risks of different short-term and long-term outcomes associated with RTS. It introduces generic standardizations and factors that should be considered by the RTS decision maker for risk assessment. The biopsychosocial model includes biological, psychological, and social factors and has been repurposed for the context of sport injury (Wiese-Bjornstal et al., 1998; Ardern et al., 2016b). For instance, after sustaining an injury, 75.5% of retired elite athletes indicated that they put pressure on themselves to return to sport as quickly as possible, followed by pressure from coaches (33.6%), sport governing body (15.5%) and teammates (13.6%) (Palmer et al., 2021). This can influence treatment decisions and outcomes after injury and therefore affect the RTS process. The Goldilocks approach uses the “acute to chronic load ratio” to permit a quantification of an athlete’s risk of subsequent injury (Blanch and Gabbett, 2016; Gabbett et al., 2016). The quintessence is that gradual increases in overall fitness (chronic load) should be sufficient to overcome sudden fatigue demands (acute load). This ratio can be a useful tool in planning load progressions in order to optimally prepare athletes for competition, minimize the risk of injury, as well as, during the RTS continuum. Another consideration is possible neuroplastic disruptions (e.g., after anterior cruciate ligament rupture) after injury, which might affect motor coordination and result in altered motor strategies becoming the norm (Lepley et al., 2015; Grooms and Myer, 2017). The “control-chaos continuum” proposes that the recovery sessions move from high control to high chaos, prescribing running loads under increasingly riskier conditions (Taberner et al., 2019). This is achieved by progressively incorporating greater perceptual and reactive neurocognitive challenges (Grooms and Myer, 2017; Taberner et al., 2019).
Lastly, it is important to frame RTS as a risk management endeavor with the risk being the inability to perform at equivalent or higher preinjury levels of performance. Injury risk management models in sports have been mostly established with a preventive mindset towards injury (van Mechelen et al., 1992; Roe et al., 2017), and already identified the need for an individualized approach (Roe et al., 2017). Therefore, a risk management perspective for RTS would allow the RTS stakeholders to reach decisions based on set criteria and lay the foundation to develop objective models of assessment. Such models could drive individualization on risk assessment and management.
5 Assessment of the return to sport progress
Evaluating the progress of RTS has proved equally challenging and the need for development and use of validated and reliable RTS assessment tools has been clearly stated (Ardern et al., 2016a; Losciale et al., 2019; Taberner, 2020; Marom et al., 2022). Marom and others demonstrated a high variability in defining, evaluating and reporting patterns of RTS after anterior cruciate ligament reconstruction (Marom et al., 2022). Patient reported outcomes provide a subjective way to assess the ongoing rehabilitation process and help understanding the level of readiness in athletes following injury (Ruzbarsky et al., 2018; Nelson et al., 2019; Marom et al., 2020). In comparison to validated patient reported outcomes (Ruzbarsky et al., 2018; Marom et al., 2020; Nelson et al., 2020; Hoenig et al., 2021) there is a lack of standardization and validation of proven RTS assessment tools (Marom et al., 2022). This limits the reliability, accuracy and overall comparability of RTS outcomes. Taken together with the high probability of reinjury in recovering athletes, it necessitates the development or usage of objective and reliable tools to accompany RTS stakeholders throughout the RTS spectrum.
Quantitative assessment of motor function has become increasingly important in clinical practice over the years. Functional assessments have been recently proposed (Hildebrandt et al., 2015) and validated (Herbst et al., 2015) in RTS after anterior cruciate ligament reconstruction, including stability tests, countermovement jumps, plyometric jumps and others (Barber-Westin and Noyes, 2011; Hildebrandt et al., 2015), however, without the use of standardized biomechanical equipment. Trasolini et al., proposed objective measurements using cameras in order to determine the range of motion in throwing athletes, during the RTS process (Trasolini et al., 2022). While this is undoubtedly a step in the right direction, such approaches have been rare and have mostly been used to test the progress of the rehabilitation without providing specific feedback on where possible limitations may arise. Further, the lack of normative, population-wide values hinders the interpretation of these outcomes.
6 Biofeedback-based return to sport: Perspectives and opportunities
The RTS research field has been continuously developing and expanding over the last years, but also faces several challenges ahead. Here, we identified unsuccessful load management and rehabilitation as major contributors to injury, a lack of consensus in the RTS terminology, presented the main frameworks that aim to guide RTS and the need for more objective and quantitative assessments. Below we argue that wider adoption and systematic use of biomechanical assessments-especially in functional settings (e.g., during running or throwing), could expand or even enhance existing RTS frameworks by enabling more rigorous quantitative assessments, which in turn could be of benefit to the overall RTS process.
Recent advances in sensor sciences have increased the opportunities for accurate, wireless and long-lasting capturing of data. Assessment methods originating from the field of biomechanics can objectively increase our understanding of healthy and pathological movement based on the analysis of kinematic (the study of the motion of bodies in space with respect to time) and kinetic (the study of the forces associated with motion) parameters (Winter 1987; Bartlett, 2007). Physiological signals pertaining the human neural circuit, such as, the electric activity of muscles and brain have also been captured to provide insights into movement (Bizzi et al., 2008; Gwin et al., 2011; Kesić and Spasić, 2016; Taborri et al., 2020). Equipment commonly used in biomechanical testing such as cameras, force plates, electromyography, wearable inertial sensors and others can be, hence, used during the RTS continuum for quantitative assessment and for detecting changes between measurement sessions in standardized settings (Pappas et al., 2016; Hainline et al., 2017). However, in RTS the biological recovery of the affected tissue must be accompanied with adequate functionality, which constitutes a much greater challenge.
Running, jumping and throwing, which are predominant in most athletic disciplines, are uniquely advantageous to be examined with biomechanical equipment from a functional perspective. Running analysis, due to movements being stereotyped and/or cyclic, can be streamlined to produce quick assessments and comparisons. Multiple force plates, cameras and wearable sensors can be used to capture large amounts of data in an on-the-field setting. Parameters, such as spatiotemporal variables, force profiles (e.g., the way forces are shaped during the time that the foot is in contact with the ground), a wide spectrum of kinematic variables (e.g., range of motion, vertical displacement) and the contribution to mechanical power from different joints have been often used to assess performance changes (Taylor, 1985; Arampatzis et al., 2000; Angelozzi et al., 2012; Santuz et al., 2016; Ekizos et al., 2021). It is therefore possible that these metrics would provide unique insights to the RTS process. However, their effectiveness and validity in the context of RTS has yet to be systematically examined. One limitation is the difficulty of direct quantitative comparisons with preinjury levels of performance. However, extending the usage of such metrics in routine preinjury baseline measurements, could enable comparisons during the recovery protocol, or normative values could be established and utilized, where applicable.
It has long been suggested that the ability to reliably quantify lower-extremity biomechanical variables during dynamic tasks could reveal mechanisms related to injury risk factors (Ford et al., 2007). Indeed, it has been shown that certain biomechanical profiles are associated with higher incidence of anterior cruciate ligament injury in high school female athletes (Pappas et al., 2016). A full-body approach to biomechanical assessment has been also suggested for the examination of pain in the elite athlete (Hainline et al., 2017). Interestingly, this has not been systematically examined in RTS settings. We argue that information of force and kinematic profiles (e.g., distinguishing the contribution and distribution of power in the involved joints during running) could be invaluable in identifying weaknesses in the recovering athlete and introducing specific exercises to alleviate them.
There is amounting evidence that load management, is a prominent mechanism which could be important not only to predict injury, but also to guide RTS (i.e., acute to chronic load ratio) (Blanch and Gabbett, 2016; Soligard et al., 2016; Eckard et al., 2018). However, a one-size-fits-all approach does not address the variability existing between injuries, players, competitive demands and response to loading. Rehabilitation and training practice as it approaches and enters the RTS has to be targeted and, thus, highly individualized. Timing is especially important, due to affecting load perception. Fitness levels, body composition and playing level often fluctuate with time (Jones et al., 2017). Together with age and history of injuries these characteristics have a significant impact on the perception and experienced stress by the body. All change constantly, are highly individual and can drastically affect the risk of injury (Ekstrand et al., 2011; Zwerver et al., 2011; Rogalski et al., 2013; Jones et al., 2017). These characteristics are equally influencing the athlete before, during and after an injury occurs and can, thus, affect the progress of the rehabilitation and the risk of reinjury. Kinetic and kinematic analyses could deliver information on how the applied force is distributed (e.g., using force plates during walking and running), identifying compensatory patterns (e.g., motion analysis in throwing tasks). Biomechanical assessments during functional movements could, thus, enable a highly customizable approach in terms of load management by monitoring and accounting for fluctuations in individual characteristics.
During RTS-besides increasing load, the human body has to constantly incorporate new information about its state (ongoing rehabilitation process) and navigate increasingly demanding tasks. Biological systems have the property of robustness, defined as the ability to maintain function despite internal and external perturbations (Kitano, 2004). Training in the presence of external perturbations improves muscle strength, stability, and balance performance (Hamed et al., 2018; Bohm et al., 2020; McCrum et al., 2022) and the progressive incorporation of highly variable, spontaneous, and unanticipated movements has been proposed in the context of RTS (Taberner et al., 2019). Human movement reveals the hallmark characteristics of complex systems (Mayer-Kress et al., 2006). The broad term “complexity” can be used to define the emergence of different (i.e., more or less complex) strategies (Dusing and Harbourne, 2010; Bisi and Stagni, 2018) to accomplish a specific motor task and enrich the training process. Maintaining functionality despite increased complexity is a challenge for the system and could induce beneficial neuromuscular adaptations. In this context, this approach could be beneficial to the recovery process of the elite athlete. Fine-tuning the range and amplitude of training complexity through objective biomechanical measurements means that this process could be more individualized, taking into account specific needs and performance goals.
We propose that biomechanical assessments would enable multiple biofeedback cycles, and, thus, a biofeedback-based approach in RTS. This approach could work independently, but also complimentary to existing RTS frameworks by providing quantitative and objective assessments. Further, it could facilitate interactions with the clinical stakeholders of the RTS process (e.g., identification of ankle instabilities through kinematic profiles or lower activation of specific muscles through electromyography), driving further clinical assessments. We argue that when employed to the RTS continuum, these biofeedback cycles could drive effective individualization. These assessments should aim to: a) identify weaknesses in kinetic and kinematic parameters, b) monitor load and complexity, c) compare current state of performance to inter- and intrapersonal values. Consequently, adjustments to the training program should be introduced. This approach accentuates the need for regular objective assessments of the athlete during the RTS continuum and the incorporation of this biofeedback to customize the load and complexity of the trainings (Figure 1).
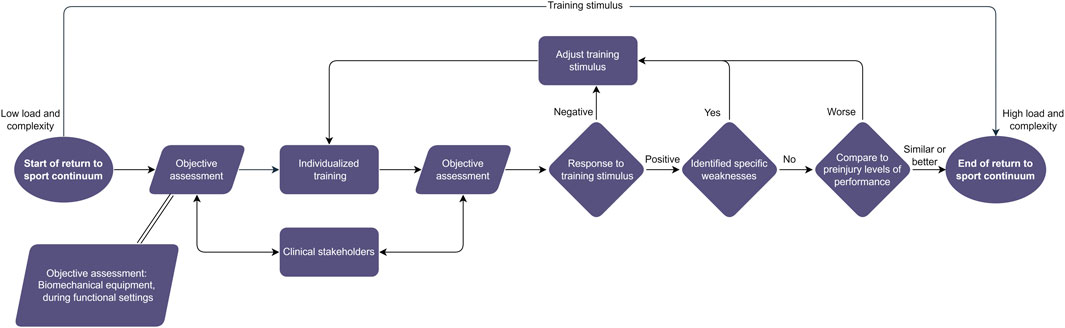
FIGURE 1. The biofeedback-based return to sport (RTS) approach. This approach places objective assessments at the core of the RTS process and applies the gathered information to inform it. The interaction with other RTS stakeholders should be emphasized. In the context of this paper objective assessments are meant as evaluations through biomechanical equipment utilized during functional settings.
Future studies should also consider a more holistic approach to RTS, by incorporating assessments of motor control. To maintain functionality during the execution of complex movements, humans rely on reflexes and sensory feedback to produce accurate, coordinated actions (Bernstein, 1967; Biewener and Daley, 2007; Grillner and El Manira, 2020). This is achieved through a modular interplay between muscles, sensory organs, and the central nervous system, which interact to produce meaningful, dynamically stable movement (Nishikawa et al., 2007). Knowing how body kinematics or muscle activation patterns behave during the production of simple repetitive activities such as locomotion is sufficient to understand the behavior of the system and quantify its ability to withstand perturbations (Dingwell and Cusumano, 2000; Ekizos et al., 2017; Santuz et al., 2018). Due to the nature of the information sought (i.e., related to motor control), these measurements often require a large number of repetitive movements. Athletic disciplines are, therefore, especially suitable since they require repetitive movement patterns or explosive movements executed with high frequency. We have recently demonstrated that measures of stability and analyses of muscle activation patterns are sufficiently accurate to confirm changes between speeds and conditions (Ekizos et al., 2018a; Santuz et al., 2018; Santuz and Akay, 2020), but also between different running techniques and adaptations to running training programs (Ekizos et al., 2017; 2018a). Such approaches are increasingly accessible (Santuz, 2022) and reliable (Santuz et al., 2017; Ekizos et al., 2018b; Fohrmann et al., 2022) and may prove valuable tools in assessing neuromuscular aspects pertaining to motor control after injury as well.
7 Conclusion
In the current manuscript we have seen that with high prevalence rates, injuries are part of elite sports and is often part of the athlete’s career in multiple ways. Further, that the management of the period after the injury is crucial for an effective RTS. There is a need for objective and reliable RTS assessment tools in order to encourage more quantitative assessments. In the ongoing discussion of how to improve the RTS process, we argue that biofeedback cycles using objective assessments could drive an effective and individualised RTS. The incorporation of biomechanical parameters derived during functional settings seems ideal for this purpose. The development of regular standardized measurement procedures could enhance the applicability of this approach by establishing baseline and normative values.
Data availability statement
The original contributions presented in the study are included in the article/supplementary material, further inquiries can be directed to the corresponding authors.
Author contributions
AE: conceptualization and writing of original manuscript; AS: input, critical review and modification. All authors contributed to the article and approved the submitted version.
Acknowledgments
We thank Reiner Günzl for the valuable discussions and the Max-Delbrück-Center for Molecular Medicine in the Helmholtz Association (MDC), Berlin, Germany for covering the publication fees.
Conflict of interest
The authors declare that the research was conducted in the absence of any commercial or financial relationships that could be construed as a potential conflict of interest.
Publisher’s note
All claims expressed in this article are solely those of the authors and do not necessarily represent those of their affiliated organizations, or those of the publisher, the editors and the reviewers. Any product that may be evaluated in this article, or claim that may be made by its manufacturer, is not guaranteed or endorsed by the publisher.
References
Alonso, J.-M., Edouard, P., Fischetto, G., Adams, B., Depiesse, F., and Mountjoy, M. (2012). Determination of future prevention strategies in elite track and field: Analysis of daegu 2011 IAAF championships injuries and illnesses surveillance. Br. J. Sports Med. 46, 505–514. doi:10.1136/bjsports-2012-091008
Angelozzi, M., Madama, M., Corsica, C., Calvisi, V., Properzi, G., McCaw, S. T., et al. (2012). Rate of force development as an adjunctive outcome measure for return-to-sport decisions after anterior cruciate ligament reconstruction. J. Orthop. Sports Phys. Ther. 42, 772–780. doi:10.2519/jospt.2012.3780
Arampatzis, A., Knicker, A., Metzler, V., and Brüggemann, G. P. (2000). Mechanical power in running: A comparison of different approaches. J. Biomech. 33, 457–463. doi:10.1016/s0021-9290(99)00187-6
Ardern, C. L., Glasgow, P., Schneiders, A., Witvrouw, E., Clarsen, B., Cools, A., et al. (2016a). 2016 consensus statement on return to sport from the first world congress in sports physical therapy, bern. Br. J. Sports Med. 50, 853–864. doi:10.1136/bjsports-2016-096278
Ardern, C. L., Kvist, J., and Webster, K. E. (2016b). Psychological aspects of anterior cruciate ligament injuries. Operative Tech. Sports Med. 24, 77–83. doi:10.1053/j.otsm.2015.09.006
Ardern, C. L., Taylor, N. F., Feller, J. A., and Webster, K. E. (2014). Fifty-five per cent return to competitive sport following anterior cruciate ligament reconstruction surgery: An updated systematic review and meta-analysis including aspects of physical functioning and contextual factors. Br. J. Sports Med. 48, 1543–1552. doi:10.1136/bjsports-2013-093398
Barber-Westin, S. D., and Noyes, F. R. (2011). Objective criteria for return to athletics after anterior cruciate ligament reconstruction and subsequent reinjury rates: A systematic review. Physician Sportsmed. 39, 100–110. doi:10.3810/psm.2011.09.1926
Bartlett, R. (2007). Introduction to sports biomechanics: Analysing human movement patterns. 2nd ed. London: Routledge. doi:10.4324/9780203462027
Biewener, A. A., and Daley, M. A. (2007). Unsteady locomotion: Integrating muscle function with whole body dynamics and neuromuscular control. J. Exp. Biol. 210, 2949–2960. doi:10.1242/jeb.005801
Bisi, M. C., and Stagni, R. (2018). Changes of human movement complexity during maturation: Quantitative assessment using multiscale entropy. Comput. Methods Biomech. Biomed. Engin 21, 325–331. doi:10.1080/10255842.2018.1448392
Bizzi, E., Cheung, V. C. K., d’Avella, A., Saltiel, P., and Tresch, M. (2008). Combining modules for movement. Brain Res. Rev. 57, 125–133. doi:10.1016/j.brainresrev.2007.08.004
Blanch, P., and Gabbett, T. J. (2016). Has the athlete trained enough to return to play safely? The acute:chronic workload ratio permits clinicians to quantify a player’s risk of subsequent injury. Br. J. Sports Med. 50, 471–475. doi:10.1136/bjsports-2015-095445
Bohm, S., Mandla-Liebsch, M., Mersmann, F., and Arampatzis, A. (2020). Exercise of dynamic stability in the presence of perturbations elicit fast improvements of simulated fall recovery and strength in older adults: A randomized controlled trial. Front. Sports Act. Living 2, 52. doi:10.3389/fspor.2020.00052
Bouchard, C., and Rankinen, T. (2001). Individual differences in response to regular physical activity. Med. Sci. Sports Exerc 33, S446–S451. discussion S452-453. doi:10.1097/00005768-200106001-00013
Buckthorpe, M., Frizziero, A., and Roi, G. S. (2019). Update on functional recovery process for the injured athlete: Return to sport continuum redefined. Br. J. Sports Med. 53, 265–267. doi:10.1136/bjsports-2018-099341
Creighton, D. W., Shrier, I., Shultz, R., Meeuwisse, W. H., and Matheson, G. O. (2010). Return-to-Play in sport: A decision-based model. Clin. J. Sport Med. 20, 379–385. doi:10.1097/JSM.0b013e3181f3c0fe
Dingwell, J. B., and Cusumano, J. P. (2000). Nonlinear time series analysis of normal and pathological human walking. Chaos 10, 848–863. doi:10.1063/1.1324008
Doege, J., Ayres, J. M., Mackay, M. J., Tarakemeh, A., Brown, S. M., Vopat, B. G., et al. (2021). Defining return to sport: A systematic review. Orthop. J. Sports Med. 9, 23259671211009589. doi:10.1177/23259671211009589
Drew, M. K., and Finch, C. F. (2016). The relationship between training load and injury, illness and soreness: A systematic and literature review. Sports Med. 46, 861–883. doi:10.1007/s40279-015-0459-8
Dusing, S. C., and Harbourne, R. T. (2010). Variability in postural control during infancy: Implications for development, assessment, and intervention. Phys. Ther. 90, 1838–1849. doi:10.2522/ptj.2010033
Eckard, T. G., Padua, D. A., Hearn, D. W., Pexa, B. S., and Frank, B. S. (2018). The relationship between training load and injury in athletes: A systematic review. Sports Med. 48, 1929–1961. doi:10.1007/s40279-018-0951-z
Edouard, P., Dandrieux, P.-E., Chapon, J., Prince, C., Charpy, S., Bruneau, A., et al. (2022). One-season epidemiology of injury complaints in athletics (track and field). Dtsch. Z Sportmed 73, 215–220. doi:10.5960/dzsm.2022.544
Ekizos, A., Santuz, A., and Arampatzis, A. (2021). Runners employ different strategies to cope with increased speeds based on their initial strike patterns. Front. Physiology 12, 686259. doi:10.3389/fphys.2021.686259
Ekizos, A., Santuz, A., and Arampatzis, A. (2018a). Short- and long-term effects of altered point of ground reaction force application on human running energetics. J. Exp. Biol. 221, jeb176719. doi:10.1242/jeb.176719
Ekizos, A., Santuz, A., and Arampatzis, A. (2017). Transition from shod to barefoot alters dynamic stability during running. Gait Posture 56, 31–36. doi:10.1016/j.gaitpost.2017.04.035
Ekizos, A., Santuz, A., Schroll, A., and Arampatzis, A. (2018b). The maximum lyapunov exponent during walking and running: Reliability assessment of different marker-sets. Front. Physiol. 9, 1101. doi:10.3389/fphys.2018.01101
Ekstrand, J., Hägglund, M., and Waldén, M. (2011). Epidemiology of muscle injuries in professional football (soccer). Am. J. Sports Med. 39, 1226–1232. doi:10.1177/0363546510395879
Feddermann-Demont, N., Junge, A., Edouard, P., Branco, P., and Alonso, J.-M. (2014). Injuries in 13 international Athletics championships between 2007–2012. Br. J. Sports Med. 48, 513–522. doi:10.1136/bjsports-2013-093087
Fohrmann, D., Hamacher, D., Sanchez-Alvarado, A., Potthast, W., Mai, P., Willwacher, S., et al. (2022). Reliability of running stability during treadmill and overground running. Sensors (Basel) 23, 347. doi:10.3390/s23010347
Ford, K. R., Myer, G. D., and Hewett, T. E. (2007). Reliability of landing 3D motion analysis: Implications for longitudinal analyses. Med. Sci. Sports Exerc 39, 2021–2028. doi:10.1249/mss.0b013e318149332d
Gabbett, T. J., Hulin, B. T., Blanch, P., and Whiteley, R. (2016). High training workloads alone do not cause sports injuries: How you get there is the real issue. Br. J. Sports Med. 50, 444–445. doi:10.1136/bjsports-2015-095567
Galambos, S. A., Terry, P. C., Moyle, G. M., Locke, S. A., and Lane, A. M. (2005). Psychological predictors of injury among elite athletes. Br. J. Sports Med. 39, 351–354. doi:10.1136/bjsm.2005.018440
Grillner, S., and El Manira, A. (2020). Current principles of motor control, with special reference to vertebrate locomotion. Physiol. Rev. 100, 271–320. doi:10.1152/physrev.00015.2019
Grindem, H., Snyder-Mackler, L., Moksnes, H., Engebretsen, L., and Risberg, M. A. (2016). Simple decision rules can reduce reinjury risk by 84% after ACL reconstruction: The Delaware-oslo ACL cohort study. Br. J. Sports Med. 50, 804–808. doi:10.1136/bjsports-2016-096031
Grooms, D. R., and Myer, G. D. (2017). Upgraded hardware─What about the software? Brain updates for return to play following ACL reconstruction. Br. J. Sports Med. 51, 418–419. doi:10.1136/bjsports-2016-096658
Gwin, J. T., Gramann, K., Makeig, S., and Ferris, D. P. (2011). Electrocortical activity is coupled to gait cycle phase during treadmill walking. NeuroImage 54, 1289–1296. doi:10.1016/j.neuroimage.2010.08.066
Hainline, B., Turner, J. A., Caneiro, J. P., Stewart, M., and Moseley, G. L. (2017). Pain in elite athletes—neurophysiological, biomechanical and psychosocial considerations: A narrative review. Br. J. Sports Med. 51, 1259–1264. doi:10.1136/bjsports-2017-097890
Hamed, A., Bohm, S., Mersmann, F., and Arampatzis, A. (2018). Follow-up efficacy of physical exercise interventions on fall incidence and fall risk in healthy older adults: A systematic review and meta-analysis. Sports Med. - Open 4, 56. doi:10.1186/s40798-018-0170-z
Herbst, E., Hoser, C., Hildebrandt, C., Raschner, C., Hepperger, C., Pointner, H., et al. (2015). Functional assessments for decision-making regarding return to sports following ACL reconstruction. Part II: Clinical application of a new test battery. Knee Surg. Sports Traumatol. Arthrosc. 23, 1283–1291. doi:10.1007/s00167-015-3546-3
Hildebrandt, C., Müller, L., Zisch, B., Huber, R., Fink, C., and Raschner, C. (2015). Functional assessments for decision-making regarding return to sports following ACL reconstruction. Part I: Development of a new test battery. Knee Surg. Sports Traumatol. Arthrosc. 23, 1273–1281. doi:10.1007/s00167-015-3529-4
Hoenig, T., Eissele, J., Strahl, A., Popp, K. L., Stürznickel, J., Ackerman, K. E., et al. (2023). Return to sport following low-risk and high-risk bone stress injuries: A systematic review and meta-analysis. Br. J. Sports Med. 57, 427–432. doi:10.1136/bjsports-2022-106328
Hoenig, T., Nelson, E. O., Troy, K. L., Wolfarth, B., Heiderscheit, B. C., and Hollander, K. (2021). Running-related injury: How long does it take? Feasibility, preliminary evaluation, and German translation of the university of Wisconsin running and recovery index. Phys. Ther. Sport 52, 204–208. doi:10.1016/j.ptsp.2021.09.007
Jacobsson, J., Timpka, T., Kowalski, J., Nilsson, S., Ekberg, J., Dahlström, Ö., et al. (2013). Injury patterns in Swedish elite athletics: Annual incidence, injury types and risk factors. Br. J. Sports Med. 47, 941–952. doi:10.1136/bjsports-2012-091651
Jones, C. M., Griffiths, P. C., and Mellalieu, S. D. (2017). Training load and fatigue marker associations with injury and illness: A systematic review of longitudinal studies. Sports Med. 47, 943–974. doi:10.1007/s40279-016-0619-5
Kelly, S., Pollock, N., Polglass, G., and Clarsen, B. (2022). Injury and illness in elite athletics: A prospective cohort study over three seasons. IJSPT 17, 420–433. doi:10.26603/001c.32589
Kesić, S., and Spasić, S. Z. (2016). Application of higuchi’s fractal dimension from basic to clinical neurophysiology: A review. Comput. Methods Programs Biomed. 133, 55–70. doi:10.1016/j.cmpb.2016.05.014
Kuipers, H., and Keizer, H. A. (1988). Overtraining in elite athletes. Review and directions for the future. Sports Med. 6, 79–92. doi:10.2165/00007256-198806020-00003
Kyritsis, P., Bahr, R., Landreau, P., Miladi, R., and Witvrouw, E. (2016). Likelihood of ACL graft rupture: Not meeting six clinical discharge criteria before return to sport is associated with a four times greater risk of rupture. Br. J. Sports Med. 50, 946–951. doi:10.1136/bjsports-2015-095908
Lai, C. C. H., Ardern, C. L., Feller, J. A., and Webster, K. E. (2018). Eighty-three per cent of elite athletes return to preinjury sport after anterior cruciate ligament reconstruction: A systematic review with meta-analysis of return to sport rates, graft rupture rates and performance outcomes. Br. J. Sports Med. 52, 128–138. doi:10.1136/bjsports-2016-096836
Lepley, A. S., Gribble, P. A., Thomas, A. C., Tevald, M. A., Sohn, D. H., and Pietrosimone, B. G. (2015). Quadriceps neural alterations in anterior cruciate ligament reconstructed patients: A 6-month longitudinal investigation. Scand. J. Med. Sci. Sports 25, 828–839. doi:10.1111/sms.12435
Losciale, J. M., Zdeb, R. M., Ledbetter, L., Reiman, M. P., and Sell, T. C. (2019). The association between passing return-to-sport criteria and second anterior cruciate ligament injury risk: A systematic review with meta-analysis. J. Orthop. Sports Phys. Ther. 49, 43–54. doi:10.2519/jospt.2019.8190
Marom, N., Xiang, W., Heath, M., Boyle, C., Fabricant, P. D., and Marx, R. G. (2020). Time interval affects physical activity scores: A comparison of the marx activity rating scale and the hospital for special surgery pediatric functional activity brief scale. Knee Surg. Sports Traumatol. Arthrosc. 28, 2619–2625. doi:10.1007/s00167-020-05895-9
Marom, N., Xiang, W., Wolfe, I., Jivanelli, B., Williams, R. J., and Marx, R. G. (2022). High variability and lack of standardization in the evaluation of return to sport after ACL reconstruction: A systematic review. Knee Surg. Sports Traumatol. Arthrosc. 30, 1369–1379. doi:10.1007/s00167-021-06594-9
Mayer-Kress, G., Liu, Y.-T., and Newell, K. M. (2006). Complex systems and human movement. Complexity 12, 40–51. doi:10.1002/cplx.20151
McCrum, C., Bhatt, T. S., Gerards, M. H. G., Karamanidis, K., Rogers, M. W., Lord, S. R., et al. (2022). Perturbation-based balance training: Principles, mechanisms and implementation in clinical practice. Front. Sports Act. Living 4, 1015394. doi:10.3389/fspor.2022.1015394
Meeuwisse, W. H. (1994). Assessing causation in sport injury: A multifactorial model. Clin. J. Sport Med. 4, 166–170. doi:10.1097/00042752-199407000-00004
Meeuwisse, W. H., Tyreman, H., Hagel, B., and Emery, C. (2007). A dynamic model of etiology in sport injury: The recursive nature of risk and causation. Clin. J. Sport Med. 17, 215–219. doi:10.1097/JSM.0b013e3180592a48
Nelson, E. O., Kliethermes, S., and Heiderscheit, B. (2020). Construct validity and responsiveness of the university of Wisconsin running injury and recovery index. J. Orthop. Sports Phys. Ther. 50, 702–710. doi:10.2519/jospt.2020.9698
Nelson, E. O., Ryan, M., AufderHeide, E., and Heiderscheit, B. (2019). Development of the university of Wisconsin running injury and recovery index. J. Orthop. Sports Phys. Ther. 49, 751–760. doi:10.2519/jospt.2019.8868
Nishikawa, K., Biewener, A. A., Aerts, P., Ahn, A. N., Chiel, H. J., Daley, M. A., et al. (2007). Neuromechanics: An integrative approach for understanding motor control. Integr. Comp. Biol. 47, 16–54. doi:10.1093/icb/icm024
Palmer, D., Cooper, D. J., Emery, C., Batt, M. E., Engebretsen, L., Scammell, B. E., et al. (2021). Self-reported sports injuries and later-life health status in 3357 retired Olympians from 131 countries: A cross-sectional survey among those competing in the games between london 1948 and PyeongChang 2018. Br. J. Sports Med. 55, 46–53. doi:10.1136/bjsports-2019-101772
Pappas, E., Shiyko, M. P., Ford, K. R., Myer, G. D., and Hewett, T. E. (2016). Biomechanical deficit profiles associated with ACL injury risk in female athletes. Med. Sci. Sports Exerc 48, 107–113. doi:10.1249/MSS.0000000000000750
Paterno, M. V., Rauh, M. J., Schmitt, L. C., Ford, K. R., and Hewett, T. E. (2014). Incidence of second ACL injuries 2 Years after primary ACL reconstruction and return to sport. Am. J. Sports Med. 42, 1567–1573. doi:10.1177/0363546514530088
Roe, M., Malone, S., Blake, C., Collins, K., Gissane, C., Büttner, F., et al. (2017). A six stage operational framework for individualising injury risk management in sport. Inj. Epidemiol. 4, 26. doi:10.1186/s40621-017-0123-x
Rogalski, B., Dawson, B., Heasman, J., and Gabbett, T. J. (2013). Training and game loads and injury risk in elite Australian footballers. J. Sci. Med. Sport 16, 499–503. doi:10.1016/j.jsams.2012.12.004
Ross, R., Goodpaster, B. H., Koch, L. G., Sarzynski, M. A., Kohrt, W. M., Johannsen, N. M., et al. (2019). Precision exercise medicine: Understanding exercise response variability. Br. J. Sports Med. 53, 1141–1153. doi:10.1136/bjsports-2018-100328
Ruzbarsky, J. J., Marom, N., and Marx, R. G. (2018). Measuring quality and outcomes in sports medicine. Clin. Sports Med. 37, 463–482. doi:10.1016/j.csm.2018.03.001
Santuz, A., and Akay, T. (2020). Fractal analysis of muscle activity patterns during locomotion: Pitfalls and how to avoid them. J. Neurophysiology 124, 1083–1091. doi:10.1152/jn.00360.2020
Santuz, A., Ekizos, A., and Arampatzis, A. (2016). A pressure plate-based method for the automatic assessment of foot strike patterns during running. Ann. Biomed. Eng. 44, 1646–1655. doi:10.1007/s10439-015-1484-3
Santuz, A., Ekizos, A., Eckardt, N., Kibele, A., and Arampatzis, A. (2018). Challenging human locomotion: Stability and modular organisation in unsteady conditions. Sci. Rep. 8, 2740. doi:10.1038/s41598-018-21018-4
Santuz, A., Ekizos, A., Janshen, L., Baltzopoulos, V., and Arampatzis, A. (2017). On the methodological implications of extracting muscle synergies from human locomotion. Int. J. Neural Syst. 27, 1750007. doi:10.1142/S0129065717500071
Santuz, A. (2022). musclesyneRgies: factorization of electromyographic data in R with sensible defaults. J. Open Source Softw. 7, 4439. doi:10.21105/joss.04439
Schwellnus, M., Soligard, T., Alonso, J.-M., Bahr, R., Clarsen, B., Dijkstra, H. P., et al. (2016). How much is too much? (Part 2) international olympic committee consensus statement on load in sport and risk of illness. Br. J. Sports Med. 50, 1043–1052. doi:10.1136/bjsports-2016-096572
Shrier, I. (2015). Strategic assessment of risk and risk tolerance (StARRT) framework for return-to-play decision-making. Br. J. Sports Med. 49, 1311–1315. doi:10.1136/bjsports-2014-094569
Snyders, C., Schwellnus, M., Sewry, N., Kaulback, K., Wood, P., Seocharan, I., et al. (2023). Symptom number and reduced preinfection training predict prolonged return to training after SARS-CoV-2 in athletes: Aware IV. Med. Sci. Sports Exerc 55, 1–8. doi:10.1249/MSS.0000000000003027
Soligard, T., Schwellnus, M., Alonso, J.-M., Bahr, R., Clarsen, B., Dijkstra, H. P., et al. (2016). How much is too much? (Part 1) international olympic committee consensus statement on load in sport and risk of injury. Br. J. Sports Med. 50, 1030–1041. doi:10.1136/bjsports-2016-096581
Soligard, T., Steffen, K., Palmer, D., Alonso, J. M., Bahr, R., Lopes, A. D., et al. (2017). Sports injury and illness incidence in the rio de Janeiro 2016 olympic summer games: A prospective study of 11274 athletes from 207 countries. Br. J. Sports Med. 51, 1265–1271. doi:10.1136/bjsports-2017-097956
Taberner, M., Allen, T., and Cohen, D. D. (2019). Progressing rehabilitation after injury: Consider the ‘control-chaos continuum. Br. J. Sports Med. 53, 1132–1136. doi:10.1136/bjsports-2018-100157
Taberner, M. (2020). Constructing a framework for return to sport in elite football (PhD academy award). Br. J. Sports Med. 54, 1176–1177. doi:10.1136/bjsports-2020-102300
Taborri, J., Keogh, J., Kos, A., Santuz, A., Umek, A., Urbanczyk, C., et al. (2020). Sport biomechanics applications using inertial, force, and emg sensors: A literature overview. Appl. Bionics Biomechanics 2020, e2041549. doi:10.1155/2020/2041549
Taylor, C. R. (1985). Force development during sustained locomotion: A determinant of gait, speed and metabolic power. J. Exp. Biol. 115, 253–262. doi:10.1242/jeb.115.1.253
Trasolini, N. A., Nicholson, K. F., Mylott, J., Bullock, G. S., Hulburt, T. C., and Waterman, B. R. (2022). Biomechanical analysis of the throwing athlete and its impact on return to sport. ASMAR 4, e83–e91. doi:10.1016/j.asmr.2021.09.027
van Mechelen, W., Hlobil, H., and Kemper, H. C. (1992). Incidence, severity, aetiology and prevention of sports injuries. A review of concepts. Sports Med. 14, 82–99. doi:10.2165/00007256-199214020-00002
Wiese-Bjornstal, D. M. (2010). Psychology and socioculture affect injury risk, response, and recovery in high-intensity athletes: A consensus statement. Scand. J. Med. Sci. Sports 20, 103–111. doi:10.1111/j.1600-0838.2010.01195.x
Wiese-bjornstal, D. M., Smith, A. M., Shaffer, S. M., and Morrey, M. A. (1998). An integrated model of response to sport injury: Psychological and sociological dynamics. J. Appl. Sport Psychol. 10, 46–69. doi:10.1080/10413209808406377
Wiggins, A. J., Grandhi, R. K., Schneider, D. K., Stanfield, D., Webster, K. E., and Myer, G. D. (2016). Risk of secondary injury in younger athletes after anterior cruciate ligament reconstruction: A systematic review and meta-analysis. Am. J. Sports Med. 44, 1861–1876. doi:10.1177/0363546515621554
Winter, D. A. (1987). The biomechanics and motor control of human gait. 4th ed. Hoboken, New Jersey, USA: John Wiley and Sons Inc.
Keywords: elite sport, locomotion, customization, biomechanical measurements, functional testing, biofeedback, athletics, neuromuscular coordination
Citation: Ekizos A and Santuz A (2023) “Biofeedback-based return to sport”: individualization through objective assessments. Front. Physiol. 14:1185556. doi: 10.3389/fphys.2023.1185556
Received: 13 March 2023; Accepted: 30 May 2023;
Published: 12 June 2023.
Edited by:
Karsten Hollander, Medical School Hamburg, GermanyReviewed by:
Michael Cassel, University of Potsdam, GermanyRamona Ritzmann, Clinic Rennbahn AG, Switzerland
Copyright © 2023 Ekizos and Santuz. This is an open-access article distributed under the terms of the Creative Commons Attribution License (CC BY). The use, distribution or reproduction in other forums is permitted, provided the original author(s) and the copyright owner(s) are credited and that the original publication in this journal is cited, in accordance with accepted academic practice. No use, distribution or reproduction is permitted which does not comply with these terms.
*Correspondence: Antonis Ekizos, YW50b25pcy5la2l6b3NAb3NwLWJlcmxpbi5kZQ==; Alessandro Santuz, YWxlc3NhbmRyby5zYW50dXpAbWRjLWJlcmxpbi5kZQ==